Abstract
1. The rat brain type IIA Na+ channel alpha-subunit was stably expressed in Chinese hamster ovary (CHO) cells. Current through the expressed Na+ channels was studied using the whole-cell configuration of the patch clamp technique. The transient Na+ current was sensitive to TTX and showed a bell-shaped peak current vs. membrane potential relation. 2. Na+ current inactivation was better described by the sum of two exponentials in the potential range -30 to + 40 mV, with a dominating fast component and a small slower component. 3. The steady-state inactivation, h infinity, was related to potential by a Boltzmann distribution, underlying three states of the inactivation gate. 4. Recovery of the channels from inactivation at different potentials in the range -70 to -120 mV were characterized by an initial delay which decreased with hyperpolarization. The time course was well fitted by the sum of two exponentials. In this case the slower exponential was the major component, and both time constants decreased with hyperpolarization. 5. For a working description of the Na+ channel inactivation in this preparation, with a minimal deviation from the Hodgkin-Huxley model, a three-state scheme of the form O<-->I1<-->I2 was proposed, replacing the original two-state scheme of the Hodgkin-Huxley model, and the rate constants are reported. 6. The instantaneous current-voltage relationship showed marked deviation from linearity and was satisfactorily fitted by the constant-field equation. 7. The time course of activation was described by an m chi model. However, the best-fitted value of chi varied with the membrane potential and had a mean value of 2. 8. Effective gating charge was determined to be 4.7e from the slope of the activation plot, plotted on a logarithmic scale. 9. The rate constants of activation, alpha m and beta m, were determined. Their functional dependence on the membrane potential was investigated.
Full text
PDF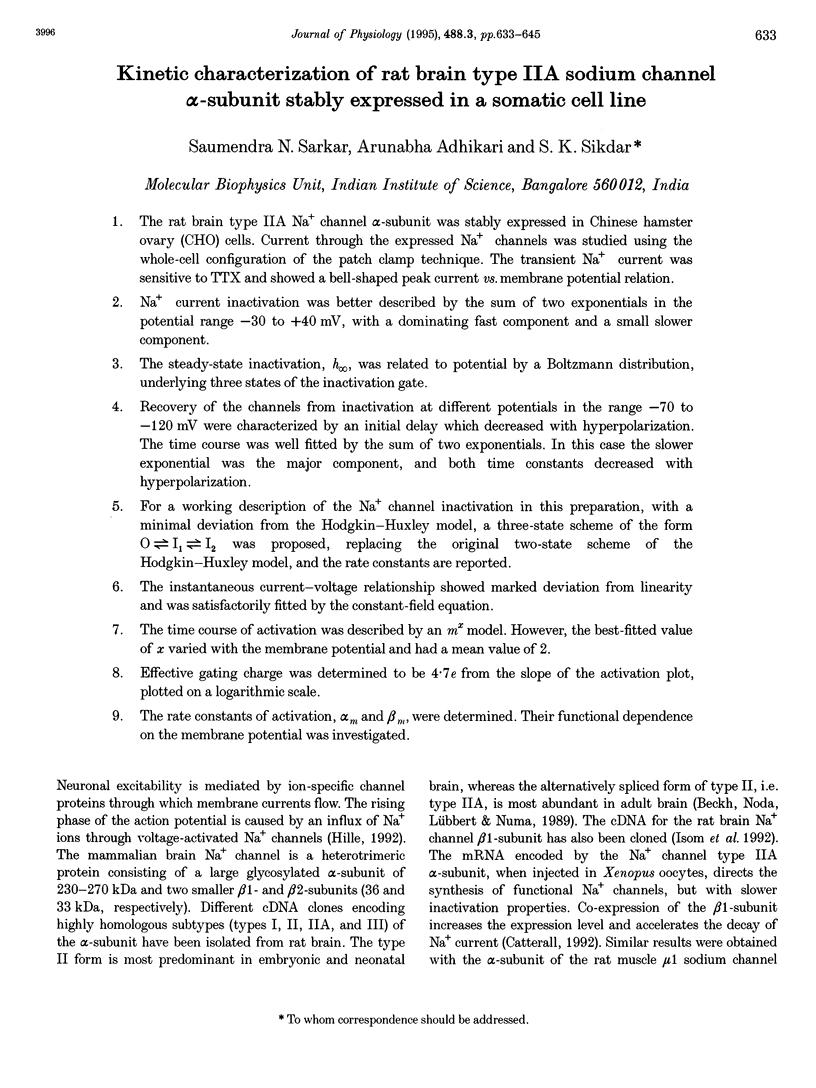
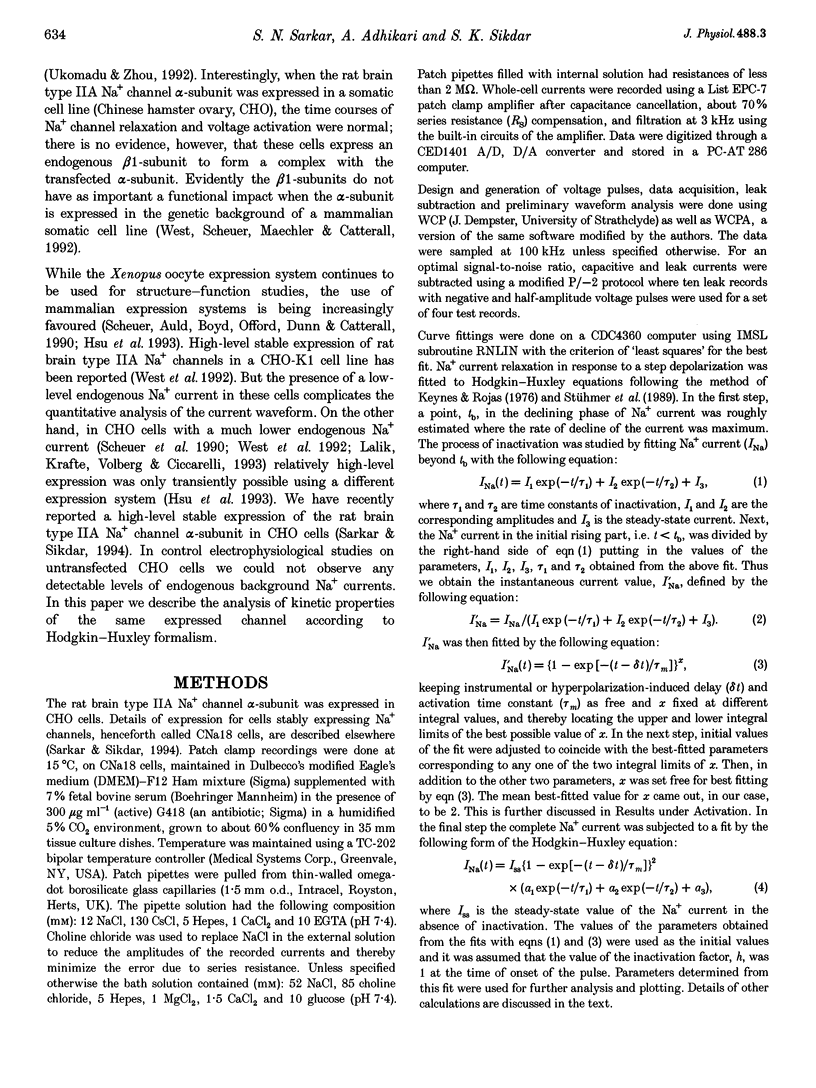
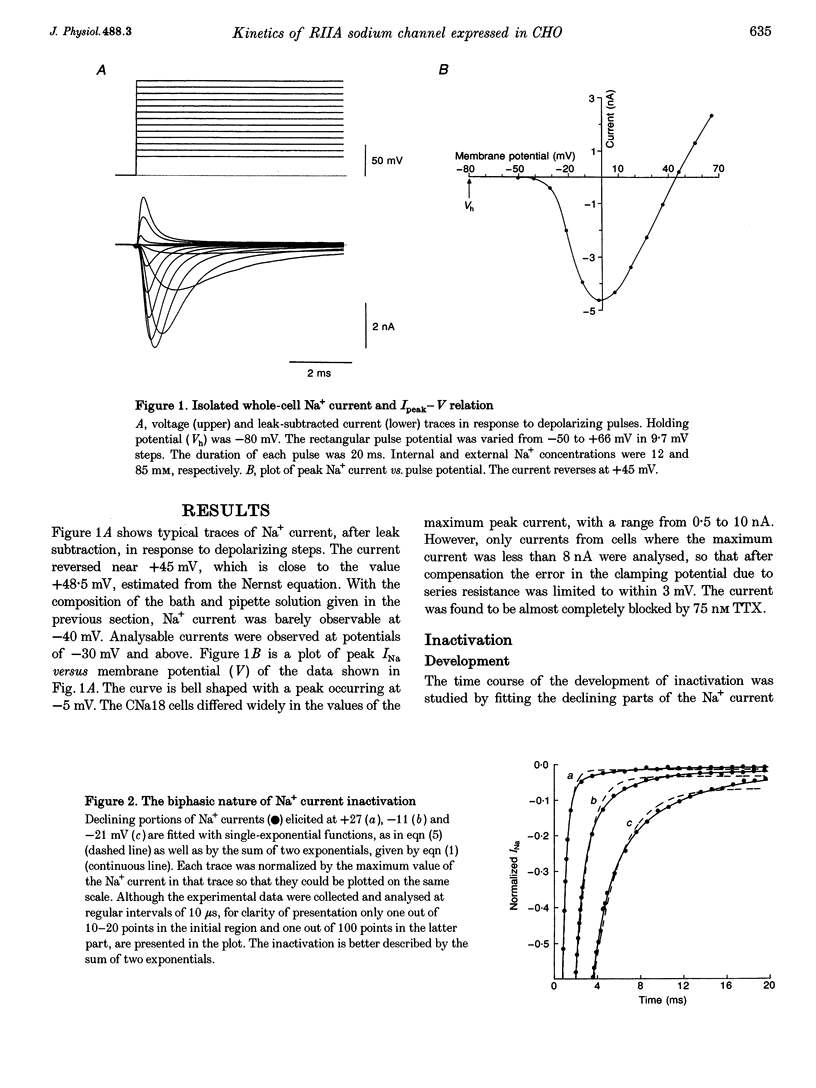
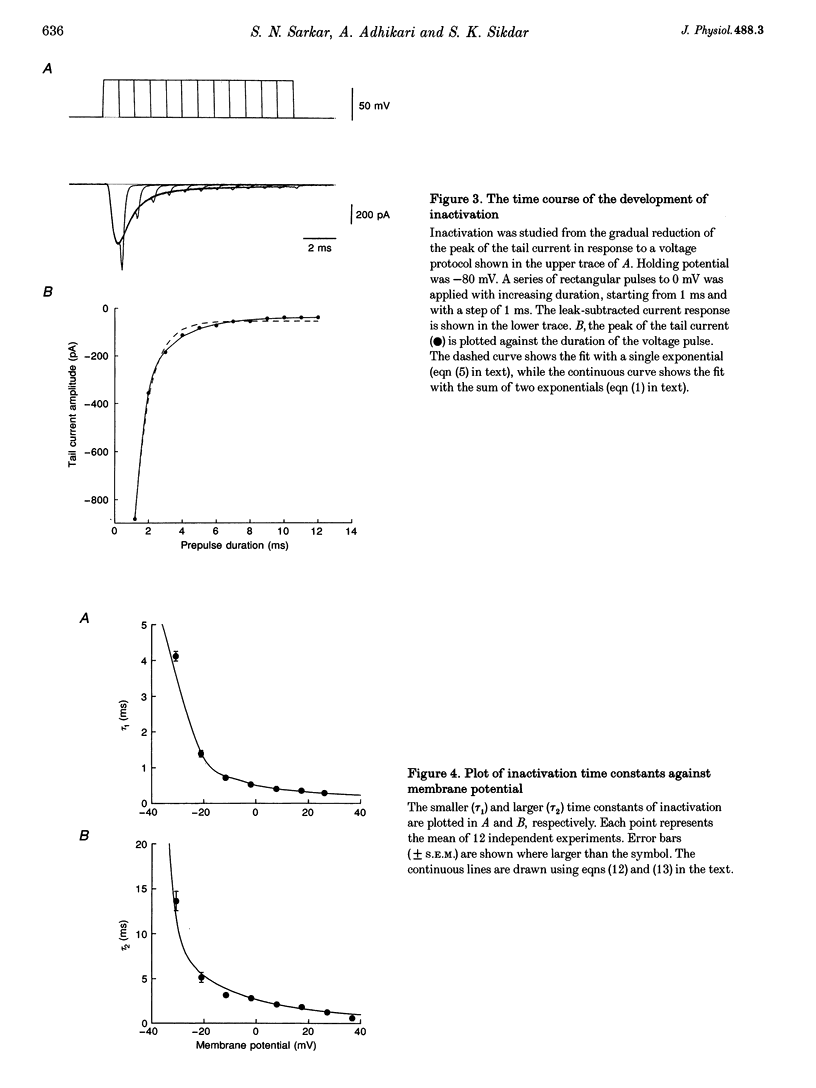
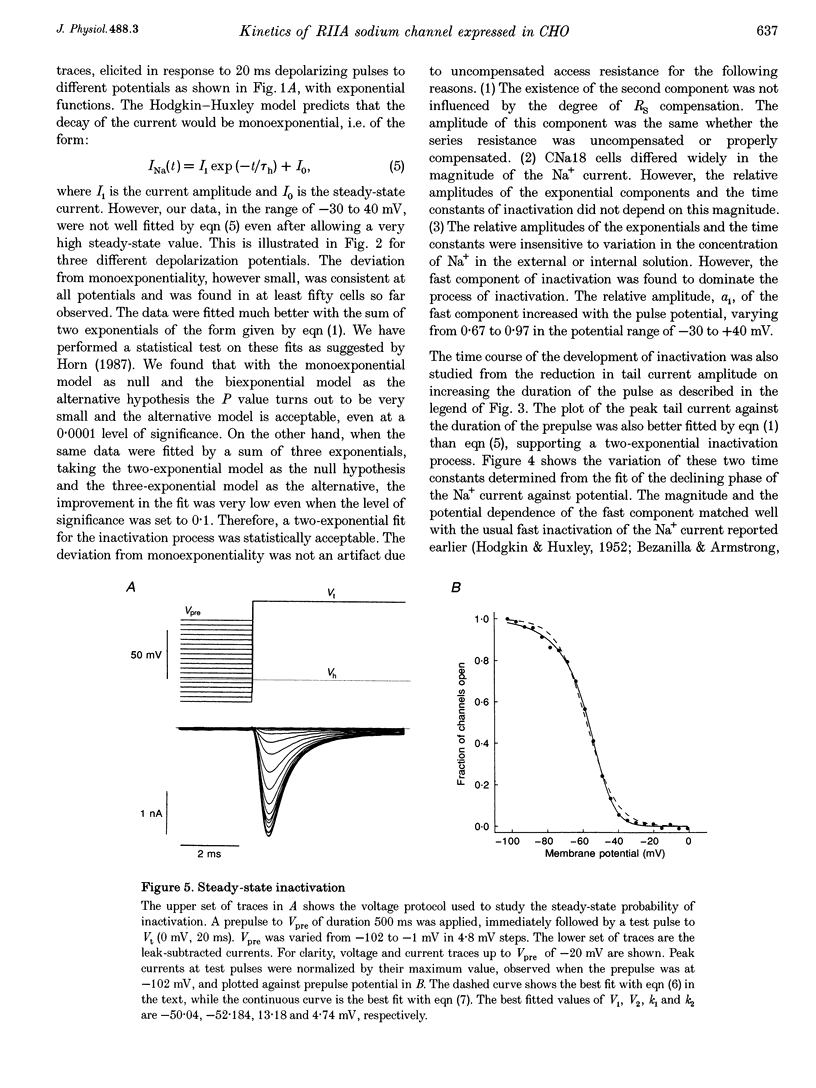
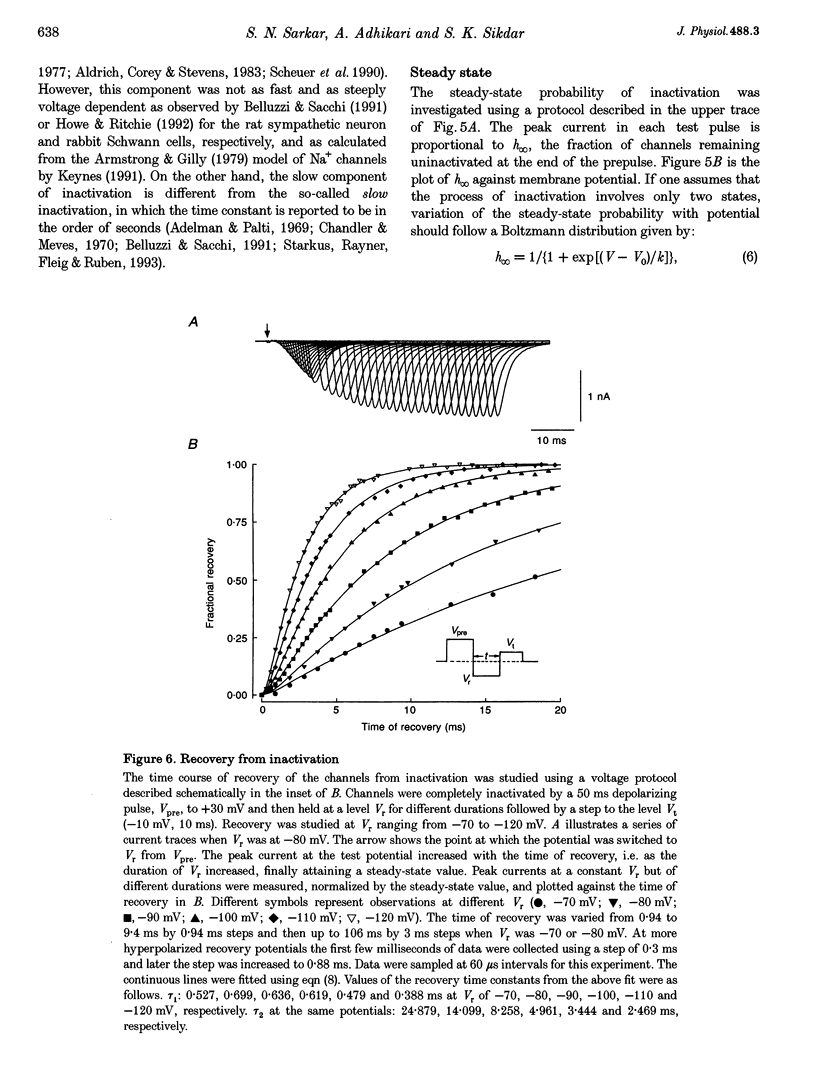
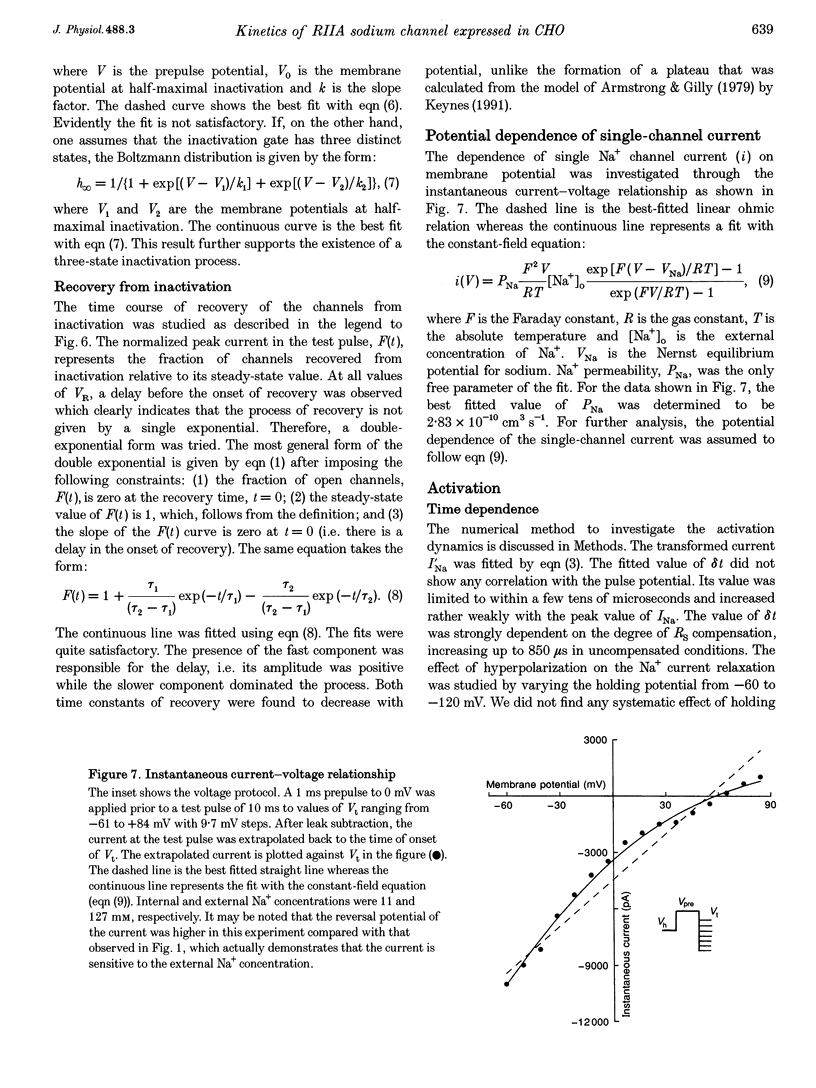
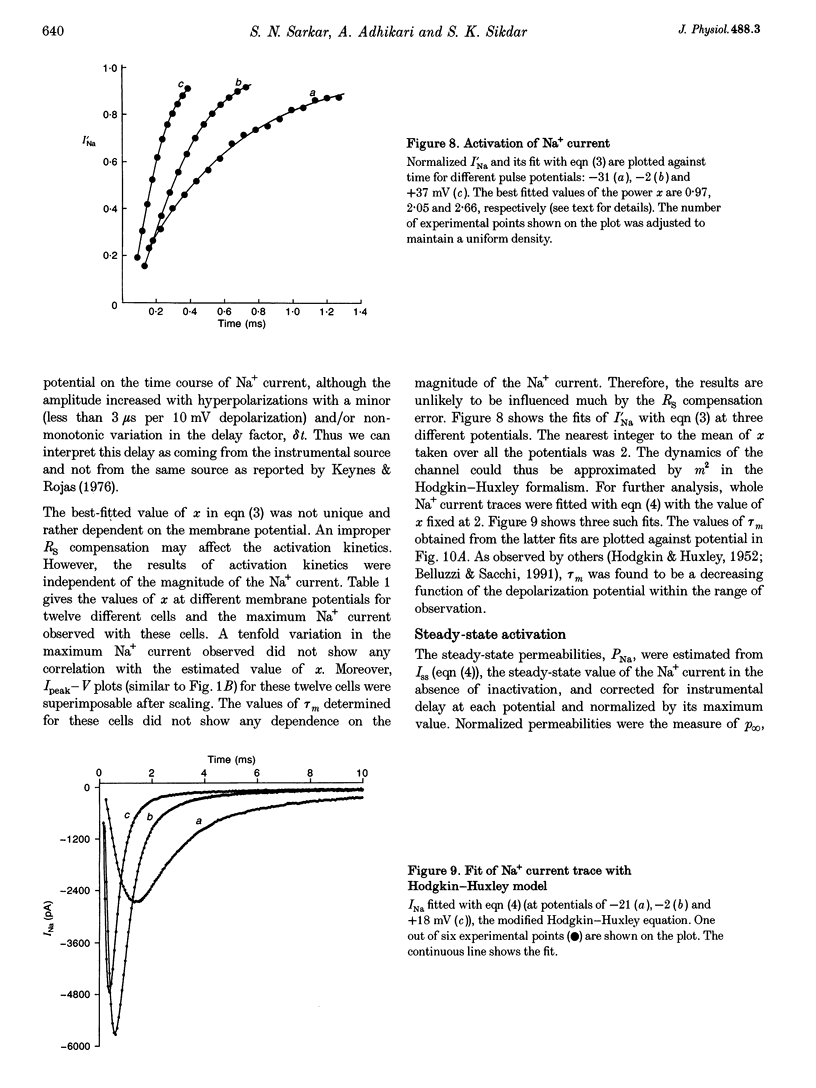
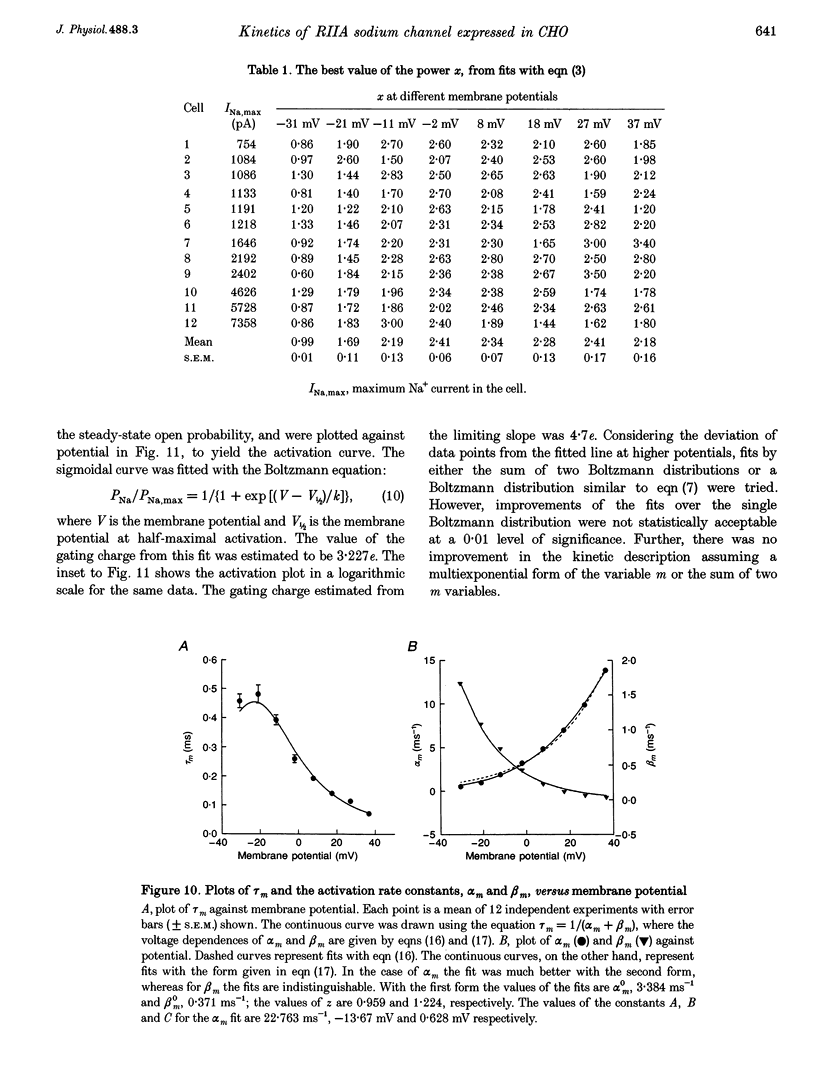
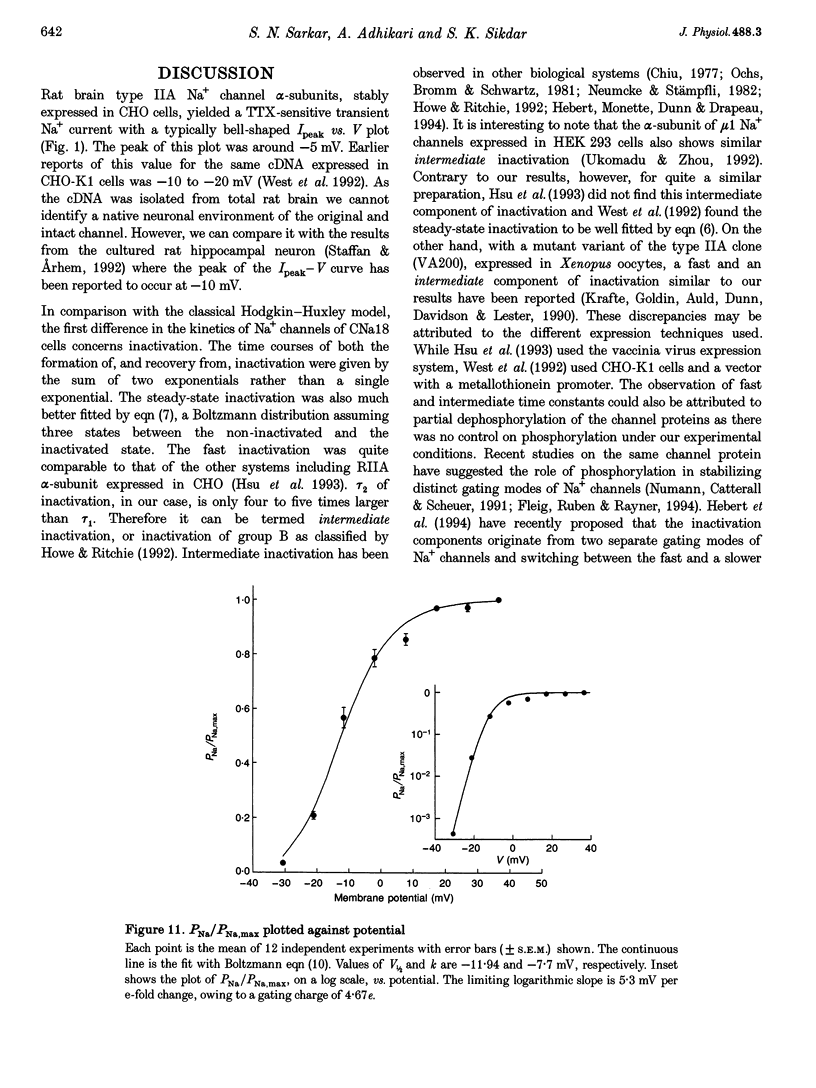
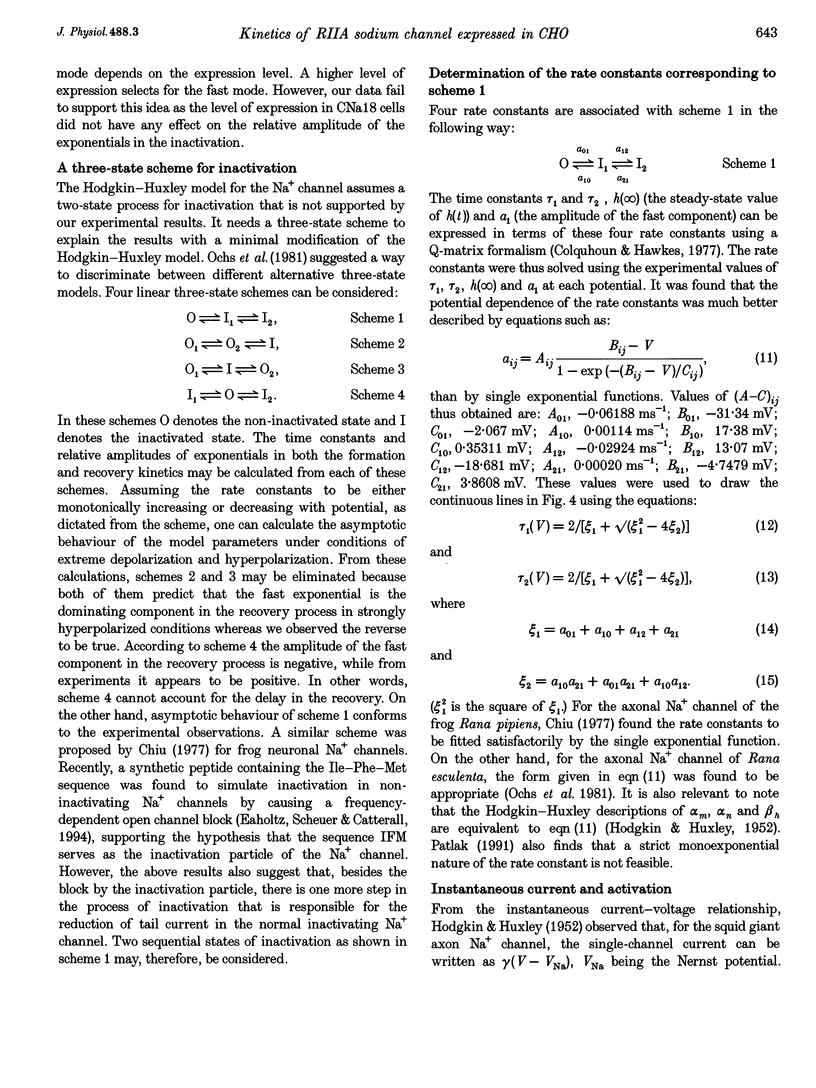
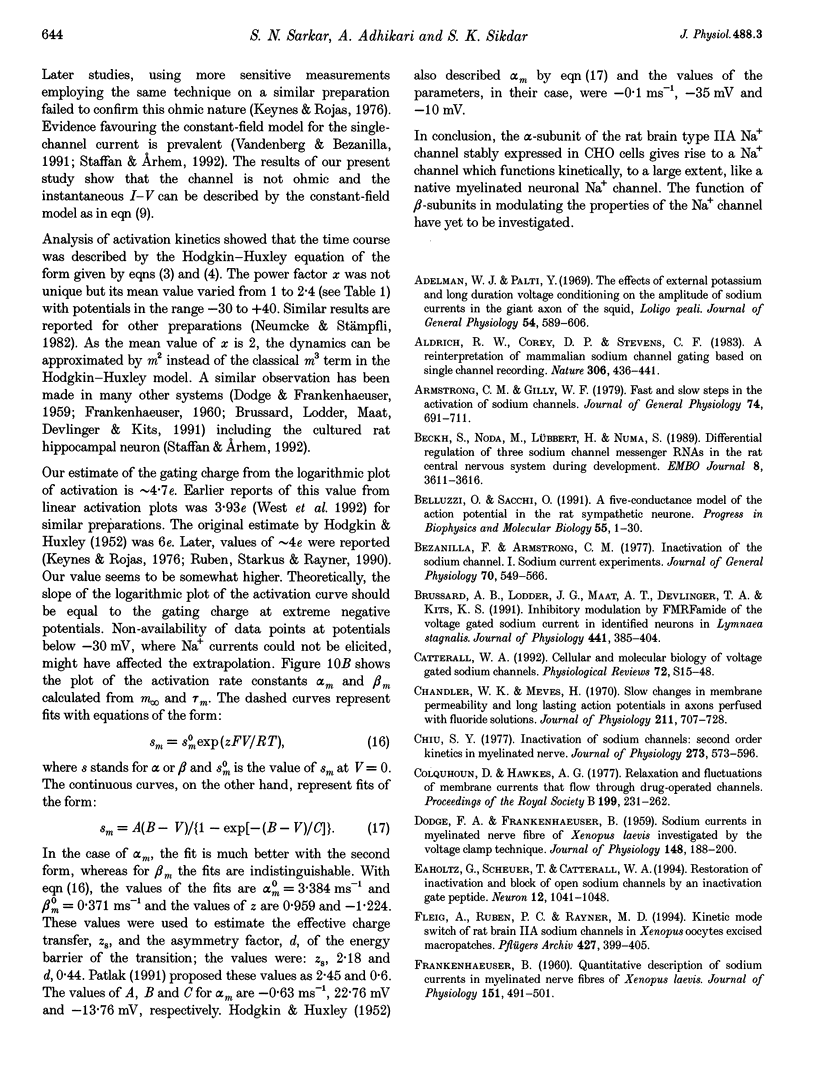
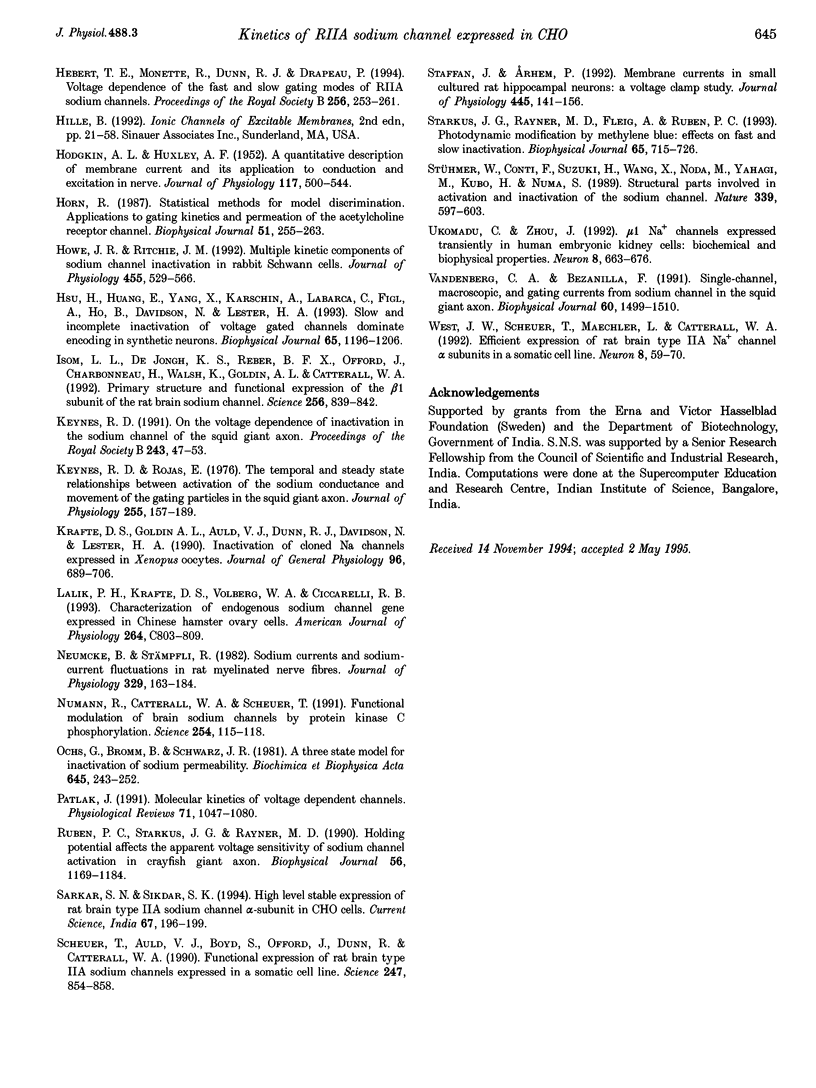
Selected References
These references are in PubMed. This may not be the complete list of references from this article.
- Adelman W. J., Jr, Palti Y. The effects of external potassium and long duration voltage conditioning on the amplitude of sodium currents in the giant axon of the squid, Loligo pealei. J Gen Physiol. 1969 Nov;54(5):589–606. doi: 10.1085/jgp.54.5.589. [DOI] [PMC free article] [PubMed] [Google Scholar]
- Aldrich R. W., Corey D. P., Stevens C. F. A reinterpretation of mammalian sodium channel gating based on single channel recording. Nature. 1983 Dec 1;306(5942):436–441. doi: 10.1038/306436a0. [DOI] [PubMed] [Google Scholar]
- Armstrong C. M., Gilly W. F. Fast and slow steps in the activation of sodium channels. J Gen Physiol. 1979 Dec;74(6):691–711. doi: 10.1085/jgp.74.6.691. [DOI] [PMC free article] [PubMed] [Google Scholar]
- Beckh S., Noda M., Lübbert H., Numa S. Differential regulation of three sodium channel messenger RNAs in the rat central nervous system during development. EMBO J. 1989 Dec 1;8(12):3611–3616. doi: 10.1002/j.1460-2075.1989.tb08534.x. [DOI] [PMC free article] [PubMed] [Google Scholar]
- Belluzzi O., Sacchi O. A five-conductance model of the action potential in the rat sympathetic neurone. Prog Biophys Mol Biol. 1991;55(1):1–30. doi: 10.1016/0079-6107(91)90009-h. [DOI] [PubMed] [Google Scholar]
- Bezanilla F., Armstrong C. M. Inactivation of the sodium channel. I. Sodium current experiments. J Gen Physiol. 1977 Nov;70(5):549–566. doi: 10.1085/jgp.70.5.549. [DOI] [PMC free article] [PubMed] [Google Scholar]
- Brussaard A. B., Lodder J. C., ter Maat A., de Vlieger T. A., Kits K. S. Inhibitory modulation by FMRFamide of the voltage-gated sodium current in identified neurones in Lymnaea stagnalis. J Physiol. 1991 Sep;441:385–404. doi: 10.1113/jphysiol.1991.sp018757. [DOI] [PMC free article] [PubMed] [Google Scholar]
- Chandler W. K., Meves H. Slow changes in membrane permeability and long-lasting action potentials in axons perfused with fluoride solutions. J Physiol. 1970 Dec;211(3):707–728. doi: 10.1113/jphysiol.1970.sp009300. [DOI] [PMC free article] [PubMed] [Google Scholar]
- Chiu S. Y. Inactivation of sodium channels: second order kinetics in myelinated nerve. J Physiol. 1977 Dec;273(3):573–596. doi: 10.1113/jphysiol.1977.sp012111. [DOI] [PMC free article] [PubMed] [Google Scholar]
- Colquhoun D., Hawkes A. G. Relaxation and fluctuations of membrane currents that flow through drug-operated channels. Proc R Soc Lond B Biol Sci. 1977 Nov 14;199(1135):231–262. doi: 10.1098/rspb.1977.0137. [DOI] [PubMed] [Google Scholar]
- DODGE F. A., FRANKENHAEUSER B. Sodium currents in the myelinated nerve fibre of Xenopus laevis investigated with the voltage clamp technique. J Physiol. 1959 Oct;148:188–200. doi: 10.1113/jphysiol.1959.sp006281. [DOI] [PMC free article] [PubMed] [Google Scholar]
- Eaholtz G., Scheuer T., Catterall W. A. Restoration of inactivation and block of open sodium channels by an inactivation gate peptide. Neuron. 1994 May;12(5):1041–1048. doi: 10.1016/0896-6273(94)90312-3. [DOI] [PubMed] [Google Scholar]
- FRANKENHAEUSER B. Quantitative description of sodium currents in myelinated nerve fibres of Xenopus laevis. J Physiol. 1960 Jun;151:491–501. doi: 10.1113/jphysiol.1960.sp006455. [DOI] [PMC free article] [PubMed] [Google Scholar]
- Fleig A., Ruben P. C., Rayner M. D. Kinetic mode switch of rat brain IIA Na channels in Xenopus oocytes excised macropatches. Pflugers Arch. 1994 Jul;427(5-6):399–405. doi: 10.1007/BF00374253. [DOI] [PubMed] [Google Scholar]
- HODGKIN A. L., HUXLEY A. F. A quantitative description of membrane current and its application to conduction and excitation in nerve. J Physiol. 1952 Aug;117(4):500–544. doi: 10.1113/jphysiol.1952.sp004764. [DOI] [PMC free article] [PubMed] [Google Scholar]
- Hebert T. E., Monette R., Dunn R. J., Drapeau P. Voltage dependencies of the fast and slow gating modes of RIIA sodium channels. Proc Biol Sci. 1994 Jun 22;256(1347):253–261. doi: 10.1098/rspb.1994.0078. [DOI] [PubMed] [Google Scholar]
- Horn R. Statistical methods for model discrimination. Applications to gating kinetics and permeation of the acetylcholine receptor channel. Biophys J. 1987 Feb;51(2):255–263. doi: 10.1016/S0006-3495(87)83331-3. [DOI] [PMC free article] [PubMed] [Google Scholar]
- Howe J. R., Ritchie J. M. Multiple kinetic components of sodium channel inactivation in rabbit Schwann cells. J Physiol. 1992 Sep;455:529–566. doi: 10.1113/jphysiol.1992.sp019315. [DOI] [PMC free article] [PubMed] [Google Scholar]
- Hsu H., Huang E., Yang X. C., Karschin A., Labarca C., Figl A., Ho B., Davidson N., Lester H. A. Slow and incomplete inactivations of voltage-gated channels dominate encoding in synthetic neurons. Biophys J. 1993 Sep;65(3):1196–1206. doi: 10.1016/S0006-3495(93)81153-6. [DOI] [PMC free article] [PubMed] [Google Scholar]
- Isom L. L., De Jongh K. S., Patton D. E., Reber B. F., Offord J., Charbonneau H., Walsh K., Goldin A. L., Catterall W. A. Primary structure and functional expression of the beta 1 subunit of the rat brain sodium channel. Science. 1992 May 8;256(5058):839–842. doi: 10.1126/science.1375395. [DOI] [PubMed] [Google Scholar]
- Johansson S., Arhem P. Membrane currents in small cultured rat hippocampal neurons: a voltage-clamp study. J Physiol. 1992 Jan;445:141–156. doi: 10.1113/jphysiol.1992.sp018916. [DOI] [PMC free article] [PubMed] [Google Scholar]
- Keynes R. D. On the voltage dependence of inactivation in the sodium channel of the squid giant axon. Proc Biol Sci. 1991 Jan 22;243(1306):47–53. doi: 10.1098/rspb.1991.0008. [DOI] [PubMed] [Google Scholar]
- Keynes R. D., Rojas E. The temporal and steady-state relationships between activation of the sodium conductance and movement of the gating particles in the squid giant axon. J Physiol. 1976 Feb;255(1):157–189. doi: 10.1113/jphysiol.1976.sp011274. [DOI] [PMC free article] [PubMed] [Google Scholar]
- Krafte D. S., Goldin A. L., Auld V. J., Dunn R. J., Davidson N., Lester H. A. Inactivation of cloned Na channels expressed in Xenopus oocytes. J Gen Physiol. 1990 Oct;96(4):689–706. doi: 10.1085/jgp.96.4.689. [DOI] [PMC free article] [PubMed] [Google Scholar]
- Lalik P. H., Krafte D. S., Volberg W. A., Ciccarelli R. B. Characterization of endogenous sodium channel gene expressed in Chinese hamster ovary cells. Am J Physiol. 1993 Apr;264(4 Pt 1):C803–C809. doi: 10.1152/ajpcell.1993.264.4.C803. [DOI] [PubMed] [Google Scholar]
- Neumcke B., Stämpfli R. Sodium currents and sodium-current fluctuations in rat myelinated nerve fibres. J Physiol. 1982 Aug;329:163–184. doi: 10.1113/jphysiol.1982.sp014296. [DOI] [PMC free article] [PubMed] [Google Scholar]
- Numann R., Catterall W. A., Scheuer T. Functional modulation of brain sodium channels by protein kinase C phosphorylation. Science. 1991 Oct 4;254(5028):115–118. doi: 10.1126/science.1656525. [DOI] [PubMed] [Google Scholar]
- Ochs G., Bromm B., Schwarz J. R. A three-state model for inactivation of sodium permeability. Biochim Biophys Acta. 1981 Jul 20;645(2):243–252. doi: 10.1016/0005-2736(81)90195-4. [DOI] [PubMed] [Google Scholar]
- Patlak J. Molecular kinetics of voltage-dependent Na+ channels. Physiol Rev. 1991 Oct;71(4):1047–1080. doi: 10.1152/physrev.1991.71.4.1047. [DOI] [PubMed] [Google Scholar]
- Ruben P. C., Starkus J. G., Rayner M. D. Holding potential affects the apparent voltage-sensitivity of sodium channel activation in crayfish giant axons. Biophys J. 1990 Nov;58(5):1169–1181. doi: 10.1016/S0006-3495(90)82458-9. [DOI] [PMC free article] [PubMed] [Google Scholar]
- Scheuer T., Auld V. J., Boyd S., Offord J., Dunn R., Catterall W. A. Functional properties of rat brain sodium channels expressed in a somatic cell line. Science. 1990 Feb 16;247(4944):854–858. doi: 10.1126/science.2154850. [DOI] [PubMed] [Google Scholar]
- Starkus J. G., Rayner M. D., Fleig A., Ruben P. C. Fast and slow inactivation of sodium channels: effects of photodynamic modification by methylene blue. Biophys J. 1993 Aug;65(2):715–726. doi: 10.1016/S0006-3495(93)81098-1. [DOI] [PMC free article] [PubMed] [Google Scholar]
- Stühmer W., Conti F., Suzuki H., Wang X. D., Noda M., Yahagi N., Kubo H., Numa S. Structural parts involved in activation and inactivation of the sodium channel. Nature. 1989 Jun 22;339(6226):597–603. doi: 10.1038/339597a0. [DOI] [PubMed] [Google Scholar]
- Ukomadu C., Zhou J., Sigworth F. J., Agnew W. S. muI Na+ channels expressed transiently in human embryonic kidney cells: biochemical and biophysical properties. Neuron. 1992 Apr;8(4):663–676. doi: 10.1016/0896-6273(92)90088-u. [DOI] [PubMed] [Google Scholar]
- Vandenberg C. A., Bezanilla F. Single-channel, macroscopic, and gating currents from sodium channels in the squid giant axon. Biophys J. 1991 Dec;60(6):1499–1510. doi: 10.1016/S0006-3495(91)82185-3. [DOI] [PMC free article] [PubMed] [Google Scholar]
- West J. W., Scheuer T., Maechler L., Catterall W. A. Efficient expression of rat brain type IIA Na+ channel alpha subunits in a somatic cell line. Neuron. 1992 Jan;8(1):59–70. doi: 10.1016/0896-6273(92)90108-p. [DOI] [PubMed] [Google Scholar]