Abstract
1. Intracellular recordings were made from 123 tonically active medial vestibular nucleus (MVN) neurones in a horizontal slice preparation of the dorsal brainstem of the rat. On the basis of their averaged action potential shapes, the cells were classified as either type A, having a single deep after-hyperpolarization (AHP; 40/123 cells, 33%), or type B, having an early fast AHP and a delayed slow AHP (83/123 cells, 67%). The two cell types were distributed throughout the rostrocaudal extent of the MVN. 2. In type A cells TEA reduced the single deep AHP and decreased the rate of spike repolarization. Depolarizing current pulses from a hyperpolarized membrane potential elicited spikes with short plateau potentials in TEA. These persisted in Ca(2+)-free medium but were abolished along with the spontaneous activity in TTX. Ca(2+)-free medium did not affect the initial rate of repolarization but reduced the deep AHP. Apamin and carbachol had little effect. 4-Aminopyridine (4-AP) slowed spike repolarization and the AHP amplitude by a small amount. Thus, in type A cells spike repolarization and AHP appear to be mediated largely by a TEA-sensitive potassium current (presumably IK) and an apamin-insensitive Ca(2+)-activated potassium current (presumably IC). 3. The early fast AHP in type B cells was readily abolished in TEA. In seven of ten type B cells tested, the spontaneous spikes developed plateau potentials of 100-120 ms duration in 10 mM TEA, which then became 7-9 s long in Ca(2+)-free medium. In the remaining three cells, the spontaneous plateaux were 1.75-2 s long in TEA, and were reduced to 30-100 ms in Ca(2+)-free medium. TTX abolished the spontaneous spikes and plateaux. The delayed AHP was abolished by apamin, which induced irregular firing. 4-AP slowed spike repolarization and abolished the fast AHP, but did not induce plateaux. Thus, in type B cells spike repolarization involves a TEA-sensitive current (presumably IK) as well as IC and the 4-AP-sensitive potassium current IA, while the apamin-sensitive potassium current IAHP is responsible for the delayed AHP. 4. The tonic activity in type B cells appears to be regulated mainly by interactions between a persistent Na+ current, which in most cells is large enough to generate plateaux when repolarization is impeded in TEA, and the hyperpolarization mediated by IAHP. About 30% of type B cells have an additional inward Ca2+ current.(ABSTRACT TRUNCATED AT 400 WORDS)
Full text
PDF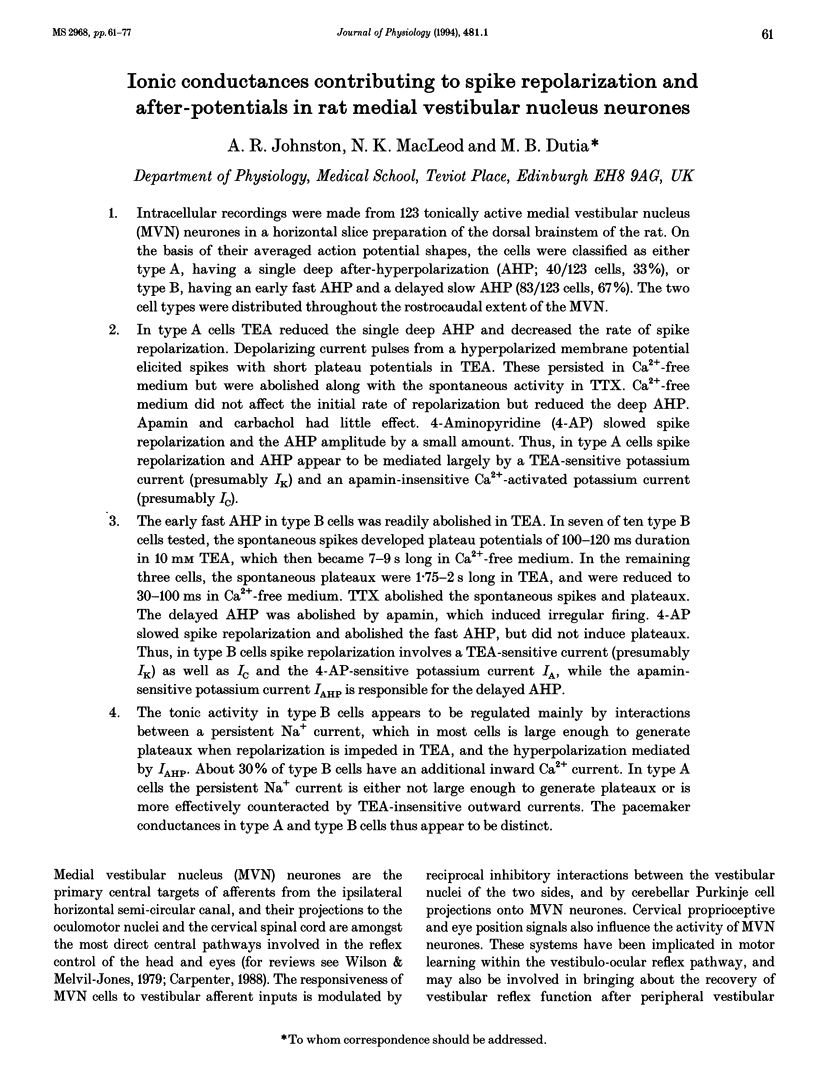
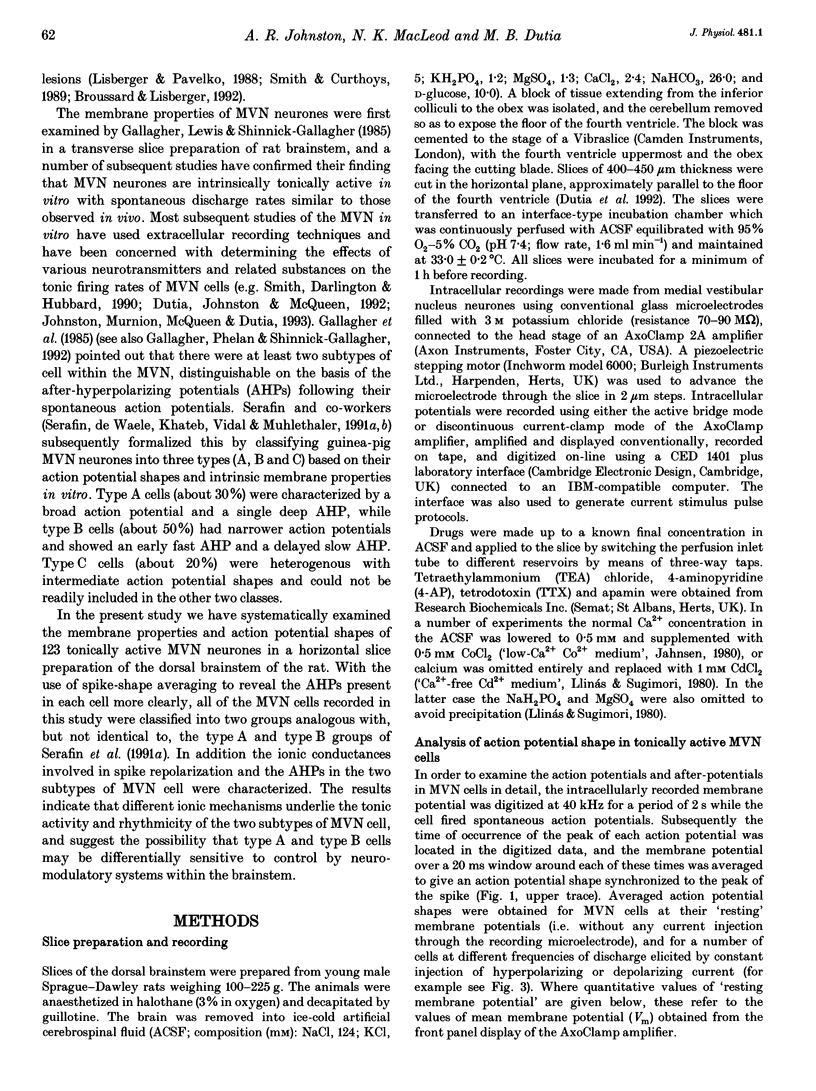
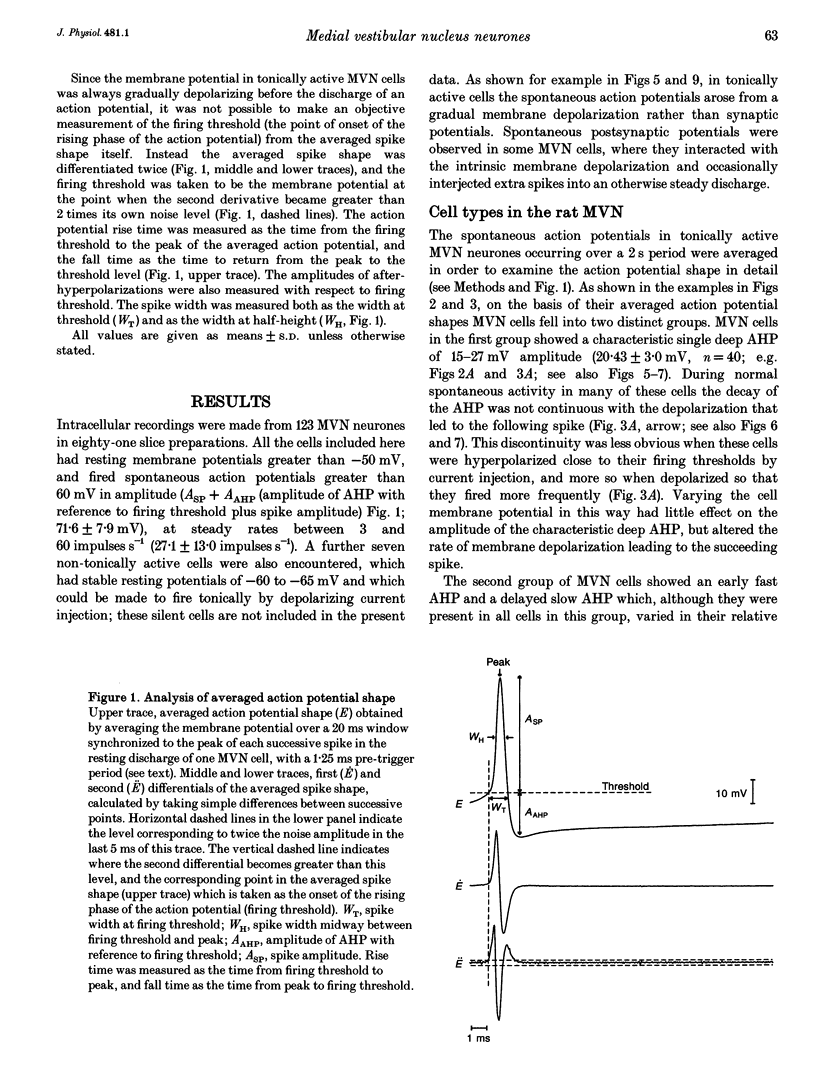
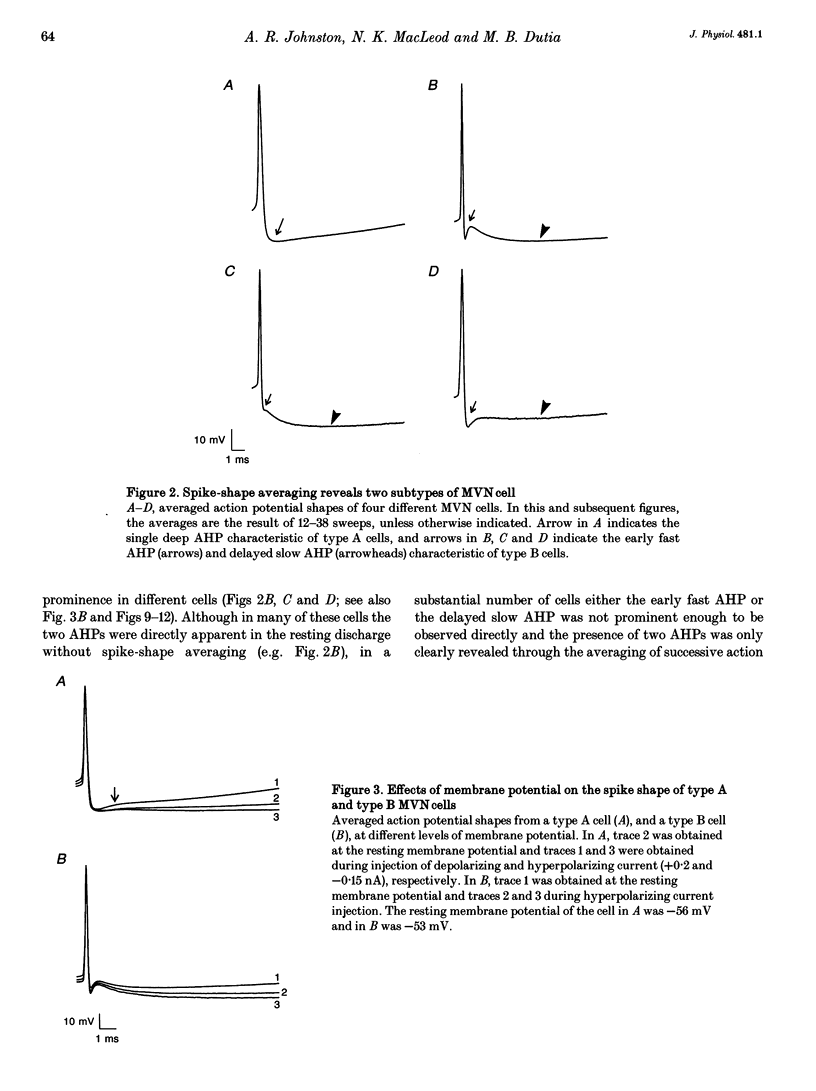
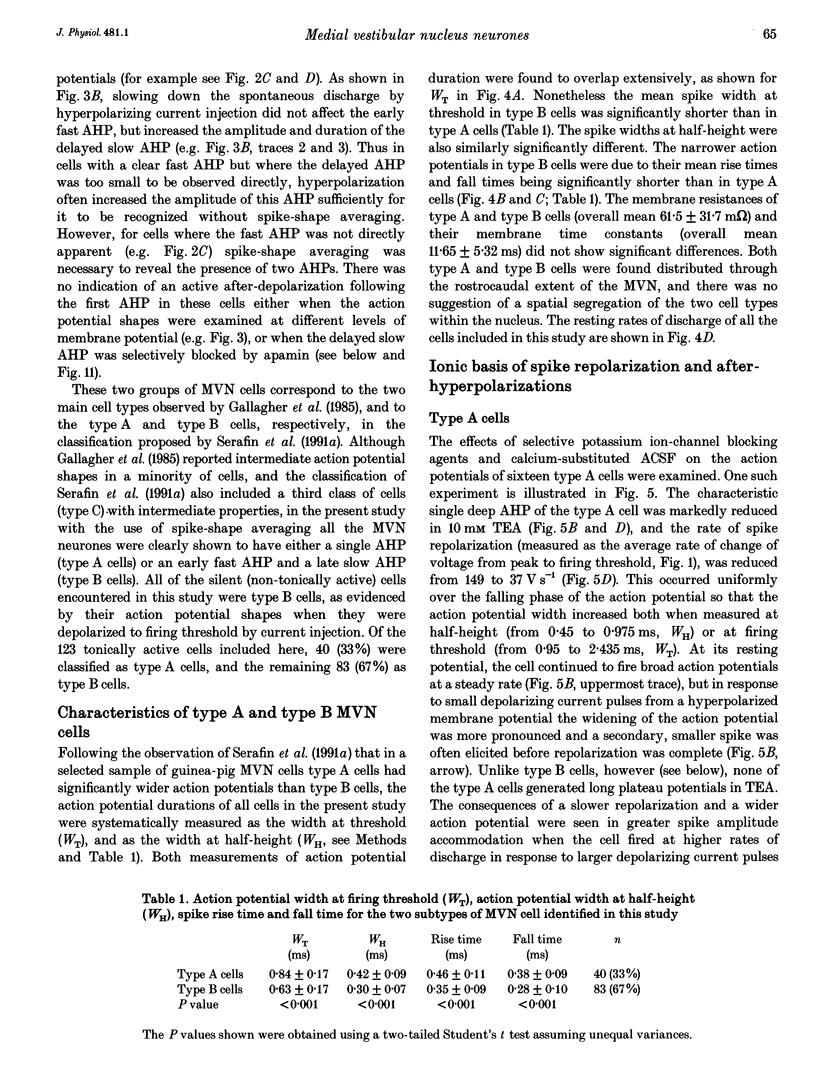
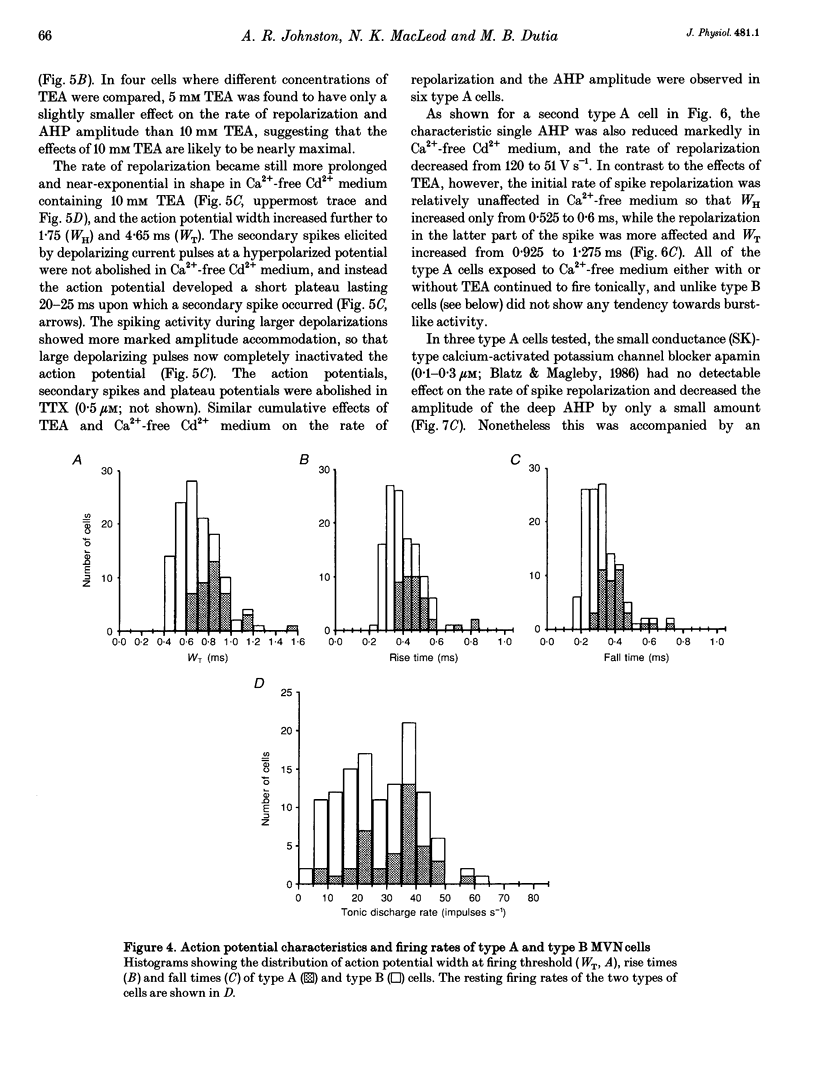
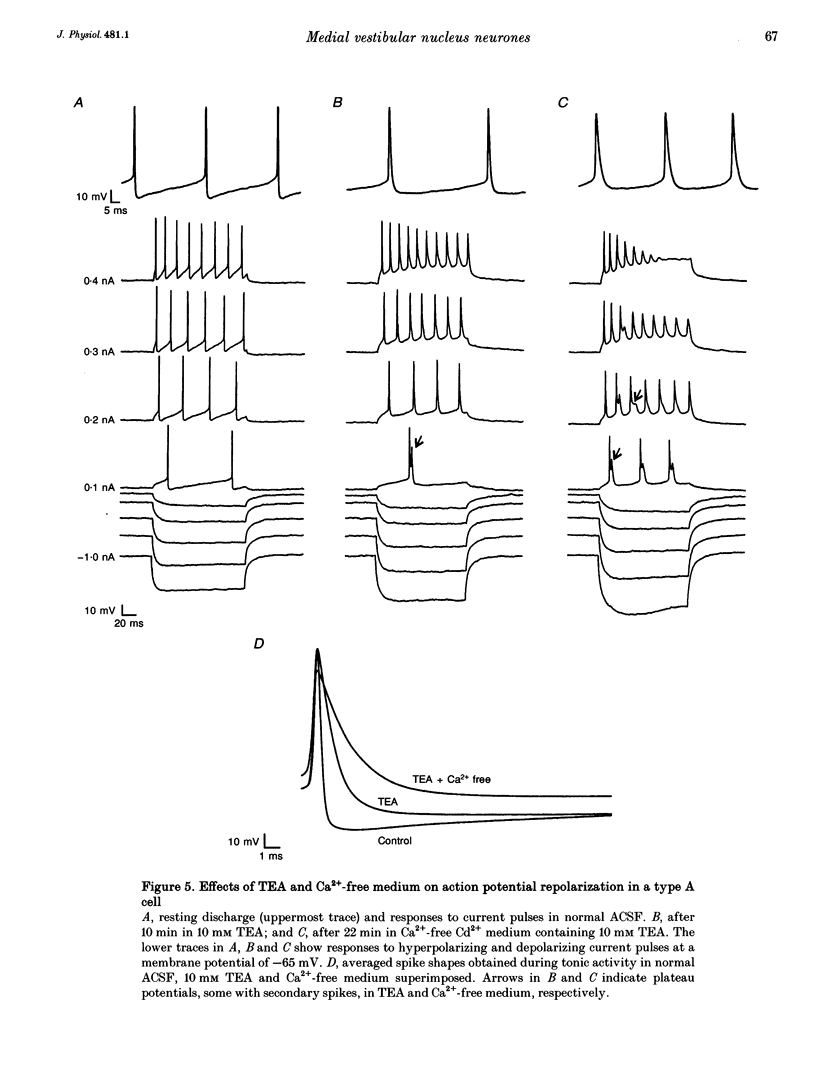
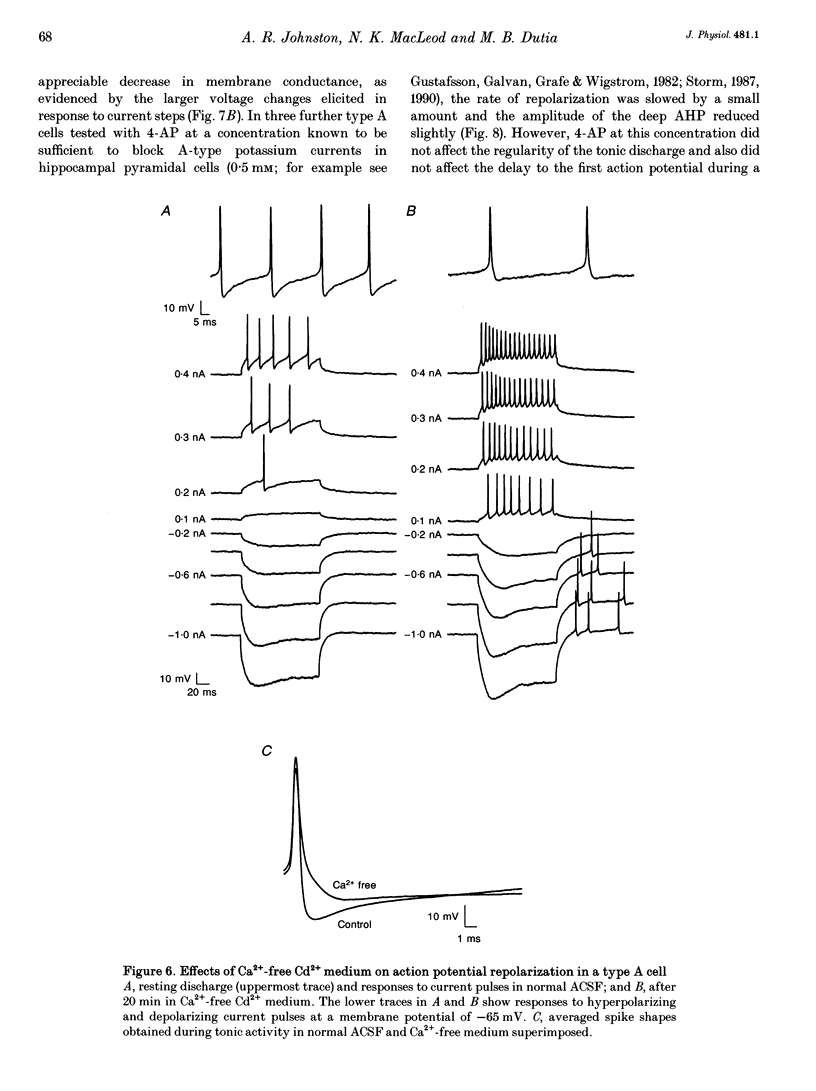
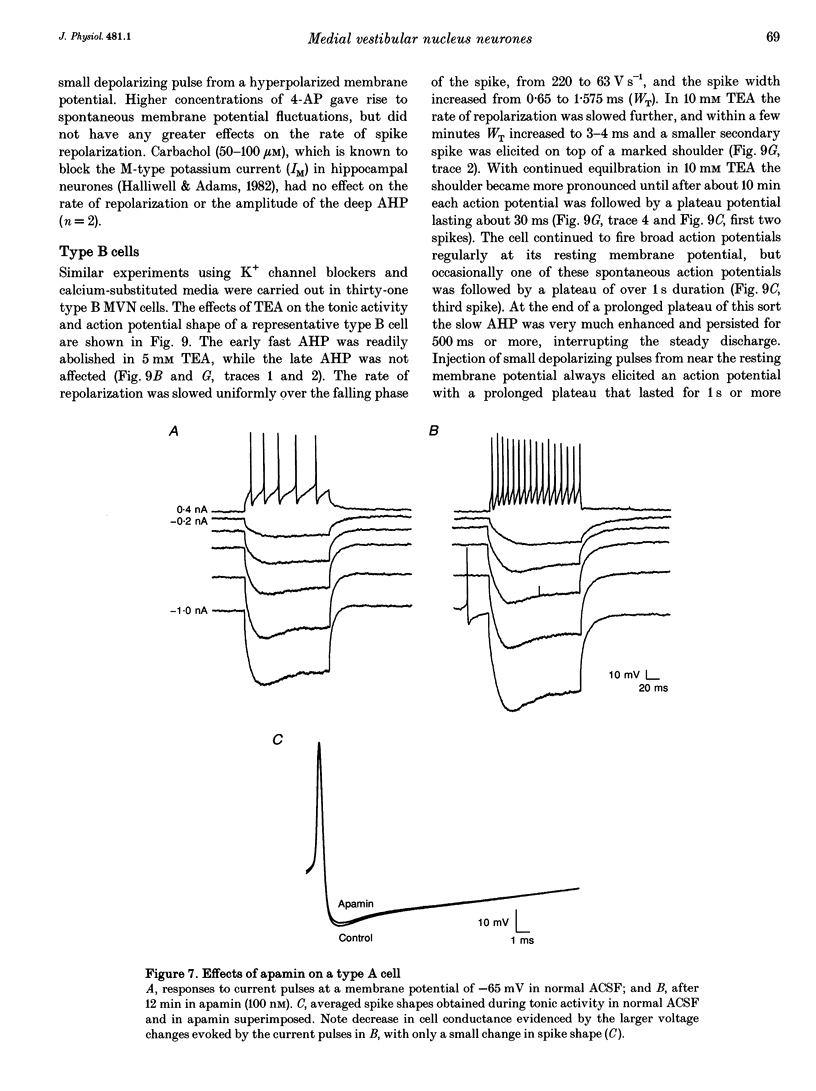
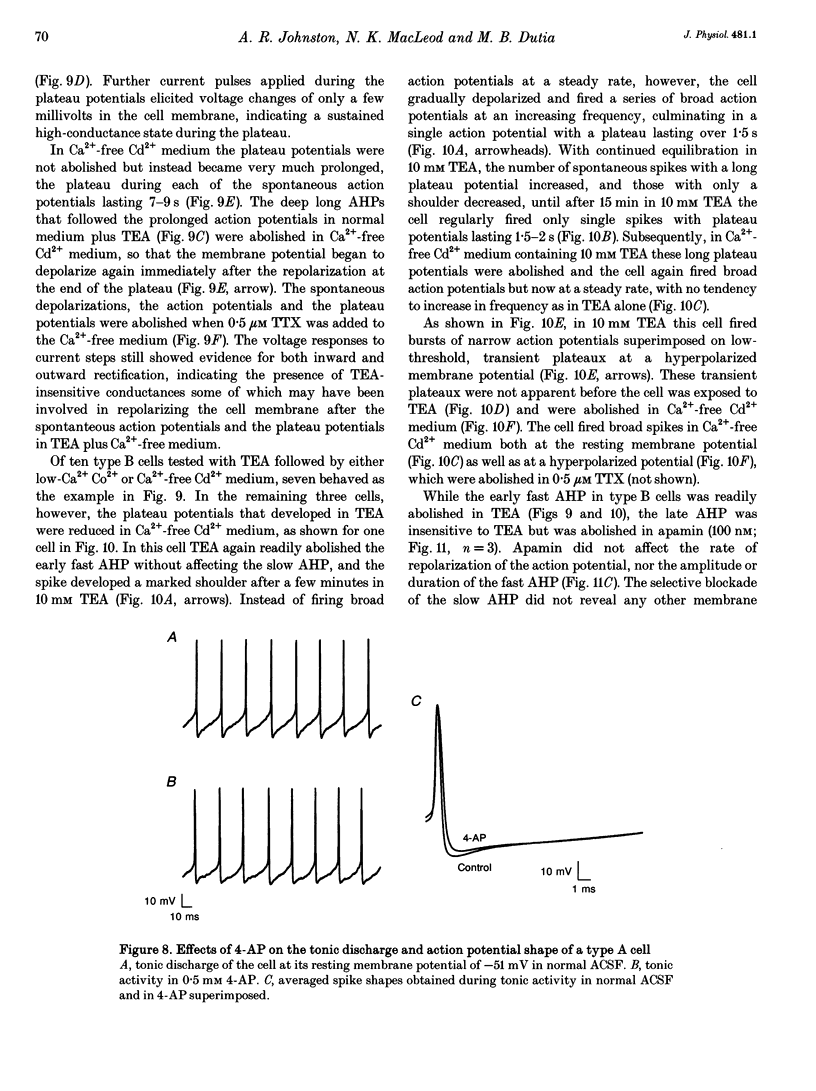
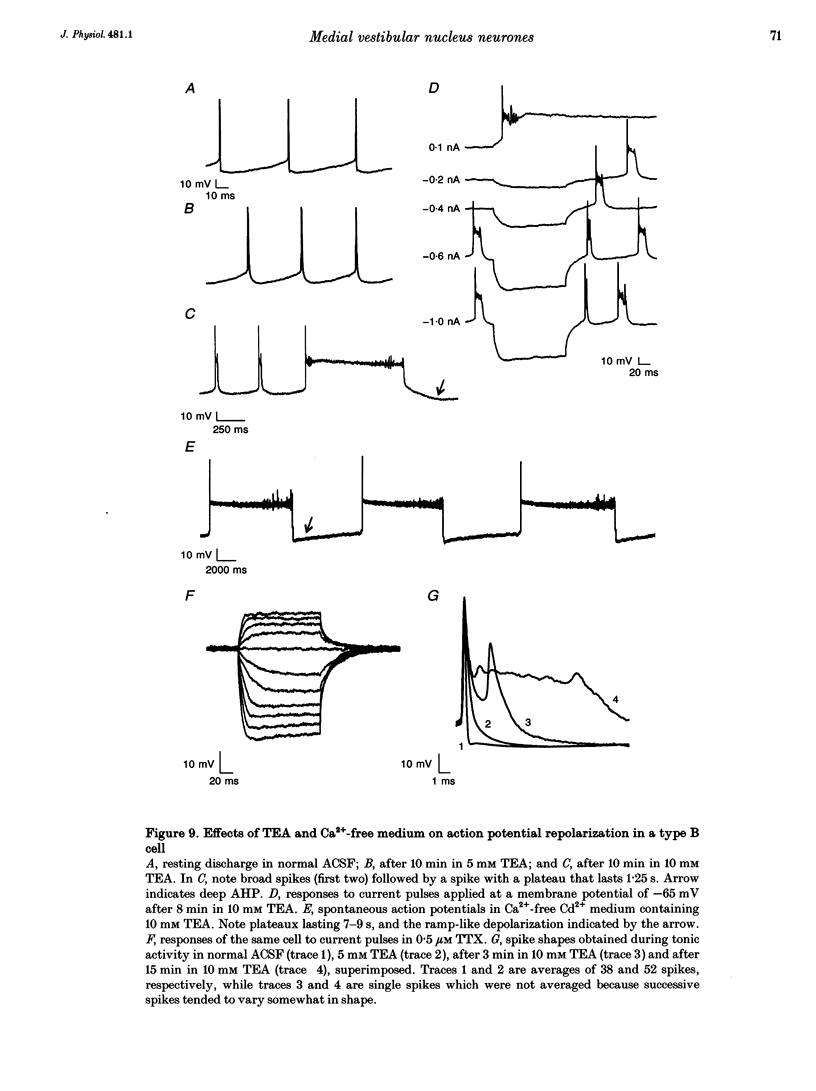
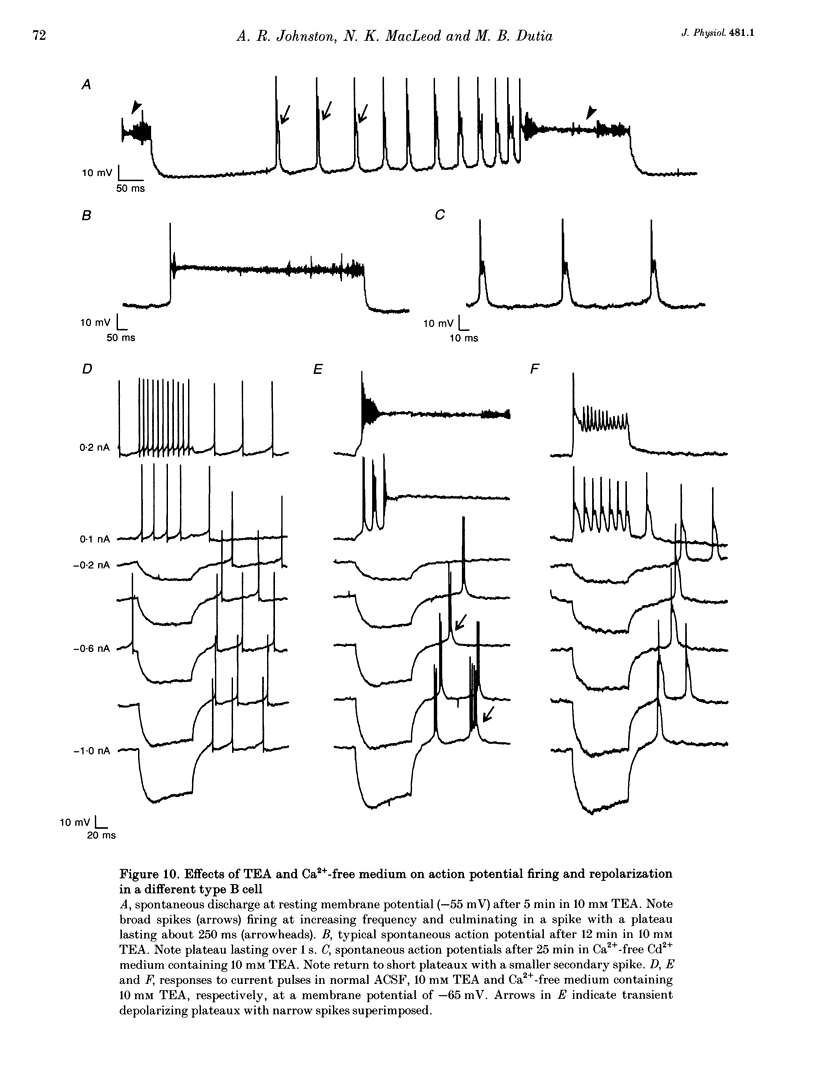
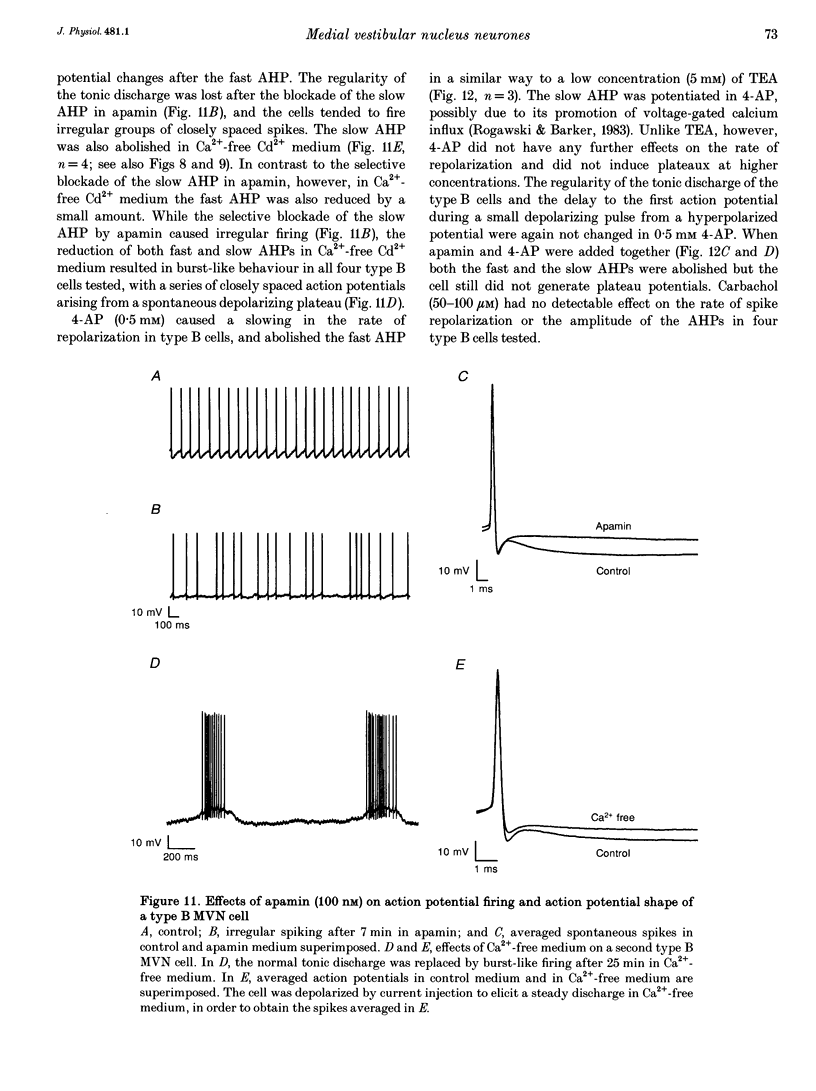
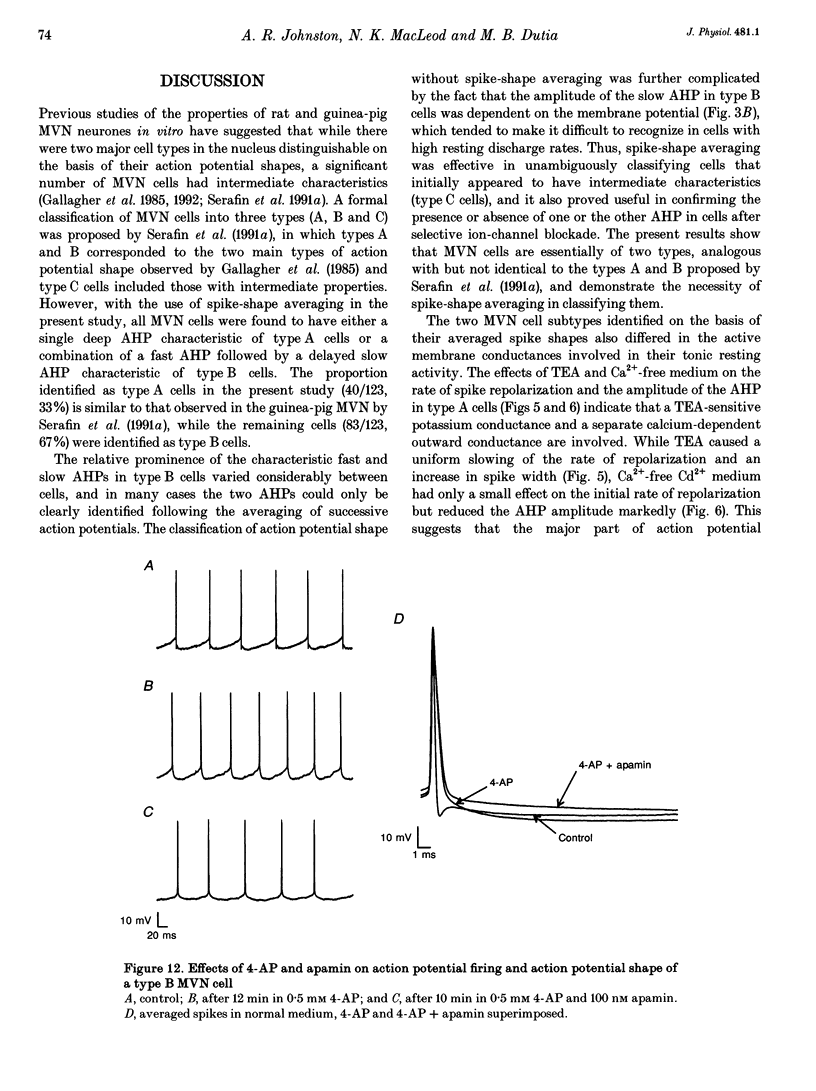
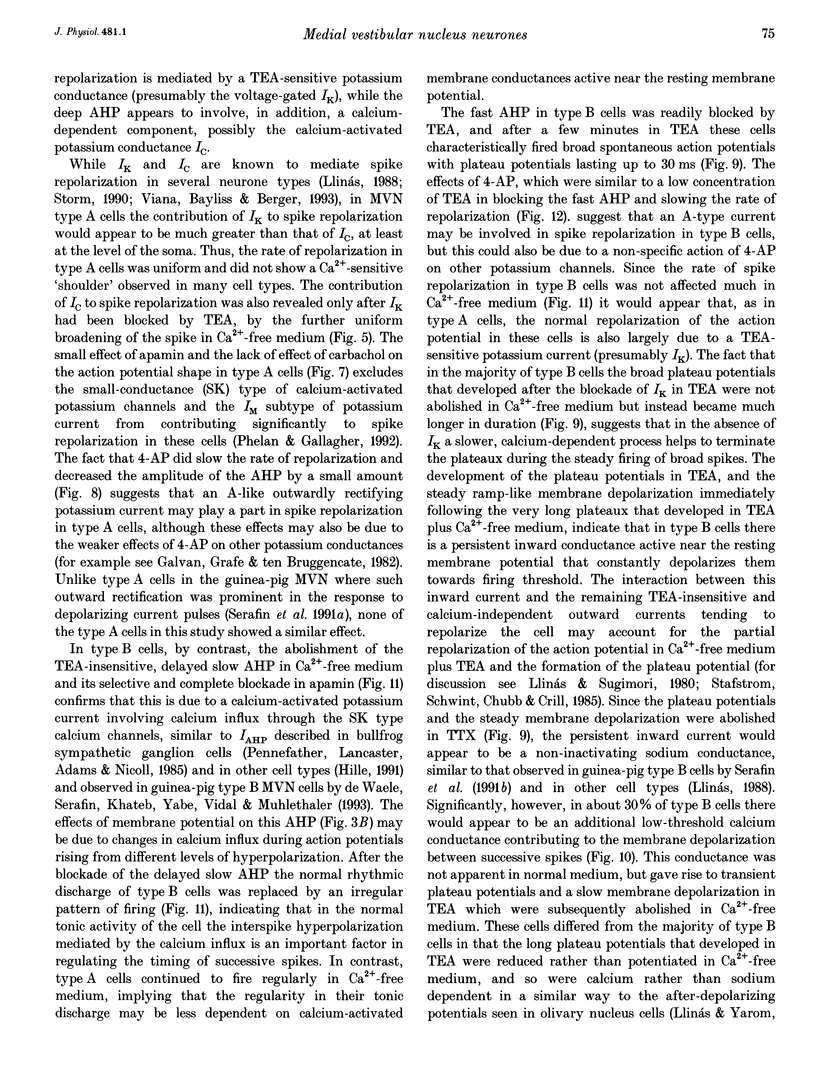
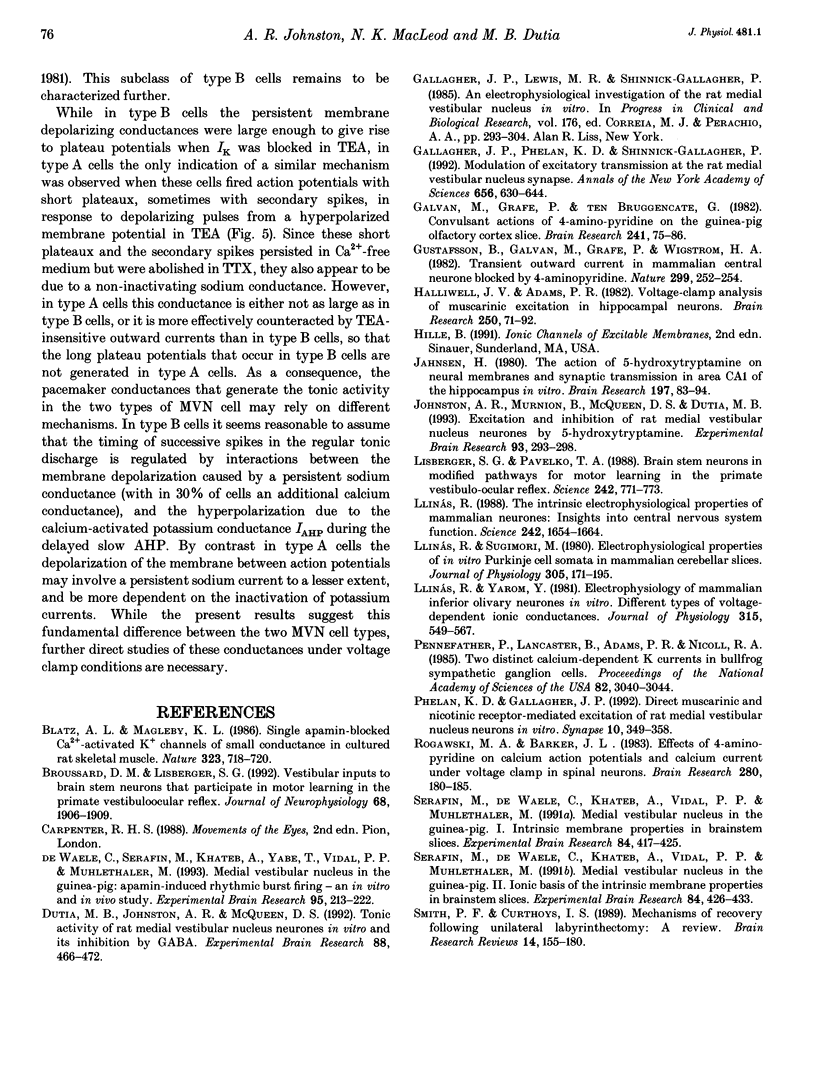
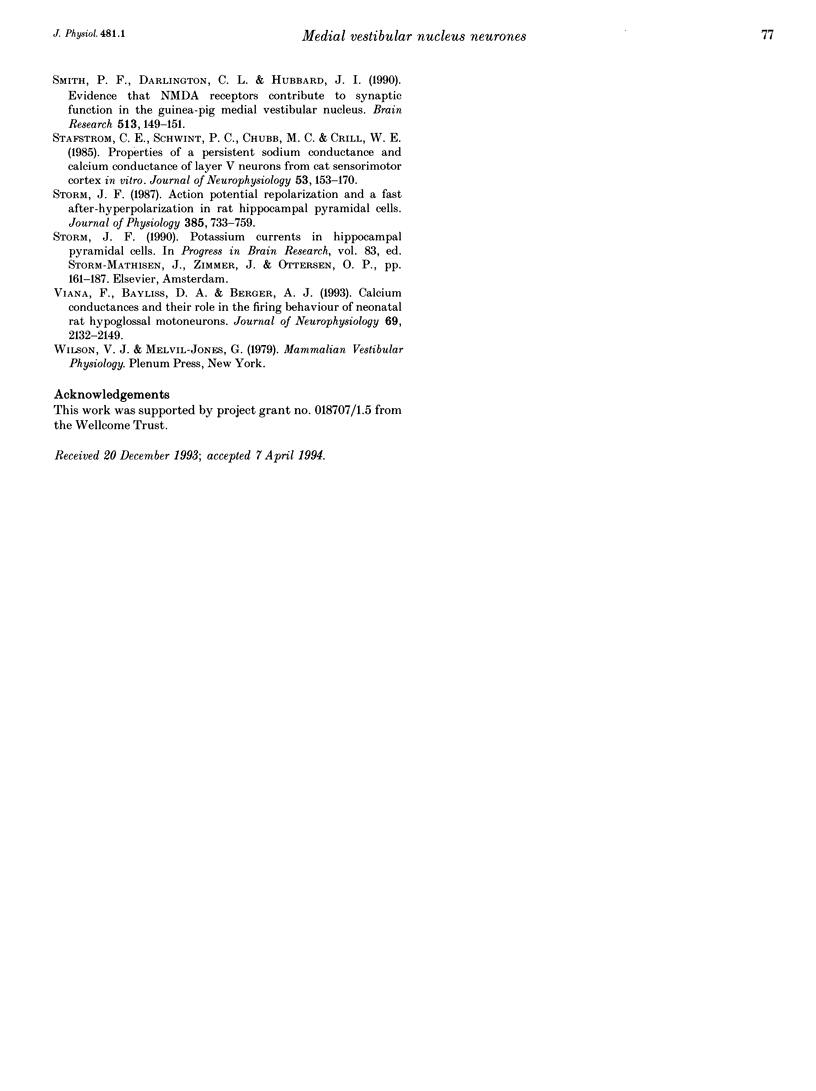
Selected References
These references are in PubMed. This may not be the complete list of references from this article.
- Blatz A. L., Magleby K. L. Single apamin-blocked Ca-activated K+ channels of small conductance in cultured rat skeletal muscle. Nature. 1986 Oct 23;323(6090):718–720. doi: 10.1038/323718a0. [DOI] [PubMed] [Google Scholar]
- Broussard D. M., Lisberger S. G. Vestibular inputs to brain stem neurons that participate in motor learning in the primate vestibuloocular reflex. J Neurophysiol. 1992 Nov;68(5):1906–1909. doi: 10.1152/jn.1992.68.5.1906. [DOI] [PubMed] [Google Scholar]
- Dutia M. B., Johnston A. R., McQueen D. S. Tonic activity of rat medial vestibular nucleus neurones in vitro and its inhibition by GABA. Exp Brain Res. 1992;88(3):466–472. doi: 10.1007/BF00228176. [DOI] [PubMed] [Google Scholar]
- Gallagher J. P., Lewis M. R., Gallagher P. S. An electrophysiological investigation of the rat medial vestibular nucleus in vitro. Prog Clin Biol Res. 1985;176:293–304. [PubMed] [Google Scholar]
- Gallagher J. P., Phelan K. D., Shinnick-Gallagher P. Modulation of excitatory transmission at the rat medial vestibular nucleus synapse. Ann N Y Acad Sci. 1992 May 22;656:630–644. doi: 10.1111/j.1749-6632.1992.tb25241.x. [DOI] [PubMed] [Google Scholar]
- Galvan M., Grafe P., ten Bruggencate G. Convulsant actions of 4-aminopyridine on the guinea-pig olfactory cortex slice. Brain Res. 1982 Jun 3;241(1):75–86. doi: 10.1016/0006-8993(82)91230-6. [DOI] [PubMed] [Google Scholar]
- Gustafsson B., Galvan M., Grafe P., Wigström H. A transient outward current in a mammalian central neurone blocked by 4-aminopyridine. Nature. 1982 Sep 16;299(5880):252–254. doi: 10.1038/299252a0. [DOI] [PubMed] [Google Scholar]
- Halliwell J. V., Adams P. R. Voltage-clamp analysis of muscarinic excitation in hippocampal neurons. Brain Res. 1982 Oct 28;250(1):71–92. doi: 10.1016/0006-8993(82)90954-4. [DOI] [PubMed] [Google Scholar]
- Jahnsen H. The action of 5-hydroxytryptamine on neuronal membranes and synaptic transmission in area CA1 of the hippocampus in vitro. Brain Res. 1980 Sep 15;197(1):83–94. doi: 10.1016/0006-8993(80)90436-9. [DOI] [PubMed] [Google Scholar]
- Johnston A. R., Murnion B., McQueen D. S., Dutia M. B. Excitation and inhibition of rat medial vestibular nucleus neurones by 5-hydroxytryptamine. Exp Brain Res. 1993;93(2):293–298. doi: 10.1007/BF00228397. [DOI] [PubMed] [Google Scholar]
- Lisberger S. G., Pavelko T. A. Brain stem neurons in modified pathways for motor learning in the primate vestibulo-ocular reflex. Science. 1988 Nov 4;242(4879):771–773. doi: 10.1126/science.3142040. [DOI] [PubMed] [Google Scholar]
- Llinás R. R. The intrinsic electrophysiological properties of mammalian neurons: insights into central nervous system function. Science. 1988 Dec 23;242(4886):1654–1664. doi: 10.1126/science.3059497. [DOI] [PubMed] [Google Scholar]
- Llinás R., Sugimori M. Electrophysiological properties of in vitro Purkinje cell somata in mammalian cerebellar slices. J Physiol. 1980 Aug;305:171–195. doi: 10.1113/jphysiol.1980.sp013357. [DOI] [PMC free article] [PubMed] [Google Scholar]
- Llinás R., Yarom Y. Electrophysiology of mammalian inferior olivary neurones in vitro. Different types of voltage-dependent ionic conductances. J Physiol. 1981 Jun;315:549–567. doi: 10.1113/jphysiol.1981.sp013763. [DOI] [PMC free article] [PubMed] [Google Scholar]
- Pennefather P., Lancaster B., Adams P. R., Nicoll R. A. Two distinct Ca-dependent K currents in bullfrog sympathetic ganglion cells. Proc Natl Acad Sci U S A. 1985 May;82(9):3040–3044. doi: 10.1073/pnas.82.9.3040. [DOI] [PMC free article] [PubMed] [Google Scholar]
- Phelan K. D., Gallagher J. P. Direct muscarinic and nicotinic receptor-mediated excitation of rat medial vestibular nucleus neurons in vitro. Synapse. 1992 Apr;10(4):349–358. doi: 10.1002/syn.890100410. [DOI] [PubMed] [Google Scholar]
- Rogawski M. A., Barker J. L. Effects of 4-aminopyridine on calcium action potentials and calcium current under voltage clamp in spinal neurons. Brain Res. 1983 Nov 28;280(1):180–185. doi: 10.1016/0006-8993(83)91190-3. [DOI] [PubMed] [Google Scholar]
- Serafin M., de Waele C., Khateb A., Vidal P. P., Mühlethaler M. Medial vestibular nucleus in the guinea-pig. I. Intrinsic membrane properties in brainstem slices. Exp Brain Res. 1991;84(2):417–425. doi: 10.1007/BF00231464. [DOI] [PubMed] [Google Scholar]
- Serafin M., de Waele C., Khateb A., Vidal P. P., Mühlethaler M. Medial vestibular nucleus in the guinea-pig. II. Ionic basis of the intrinsic membrane properties in brainstem slices. Exp Brain Res. 1991;84(2):426–433. doi: 10.1007/BF00231465. [DOI] [PubMed] [Google Scholar]
- Smith P. F., Curthoys I. S. Mechanisms of recovery following unilateral labyrinthectomy: a review. Brain Res Brain Res Rev. 1989 Apr-Jun;14(2):155–180. doi: 10.1016/0165-0173(89)90013-1. [DOI] [PubMed] [Google Scholar]
- Smith P. F., Darlington C. L., Hubbard J. I. Evidence that NMDA receptors contribute to synaptic function in the guinea pig medial vestibular nucleus. Brain Res. 1990 Apr 9;513(1):149–151. doi: 10.1016/0006-8993(90)91101-l. [DOI] [PubMed] [Google Scholar]
- Stafstrom C. E., Schwindt P. C., Chubb M. C., Crill W. E. Properties of persistent sodium conductance and calcium conductance of layer V neurons from cat sensorimotor cortex in vitro. J Neurophysiol. 1985 Jan;53(1):153–170. doi: 10.1152/jn.1985.53.1.153. [DOI] [PubMed] [Google Scholar]
- Storm J. F. Action potential repolarization and a fast after-hyperpolarization in rat hippocampal pyramidal cells. J Physiol. 1987 Apr;385:733–759. doi: 10.1113/jphysiol.1987.sp016517. [DOI] [PMC free article] [PubMed] [Google Scholar]
- Storm J. F. Potassium currents in hippocampal pyramidal cells. Prog Brain Res. 1990;83:161–187. doi: 10.1016/s0079-6123(08)61248-0. [DOI] [PubMed] [Google Scholar]
- Viana F., Bayliss D. A., Berger A. J. Calcium conductances and their role in the firing behavior of neonatal rat hypoglossal motoneurons. J Neurophysiol. 1993 Jun;69(6):2137–2149. doi: 10.1152/jn.1993.69.6.2137. [DOI] [PubMed] [Google Scholar]
- de Waele C., Serafin M., Khateb A., Yabe T., Vidal P. P., Mühlethaler M. Medial vestibular nucleus in the guinea-pig: apamin-induced rhythmic burst firing--an in vitro and in vivo study. Exp Brain Res. 1993;95(2):213–222. doi: 10.1007/BF00229780. [DOI] [PubMed] [Google Scholar]