Abstract
1. Thin brainstem slices (150 microns thickness) were taken from mature rats, and membrane potentials (Em) and currents (Im) in the dorsal vagal neurons (DVN) were analysed with whole-cell patch clamp techniques during anoxia. 2. At a holding potential (Vh) of -50 mV, a sustained anoxia-induced outward current (AOC) of 92 +/- 44 pA (reversal potential (Erev), -78 +/- 12 mV) and a concomitant increase of membrane conductance (gm) from 2.2 +/- 0.45 to 5.9 +/- 2.4 nS were revealed in 40% of 142 DVN analysed. The AOC led to a hyperpolarization of the cells by 14.4 +/- 6.1 mV from a mean resting Em of -51 +/- 6 mV, and to blockade of spontaneous action potential discharges. In the remaining DVN, anoxia had almost no effect on Em, Im or gm and did not block spontaneous action potential discharges. 3. The AOC was not affected by 0.5 microM tetrodotoxin (TTX), 2 mM Mn2+, 50 microM cyanonitroquinoxaline dione (CNQX) or 100 microM bicuculline. 4. Elevation of the extracellular [K+] from 3 to 10 mM resulted in a positive shift of Erev of the AOC by 23 mV, whereas an increase in the [Cl-] of the patch pipette solution from 5 to 144 mM had no effect on Erev. 5. In DVN responding with an AOC, addition of 200 microM diazoxide, an activator of ATP-sensitive K+ (KATP) channels, to oxygenated solutions elicited a similar outward current (Erev = -79 +/- 5.5 mV, n = 12) and increase in gm. Diazoxide did not affect Em, Im or gm in cells which did not show an AOC. 6. In a subpopulation of DVN (n = 26), spontaneous activation of a KATP current with an Erev of -80 +/- 6 mV was observed. As analysed in four of these cells, an AOC was revealed during the initial phase of development of the spontaneous outward current but not under steady-state conditions. 7. The AOC, the diazoxide-induced current, and the spontaneous outward current were completely blocked upon bath application of the KATP channel blocker tolbutamide (100-200 microM). 8. The results indicate that the sustained anoxia-induced outward current of dorsal vagal neurons is due to activation of KATP channels. A possible physiological role of functional inactivation of these cells during metabolic disturbances is discussed.
Full text
PDF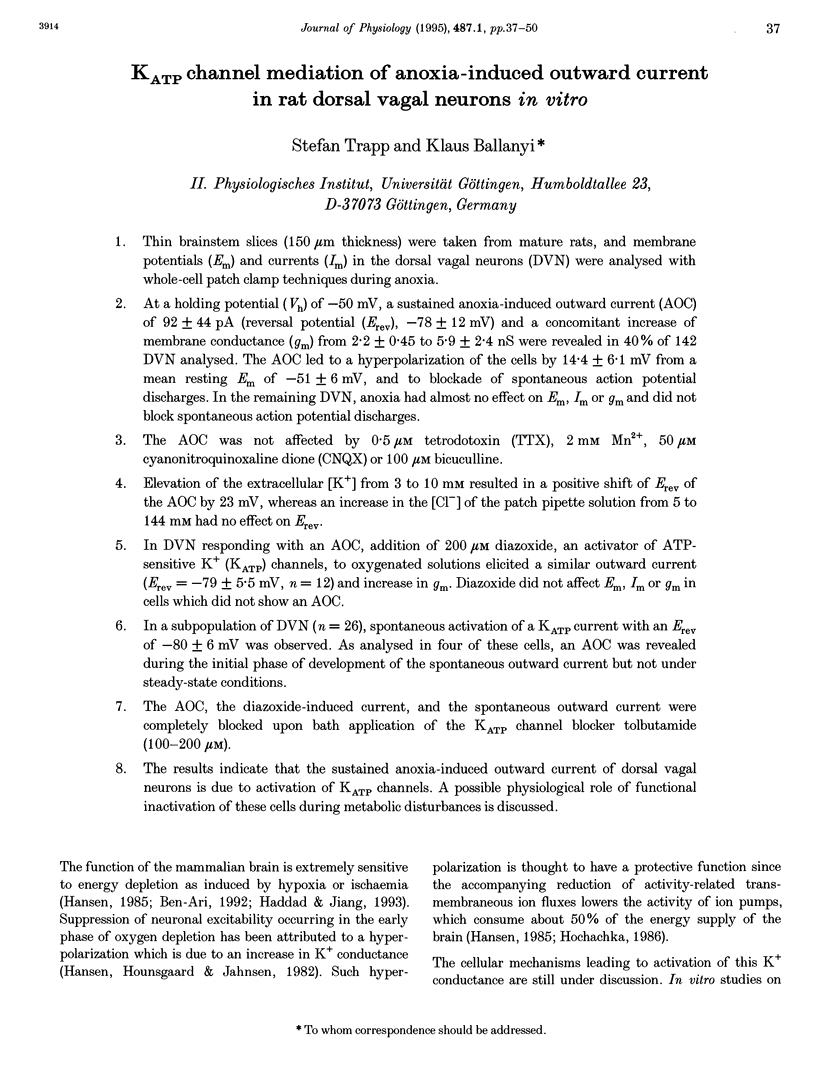
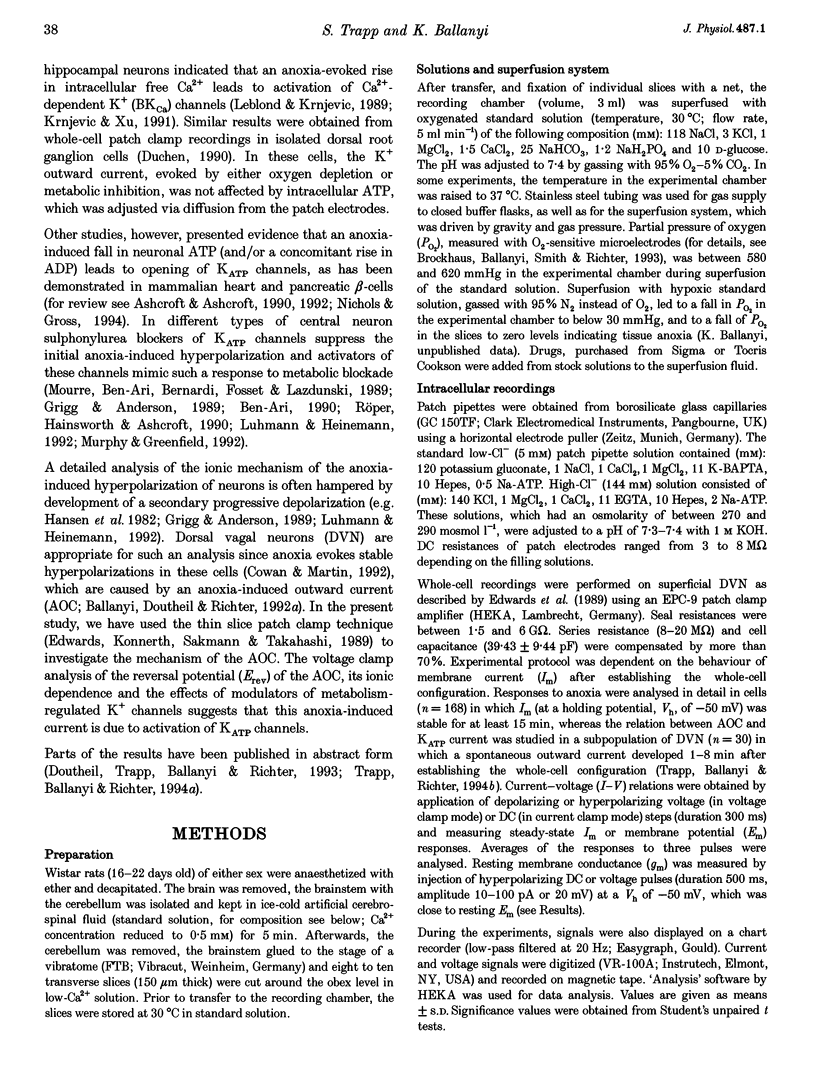
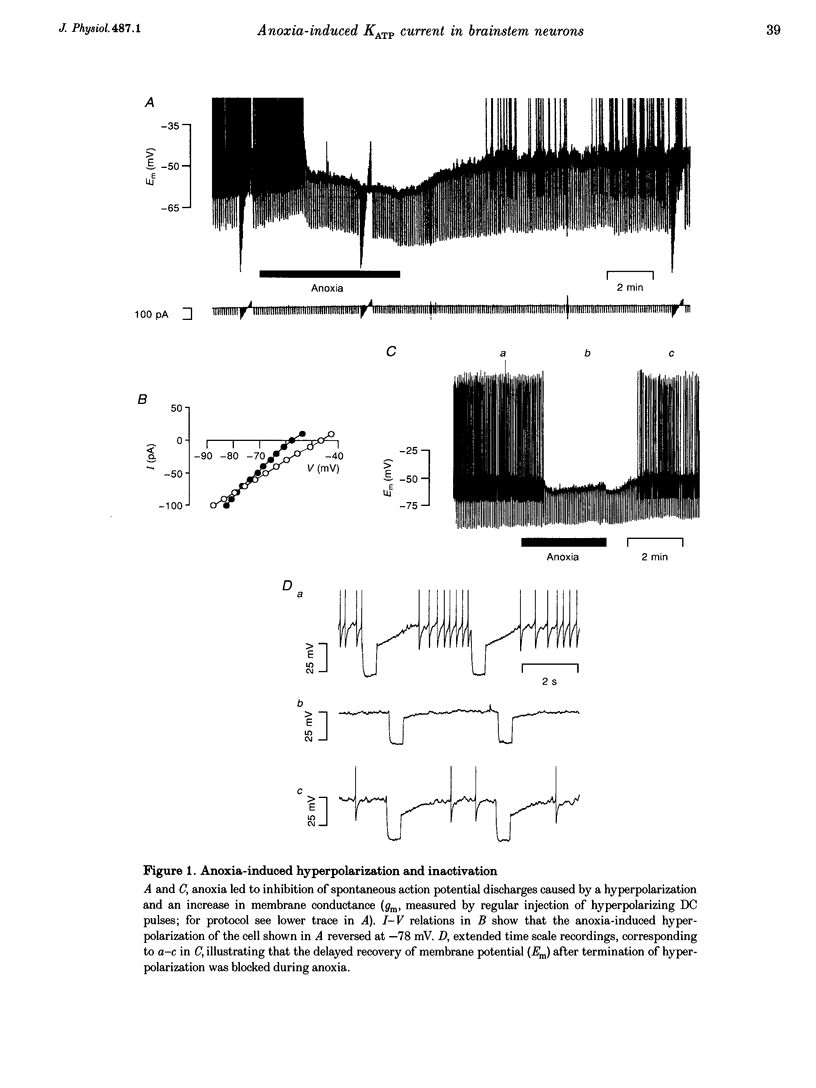
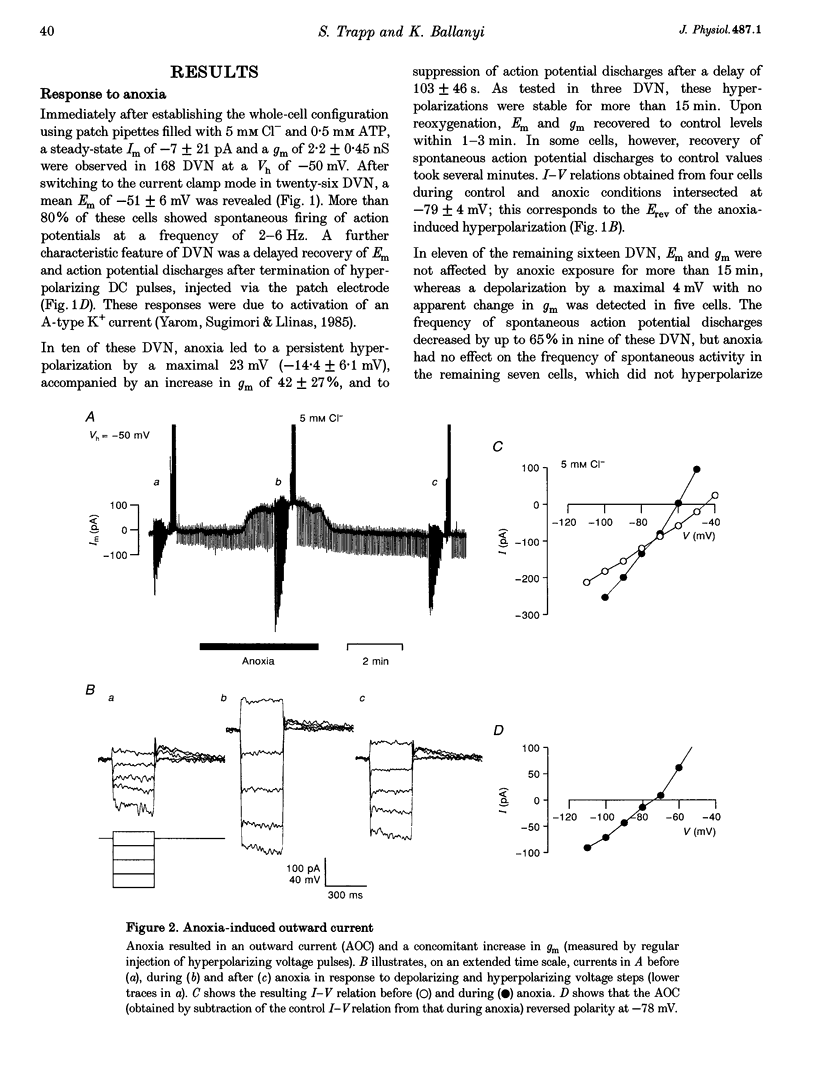
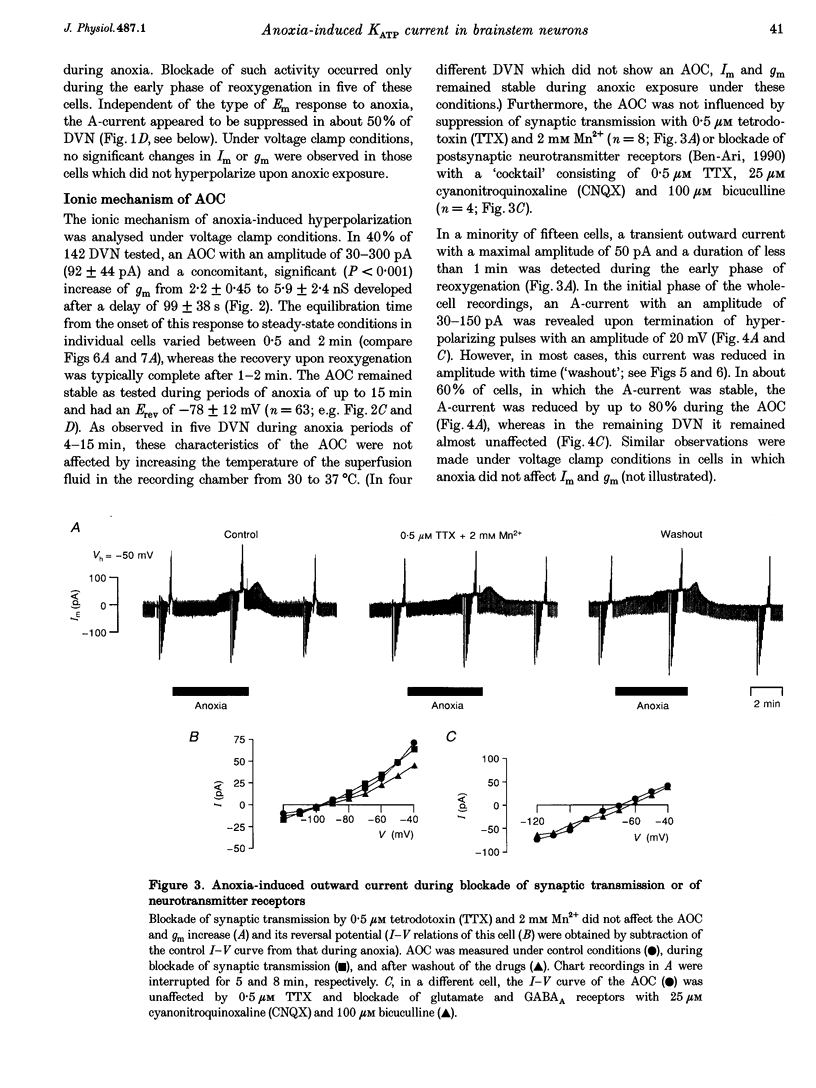
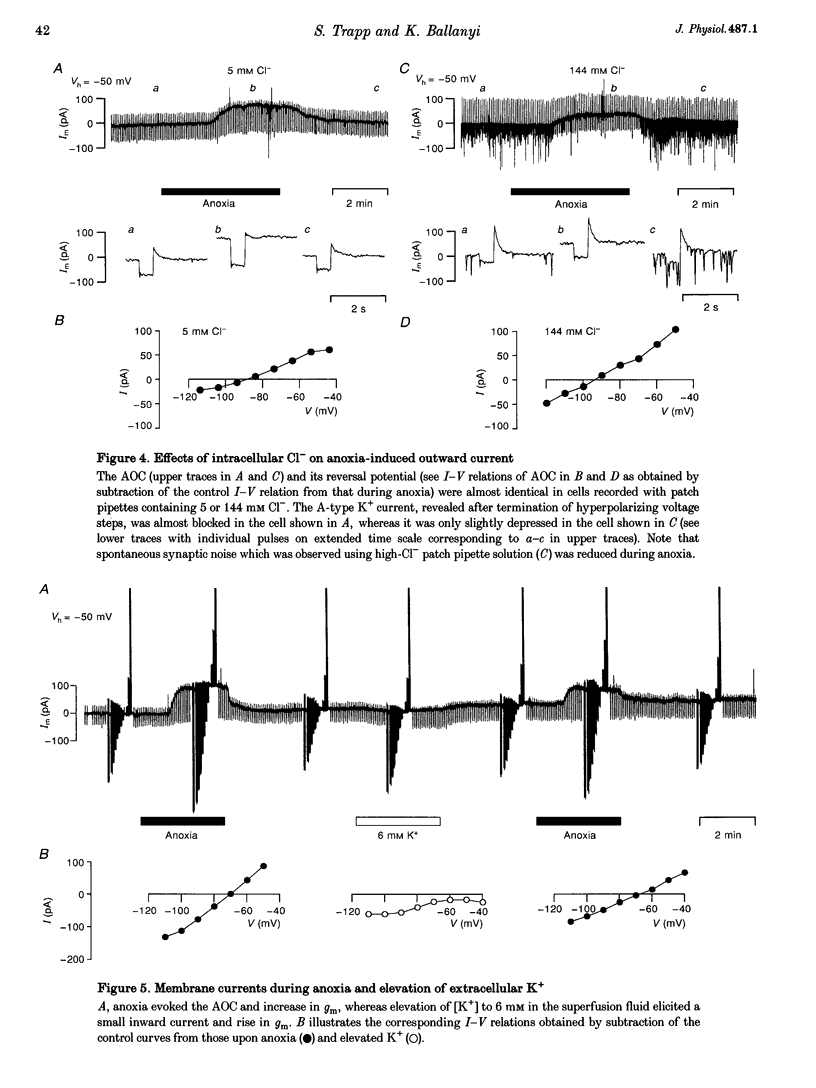
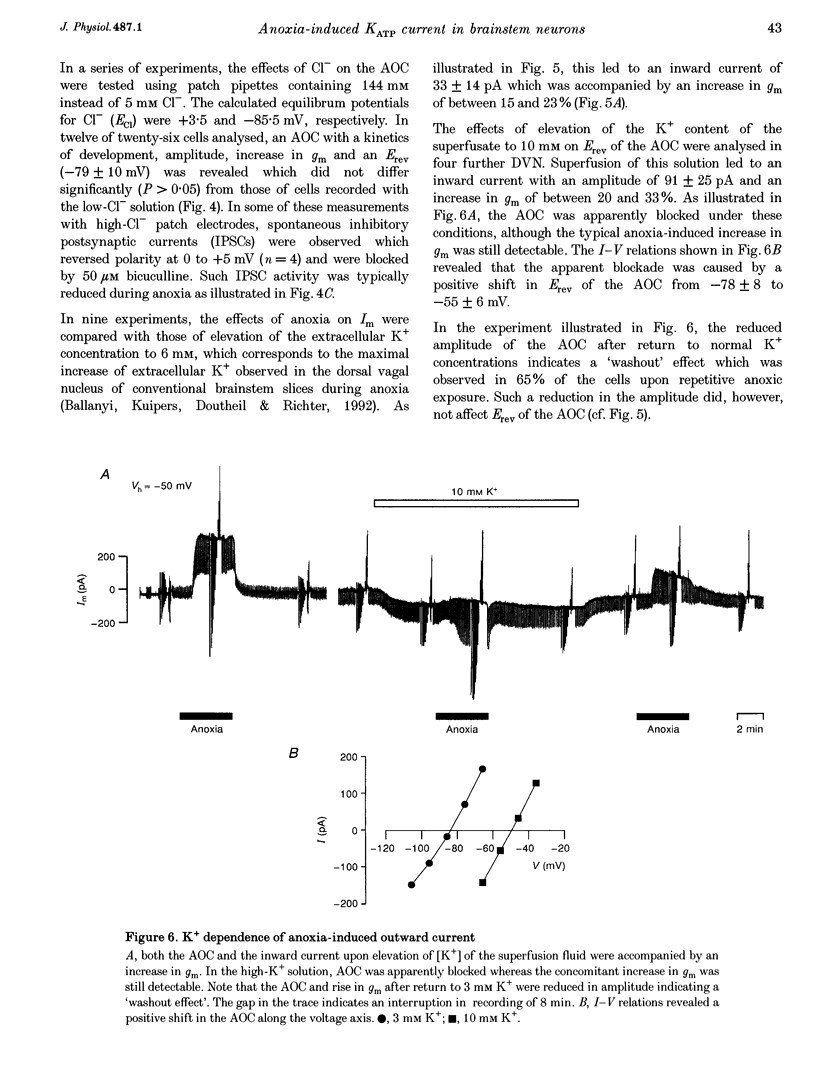
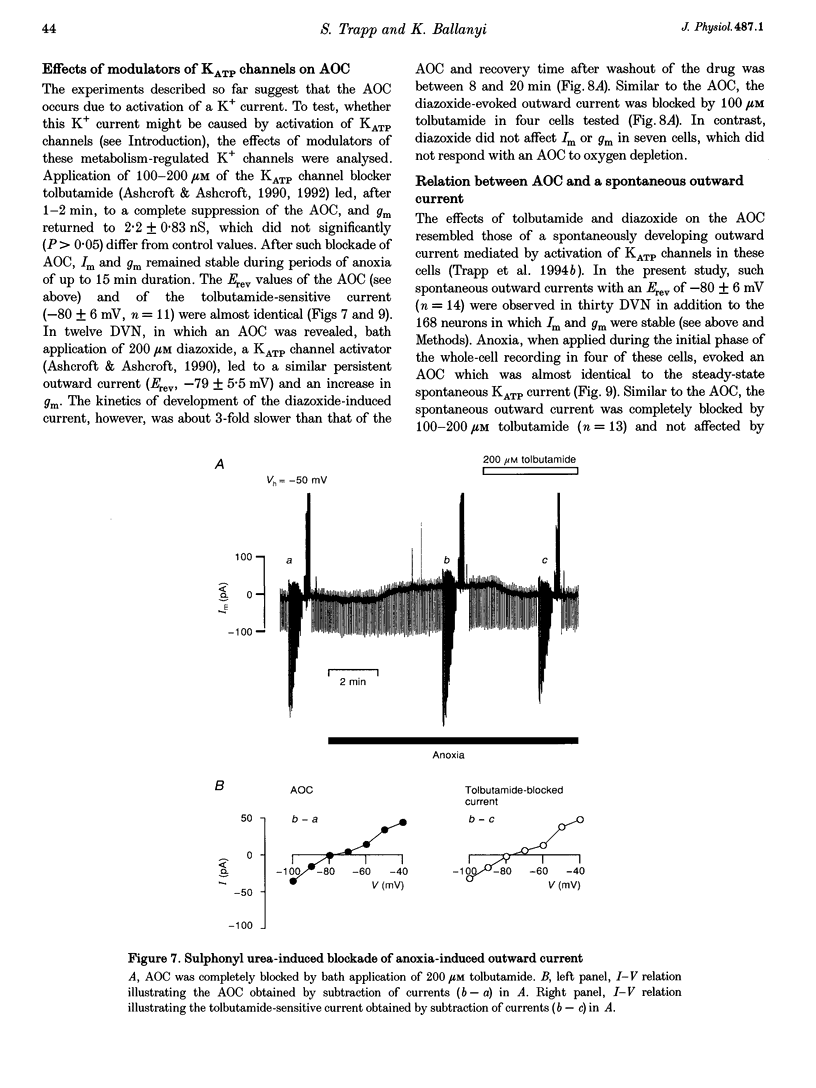
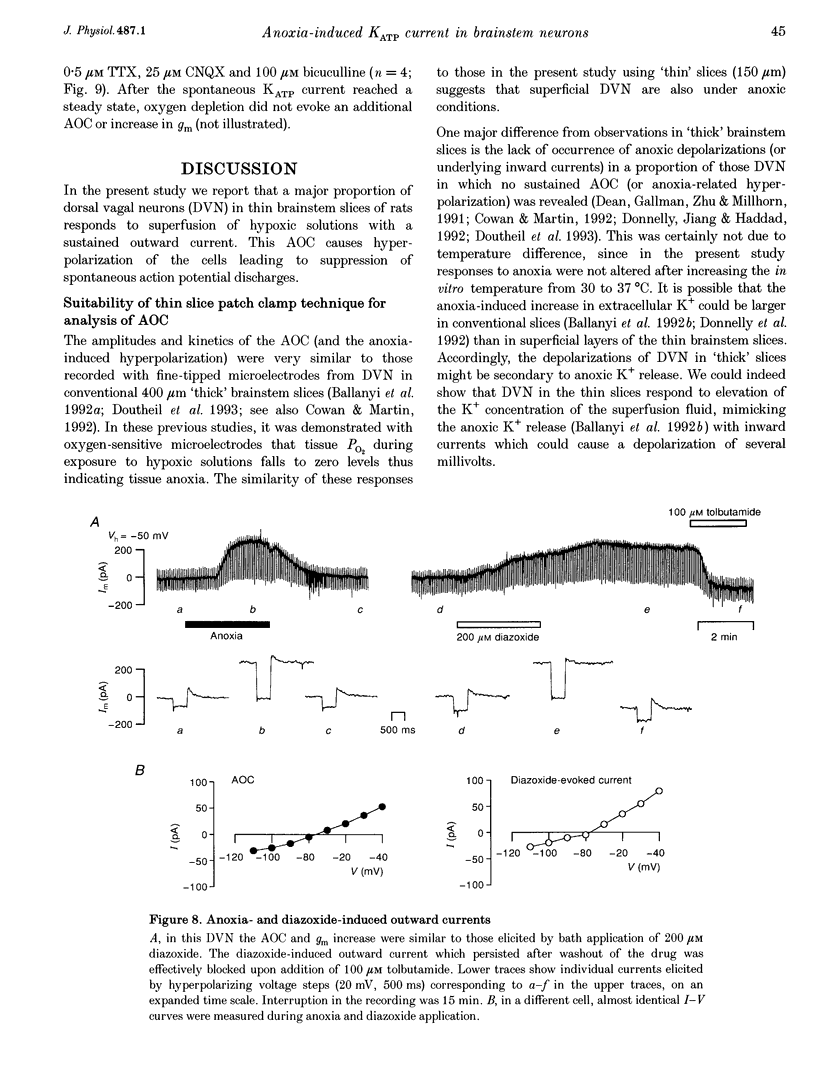
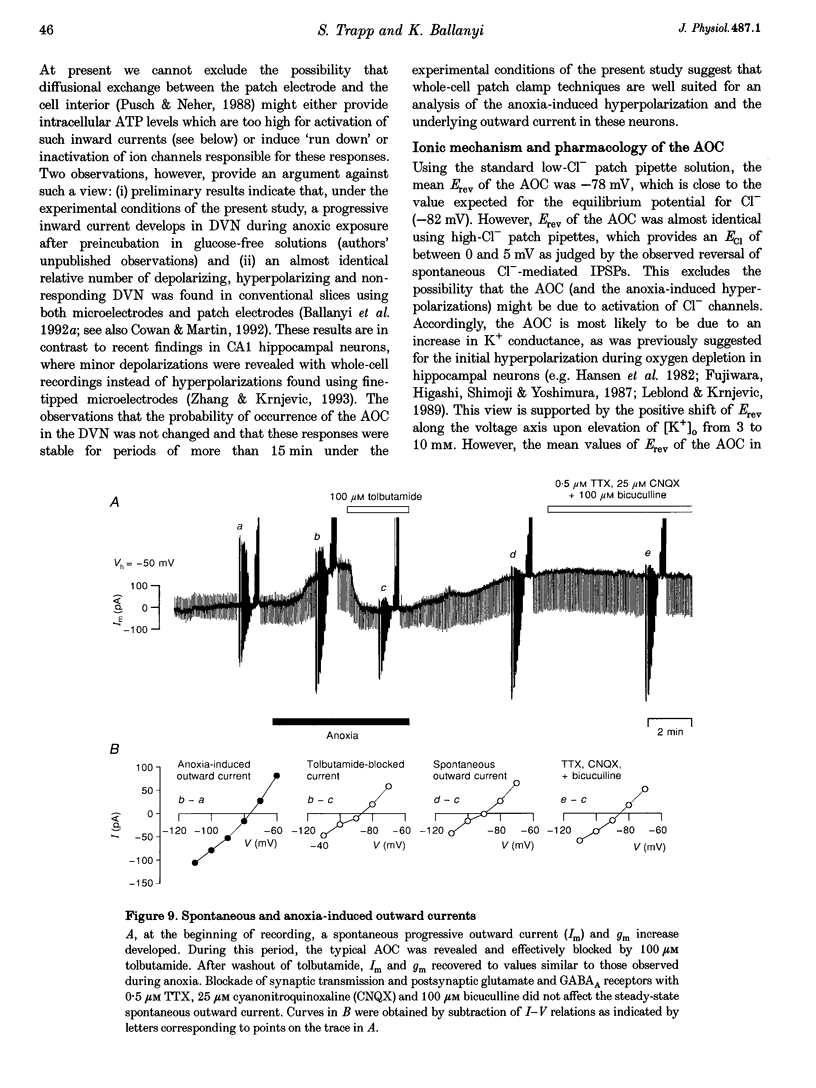
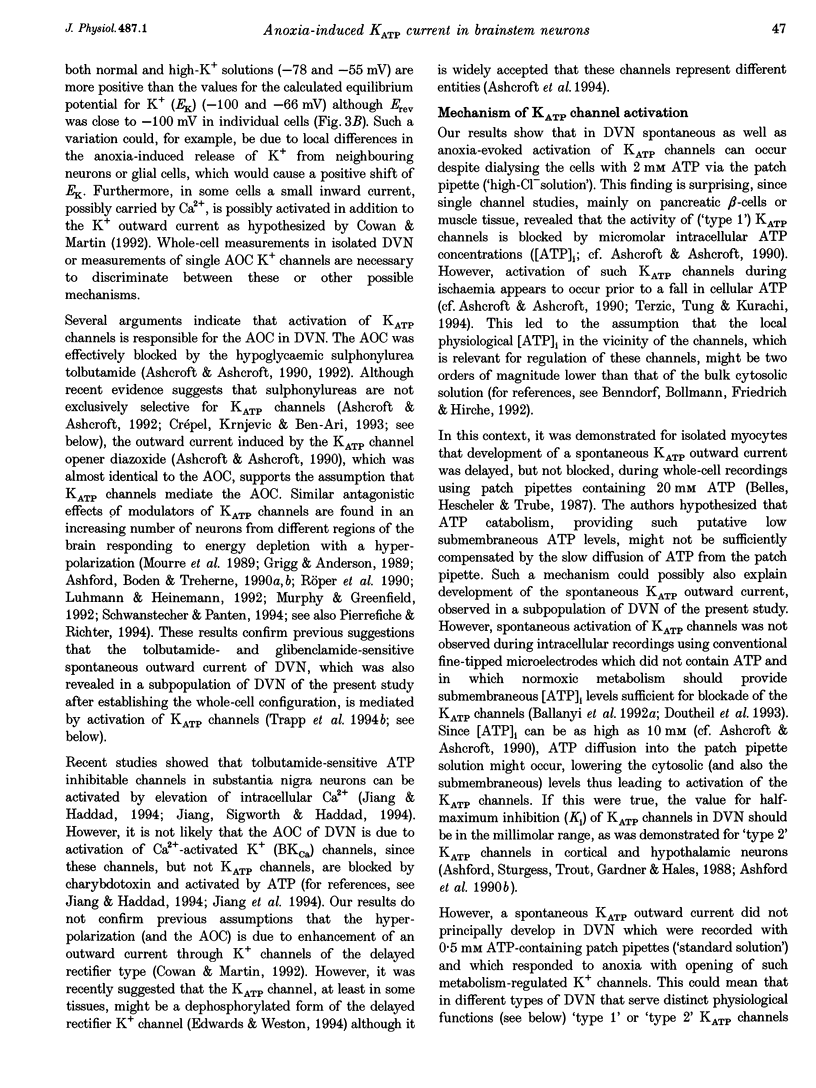
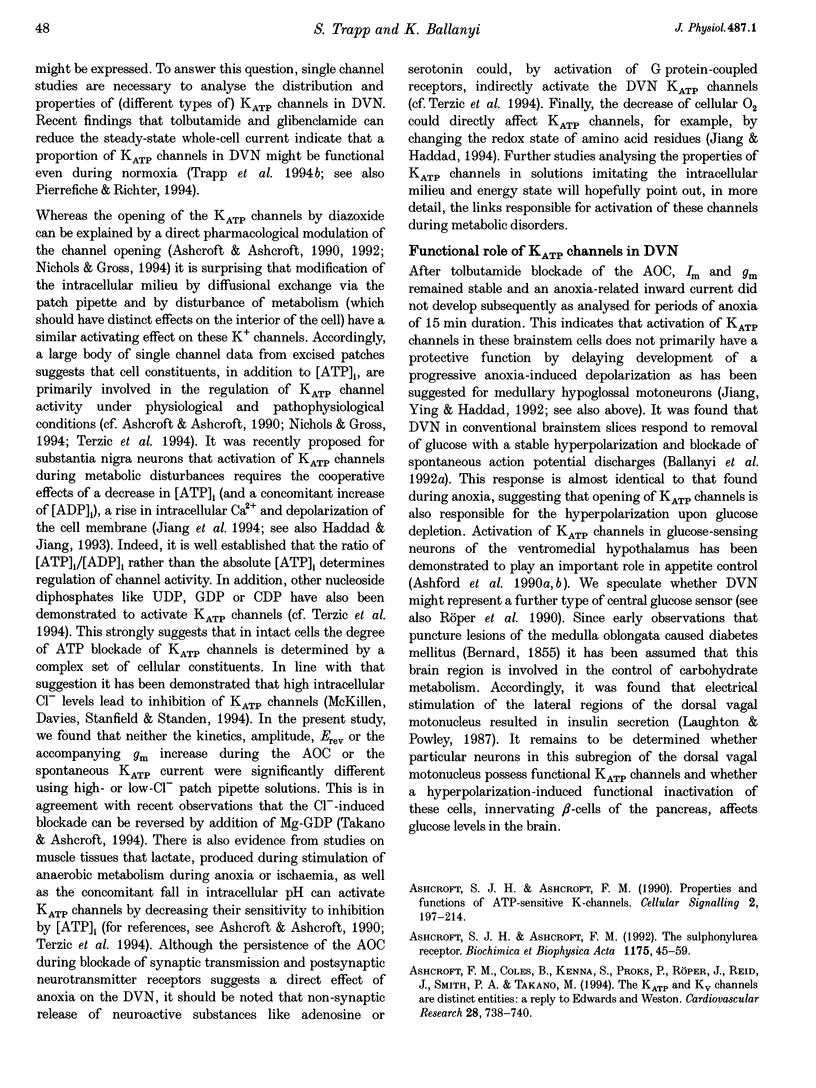
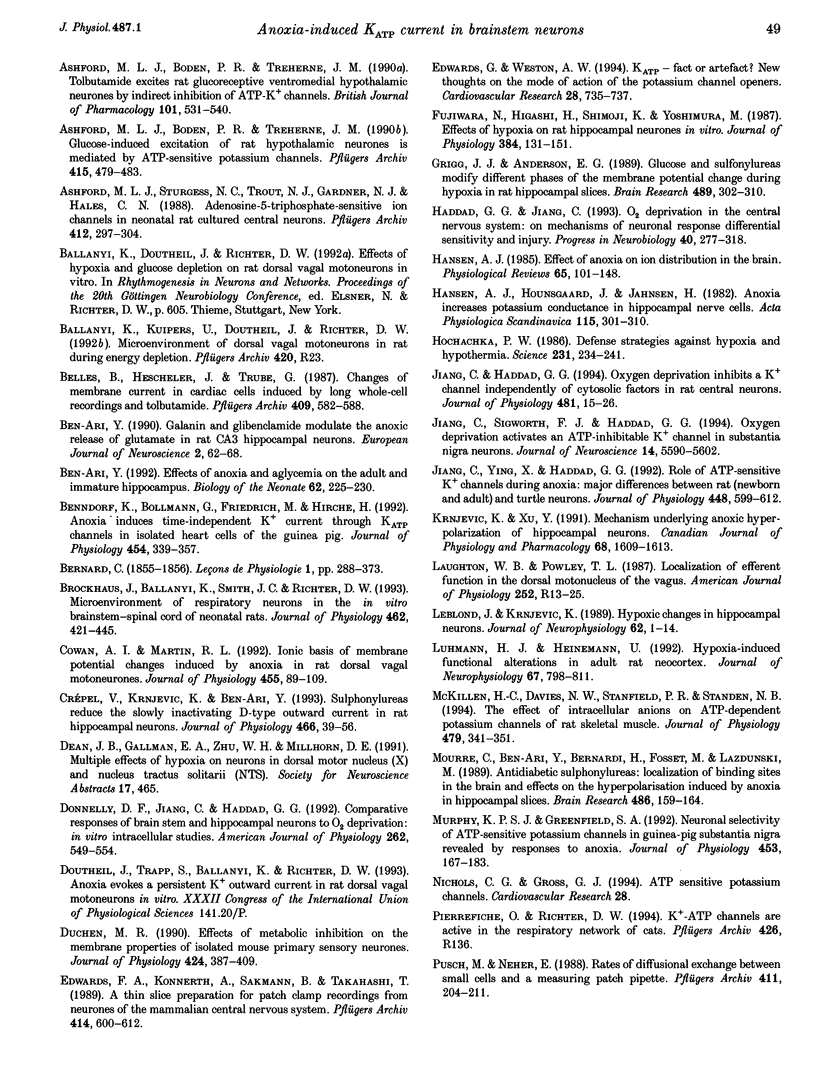
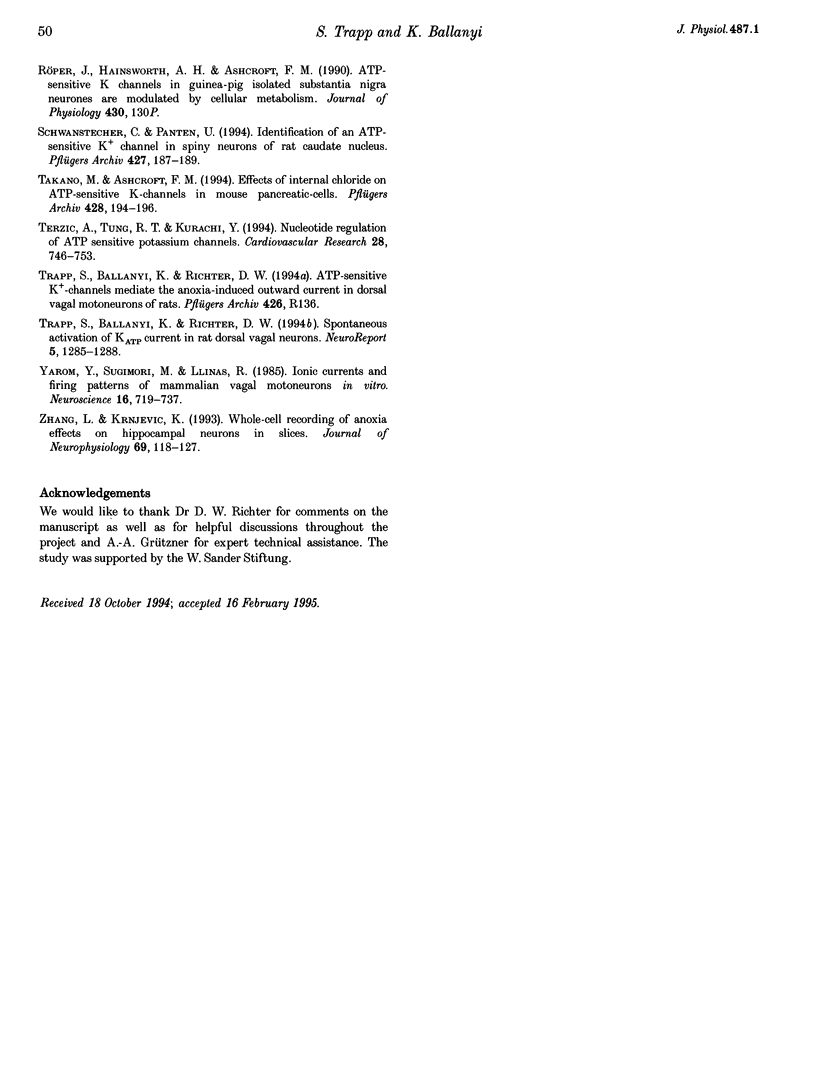
Selected References
These references are in PubMed. This may not be the complete list of references from this article.
- Ashcroft F. M., Coles B., Kenna S., Proks P., Röper J., Reid J., Smith P. A., Takano M. The KATP and Kv channels are distinct entities: a reply to Edwards and Weston. Cardiovasc Res. 1994 Jun;28(6):738–745. doi: 10.1093/cvr/28.6.738. [DOI] [PubMed] [Google Scholar]
- Ashcroft S. J., Ashcroft F. M. Properties and functions of ATP-sensitive K-channels. Cell Signal. 1990;2(3):197–214. doi: 10.1016/0898-6568(90)90048-f. [DOI] [PubMed] [Google Scholar]
- Ashcroft S. J., Ashcroft F. M. The sulfonylurea receptor. Biochim Biophys Acta. 1992 Dec 15;1175(1):45–59. doi: 10.1016/0167-4889(92)90008-y. [DOI] [PubMed] [Google Scholar]
- Ashford M. L., Boden P. R., Treherne J. M. Glucose-induced excitation of hypothalamic neurones is mediated by ATP-sensitive K+ channels. Pflugers Arch. 1990 Jan;415(4):479–483. doi: 10.1007/BF00373626. [DOI] [PubMed] [Google Scholar]
- Ashford M. L., Boden P. R., Treherne J. M. Tolbutamide excites rat glucoreceptive ventromedial hypothalamic neurones by indirect inhibition of ATP-K+ channels. Br J Pharmacol. 1990 Nov;101(3):531–540. doi: 10.1111/j.1476-5381.1990.tb14116.x. [DOI] [PMC free article] [PubMed] [Google Scholar]
- Ashford M. L., Sturgess N. C., Trout N. J., Gardner N. J., Hales C. N. Adenosine-5'-triphosphate-sensitive ion channels in neonatal rat cultured central neurones. Pflugers Arch. 1988 Aug;412(3):297–304. doi: 10.1007/BF00582512. [DOI] [PubMed] [Google Scholar]
- Belles B., Hescheler J., Trube G. Changes of membrane currents in cardiac cells induced by long whole-cell recordings and tolbutamide. Pflugers Arch. 1987 Aug;409(6):582–588. doi: 10.1007/BF00584657. [DOI] [PubMed] [Google Scholar]
- Ben-Ari Y. Effects of anoxia and aglycemia on the adult and immature hippocampus. Biol Neonate. 1992;62(4):225–230. doi: 10.1159/000243875. [DOI] [PubMed] [Google Scholar]
- Ben-Ari Y. Galanin and Glibenclamide Modulate the Anoxic Release of Glutamate in Rat CA3 Hippocampal Neurons. Eur J Neurosci. 1990 Jan;2(1):62–68. doi: 10.1111/j.1460-9568.1990.tb00381.x. [DOI] [PubMed] [Google Scholar]
- Benndorf K., Bollmann G., Friedrich M., Hirche H. Anoxia induces time-independent K+ current through KATP channels in isolated heart cells of the guinea-pig. J Physiol. 1992 Aug;454:339–357. doi: 10.1113/jphysiol.1992.sp019267. [DOI] [PMC free article] [PubMed] [Google Scholar]
- Brockhaus J., Ballanyi K., Smith J. C., Richter D. W. Microenvironment of respiratory neurons in the in vitro brainstem-spinal cord of neonatal rats. J Physiol. 1993 Mar;462:421–445. doi: 10.1113/jphysiol.1993.sp019562. [DOI] [PMC free article] [PubMed] [Google Scholar]
- Cowan A. I., Martin R. L. Ionic basis of membrane potential changes induced by anoxia in rat dorsal vagal motoneurones. J Physiol. 1992 Sep;455:89–109. doi: 10.1113/jphysiol.1992.sp019292. [DOI] [PMC free article] [PubMed] [Google Scholar]
- Crépel V., Krnjević K., Ben-Ari Y. Sulphonylureas reduce the slowly inactivating D-type outward current in rat hippocampal neurons. J Physiol. 1993 Jul;466:39–54. [PMC free article] [PubMed] [Google Scholar]
- Duchen M. R. Effects of metabolic inhibition on the membrane properties of isolated mouse primary sensory neurones. J Physiol. 1990 May;424:387–409. doi: 10.1113/jphysiol.1990.sp018073. [DOI] [PMC free article] [PubMed] [Google Scholar]
- Edwards F. A., Konnerth A., Sakmann B., Takahashi T. A thin slice preparation for patch clamp recordings from neurones of the mammalian central nervous system. Pflugers Arch. 1989 Sep;414(5):600–612. doi: 10.1007/BF00580998. [DOI] [PubMed] [Google Scholar]
- Edwards G., Weston A. H. KATP--fact or artefact? New thoughts on the mode of action of the potassium channel openers. Cardiovasc Res. 1994 Jun;28(6):735–745. doi: 10.1093/cvr/28.6.735. [DOI] [PubMed] [Google Scholar]
- Fujiwara N., Higashi H., Shimoji K., Yoshimura M. Effects of hypoxia on rat hippocampal neurones in vitro. J Physiol. 1987 Mar;384:131–151. doi: 10.1113/jphysiol.1987.sp016447. [DOI] [PMC free article] [PubMed] [Google Scholar]
- Grigg J. J., Anderson E. G. Glucose and sulfonylureas modify different phases of the membrane potential change during hypoxia in rat hippocampal slices. Brain Res. 1989 Jun 12;489(2):302–310. doi: 10.1016/0006-8993(89)90863-9. [DOI] [PubMed] [Google Scholar]
- Haddad G. G., Jiang C. O2 deprivation in the central nervous system: on mechanisms of neuronal response, differential sensitivity and injury. Prog Neurobiol. 1993 Mar;40(3):277–318. doi: 10.1016/0301-0082(93)90014-j. [DOI] [PubMed] [Google Scholar]
- Hansen A. J. Effect of anoxia on ion distribution in the brain. Physiol Rev. 1985 Jan;65(1):101–148. doi: 10.1152/physrev.1985.65.1.101. [DOI] [PubMed] [Google Scholar]
- Hansen A. J., Hounsgaard J., Jahnsen H. Anoxia increases potassium conductance in hippocampal nerve cells. Acta Physiol Scand. 1982 Jul;115(3):301–310. doi: 10.1111/j.1748-1716.1982.tb07082.x. [DOI] [PubMed] [Google Scholar]
- Hochachka P. W. Defense strategies against hypoxia and hypothermia. Science. 1986 Jan 17;231(4735):234–241. doi: 10.1126/science.2417316. [DOI] [PubMed] [Google Scholar]
- Jiang C., Haddad G. G. Oxygen deprivation inhibits a K+ channel independently of cytosolic factors in rat central neurons. J Physiol. 1994 Nov 15;481(Pt 1):15–26. doi: 10.1113/jphysiol.1994.sp020415. [DOI] [PMC free article] [PubMed] [Google Scholar]
- Jiang C., Sigworth F. J., Haddad G. G. Oxygen deprivation activates an ATP-inhibitable K+ channel in substantia nigra neurons. J Neurosci. 1994 Sep;14(9):5590–5602. doi: 10.1523/JNEUROSCI.14-09-05590.1994. [DOI] [PMC free article] [PubMed] [Google Scholar]
- Jiang C., Xia Y., Haddad G. G. Role of ATP-sensitive K+ channels during anoxia: major differences between rat (newborn and adult) and turtle neurons. J Physiol. 1992 Mar;448:599–612. doi: 10.1113/jphysiol.1992.sp019060. [DOI] [PMC free article] [PubMed] [Google Scholar]
- Krnjević K., Xu Y. Mechanisms underlying anoxic hyperpolarization of hippocampal neurons. Can J Physiol Pharmacol. 1990 Dec;68(12):1609–1613. doi: 10.1139/y90-245. [DOI] [PubMed] [Google Scholar]
- Leblond J., Krnjevic K. Hypoxic changes in hippocampal neurons. J Neurophysiol. 1989 Jul;62(1):1–14. doi: 10.1152/jn.1989.62.1.1. [DOI] [PubMed] [Google Scholar]
- Luhmann H. J., Heinemann U. Hypoxia-induced functional alterations in adult rat neocortex. J Neurophysiol. 1992 Apr;67(4):798–811. doi: 10.1152/jn.1992.67.4.798. [DOI] [PubMed] [Google Scholar]
- McKillen H. C., Davies N. W., Stanfield P. R., Standen N. B. The effect of intracellular anions on ATP-dependent potassium channels of rat skeletal muscle. J Physiol. 1994 Sep 15;479(Pt 3):341–351. doi: 10.1113/jphysiol.1994.sp020300. [DOI] [PMC free article] [PubMed] [Google Scholar]
- Mourre C., Ben Ari Y., Bernardi H., Fosset M., Lazdunski M. Antidiabetic sulfonylureas: localization of binding sites in the brain and effects on the hyperpolarization induced by anoxia in hippocampal slices. Brain Res. 1989 May 1;486(1):159–164. doi: 10.1016/0006-8993(89)91288-2. [DOI] [PubMed] [Google Scholar]
- Murphy K. P., Greenfield S. A. Neuronal selectivity of ATP-sensitive potassium channels in guinea-pig substantia nigra revealed by responses to anoxia. J Physiol. 1992;453:167–183. doi: 10.1113/jphysiol.1992.sp019222. [DOI] [PMC free article] [PubMed] [Google Scholar]
- Pusch M., Neher E. Rates of diffusional exchange between small cells and a measuring patch pipette. Pflugers Arch. 1988 Feb;411(2):204–211. doi: 10.1007/BF00582316. [DOI] [PubMed] [Google Scholar]
- Schwanstecher C., Panten U. Identification of an ATP-sensitive K+ channel in spiny neurons of rat caudate nucleus. Pflugers Arch. 1994 May;427(1-2):187–189. doi: 10.1007/BF00585961. [DOI] [PubMed] [Google Scholar]
- Terzic A., Tung R. T., Kurachi Y. Nucleotide regulation of ATP sensitive potassium channels. Cardiovasc Res. 1994 Jun;28(6):746–753. doi: 10.1093/cvr/28.6.746. [DOI] [PubMed] [Google Scholar]
- Trapp S., Ballanyi K., Richter D. W. Spontaneous activation of KATP current in rat dorsal vagal neurones. Neuroreport. 1994 Jun 2;5(10):1285–1288. doi: 10.1097/00001756-199406020-00033. [DOI] [PubMed] [Google Scholar]
- Yarom Y., Sugimori M., Llinás R. Ionic currents and firing patterns of mammalian vagal motoneurons in vitro. Neuroscience. 1985 Dec;16(4):719–737. doi: 10.1016/0306-4522(85)90090-9. [DOI] [PubMed] [Google Scholar]
- Zhang L., Krnjević K. Whole-cell recording of anoxic effects on hippocampal neurons in slices. J Neurophysiol. 1993 Jan;69(1):118–127. doi: 10.1152/jn.1993.69.1.118. [DOI] [PubMed] [Google Scholar]