Abstract
1. Using the whole-cell patch clamp technique, the voltage-gated currents of neurons acutely isolated from the Xenopus embryo spinal cord were studied. 2. The spinal neurons possessed a very fast Na+ current, which activated with time constants that ranged from 0.1 to 0.25 ms. It was also subject to rapid inactivation with time constants ranging from 0.3 to 8 ms. This current could only be fitted with Hodgkin-Huxley equations once the rapid inactivation that occurs by the time of the peak current had been taken into account. 3. Xenopus embryo neurons also possessed a mixture of kinetically similar Ca2+ currents, which activated with time constants that ranged from 0.3 to 0.8 ms. Sometimes the Ca2+ currents showed very slow inactivation at more positive voltages (> 20 mV). The Ca2+ current was modelled as a single non-inactivating current. 4. As might be expected, the embryonic neurons possessed a mixture of outward currents that were hard to separate either pharmacologically or through differences in voltage dependence. The delayed rectifier seemed to consist of varying proportions of two currents: a fast-activating K+ current (with time constants of activation ranging from 0.6 to 2 ms) and a slow K+ current (with time constants of activation ranging from 5 to 25 ms). The slow current was occasionally seen in isolation. 5. For the Ca2+, fast K+ and slow K+ currents the rate of deactivation was faster than would be predicted from the kinetics of activation. This was modelled by allowing the closing rate constant of the channels to be described by one of two different functions of voltage that between them covered the whole range of transmembrane voltage. Although this was done for empirical reasons, it could be interpreted to suggest that the channels have more than one open state and predominantly close from a state that is distinct from the one to which they originally opened.
Full text
PDF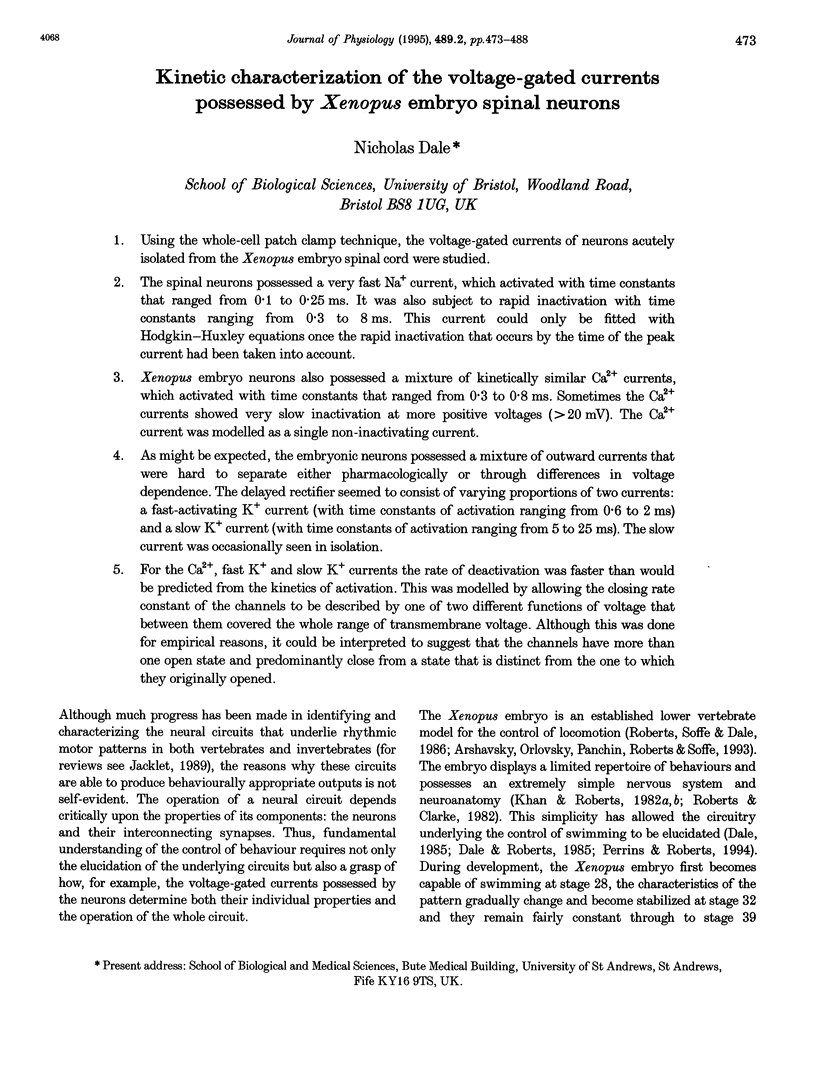
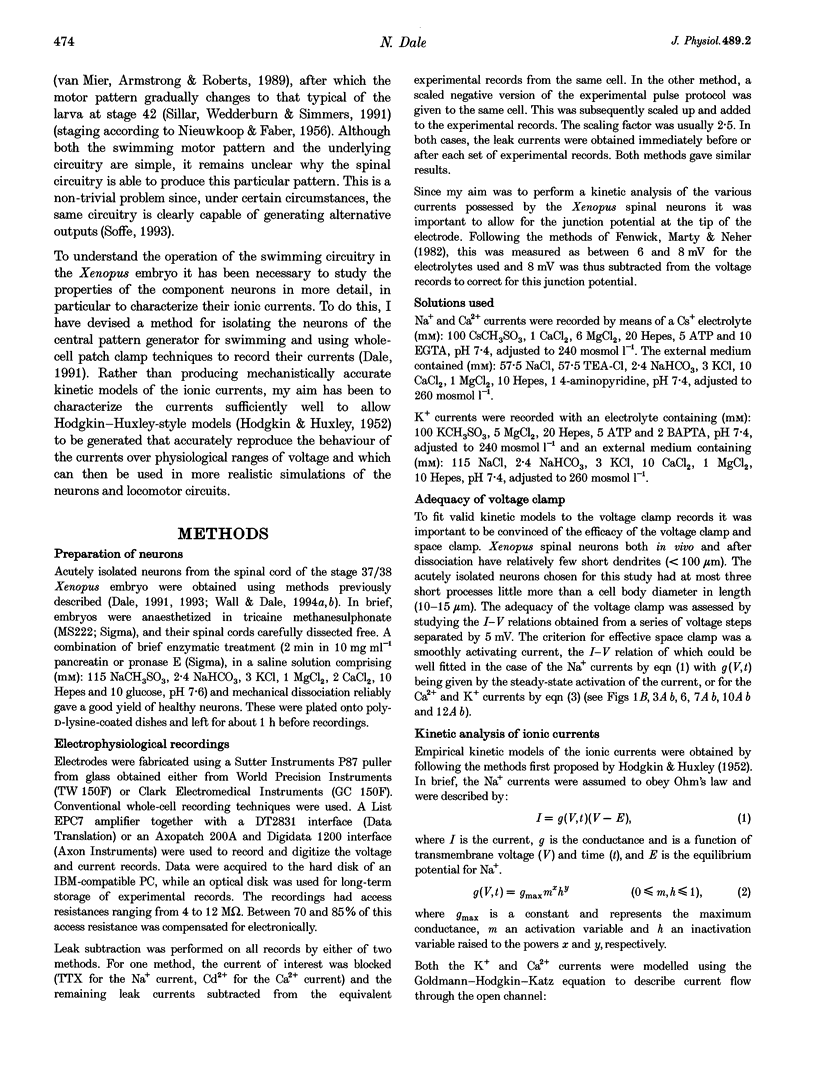
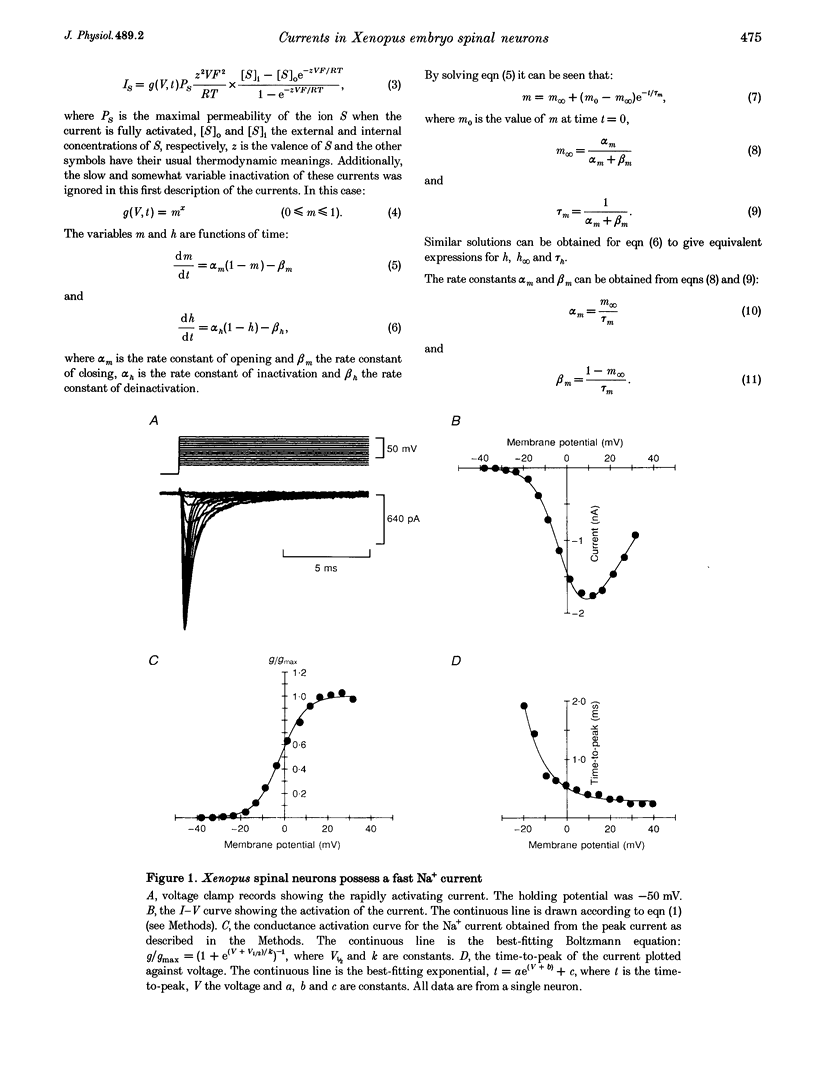
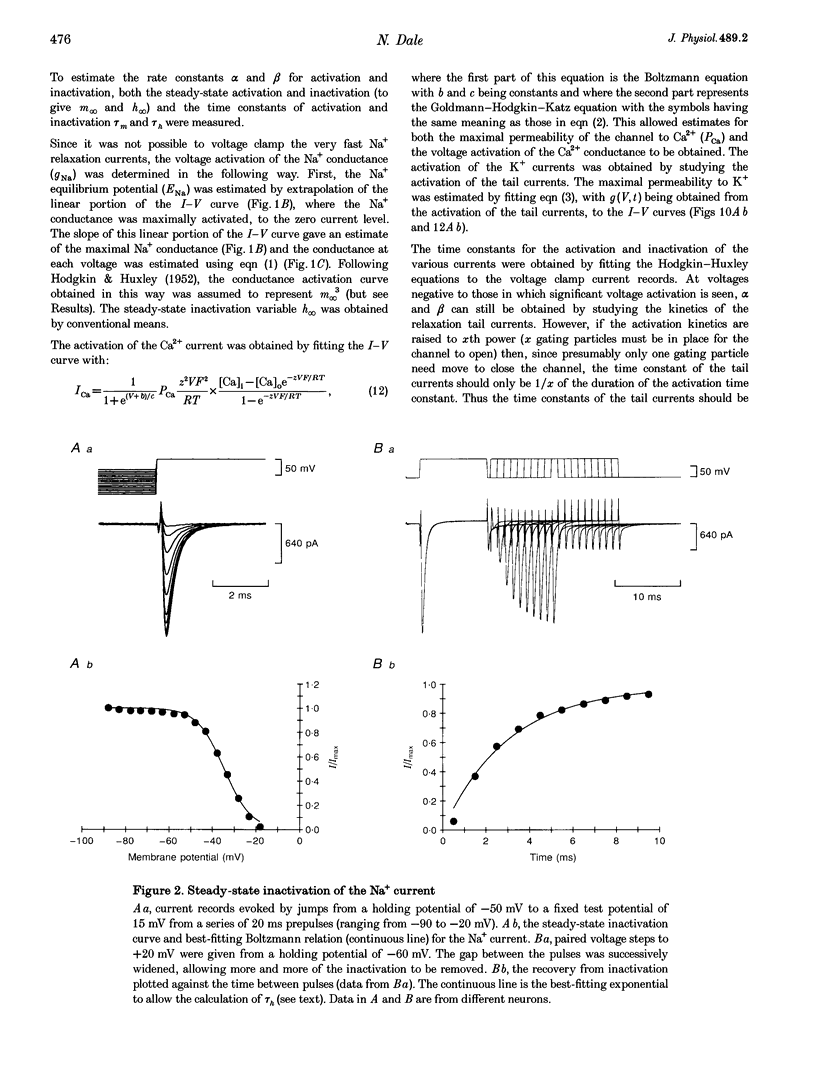
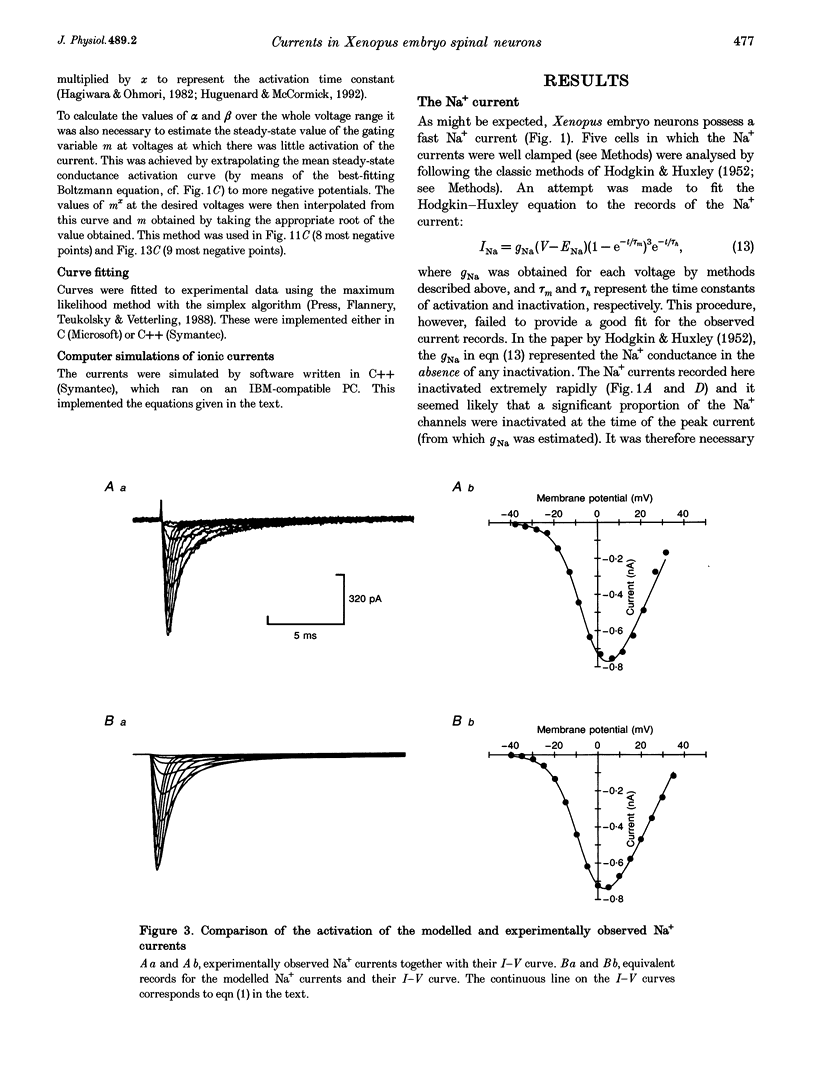
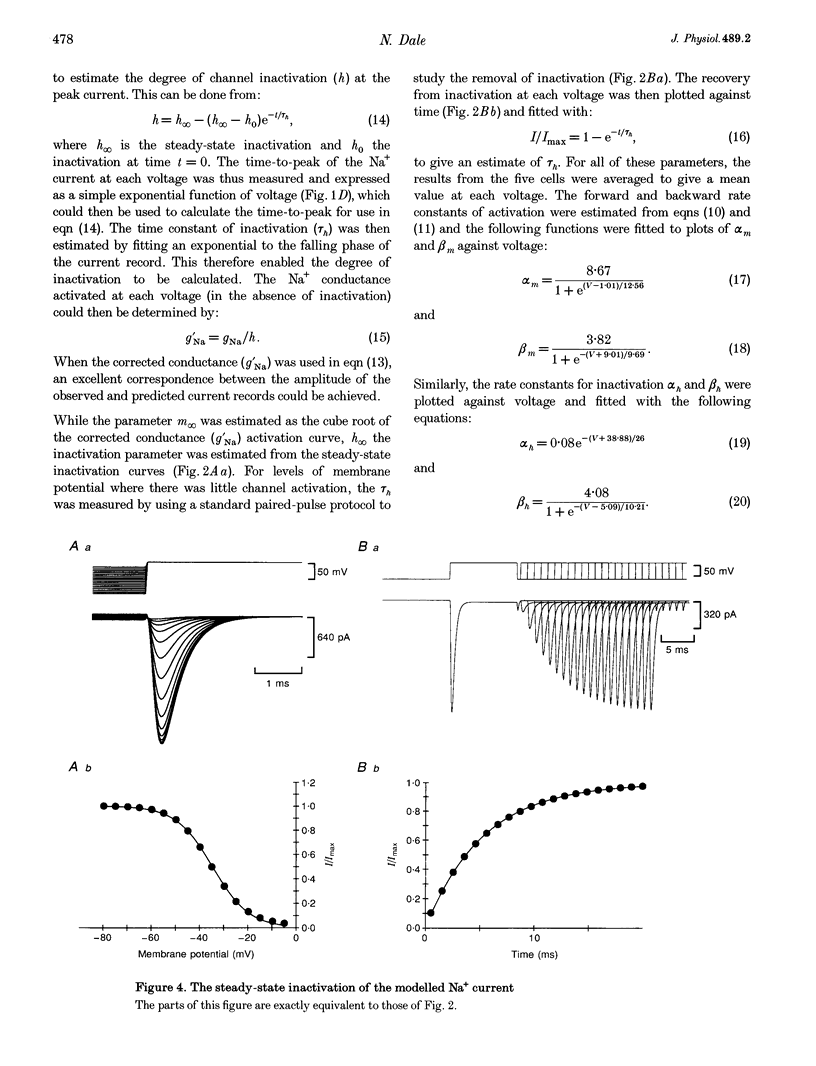
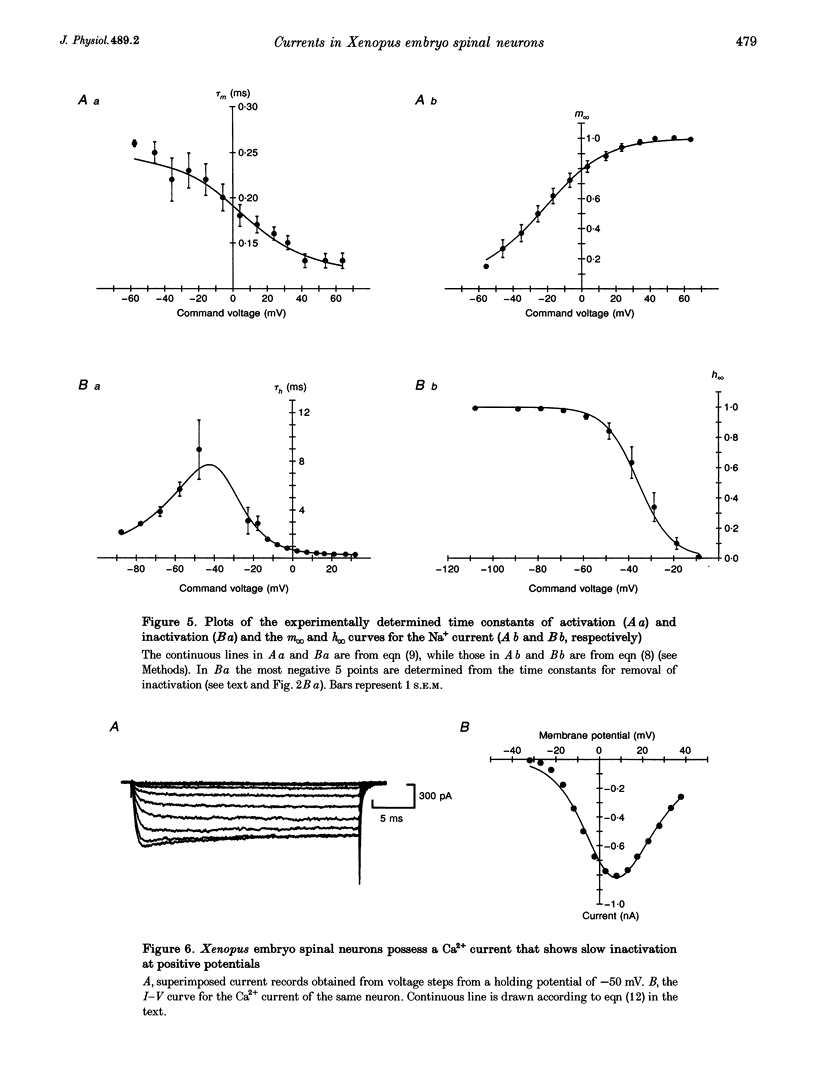
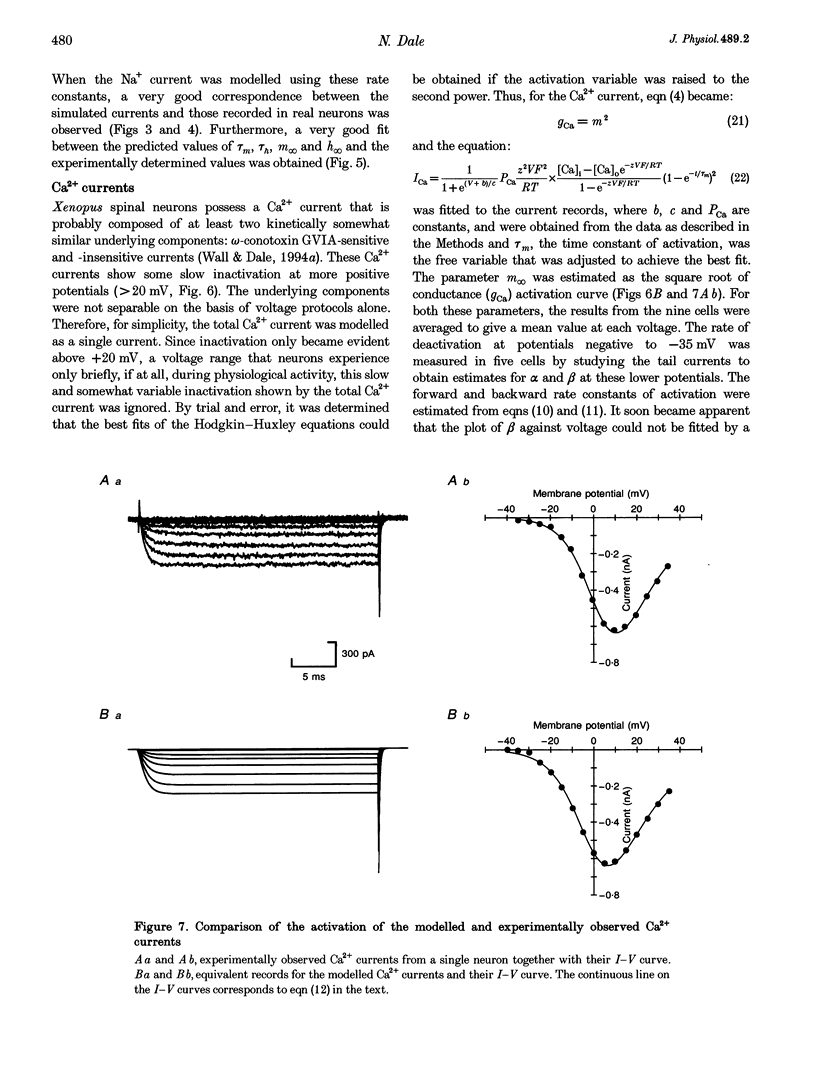
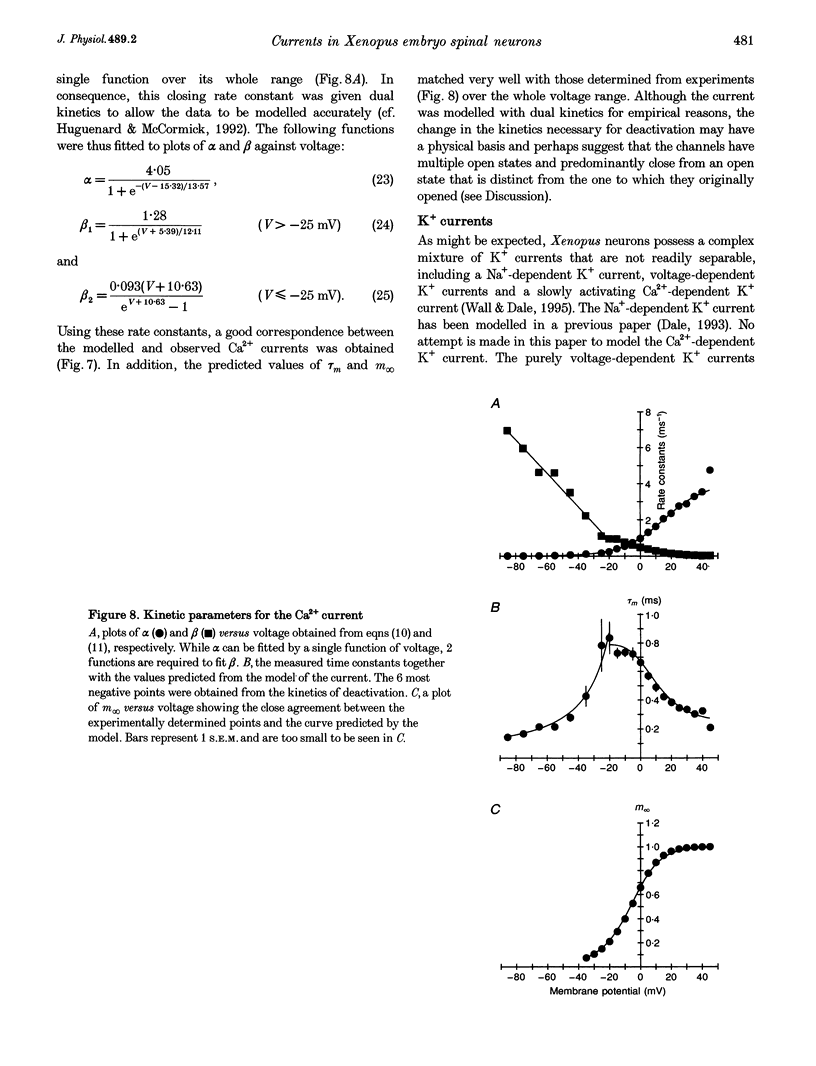
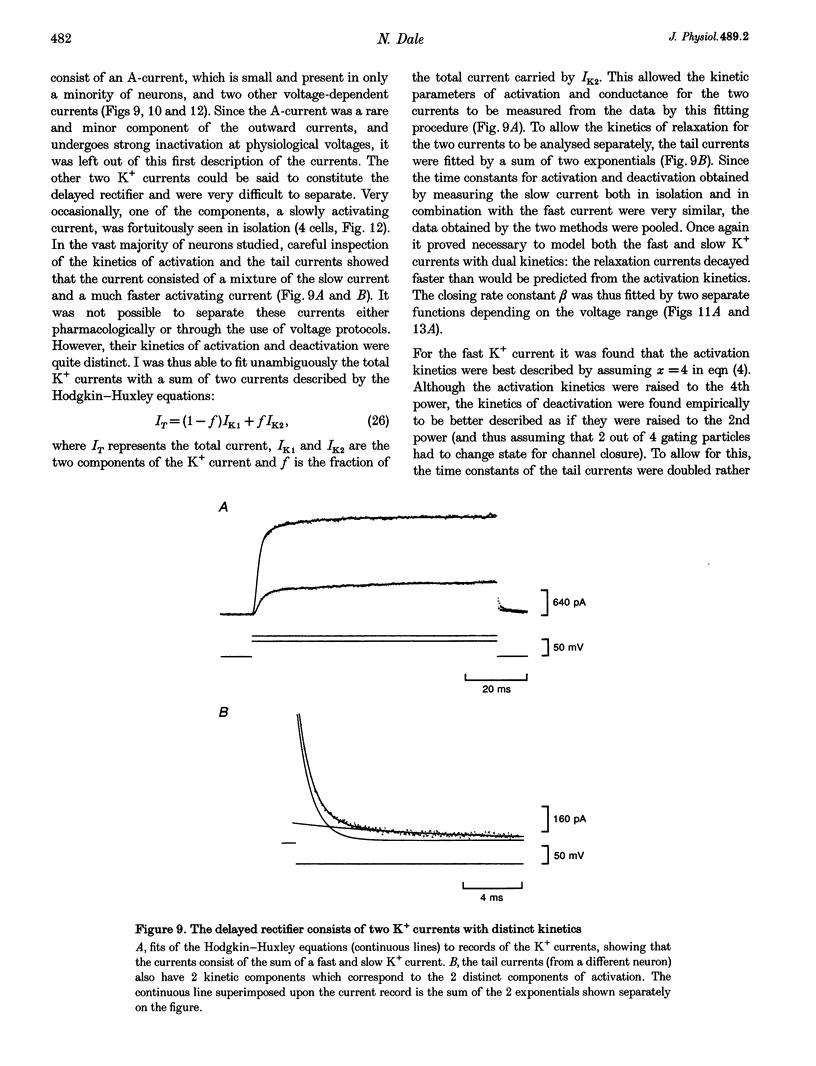
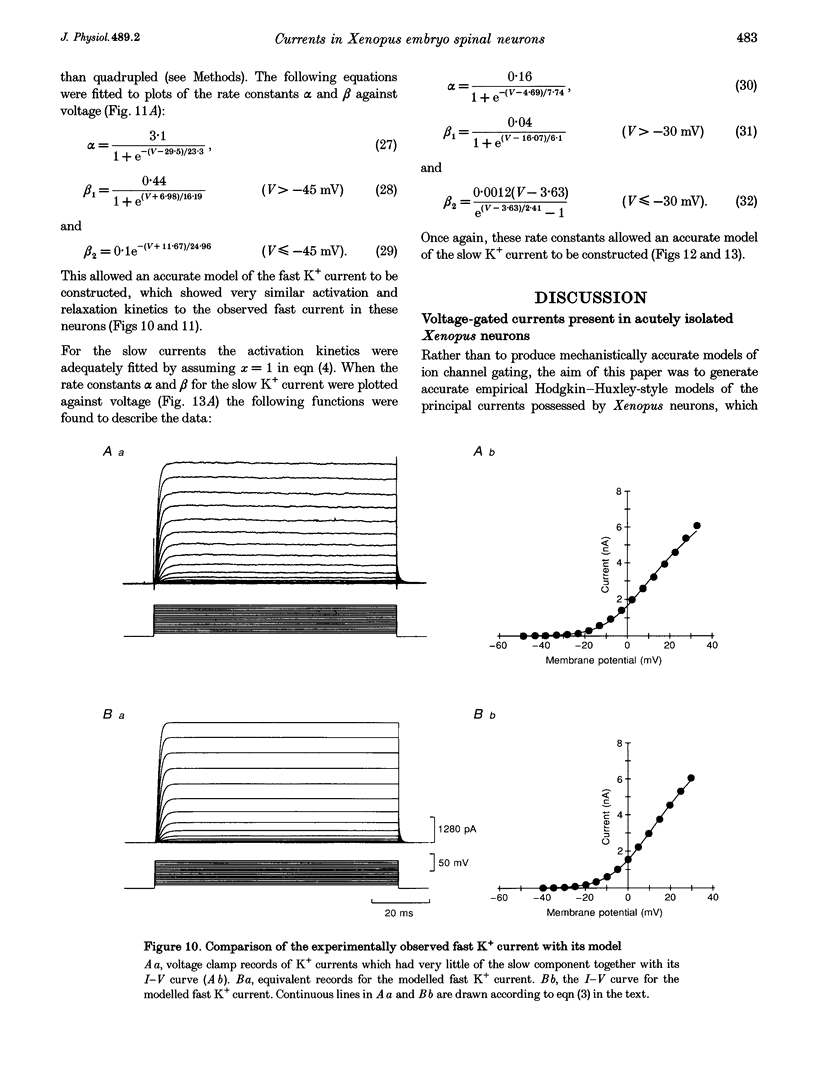
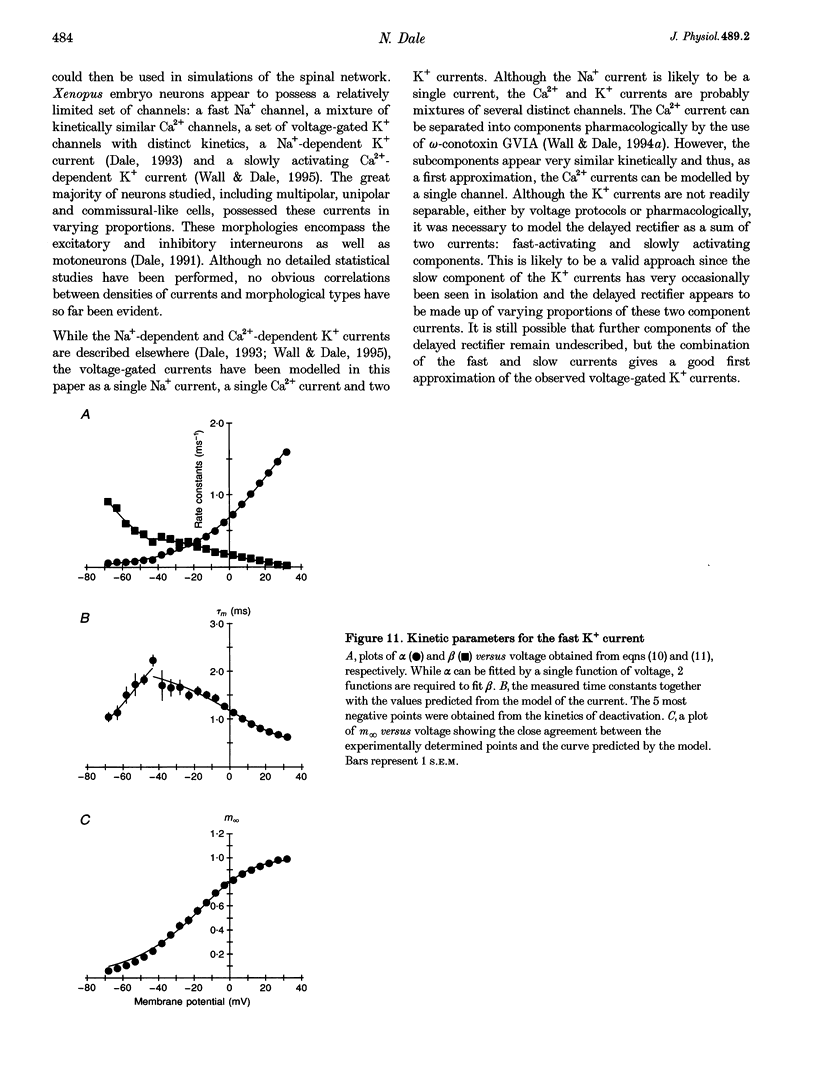
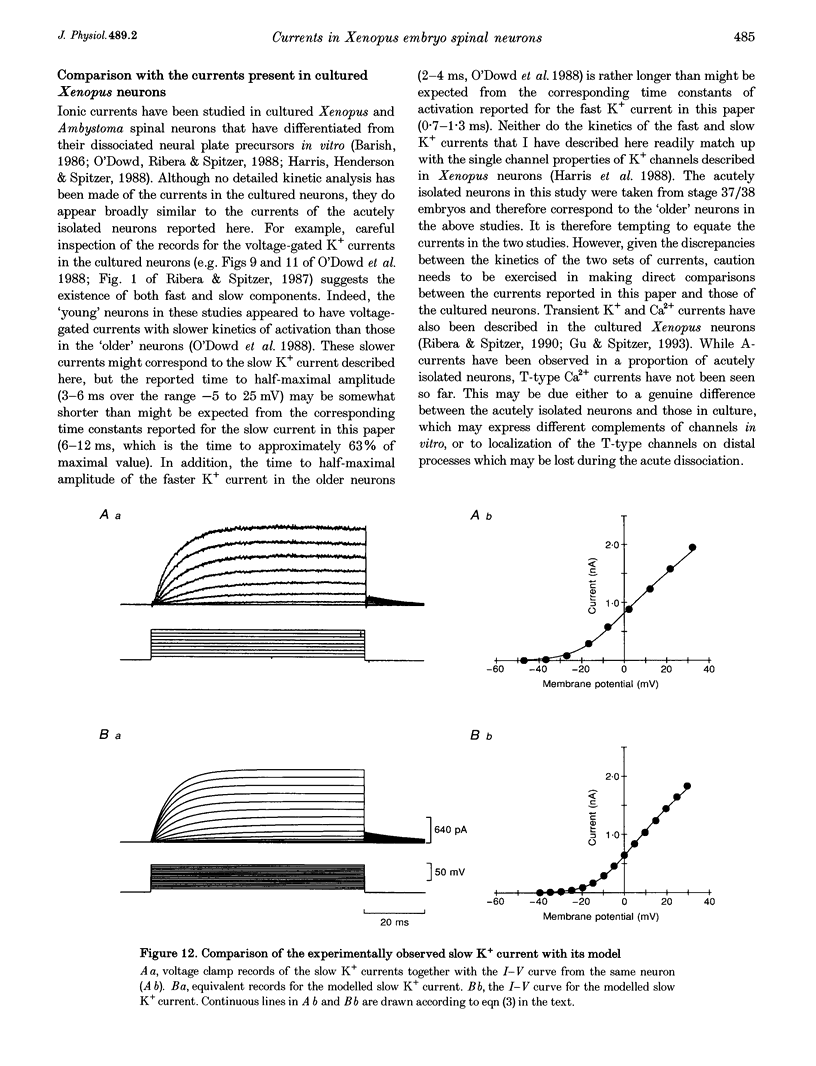
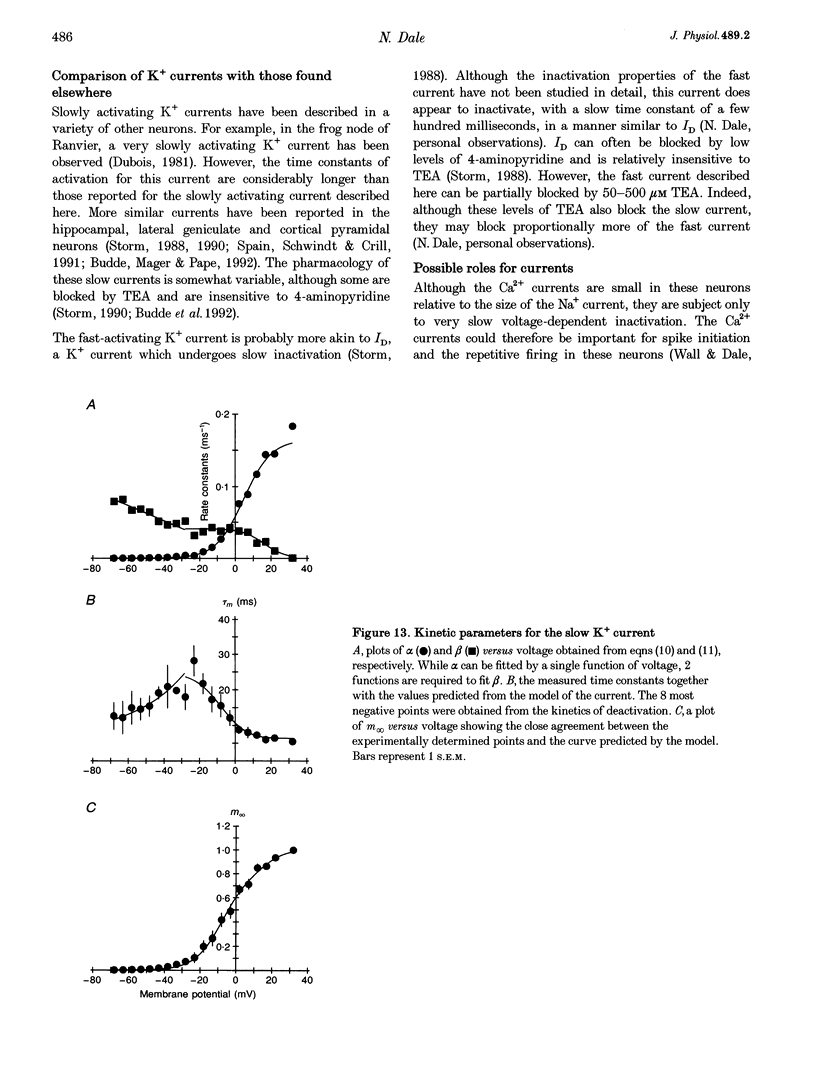
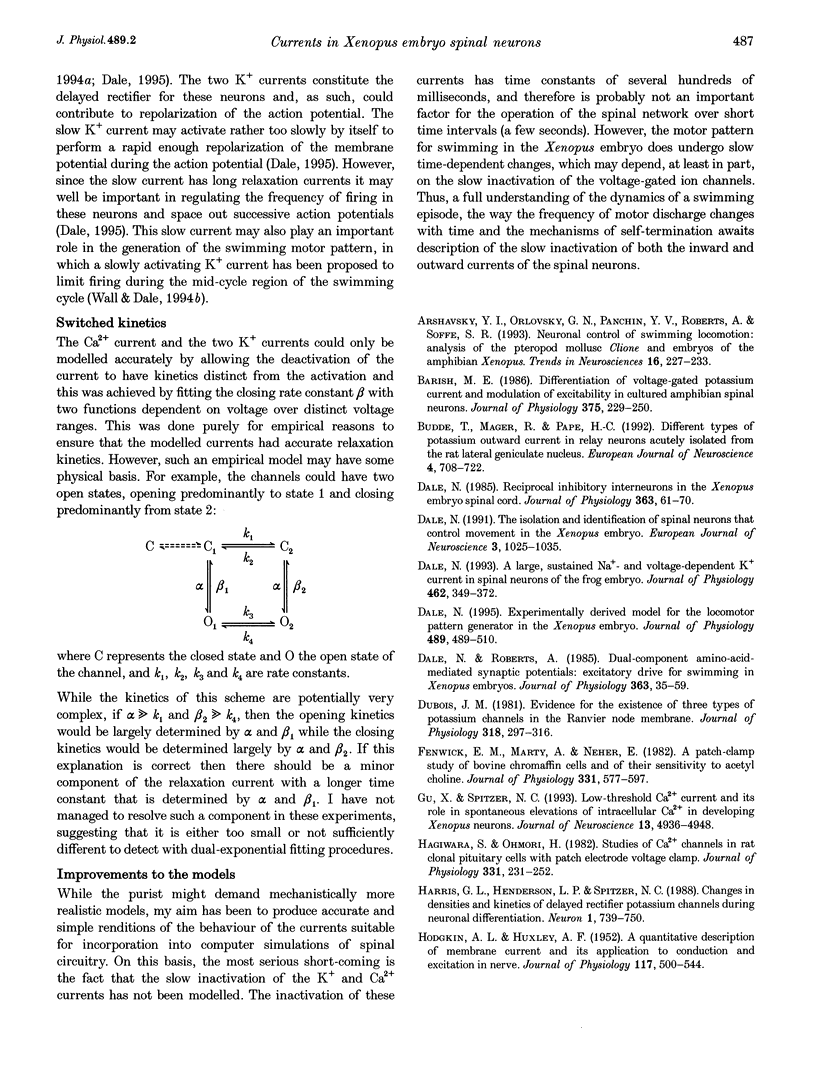
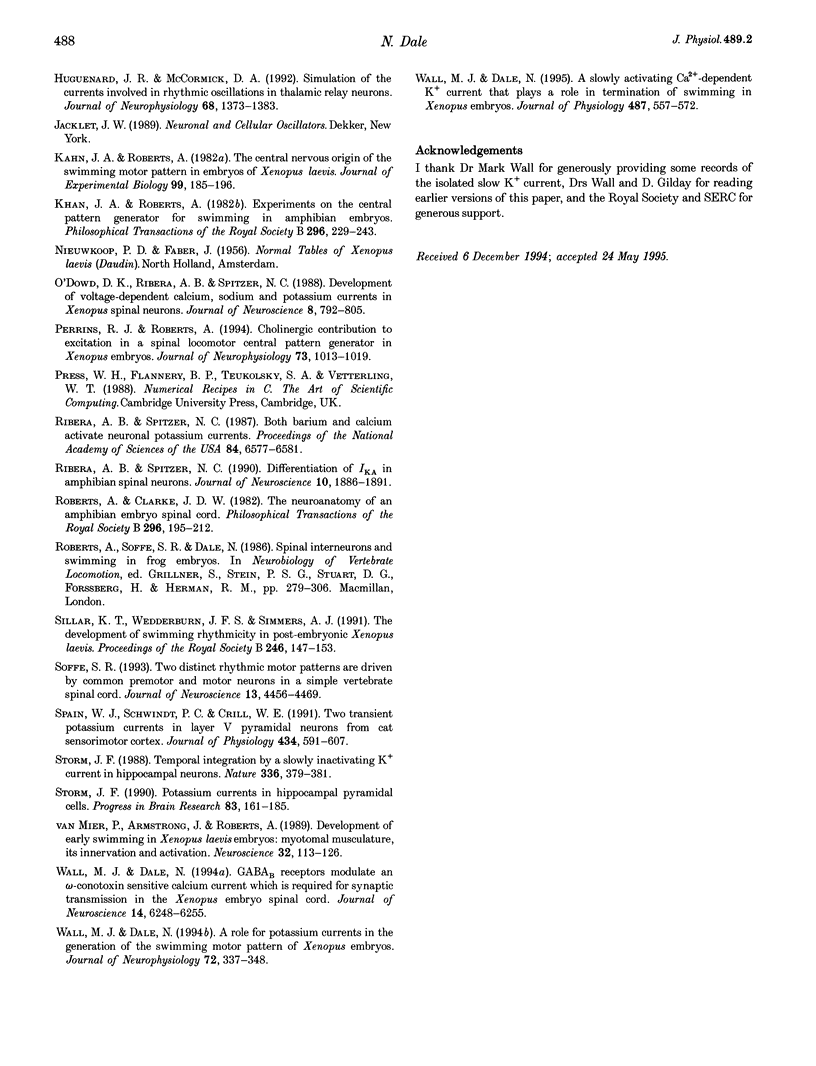
Selected References
These references are in PubMed. This may not be the complete list of references from this article.
- Arshavsky YuI, Orlovsky G. N., Panchin YuV, Roberts A., Soffe S. R. Neuronal control of swimming locomotion: analysis of the pteropod mollusc Clione and embryos of the amphibian Xenopus. Trends Neurosci. 1993 Jun;16(6):227–233. doi: 10.1016/0166-2236(93)90161-e. [DOI] [PubMed] [Google Scholar]
- Barish M. E. Differentiation of voltage-gated potassium current and modulation of excitability in cultured amphibian spinal neurones. J Physiol. 1986 Jun;375:229–250. doi: 10.1113/jphysiol.1986.sp016114. [DOI] [PMC free article] [PubMed] [Google Scholar]
- Budde Thomas, Mager Ralph, Pape Hans-Christian. Different Types of Potassium Outward Current in Relay Neurons Acutely Isolated from the Rat Lateral Geniculate Nucleus. Eur J Neurosci. 1992;4(8):708–722. doi: 10.1111/j.1460-9568.1992.tb00180.x. [DOI] [PubMed] [Google Scholar]
- Dale N. A large, sustained Na(+)- and voltage-dependent K+ current in spinal neurons of the frog embryo. J Physiol. 1993 Mar;462:349–372. doi: 10.1113/jphysiol.1993.sp019559. [DOI] [PMC free article] [PubMed] [Google Scholar]
- Dale N. Experimentally derived model for the locomotor pattern generator in the Xenopus embryo. J Physiol. 1995 Dec 1;489(Pt 2):489–510. doi: 10.1113/jphysiol.1995.sp021067. [DOI] [PMC free article] [PubMed] [Google Scholar]
- Dale N. Reciprocal inhibitory interneurones in the Xenopus embryo spinal cord. J Physiol. 1985 Jun;363:61–70. doi: 10.1113/jphysiol.1985.sp015695. [DOI] [PMC free article] [PubMed] [Google Scholar]
- Dale N., Roberts A. Dual-component amino-acid-mediated synaptic potentials: excitatory drive for swimming in Xenopus embryos. J Physiol. 1985 Jun;363:35–59. doi: 10.1113/jphysiol.1985.sp015694. [DOI] [PMC free article] [PubMed] [Google Scholar]
- Dale Nicholas. The Isolation and Identification of Spinal Neurons That Control Movement in the Xenopus Embryo. Eur J Neurosci. 1991;3(10):1025–1035. doi: 10.1111/j.1460-9568.1991.tb00039.x. [DOI] [PubMed] [Google Scholar]
- Dubois J. M. Evidence for the existence of three types of potassium channels in the frog Ranvier node membrane. J Physiol. 1981 Sep;318:297–316. doi: 10.1113/jphysiol.1981.sp013865. [DOI] [PMC free article] [PubMed] [Google Scholar]
- Fenwick E. M., Marty A., Neher E. A patch-clamp study of bovine chromaffin cells and of their sensitivity to acetylcholine. J Physiol. 1982 Oct;331:577–597. doi: 10.1113/jphysiol.1982.sp014393. [DOI] [PMC free article] [PubMed] [Google Scholar]
- Gu X., Spitzer N. C. Low-threshold Ca2+ current and its role in spontaneous elevations of intracellular Ca2+ in developing Xenopus neurons. J Neurosci. 1993 Nov;13(11):4936–4948. doi: 10.1523/JNEUROSCI.13-11-04936.1993. [DOI] [PMC free article] [PubMed] [Google Scholar]
- HODGKIN A. L., HUXLEY A. F. A quantitative description of membrane current and its application to conduction and excitation in nerve. J Physiol. 1952 Aug;117(4):500–544. doi: 10.1113/jphysiol.1952.sp004764. [DOI] [PMC free article] [PubMed] [Google Scholar]
- Hagiwara S., Ohmori H. Studies of calcium channels in rat clonal pituitary cells with patch electrode voltage clamp. J Physiol. 1982 Oct;331:231–252. doi: 10.1113/jphysiol.1982.sp014371. [DOI] [PMC free article] [PubMed] [Google Scholar]
- Harris G. L., Henderson L. P., Spitzer N. C. Changes in densities and kinetics of delayed rectifier potassium channels during neuronal differentiation. Neuron. 1988 Oct;1(8):739–750. doi: 10.1016/0896-6273(88)90172-9. [DOI] [PubMed] [Google Scholar]
- Huguenard J. R., McCormick D. A. Simulation of the currents involved in rhythmic oscillations in thalamic relay neurons. J Neurophysiol. 1992 Oct;68(4):1373–1383. doi: 10.1152/jn.1992.68.4.1373. [DOI] [PubMed] [Google Scholar]
- Kahn J. A., Roberts A. Experiments on the central pattern generator for swimming in amphibian embryos. Philos Trans R Soc Lond B Biol Sci. 1982 Jan 27;296(1081):229–243. doi: 10.1098/rstb.1982.0004. [DOI] [PubMed] [Google Scholar]
- Kahn J. A., Roberts A. The central nervous origin of the swimming motor pattern in embryos of Xenopus laevis. J Exp Biol. 1982 Aug;99:185–196. doi: 10.1242/jeb.99.1.185. [DOI] [PubMed] [Google Scholar]
- O'Dowd D. K., Ribera A. B., Spitzer N. C. Development of voltage-dependent calcium, sodium, and potassium currents in Xenopus spinal neurons. J Neurosci. 1988 Mar;8(3):792–805. doi: 10.1523/JNEUROSCI.08-03-00792.1988. [DOI] [PMC free article] [PubMed] [Google Scholar]
- Perrins R., Roberts A. Cholinergic contribution to excitation in a spinal locomotor central pattern generator in Xenopus embryos. J Neurophysiol. 1995 Mar;73(3):1013–1019. doi: 10.1152/jn.1995.73.3.1013. [DOI] [PubMed] [Google Scholar]
- Ribera A. B., Spitzer N. C. Both barium and calcium activate neuronal potassium currents. Proc Natl Acad Sci U S A. 1987 Sep;84(18):6577–6581. doi: 10.1073/pnas.84.18.6577. [DOI] [PMC free article] [PubMed] [Google Scholar]
- Ribera A. B., Spitzer N. C. Differentiation of IKA in amphibian spinal neurons. J Neurosci. 1990 Jun;10(6):1886–1891. doi: 10.1523/JNEUROSCI.10-06-01886.1990. [DOI] [PMC free article] [PubMed] [Google Scholar]
- Roberts A., Clarke J. D. The neuroanatomy of an amphibian embryo spinal cord. Philos Trans R Soc Lond B Biol Sci. 1982 Jan 27;296(1081):195–212. doi: 10.1098/rstb.1982.0002. [DOI] [PubMed] [Google Scholar]
- Sillar K. T., Wedderburn J. F., Simmers A. J. The development of swimming rhythmicity in post-embryonic Xenopus laevis. Proc Biol Sci. 1991 Nov 22;246(1316):147–153. doi: 10.1098/rspb.1991.0137. [DOI] [PubMed] [Google Scholar]
- Soffe S. R. Two distinct rhythmic motor patterns are driven by common premotor and motor neurons in a simple vertebrate spinal cord. J Neurosci. 1993 Oct;13(10):4456–4469. doi: 10.1523/JNEUROSCI.13-10-04456.1993. [DOI] [PMC free article] [PubMed] [Google Scholar]
- Spain W. J., Schwindt P. C., Crill W. E. Two transient potassium currents in layer V pyramidal neurones from cat sensorimotor cortex. J Physiol. 1991 Mar;434:591–607. doi: 10.1113/jphysiol.1991.sp018488. [DOI] [PMC free article] [PubMed] [Google Scholar]
- Storm J. F. Potassium currents in hippocampal pyramidal cells. Prog Brain Res. 1990;83:161–187. doi: 10.1016/s0079-6123(08)61248-0. [DOI] [PubMed] [Google Scholar]
- Storm J. F. Temporal integration by a slowly inactivating K+ current in hippocampal neurons. Nature. 1988 Nov 24;336(6197):379–381. doi: 10.1038/336379a0. [DOI] [PubMed] [Google Scholar]
- Wall M. J., Dale N. A role for potassium currents in the generation of the swimming motor pattern of Xenopus embryos. J Neurophysiol. 1994 Jul;72(1):337–348. doi: 10.1152/jn.1994.72.1.337. [DOI] [PubMed] [Google Scholar]
- Wall M. J., Dale N. A slowly activating Ca(2+)-dependent K+ current that plays a role in termination of swimming in Xenopus embryos. J Physiol. 1995 Sep 15;487(Pt 3):557–572. doi: 10.1113/jphysiol.1995.sp020900. [DOI] [PMC free article] [PubMed] [Google Scholar]
- Wall M. J., Dale N. GABAB receptors modulate an omega-conotoxin-sensitive calcium current that is required for synaptic transmission in the Xenopus embryo spinal cord. J Neurosci. 1994 Oct;14(10):6248–6255. doi: 10.1523/JNEUROSCI.14-10-06248.1994. [DOI] [PMC free article] [PubMed] [Google Scholar]
- van Mier P., Armstrong J., Roberts A. Development of early swimming in Xenopus laevis embryos: myotomal musculature, its innervation and activation. Neuroscience. 1989;32(1):113–126. doi: 10.1016/0306-4522(89)90111-5. [DOI] [PubMed] [Google Scholar]