Abstract
1. The molecular mechanisms underlying pH regulation of skeletal muscle ATP-sensitive K+ (KATP) channels were studied using the patch clamp technique in the inside-out configuration. Two effects of intracellular protons were studied in detail: the decrease in magnitude of single-channel currents and the increase in open probability (Po) of nucleotide-inhibited channels. 2. The pH dependence of inward unit currents under different ionic conditions was in poor agreement with either a direct block of the pore by protons or an indirect proton-induced conformational change, but was compatible with the protonation of surface charges located near the cytoplasmic entrance of the pore. This latter electrostatic mechanism was modelled using Gouy-Chapman-Stern theory, which predicted the data accurately with a surface charge density of about 0.1 negative elementary charges per square nanometre and a pK (pH value for 50% effect) value for protonation of these charges of 6.25. The same mechanism, i.e. neutralization of negative surface charges by cation binding, could also account for the previously reported reduction of inward unit currents by Mg2+. 3. Intracellular alkalization did not affect Po of the KATP channels. Acidification increased Po. In the presence of 0.1 mM ATP (no Mg2+), the channel activation vs. pH relationship could be fitted with a sigmoid curve with a Hill coefficient slightly above 2 and a pK value of 6. This latter value was dependent on the ATP concentration, decreasing from 6.3 in 30 microM ATP to 5.3 in 1 microM ATP. 4. Conversely, the channel inhibition vs. ATP concentration curve was shifted to the right when the pH was lowered. At pH 7.1, the ATP concentration causing half-maximal inhibition was about 10 microM. At pH 5.4, it was about 400 microM. The Hill coefficient values remained slightly below 2. Similar effects were observed when ADP was used as the inhibitory nucleotide. 5. These results confirm that a reciprocal competitive link exists between proton and nucleotide binding sites. Quantitatively, they are in full agreement with a steady-state model of a KATP channel possessing four identical protonation sites (microscopic pK, 6) allosterically connected to the channel open state and two identical nucleotide sites (microscopic ATP dissociation constant, approximately 30 microM) connected to the closed state.
Full text
PDF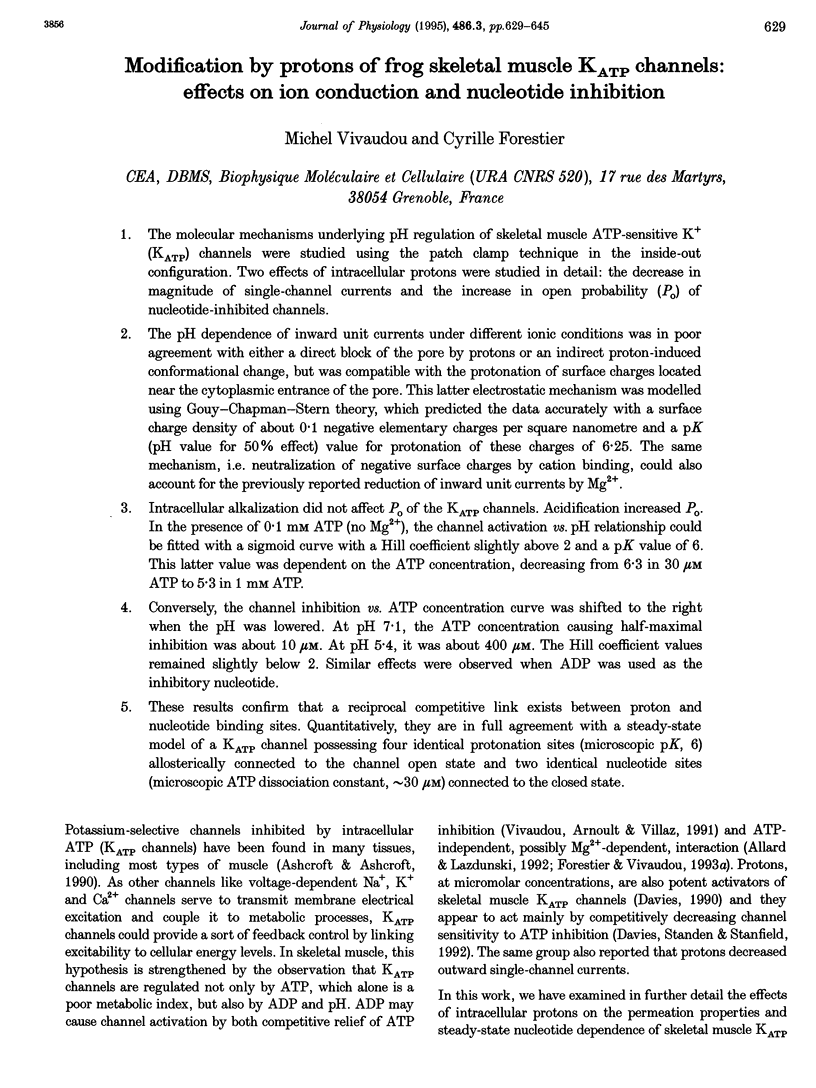
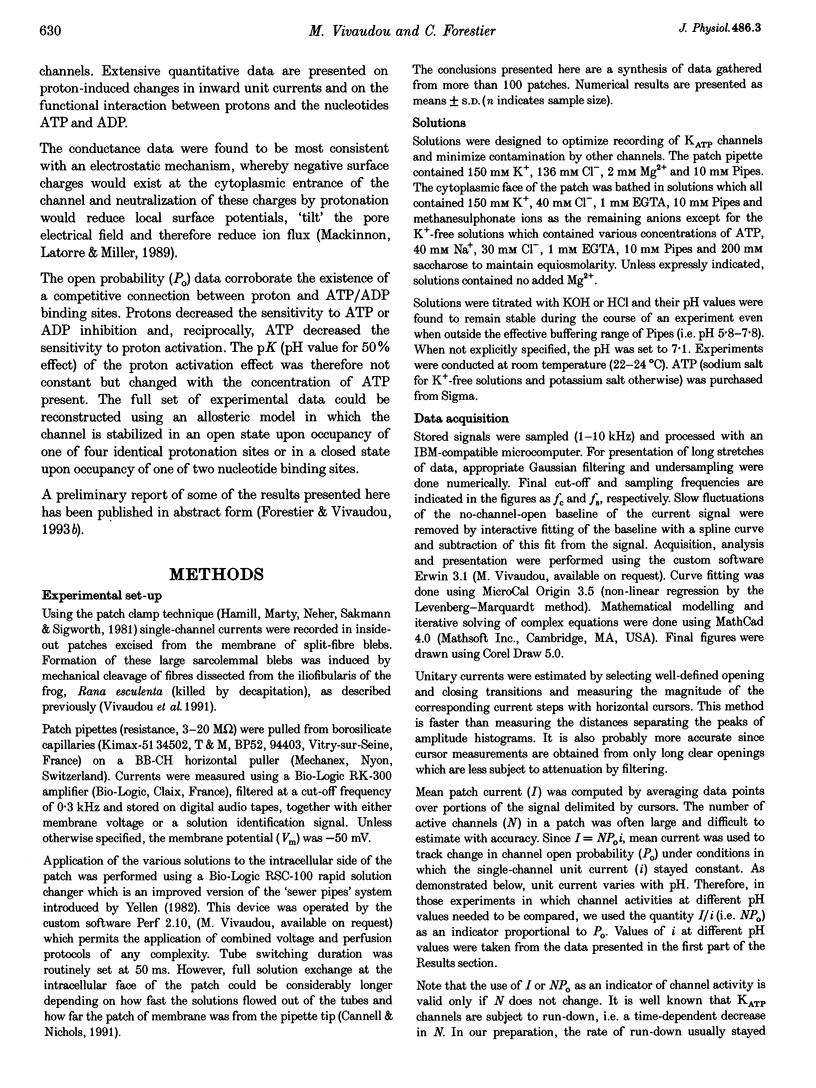
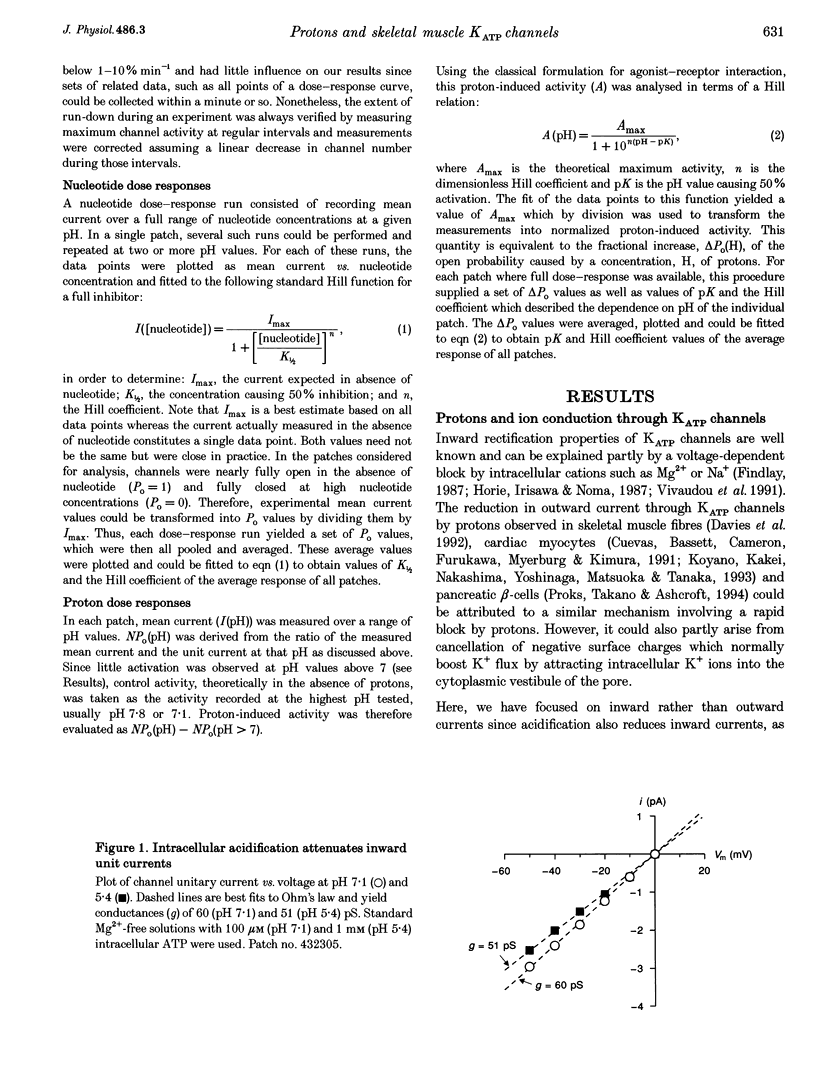
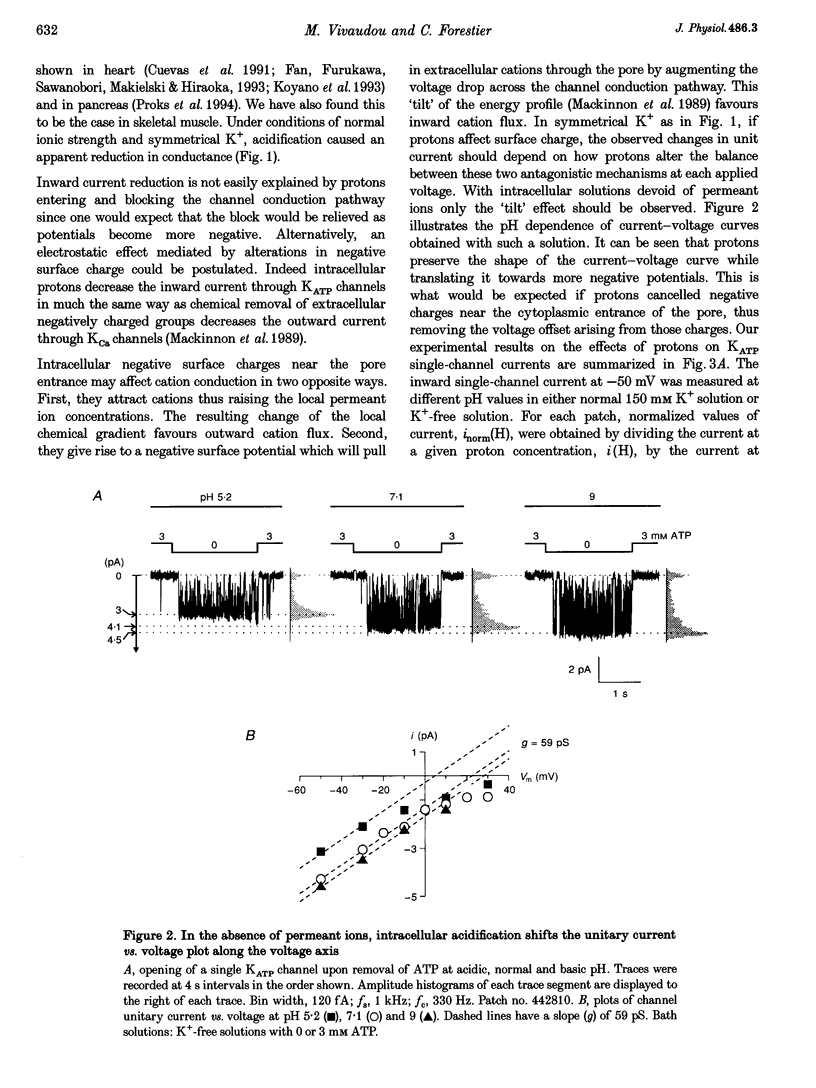
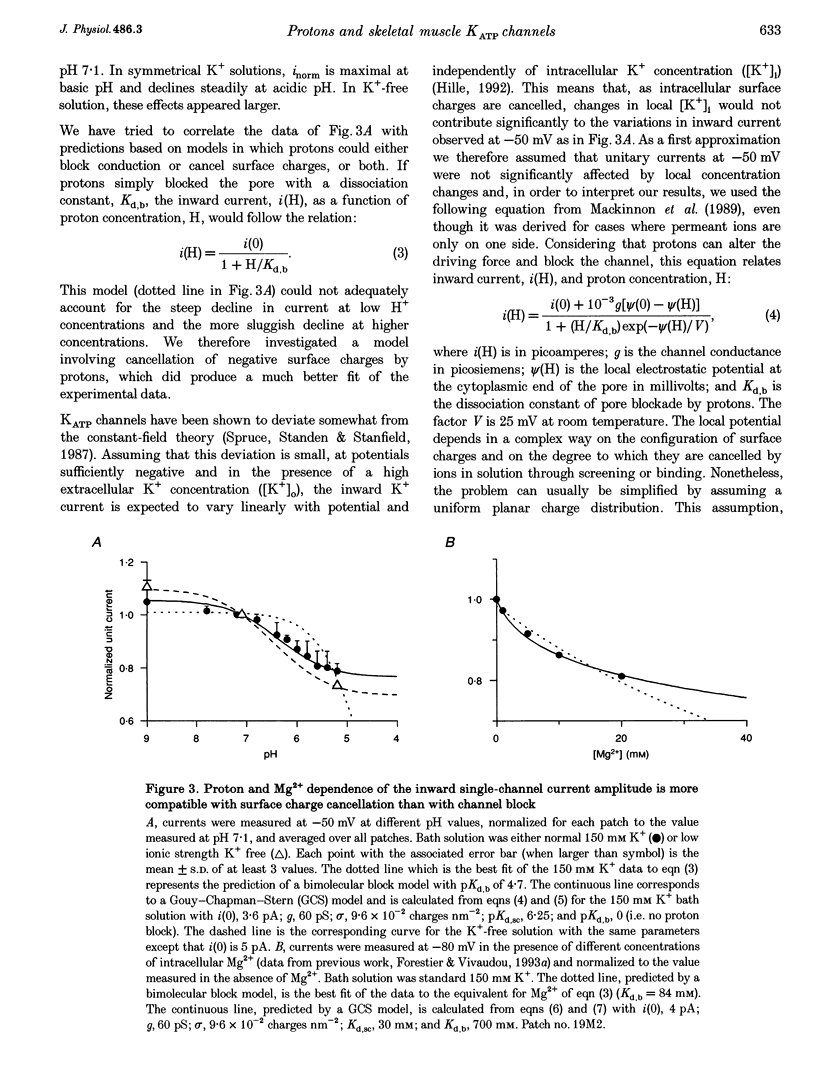
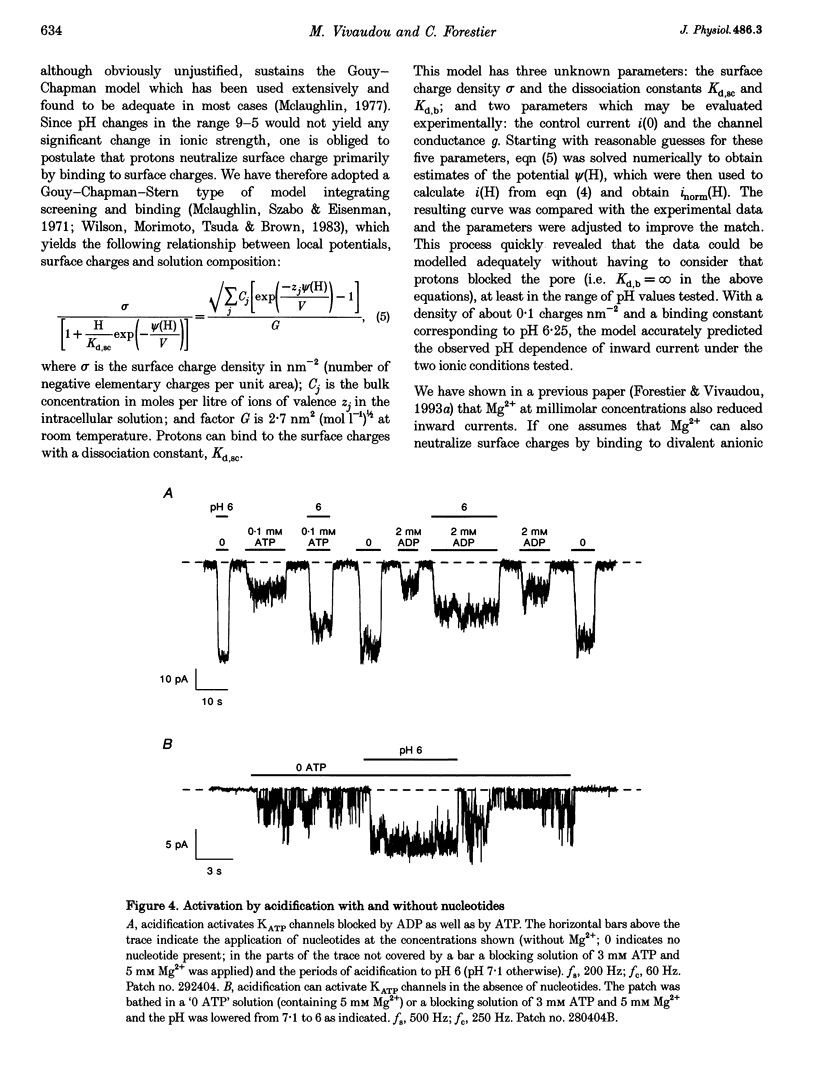
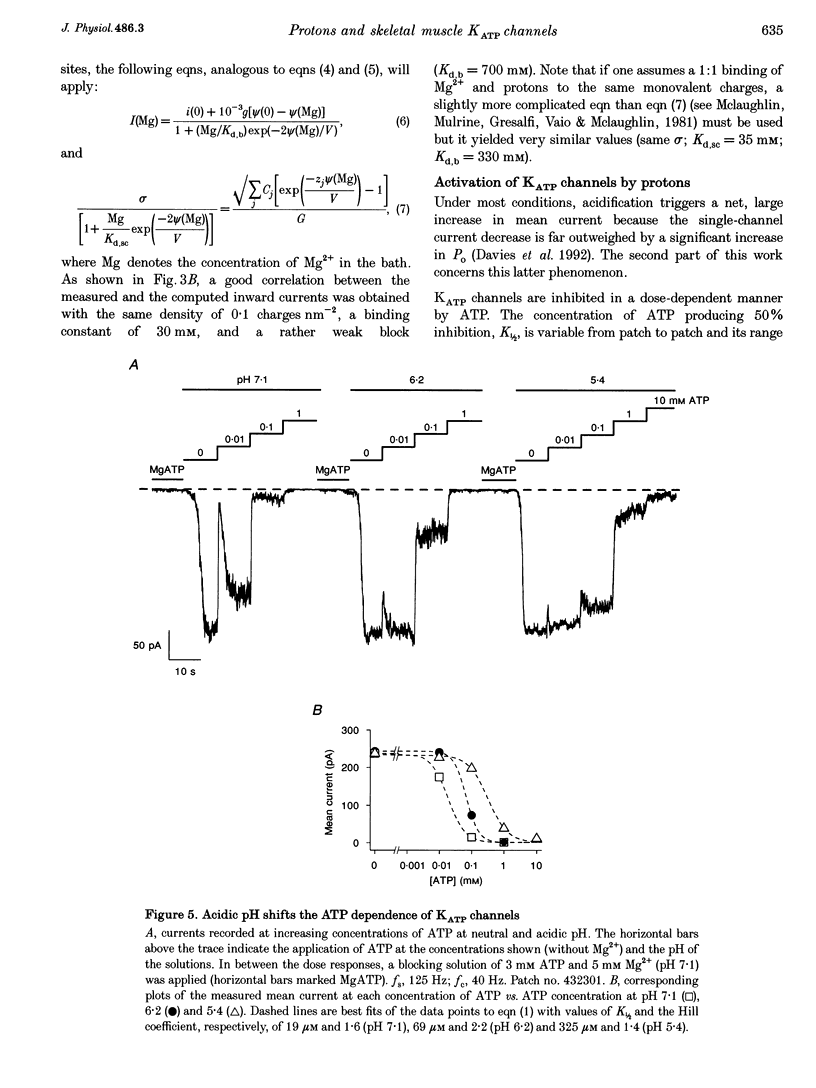
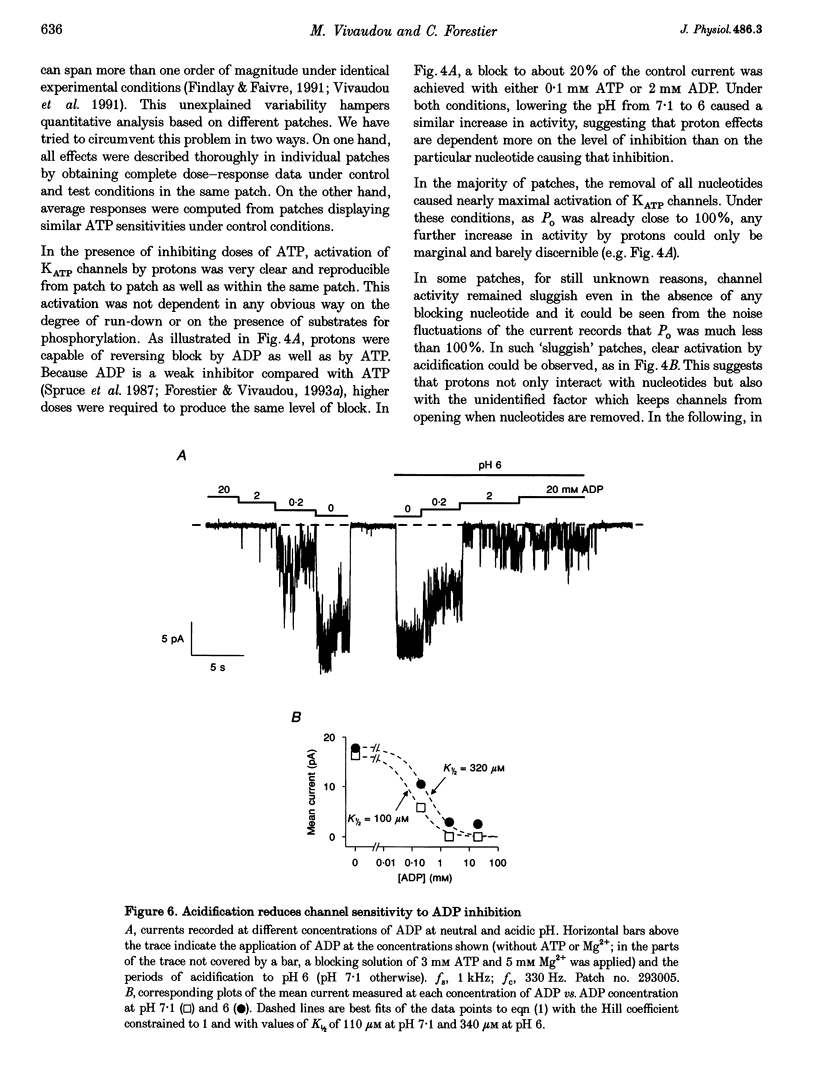
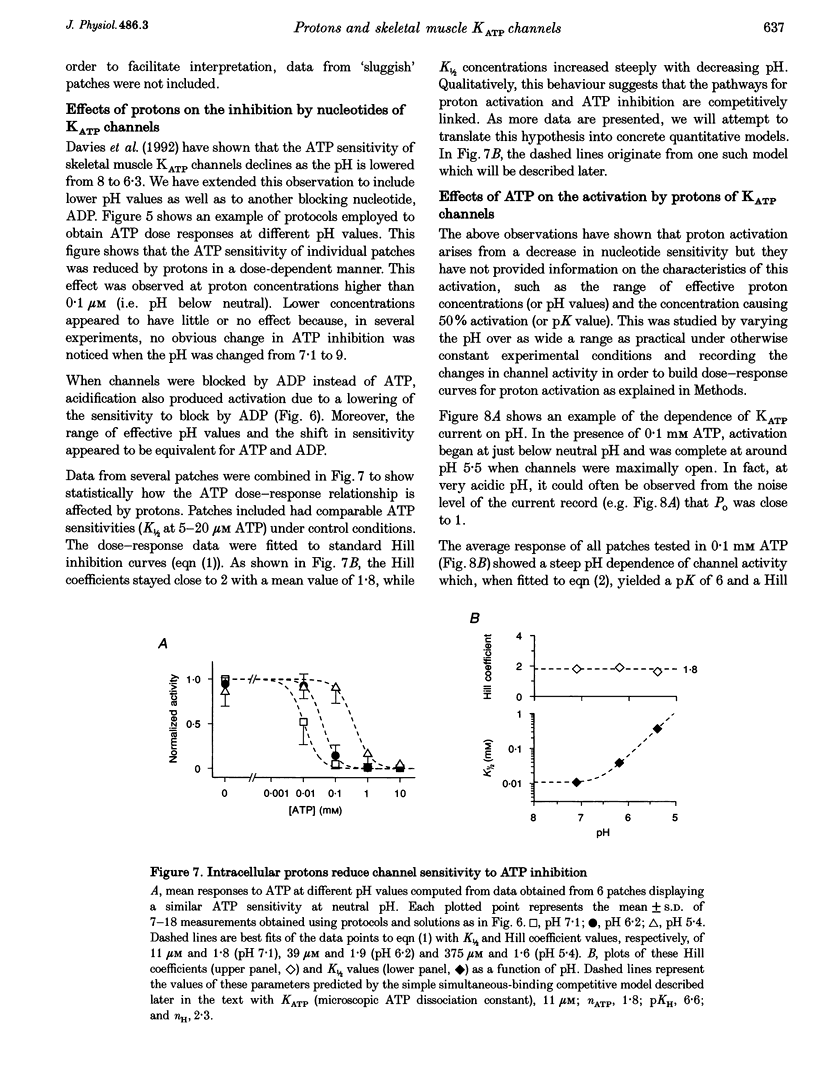
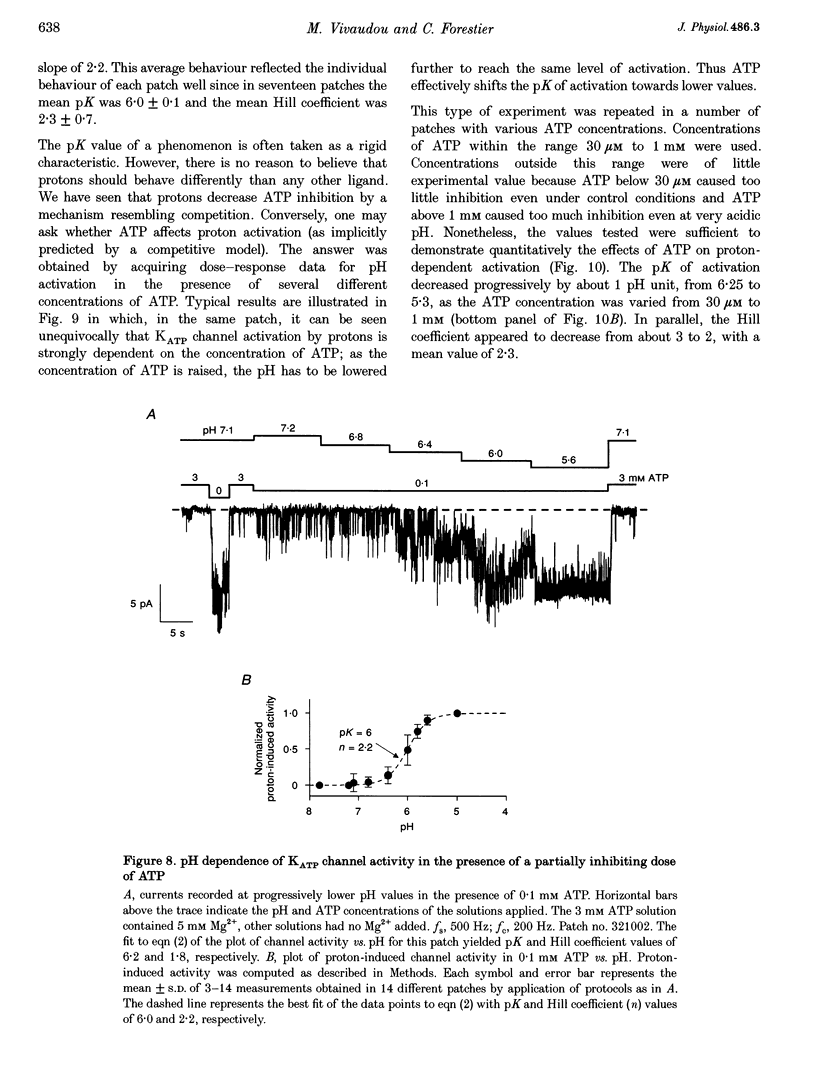
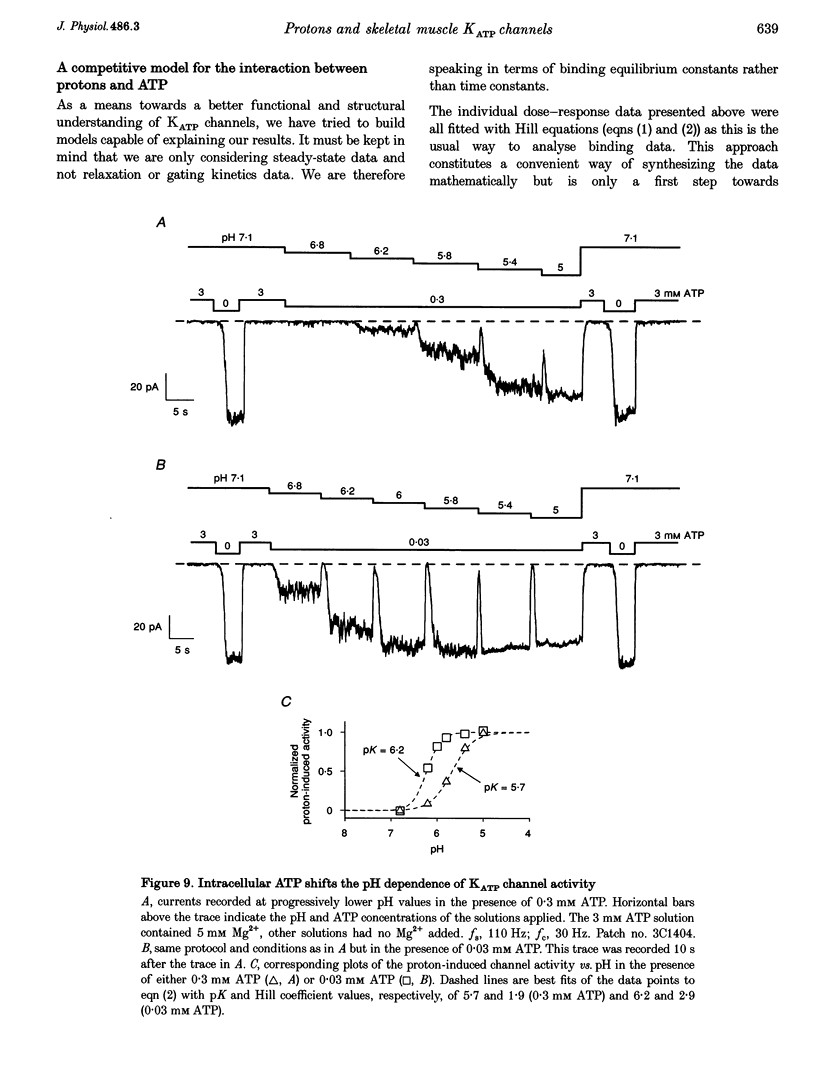
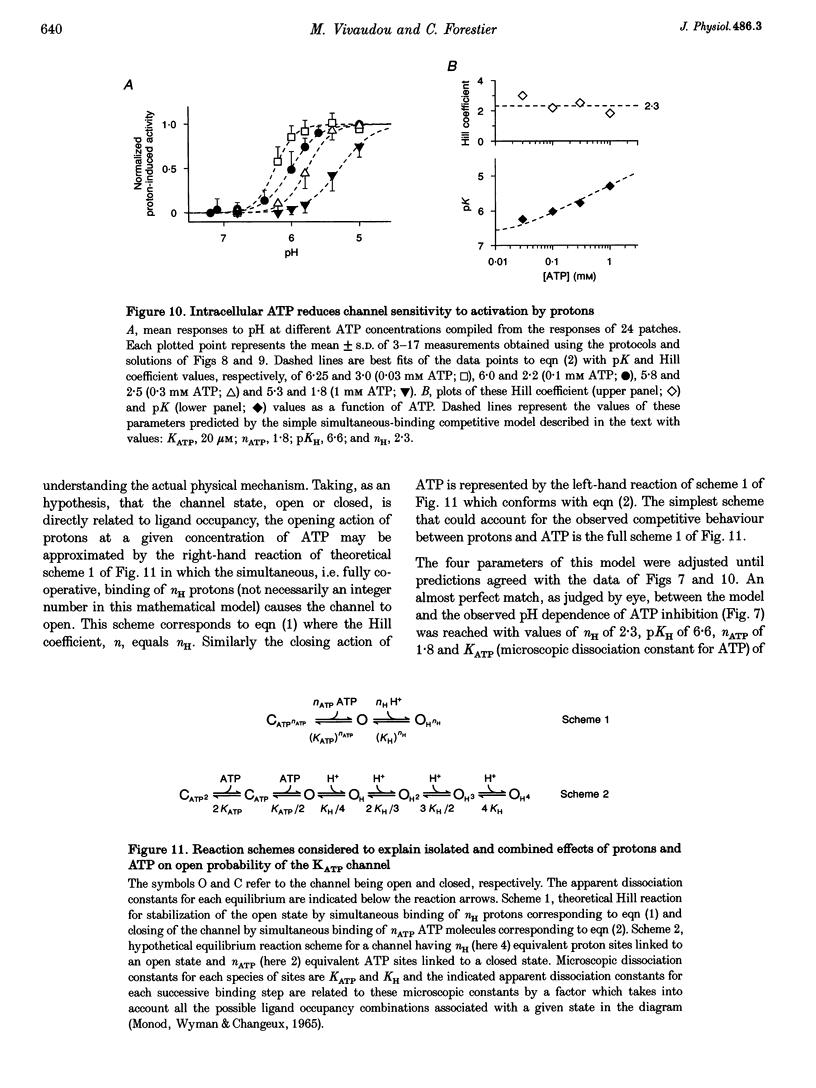
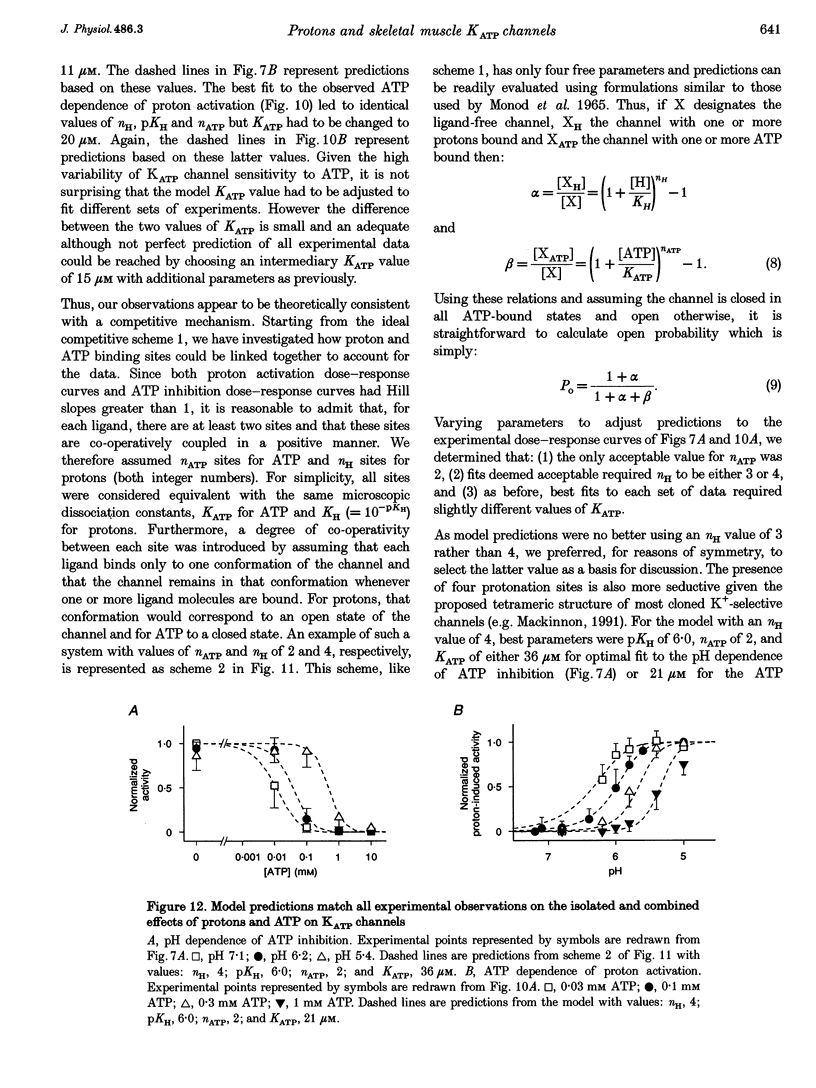
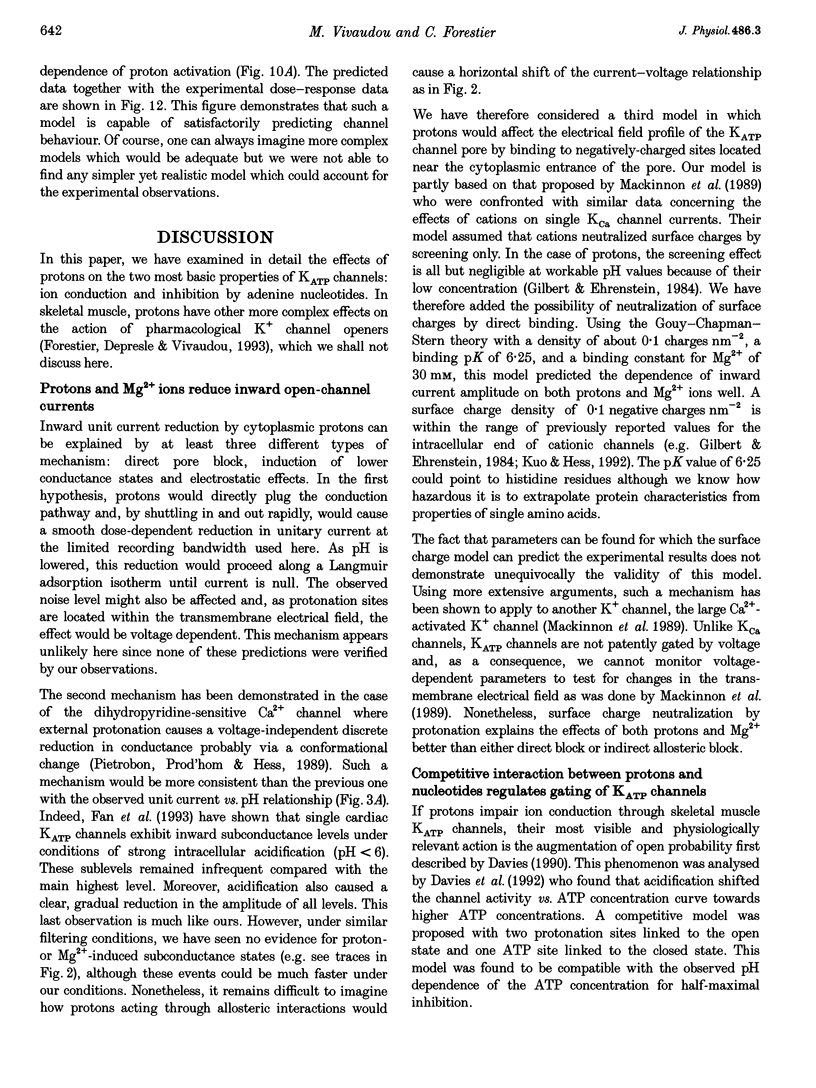
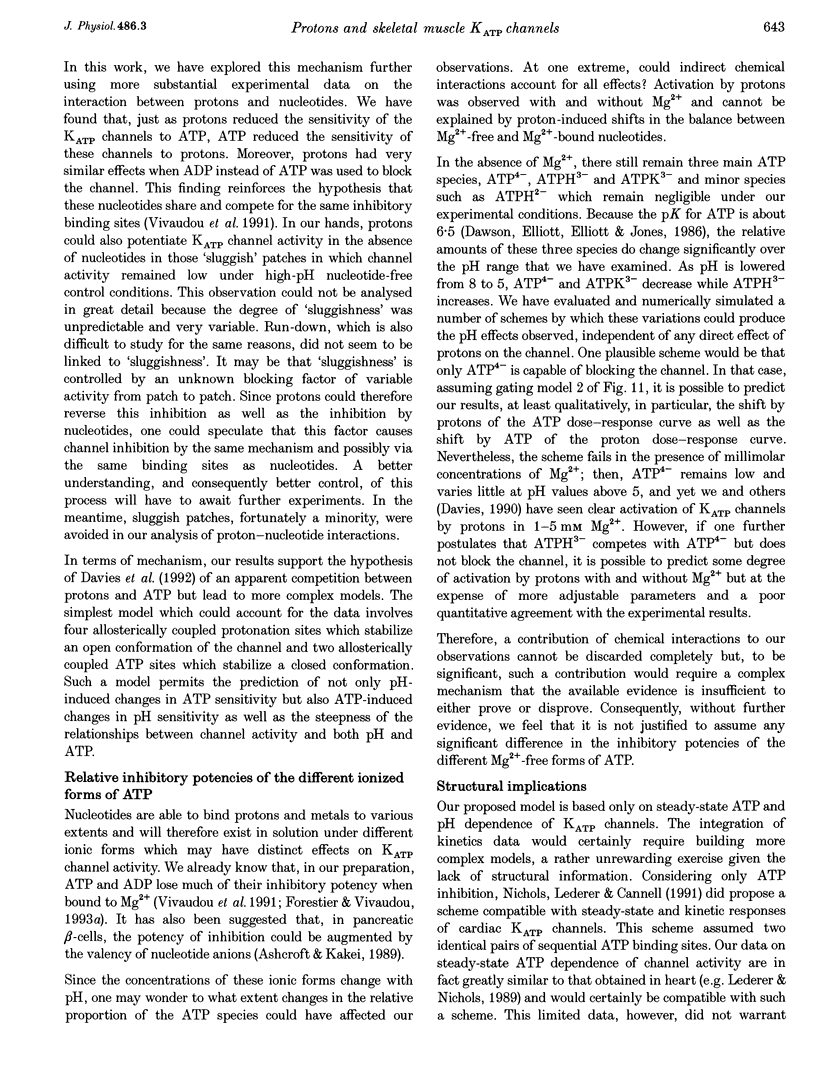
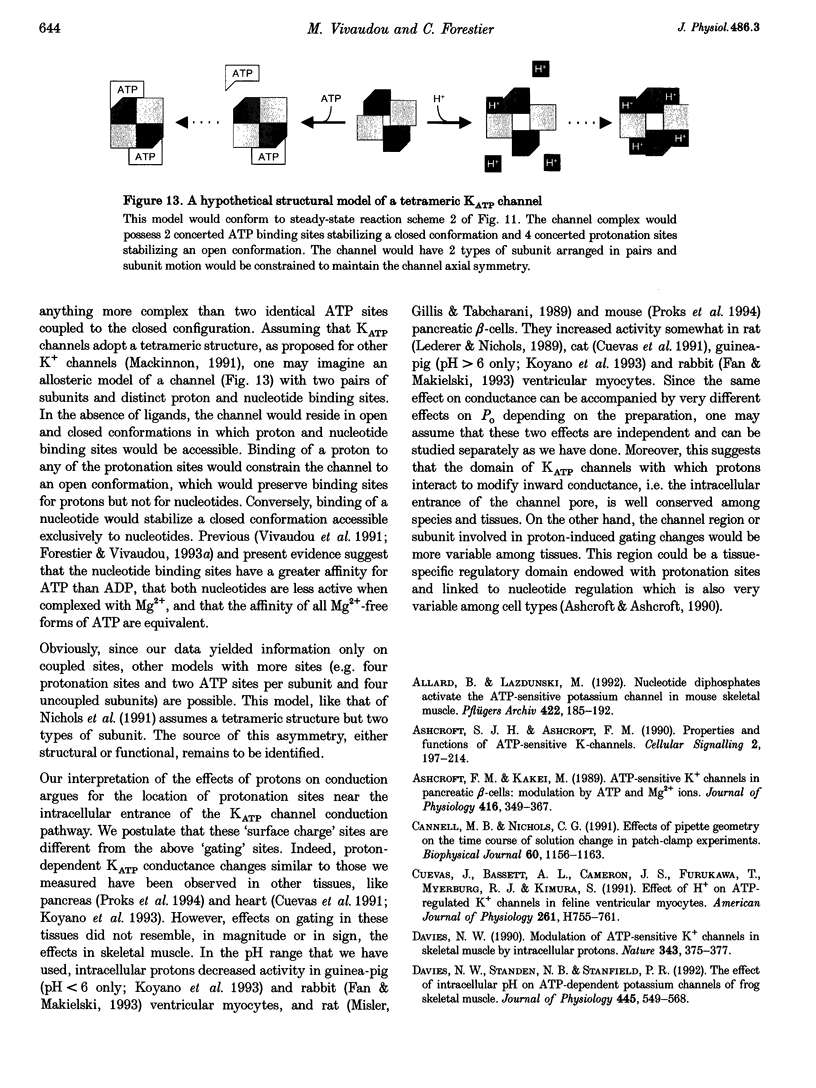
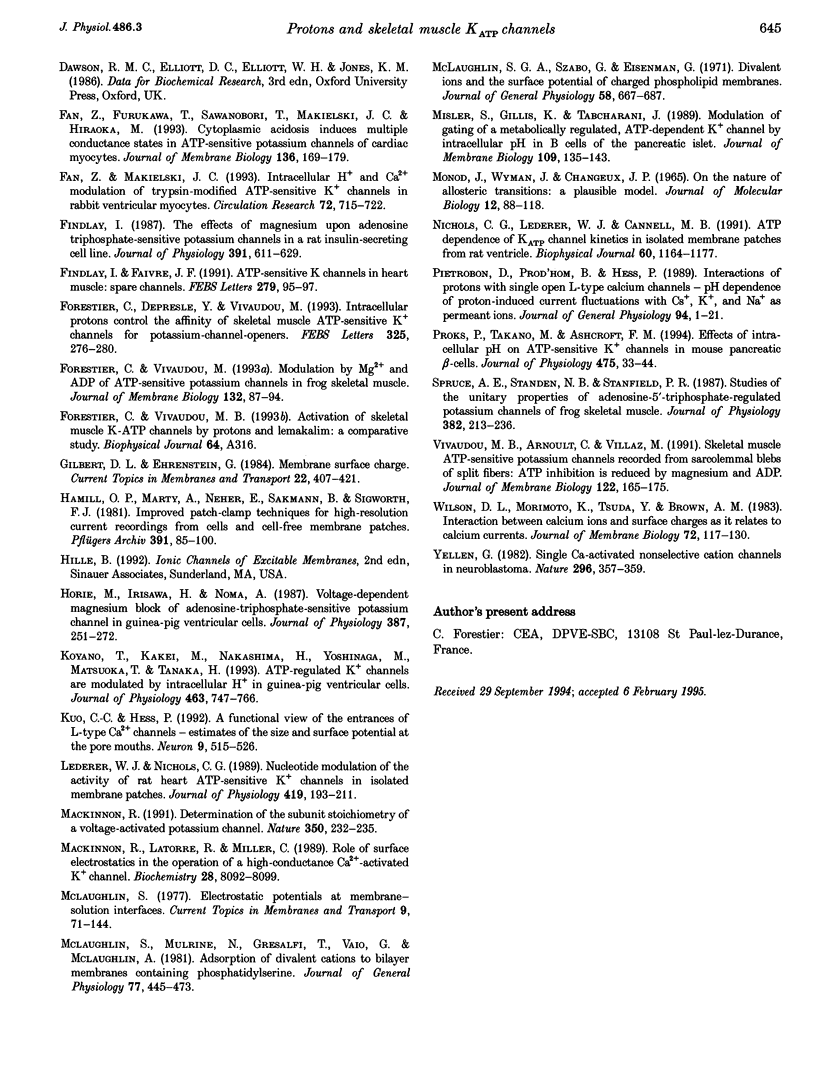
Images in this article
Selected References
These references are in PubMed. This may not be the complete list of references from this article.
- Allard B., Lazdunski M. Nucleotide diphosphates activate the ATP-sensitive potassium channel in mouse skeletal muscle. Pflugers Arch. 1992 Nov;422(2):185–192. doi: 10.1007/BF00370419. [DOI] [PubMed] [Google Scholar]
- Ashcroft F. M., Kakei M. ATP-sensitive K+ channels in rat pancreatic beta-cells: modulation by ATP and Mg2+ ions. J Physiol. 1989 Sep;416:349–367. doi: 10.1113/jphysiol.1989.sp017765. [DOI] [PMC free article] [PubMed] [Google Scholar]
- Ashcroft S. J., Ashcroft F. M. Properties and functions of ATP-sensitive K-channels. Cell Signal. 1990;2(3):197–214. doi: 10.1016/0898-6568(90)90048-f. [DOI] [PubMed] [Google Scholar]
- Cannell M. B., Nichols C. G. Effects of pipette geometry on the time course of solution change in patch clamp experiments. Biophys J. 1991 Nov;60(5):1156–1163. doi: 10.1016/S0006-3495(91)82151-8. [DOI] [PMC free article] [PubMed] [Google Scholar]
- Cuevas J., Bassett A. L., Cameron J. S., Furukawa T., Myerburg R. J., Kimura S. Effect of H+ on ATP-regulated K+ channels in feline ventricular myocytes. Am J Physiol. 1991 Sep;261(3 Pt 2):H755–H761. doi: 10.1152/ajpheart.1991.261.3.H755. [DOI] [PubMed] [Google Scholar]
- Davies N. W. Modulation of ATP-sensitive K+ channels in skeletal muscle by intracellular protons. Nature. 1990 Jan 25;343(6256):375–377. doi: 10.1038/343375a0. [DOI] [PubMed] [Google Scholar]
- Davies N. W., Standen N. B., Stanfield P. R. The effect of intracellular pH on ATP-dependent potassium channels of frog skeletal muscle. J Physiol. 1992 Jan;445:549–568. doi: 10.1113/jphysiol.1992.sp018939. [DOI] [PMC free article] [PubMed] [Google Scholar]
- Fan Z., Furukawa T., Sawanobori T., Makielski J. C., Hiraoka M. Cytoplasmic acidosis induces multiple conductance states in ATP-sensitive potassium channels of cardiac myocytes. J Membr Biol. 1993 Nov;136(2):169–179. doi: 10.1007/BF02505761. [DOI] [PubMed] [Google Scholar]
- Fan Z., Makielski J. C. Intracellular H+ and Ca2+ modulation of trypsin-modified ATP-sensitive K+ channels in rabbit ventricular myocytes. Circ Res. 1993 Mar;72(3):715–722. doi: 10.1161/01.res.72.3.715. [DOI] [PubMed] [Google Scholar]
- Findlay I., Faivre J. F. ATP-sensitive K channels in heart muscle. Spare channels. FEBS Lett. 1991 Feb 11;279(1):95–97. doi: 10.1016/0014-5793(91)80259-6. [DOI] [PubMed] [Google Scholar]
- Findlay I. The effects of magnesium upon adenosine triphosphate-sensitive potassium channels in a rat insulin-secreting cell line. J Physiol. 1987 Oct;391:611–629. doi: 10.1113/jphysiol.1987.sp016759. [DOI] [PMC free article] [PubMed] [Google Scholar]
- Forestier C., Depresle Y., Vivaudou M. Intracellular protons control the affinity of skeletal muscle ATP-sensitive K+ channels for potassium-channel-openers. FEBS Lett. 1993 Jul 5;325(3):276–280. doi: 10.1016/0014-5793(93)81088-h. [DOI] [PubMed] [Google Scholar]
- Forestier C., Vivaudou M. Modulation by Mg2+ and ADP of ATP-sensitive potassium channels in frog skeletal muscle. J Membr Biol. 1993 Feb;132(1):87–94. doi: 10.1007/BF00233054. [DOI] [PubMed] [Google Scholar]
- Hamill O. P., Marty A., Neher E., Sakmann B., Sigworth F. J. Improved patch-clamp techniques for high-resolution current recording from cells and cell-free membrane patches. Pflugers Arch. 1981 Aug;391(2):85–100. doi: 10.1007/BF00656997. [DOI] [PubMed] [Google Scholar]
- Horie M., Irisawa H., Noma A. Voltage-dependent magnesium block of adenosine-triphosphate-sensitive potassium channel in guinea-pig ventricular cells. J Physiol. 1987 Jun;387:251–272. doi: 10.1113/jphysiol.1987.sp016572. [DOI] [PMC free article] [PubMed] [Google Scholar]
- Koyano T., Kakei M., Nakashima H., Yoshinaga M., Matsuoka T., Tanaka H. ATP-regulated K+ channels are modulated by intracellular H+ in guinea-pig ventricular cells. J Physiol. 1993 Apr;463:747–766. doi: 10.1113/jphysiol.1993.sp019620. [DOI] [PMC free article] [PubMed] [Google Scholar]
- Kuo C. C., Hess P. A functional view of the entrances of L-type Ca2+ channels: estimates of the size and surface potential at the pore mouths. Neuron. 1992 Sep;9(3):515–526. doi: 10.1016/0896-6273(92)90189-k. [DOI] [PubMed] [Google Scholar]
- Lederer W. J., Nichols C. G. Nucleotide modulation of the activity of rat heart ATP-sensitive K+ channels in isolated membrane patches. J Physiol. 1989 Dec;419:193–211. doi: 10.1113/jphysiol.1989.sp017869. [DOI] [PMC free article] [PubMed] [Google Scholar]
- MONOD J., WYMAN J., CHANGEUX J. P. ON THE NATURE OF ALLOSTERIC TRANSITIONS: A PLAUSIBLE MODEL. J Mol Biol. 1965 May;12:88–118. doi: 10.1016/s0022-2836(65)80285-6. [DOI] [PubMed] [Google Scholar]
- MacKinnon R. Determination of the subunit stoichiometry of a voltage-activated potassium channel. Nature. 1991 Mar 21;350(6315):232–235. doi: 10.1038/350232a0. [DOI] [PubMed] [Google Scholar]
- MacKinnon R., Latorre R., Miller C. Role of surface electrostatics in the operation of a high-conductance Ca2+-activated K+ channel. Biochemistry. 1989 Oct 3;28(20):8092–8099. doi: 10.1021/bi00446a020. [DOI] [PubMed] [Google Scholar]
- McLaughlin S. G., Szabo G., Eisenman G. Divalent ions and the surface potential of charged phospholipid membranes. J Gen Physiol. 1971 Dec;58(6):667–687. doi: 10.1085/jgp.58.6.667. [DOI] [PMC free article] [PubMed] [Google Scholar]
- McLaughlin S., Mulrine N., Gresalfi T., Vaio G., McLaughlin A. Adsorption of divalent cations to bilayer membranes containing phosphatidylserine. J Gen Physiol. 1981 Apr;77(4):445–473. doi: 10.1085/jgp.77.4.445. [DOI] [PMC free article] [PubMed] [Google Scholar]
- Misler S., Gillis K., Tabcharani J. Modulation of gating of a metabolically regulated, ATP-dependent K+ channel by intracellular pH in B cells of the pancreatic islet. J Membr Biol. 1989 Jul;109(2):135–143. doi: 10.1007/BF01870852. [DOI] [PubMed] [Google Scholar]
- Nichols C. G., Lederer W. J., Cannell M. B. ATP dependence of KATP channel kinetics in isolated membrane patches from rat ventricle. Biophys J. 1991 Nov;60(5):1164–1177. doi: 10.1016/S0006-3495(91)82152-X. [DOI] [PMC free article] [PubMed] [Google Scholar]
- Pietrobon D., Prod'hom B., Hess P. Interactions of protons with single open L-type calcium channels. pH dependence of proton-induced current fluctuations with Cs+, K+, and Na+ as permeant ions. J Gen Physiol. 1989 Jul;94(1):1–21. doi: 10.1085/jgp.94.1.1. [DOI] [PMC free article] [PubMed] [Google Scholar]
- Proks P., Takano M., Ashcroft F. M. Effects of intracellular pH on ATP-sensitive K+ channels in mouse pancreatic beta-cells. J Physiol. 1994 Feb 15;475(1):33–44. doi: 10.1113/jphysiol.1994.sp020047. [DOI] [PMC free article] [PubMed] [Google Scholar]
- Spruce A. E., Standen N. B., Stanfield P. R. Studies of the unitary properties of adenosine-5'-triphosphate-regulated potassium channels of frog skeletal muscle. J Physiol. 1987 Jan;382:213–236. doi: 10.1113/jphysiol.1987.sp016364. [DOI] [PMC free article] [PubMed] [Google Scholar]
- Vivaudou M. B., Arnoult C., Villaz M. Skeletal muscle ATP-sensitive K+ channels recorded from sarcolemmal blebs of split fibers: ATP inhibition is reduced by magnesium and ADP. J Membr Biol. 1991 Jun;122(2):165–175. doi: 10.1007/BF01872639. [DOI] [PubMed] [Google Scholar]
- Wilson D. L., Morimoto K., Tsuda Y., Brown A. M. Interaction between calcium ions and surface charge as it relates to calcium currents. J Membr Biol. 1983;72(1-2):117–130. doi: 10.1007/BF01870319. [DOI] [PubMed] [Google Scholar]
- Yellen G. Single Ca2+-activated nonselective cation channels in neuroblastoma. Nature. 1982 Mar 25;296(5855):357–359. doi: 10.1038/296357a0. [DOI] [PubMed] [Google Scholar]