Abstract
1. In the rabbit nodose ganglion, C-type fibre neurones (C neurones) can be divided into two subtypes according to their after-hyperpolarizing potential (AHP) i.e. those with a fast AHP only and those with a fast AHP and a subsequent slow AHP produced by a slow calcium-dependent potassium current. In addition we have shown that some C neurones can be divided into two groups according to the effect of membrane hyperpolarization on their spikes i.e. type 1 in which duration and amplitude do not change and type 2 in which duration and amplitude decrease significantly. 2. In the present report we studied the effect of A-current (IA) on spike duration, amplitude and slow AHP using intracellular recording techniques. 3. To detect the presence of IA, we first applied a series of increasing rectangular hyperpolarizing pulses to remove IA inactivation and then a short depolarizing pulse to trigger a spike. In type 1 C neurones the lag time of the spike in relation to hyperpolarization remains constant whereas in type 2 C neurones the spike only appears after IA inactivation and lag time in relation to hyperpolarization is lengthened. Thus, type 2 C neurones have an IA while type 1 C neurones do not. The fact that addition of cadmium did not change the lag time in type 2 C neurones shows that the IA is not calcium dependent. 4. Nodose neurones can be orthodromically activated by stimulation of the vagal peripheral process. In this way, after a hyperpolarizing pulse, IA can be fully activated by the orthodromic spike itself. Under these conditions it is possible to analyse the effects of IA on the spike. This was done by increasing either the hyperpolarizing potential, pulse duration, or the delay of the spike after the end of the pulse. We observed that maximum IA inactivation removal was always associated with the lowest duration and amplitude of the spike. 5. When IA inhibitors, 4-aminopyridine (4-AP) or catechol, were applied to type 2 C neurones, the delay of the spike after the hyperpolarization-depolarization test was no longer observed. In addition 4-AP abolished the shortening of the duration of the spike induced by steady hyperpolarization. 6. In type 2 C neurones with slow AHP, the IA-related decrease in spike duration was associated with a disappearance of the slow AHP. This indicates that IA decreases the calcium influx during the spike.(ABSTRACT TRUNCATED AT 400 WORDS)
Full text
PDF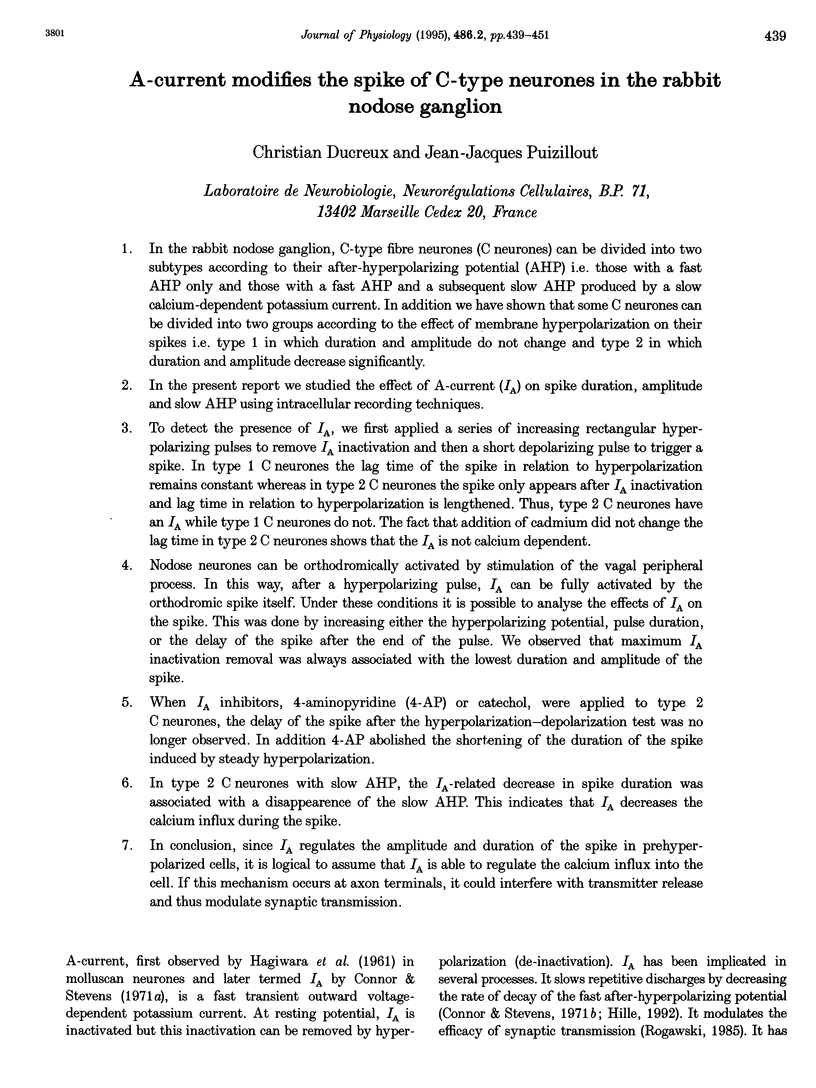
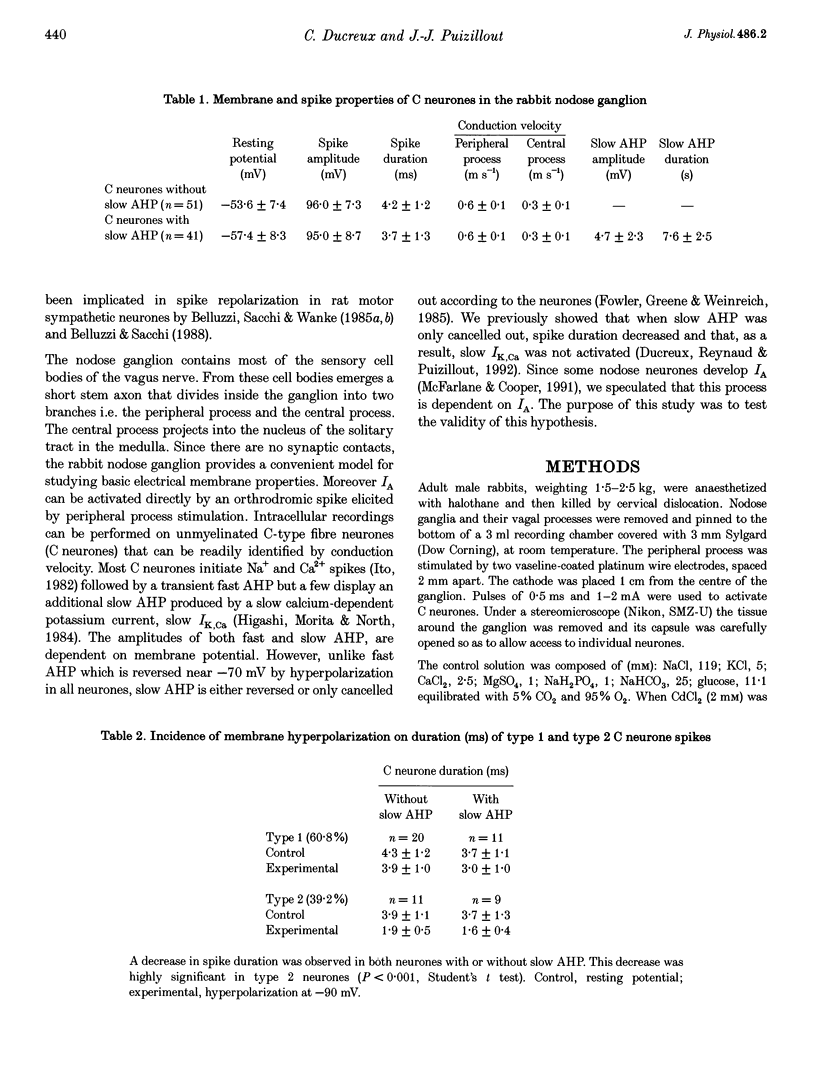
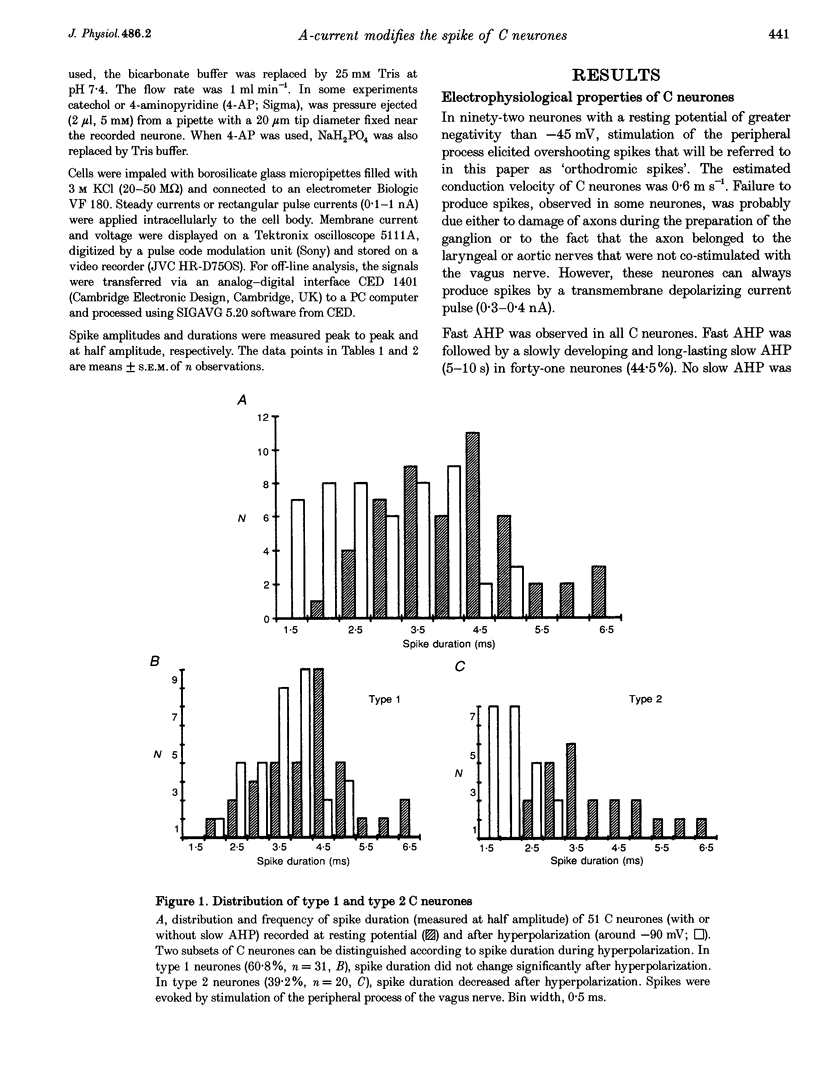
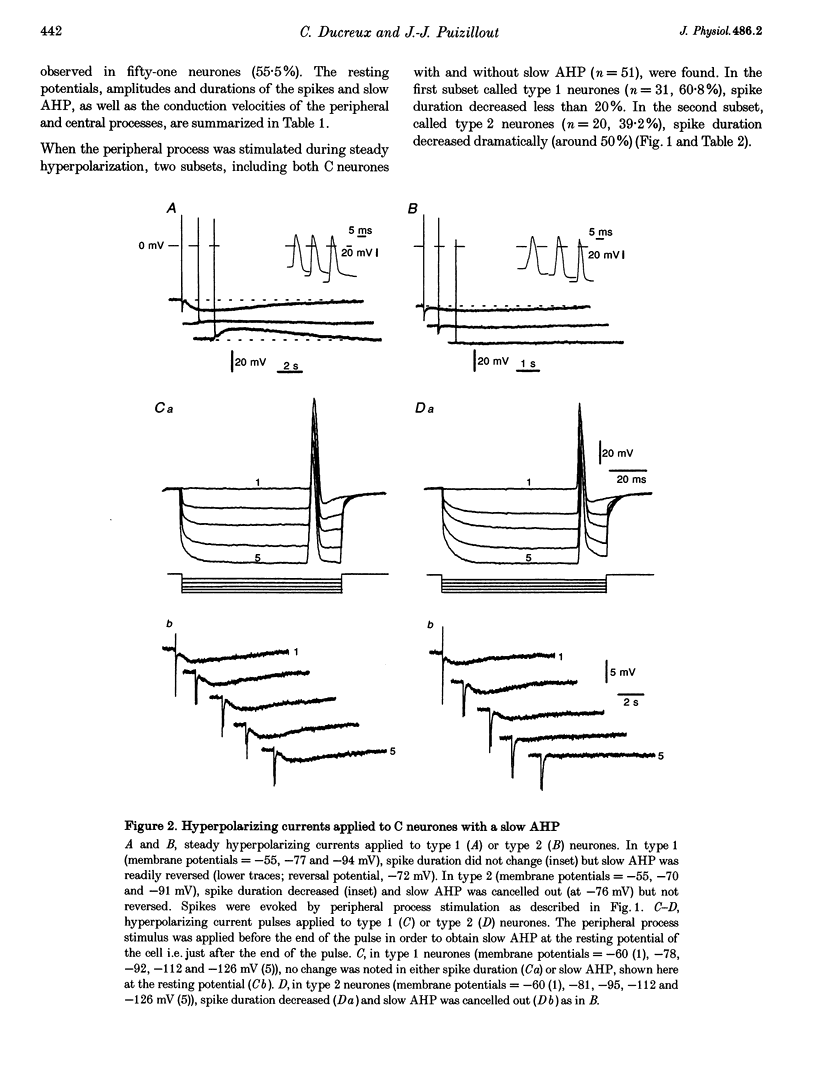
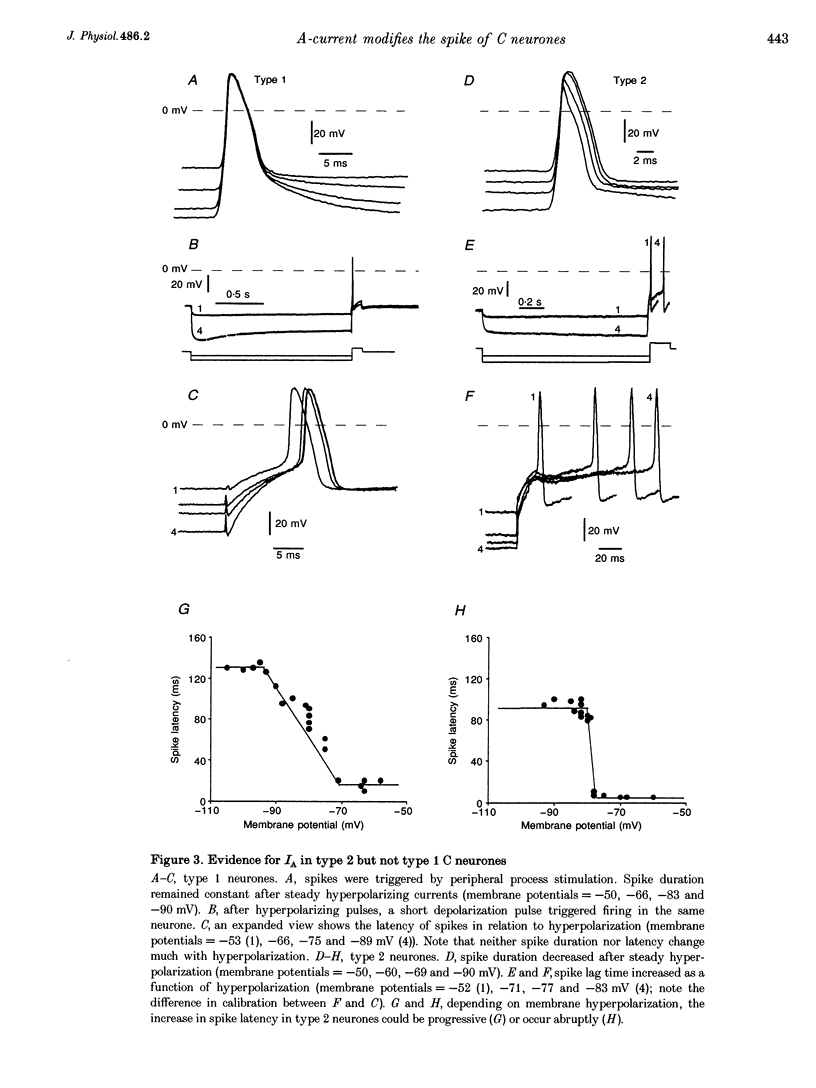
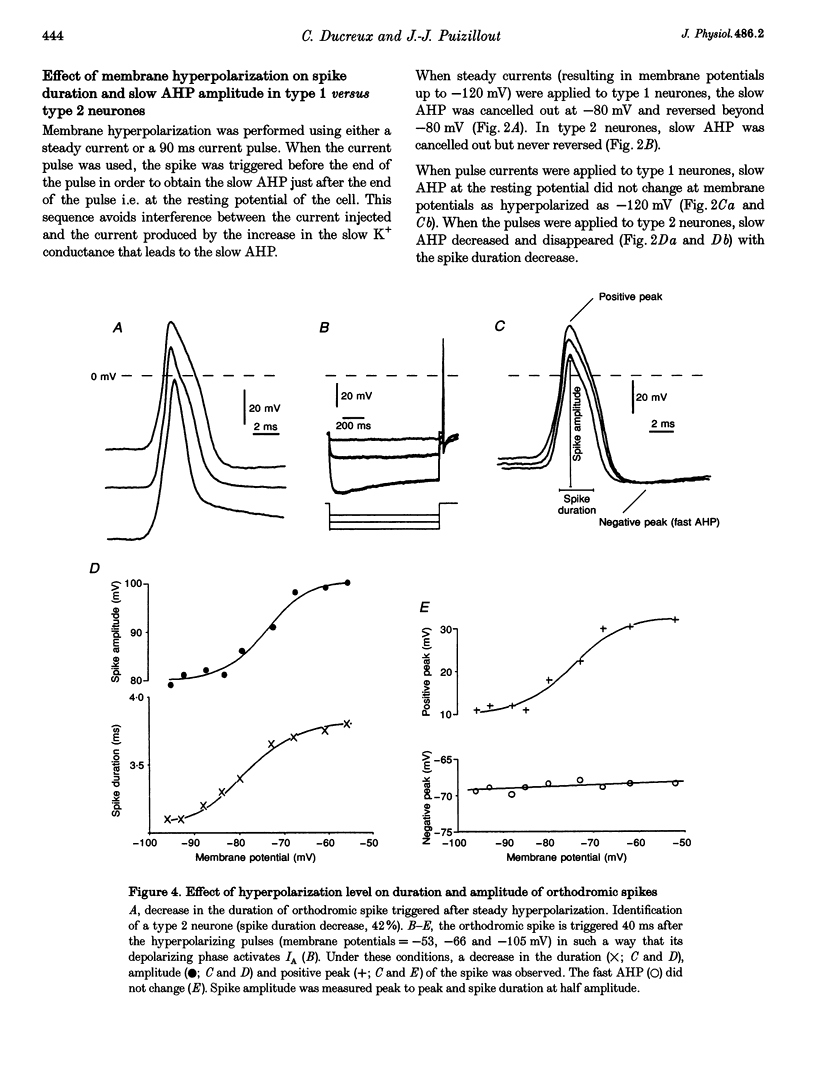
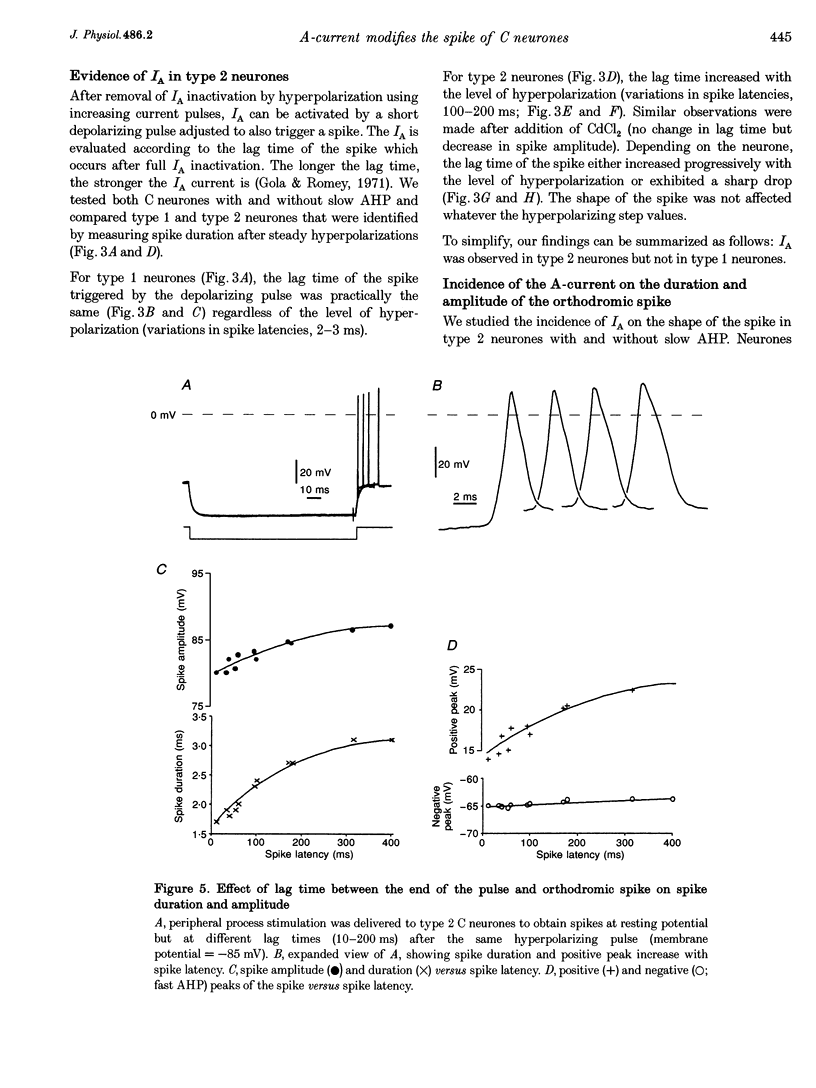
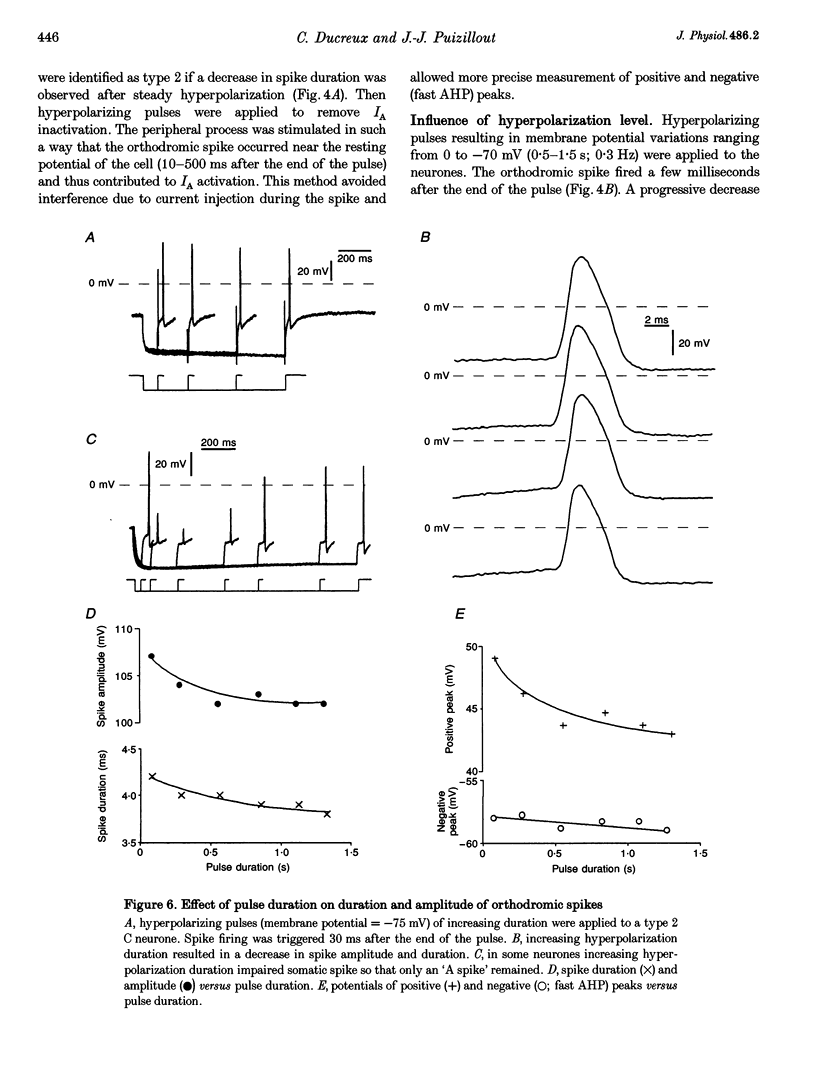
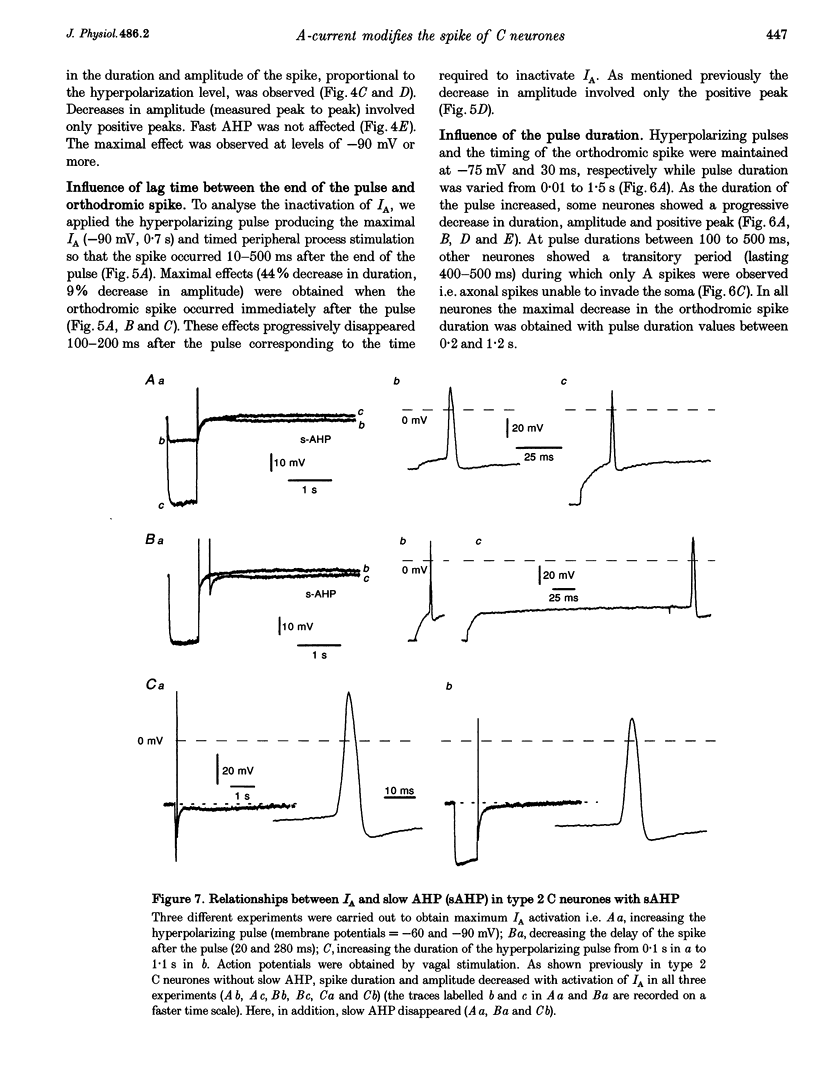
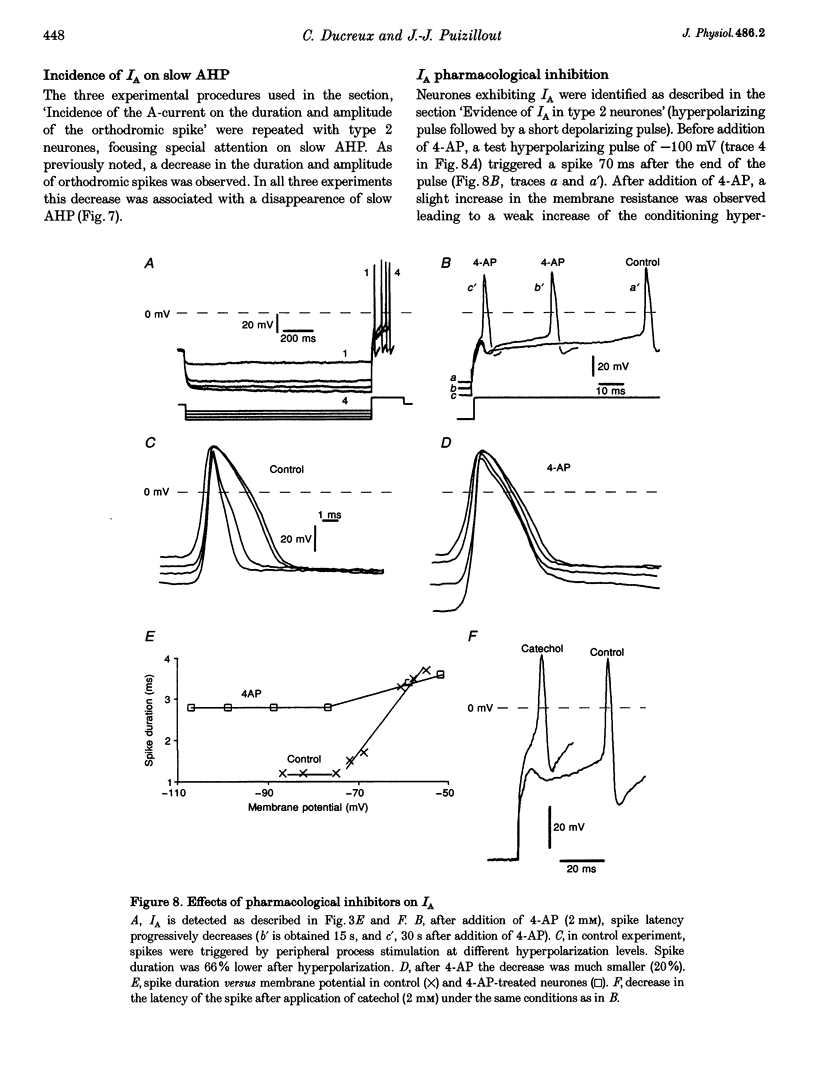
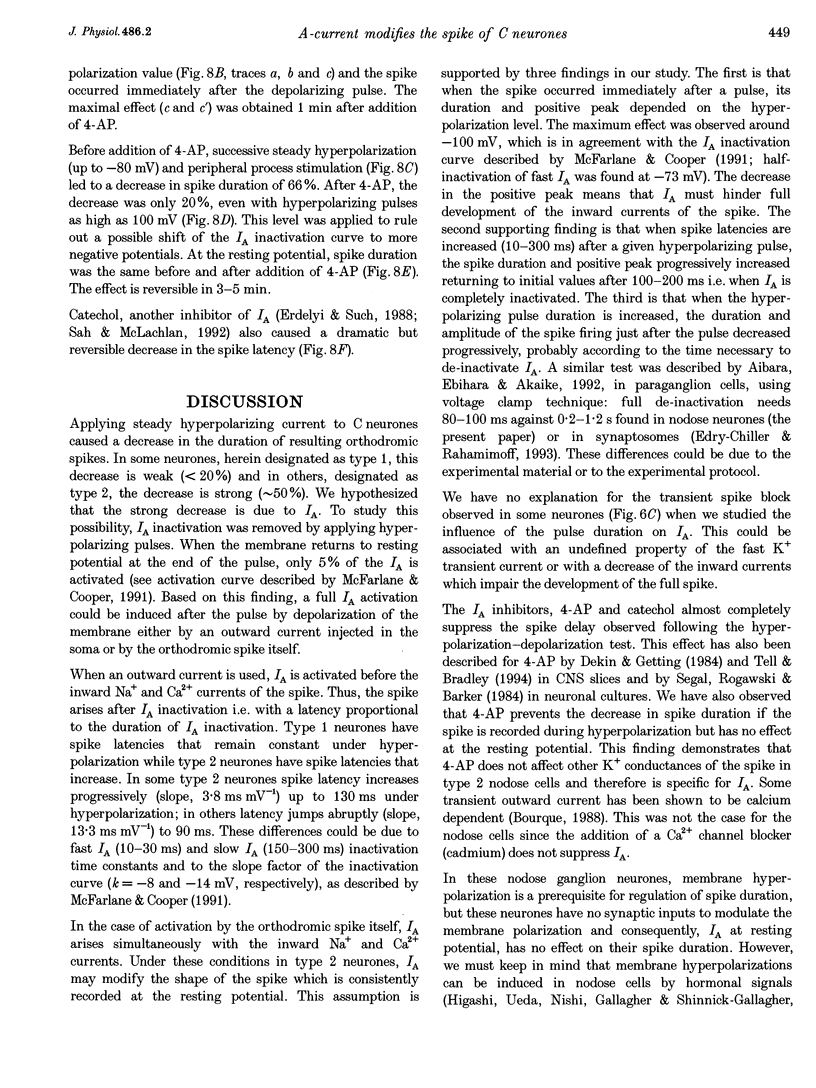
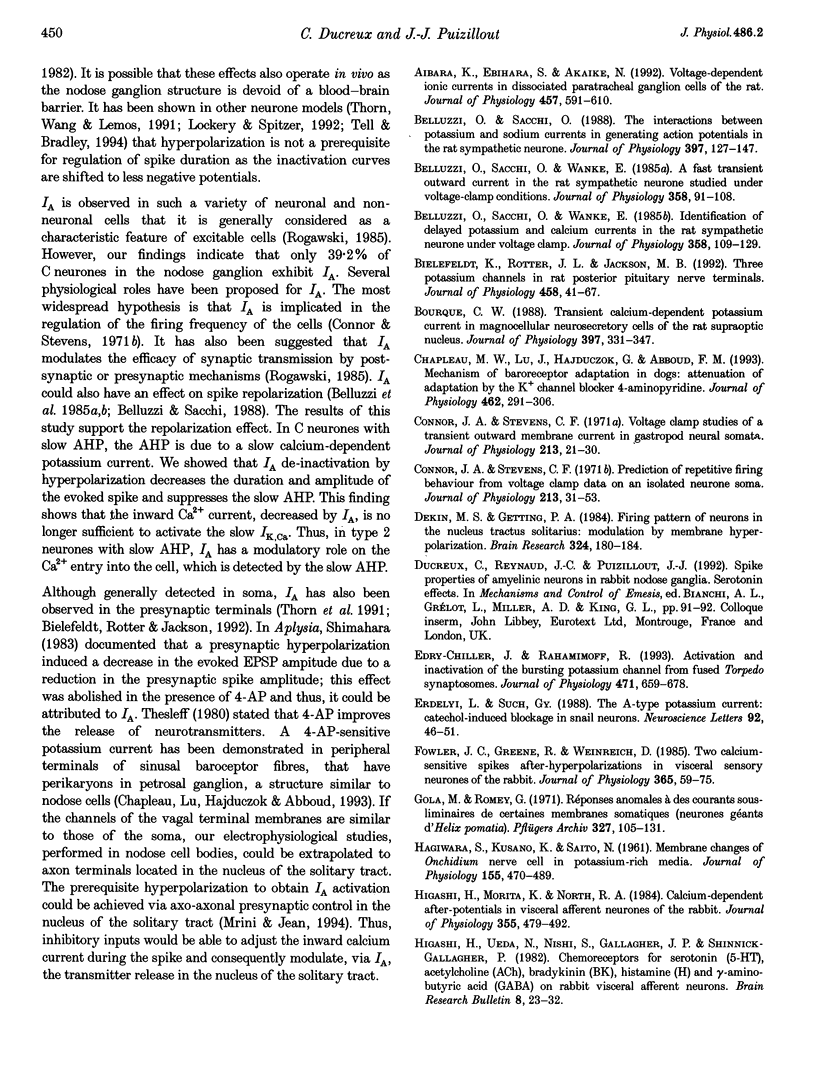
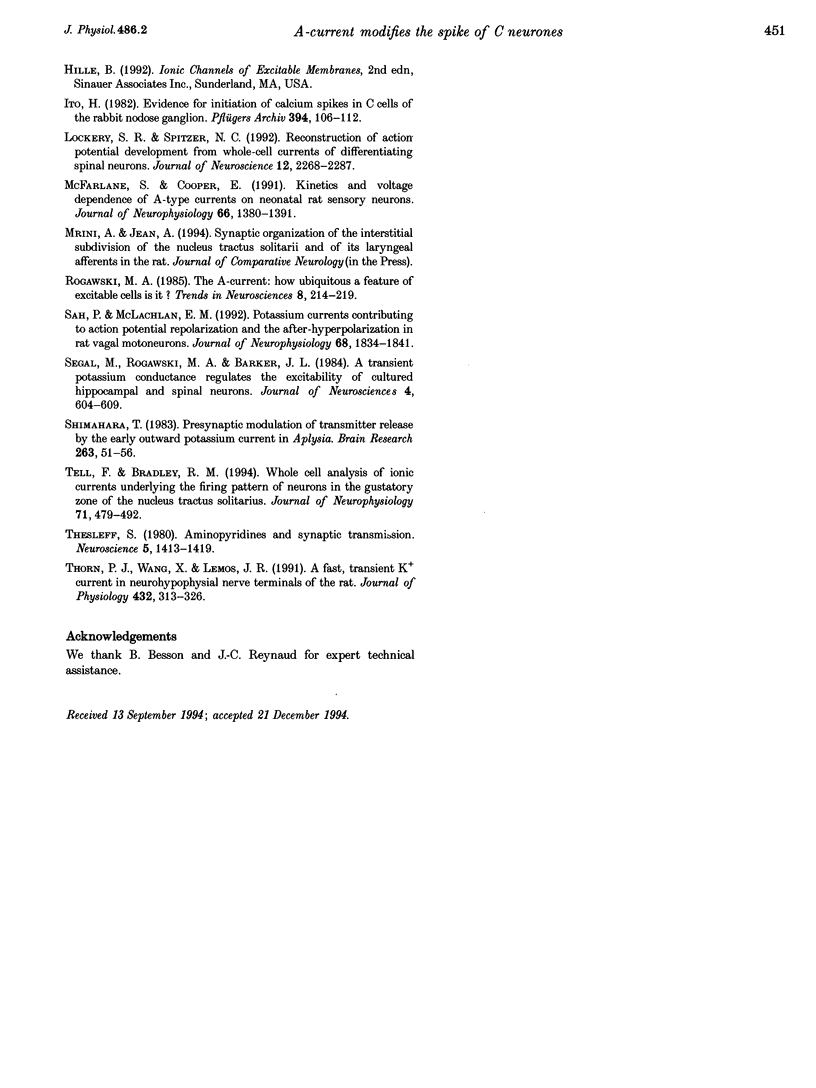
Selected References
These references are in PubMed. This may not be the complete list of references from this article.
- Aibara K., Ebihara S., Akaike N. Voltage-dependent ionic currents in dissociated paratracheal ganglion cells of the rat. J Physiol. 1992 Nov;457:591–610. doi: 10.1113/jphysiol.1992.sp019396. [DOI] [PMC free article] [PubMed] [Google Scholar]
- Belluzzi O., Sacchi O. The interactions between potassium and sodium currents in generating action potentials in the rat sympathetic neurone. J Physiol. 1988 Mar;397:127–147. doi: 10.1113/jphysiol.1988.sp016992. [DOI] [PMC free article] [PubMed] [Google Scholar]
- Belluzzi O., Sacchi O., Wanke E. A fast transient outward current in the rat sympathetic neurone studied under voltage-clamp conditions. J Physiol. 1985 Jan;358:91–108. doi: 10.1113/jphysiol.1985.sp015542. [DOI] [PMC free article] [PubMed] [Google Scholar]
- Belluzzi O., Sacchi O., Wanke E. Identification of delayed potassium and calcium currents in the rat sympathetic neurone under voltage clamp. J Physiol. 1985 Jan;358:109–129. doi: 10.1113/jphysiol.1985.sp015543. [DOI] [PMC free article] [PubMed] [Google Scholar]
- Bielefeldt K., Rotter J. L., Jackson M. B. Three potassium channels in rat posterior pituitary nerve terminals. J Physiol. 1992 Dec;458:41–67. doi: 10.1113/jphysiol.1992.sp019405. [DOI] [PMC free article] [PubMed] [Google Scholar]
- Bourque C. W. Transient calcium-dependent potassium current in magnocellular neurosecretory cells of the rat supraoptic nucleus. J Physiol. 1988 Mar;397:331–347. doi: 10.1113/jphysiol.1988.sp017004. [DOI] [PMC free article] [PubMed] [Google Scholar]
- Chapleau M. W., Lu J., Hajduczok G., Abboud F. M. Mechanism of baroreceptor adaptation in dogs: attenuation of adaptation by the K+ channel blocker 4-aminopyridine. J Physiol. 1993 Mar;462:291–306. doi: 10.1113/jphysiol.1993.sp019556. [DOI] [PMC free article] [PubMed] [Google Scholar]
- Connor J. A., Stevens C. F. Prediction of repetitive firing behaviour from voltage clamp data on an isolated neurone soma. J Physiol. 1971 Feb;213(1):31–53. doi: 10.1113/jphysiol.1971.sp009366. [DOI] [PMC free article] [PubMed] [Google Scholar]
- Connor J. A., Stevens C. F. Voltage clamp studies of a transient outward membrane current in gastropod neural somata. J Physiol. 1971 Feb;213(1):21–30. doi: 10.1113/jphysiol.1971.sp009365. [DOI] [PMC free article] [PubMed] [Google Scholar]
- Dekin M. S., Getting P. A. Firing pattern of neurons in the nucleus tractus solitarius: modulation by membrane hyperpolarization. Brain Res. 1984 Dec 17;324(1):180–184. doi: 10.1016/0006-8993(84)90640-1. [DOI] [PubMed] [Google Scholar]
- Edry-Schiller J., Rahamimoff R. Activation and inactivation of the bursting potassium channel from fused Torpedo synaptosomes. J Physiol. 1993 Nov;471:659–678. doi: 10.1113/jphysiol.1993.sp019921. [DOI] [PMC free article] [PubMed] [Google Scholar]
- Erdélyi L., Such G. The A-type potassium current: catechol-induced blockage in snail neurons. Neurosci Lett. 1988 Sep 23;92(1):46–51. doi: 10.1016/0304-3940(88)90740-9. [DOI] [PubMed] [Google Scholar]
- Fowler J. C., Greene R., Weinreich D. Two calcium-sensitive spike after-hyperpolarizations in visceral sensory neurones of the rabbit. J Physiol. 1985 Aug;365:59–75. doi: 10.1113/jphysiol.1985.sp015759. [DOI] [PMC free article] [PubMed] [Google Scholar]
- Gola M., Romey G. Responses anomales a des courants sous-liminaires de certaines membranes somatiques (neurones géants d'Helix pomatia). Analyse par la methode du voltage impose. Pflugers Arch. 1971;327(2):105–131. doi: 10.1007/BF00587365. [DOI] [PubMed] [Google Scholar]
- HAGIWARA S., KUSANO K., SAITO N. Membrane changes of Onchidium nerve cell in potassium-rich media. J Physiol. 1961 Mar;155:470–489. doi: 10.1113/jphysiol.1961.sp006640. [DOI] [PMC free article] [PubMed] [Google Scholar]
- Higashi H., Morita K., North R. A. Calcium-dependent after-potentials in visceral afferent neurones of the rabbit. J Physiol. 1984 Oct;355:479–492. doi: 10.1113/jphysiol.1984.sp015433. [DOI] [PMC free article] [PubMed] [Google Scholar]
- Higashi H., Ueda N., Nishi S., Gallagher J. P., Shinnick-Gallagher P. Chemoreceptors for serotonin (5-HT), acetylcholine (ACh), bradykinin (BK), histamine (H) and gamma-aminobutyric acid (GABA) on rabbit visceral afferent neurons. Brain Res Bull. 1982 Jan;8(1):23–32. doi: 10.1016/0361-9230(82)90023-5. [DOI] [PubMed] [Google Scholar]
- Ito H. Evidence for initiation of calcium spikes in C-cells of the rabbit nodose ganglion. Pflugers Arch. 1982 Aug;394(2):106–112. doi: 10.1007/BF00582910. [DOI] [PubMed] [Google Scholar]
- Lockery S. R., Spitzer N. C. Reconstruction of action potential development from whole-cell currents of differentiating spinal neurons. J Neurosci. 1992 Jun;12(6):2268–2287. doi: 10.1523/JNEUROSCI.12-06-02268.1992. [DOI] [PMC free article] [PubMed] [Google Scholar]
- McFarlane S., Cooper E. Kinetics and voltage dependence of A-type currents on neonatal rat sensory neurons. J Neurophysiol. 1991 Oct;66(4):1380–1391. doi: 10.1152/jn.1991.66.4.1380. [DOI] [PubMed] [Google Scholar]
- Sah P., McLachlan E. M. Potassium currents contributing to action potential repolarization and the afterhyperpolarization in rat vagal motoneurons. J Neurophysiol. 1992 Nov;68(5):1834–1841. doi: 10.1152/jn.1992.68.5.1834. [DOI] [PubMed] [Google Scholar]
- Segal M., Rogawski M. A., Barker J. L. A transient potassium conductance regulates the excitability of cultured hippocampal and spinal neurons. J Neurosci. 1984 Feb;4(2):604–609. doi: 10.1523/JNEUROSCI.04-02-00604.1984. [DOI] [PMC free article] [PubMed] [Google Scholar]
- Shimahara T. Presynaptic modulation of transmitter release by the early outward potassium current in Aplysia. Brain Res. 1983 Mar 14;263(1):51–56. doi: 10.1016/0006-8993(83)91199-x. [DOI] [PubMed] [Google Scholar]
- Tell F., Bradley R. M. Whole-cell analysis of ionic currents underlying the firing pattern of neurons in the gustatory zone of the nucleus tractus solitarii. J Neurophysiol. 1994 Feb;71(2):479–492. doi: 10.1152/jn.1994.71.2.479. [DOI] [PubMed] [Google Scholar]
- Thesleff S. Aminopyridines and synaptic transmission. Neuroscience. 1980;5(8):1413–1419. doi: 10.1016/0306-4522(80)90002-0. [DOI] [PubMed] [Google Scholar]
- Thorn P. J., Wang X. M., Lemos J. R. A fast, transient K+ current in neurohypophysial nerve terminals of the rat. J Physiol. 1991 Jan;432:313–326. doi: 10.1113/jphysiol.1991.sp018386. [DOI] [PMC free article] [PubMed] [Google Scholar]