Abstract
1. A method has been developed to discriminate between the rate of ATP hydrolysis associated with calcium uptake into the sarcoplasmic reticulum (SR) and force development of the contractile apparatus in mechanically or saponin-skinned skeletal muscle fibres. The rate of ATP hydrolysis was determined in fibres of different types from the iliofibularis muscle of Xenopus laevis by enzymatic coupling of ATP re-synthesis to the oxidation of NADH. 2. The ATPase activity was determined before and after exposure of the preparations for 30 min to a solution containing 0.5% Triton X-100, which effectively abolishes the SR ATPase activity. The fibres were activated in a solution containing 5 mM caffeine to ensure that calcium uptake into the SR was maximal. 3. At saturating Ca2+ concentrations the actomyosin (AM) and SR ATPase activities in fast-twitch fibres, at 4.3 degrees C, amounted to 1.52 +/- 0.07 and 0.58 +/- 0.10 mumol s-1 (g dry wt)-1, respectively (means +/- S.E.M.; n = 25). The SR ATPase activity was 25% of the total ATPase activity. At submaximal calcium concentrations the AM ATPase activity varied in proportion to the isometric force. 4. The calcium sensitivity of the SR ATPase was larger than that of the AM ATPase and its dependence on [Ca2+] was less steep. The AM ATPase activity was half-maximal at a pCa of 6.11 (pCa = -log [Ca2+]) whereas the SR ATPase activity was half-maximal at a pCa of 6.62. 5. In Triton X-100-treated fibres, at different 2,3-butanedione monoxime (BDM) concentrations, the AM ATPase activity and isometric force varied proportionally. The SR ATPase activity determined by extrapolation of the total ATPase activity in mechanically skinned or saponin-treated fibres to zero force, was independent of the BDM concentration in the range studied (0-20 mM). The values obtained for the SR ATPase activity in this way were similar to those obtained with Triton X-100 treatment. 6. The AM ATPase activity in slow-twitch fibres amounted to 0.74 +/- 0.13 mumol s-1 (g dry wt)-1, i.e. about a factor of two smaller than in fast-twitch fibres. The SR ATPase activity amounted to 0.47 +/- 0.07 mumol s-1 (g dry wt)-1, i.e. rather similar to the value in fast-twitch fibres. The proportion of the total ATPase activity that was due to SR ATPase (40%) was larger than in fast-twitch fibres. 7. The temperature dependence of the AM and SR ATPase activities in fast-twitch fibres differed. In the temperature range 5-10 degrees C, the relative changes in AM and SR ATPase activities for a 10 degrees C temperature change (Q10) were 3.9 +/- 0.3 and 7.2 +/- 1.5, respectively.(ABSTRACT TRUNCATED AT 400 WORDS)
Full text
PDF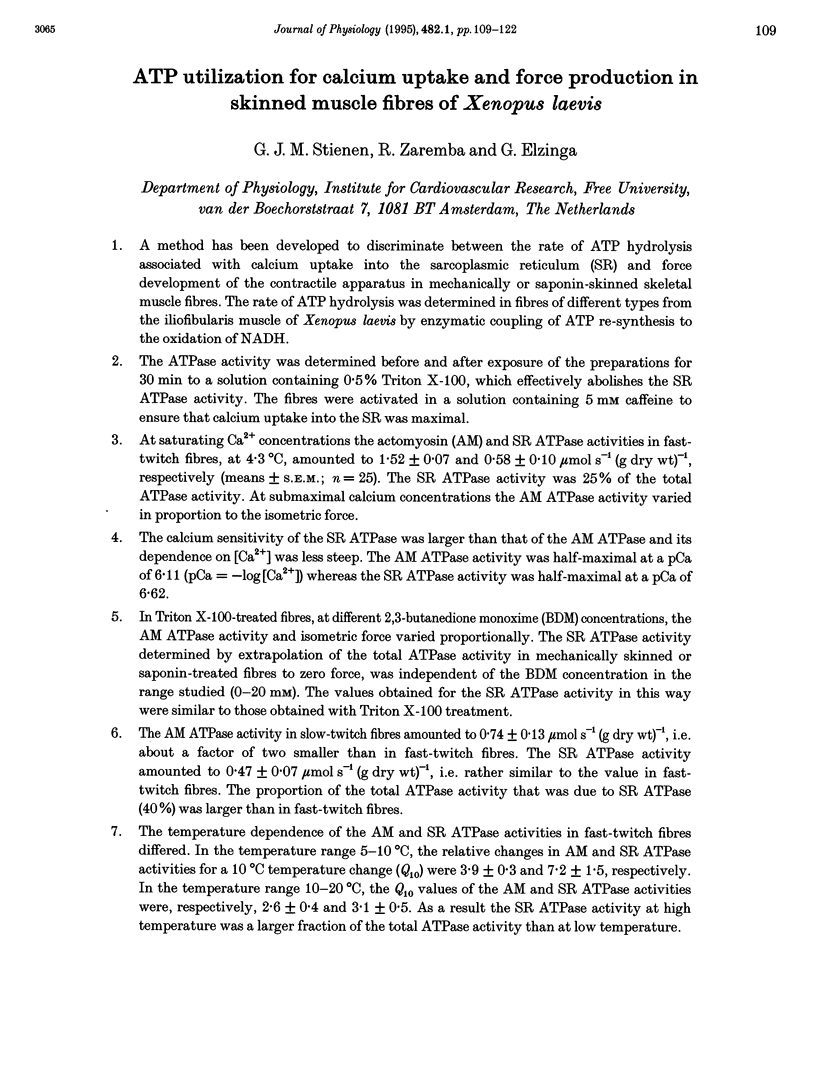
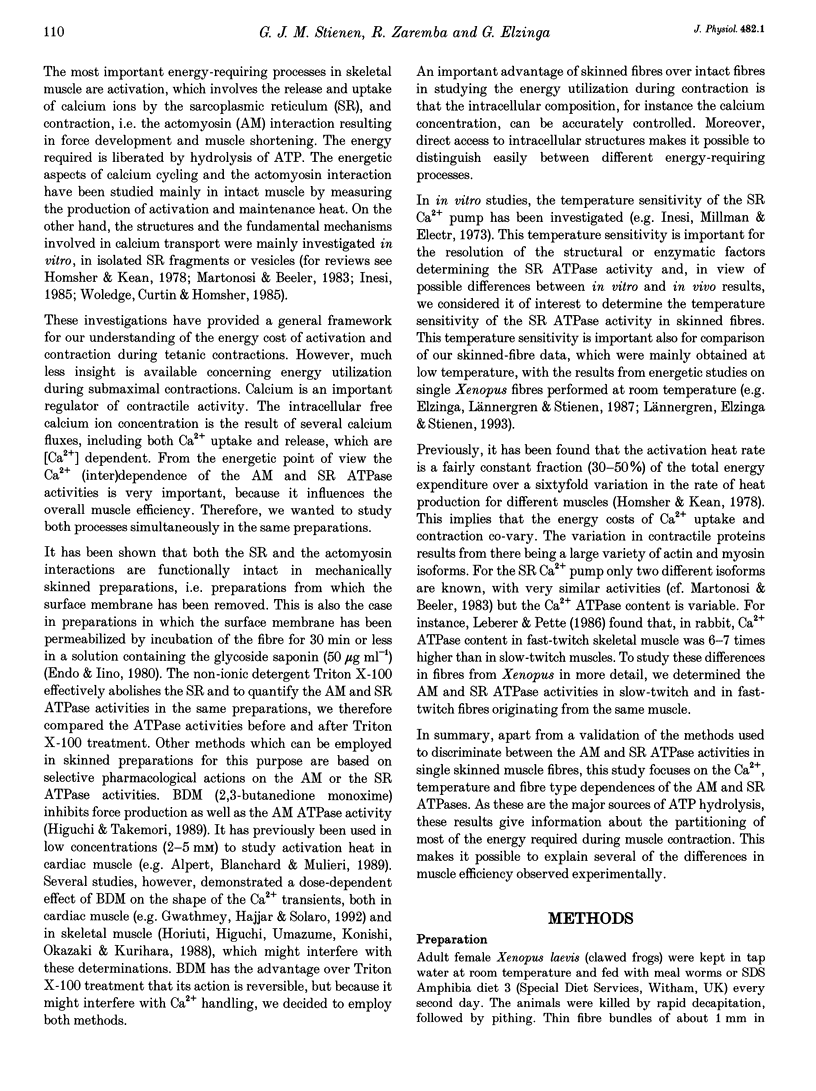
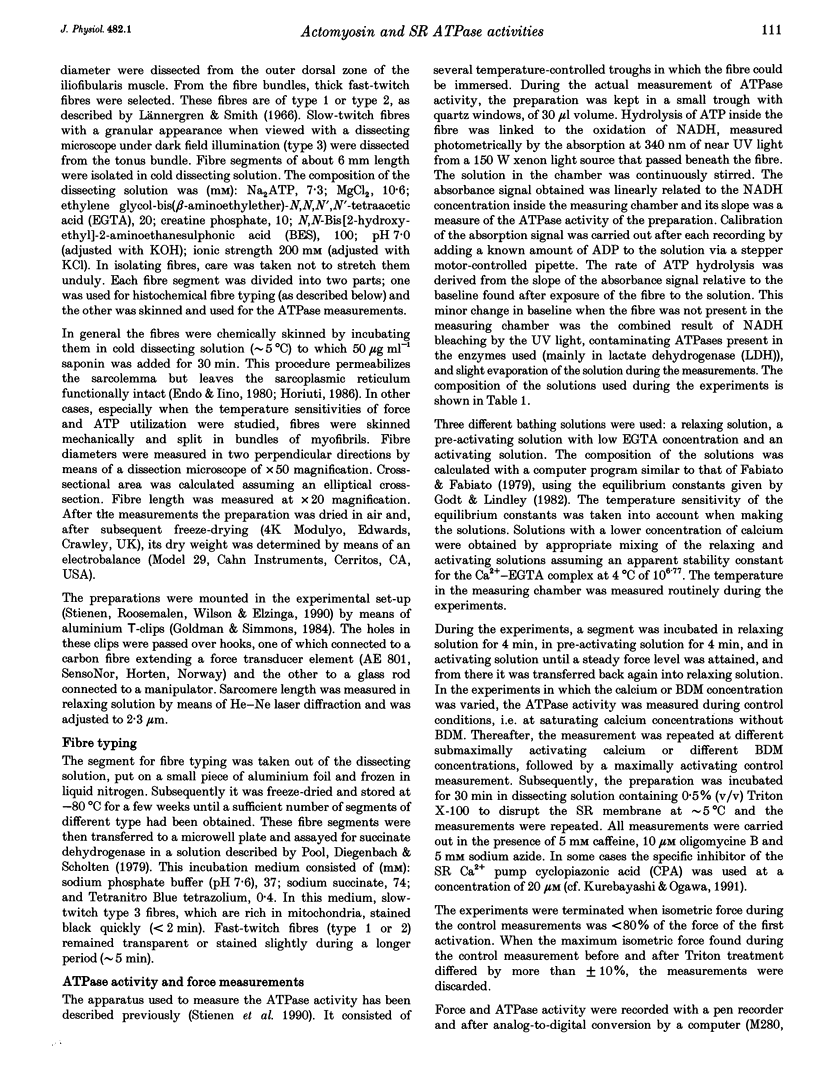
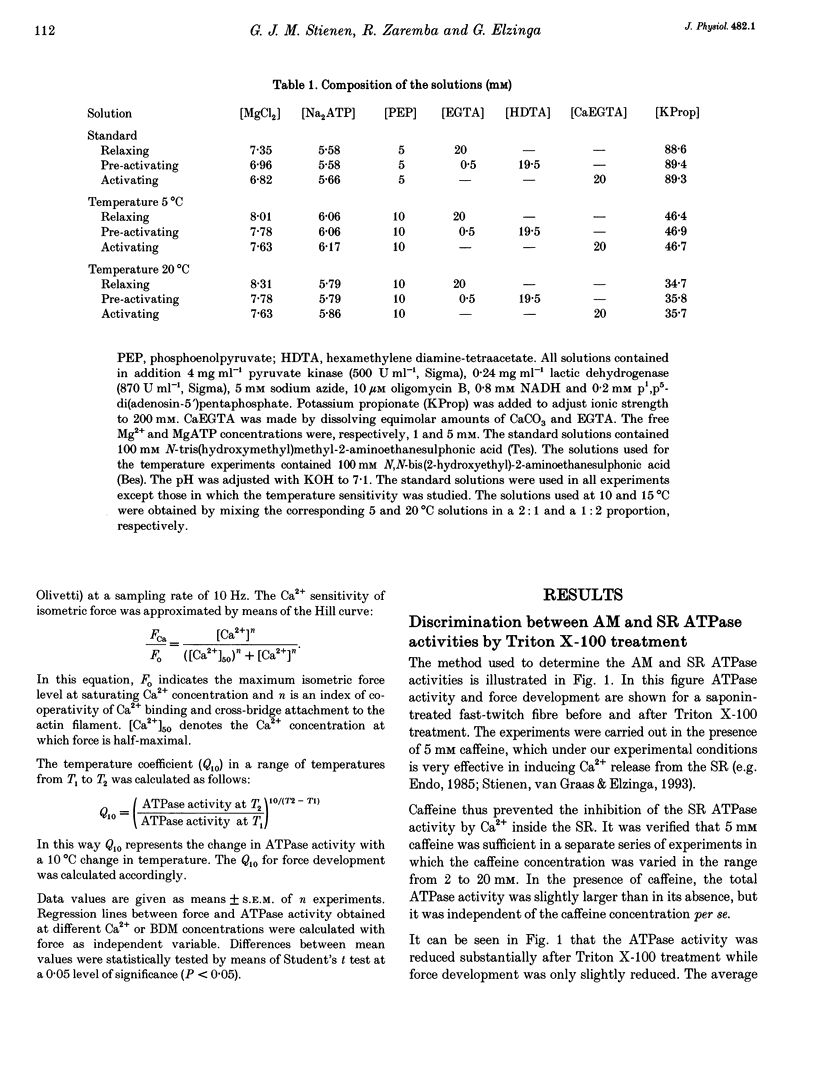
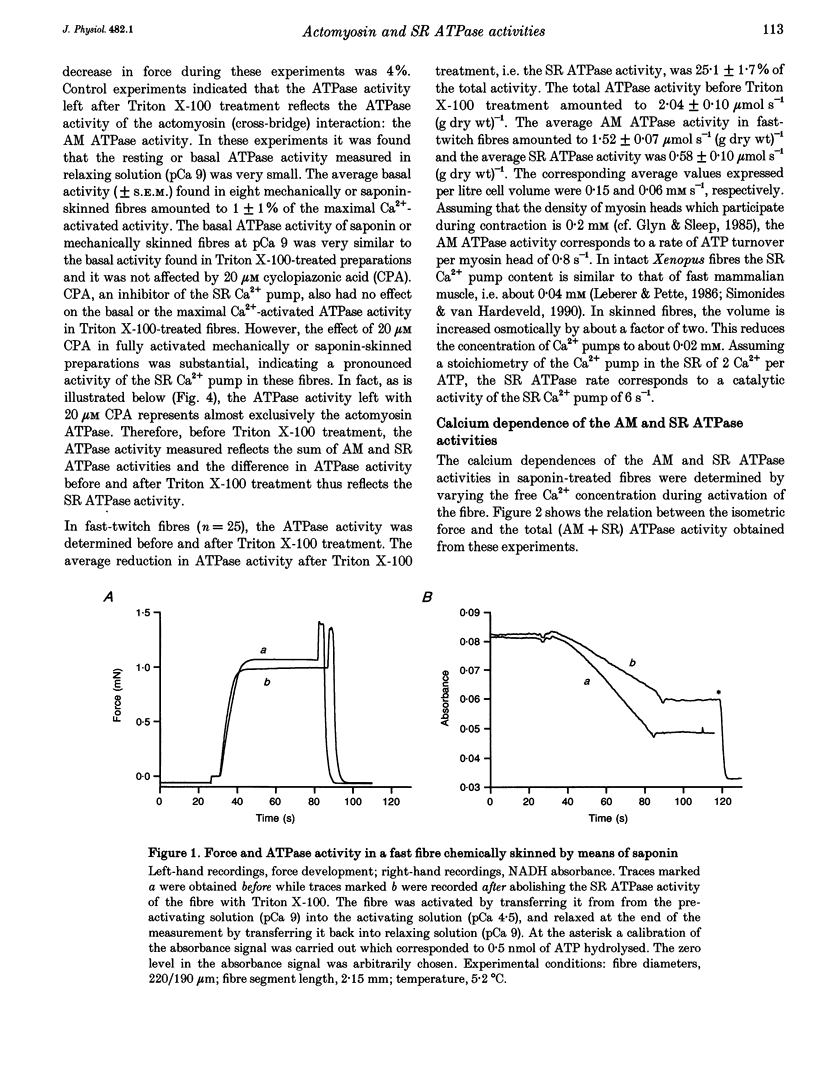
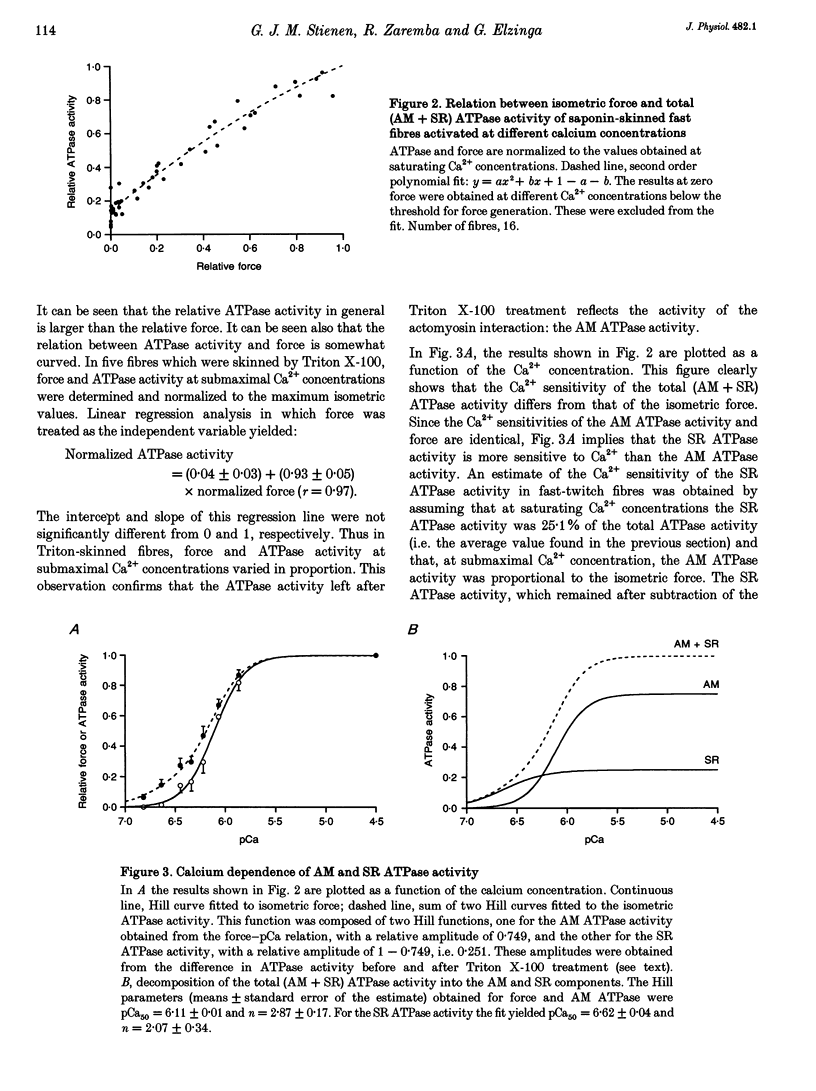
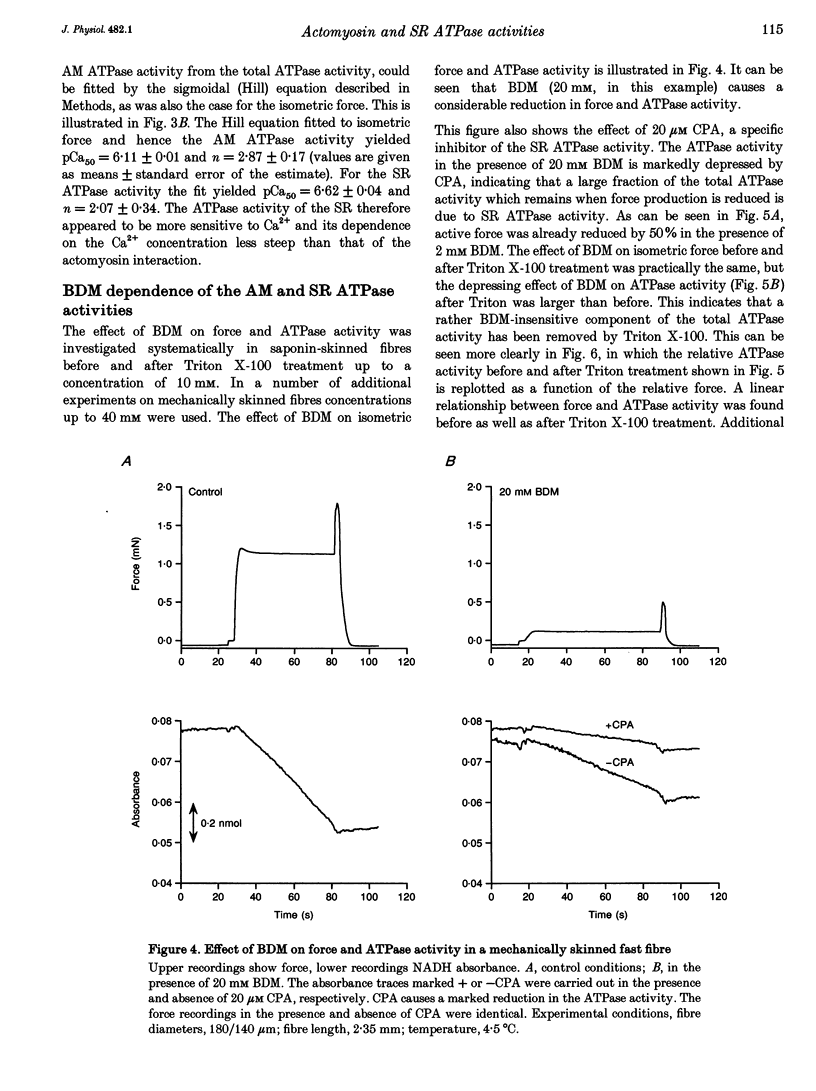
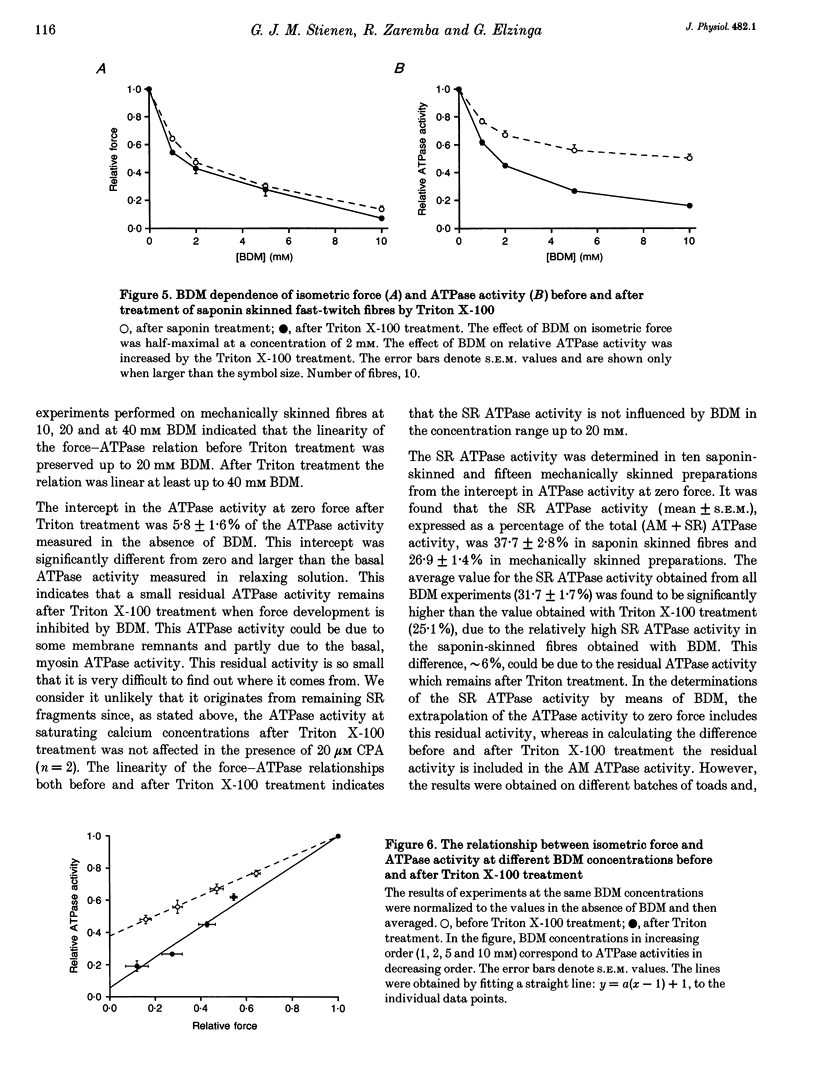
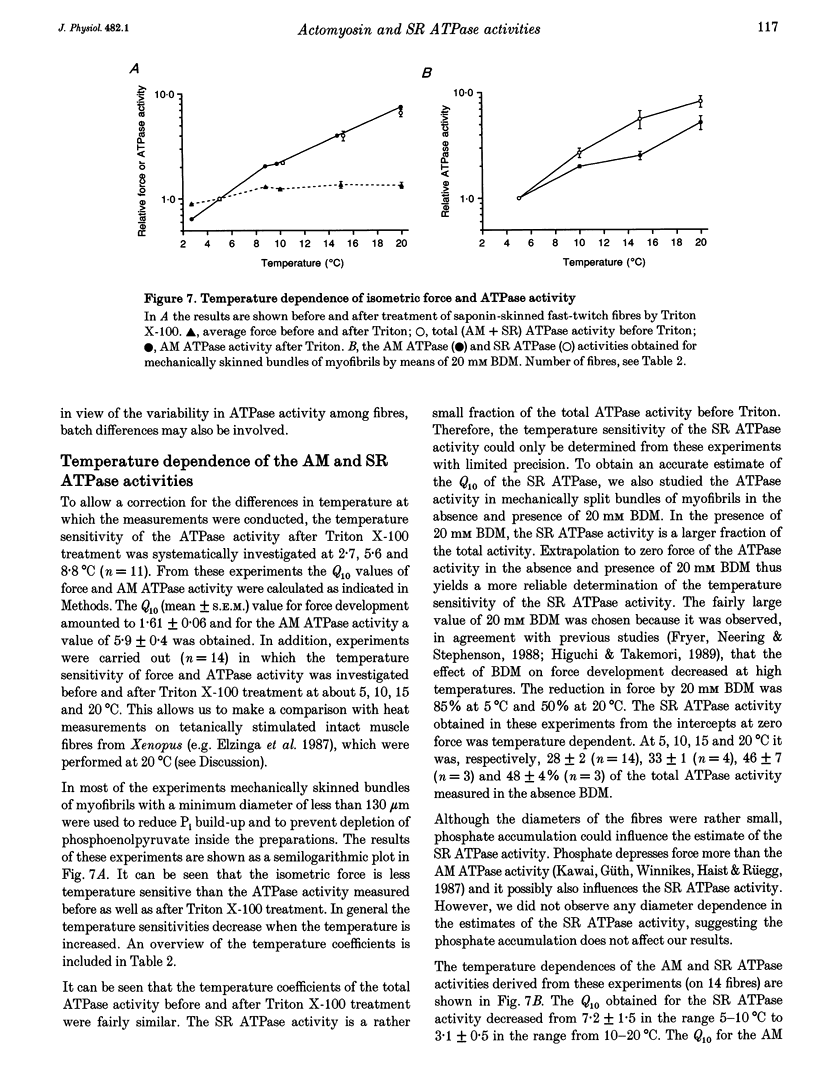
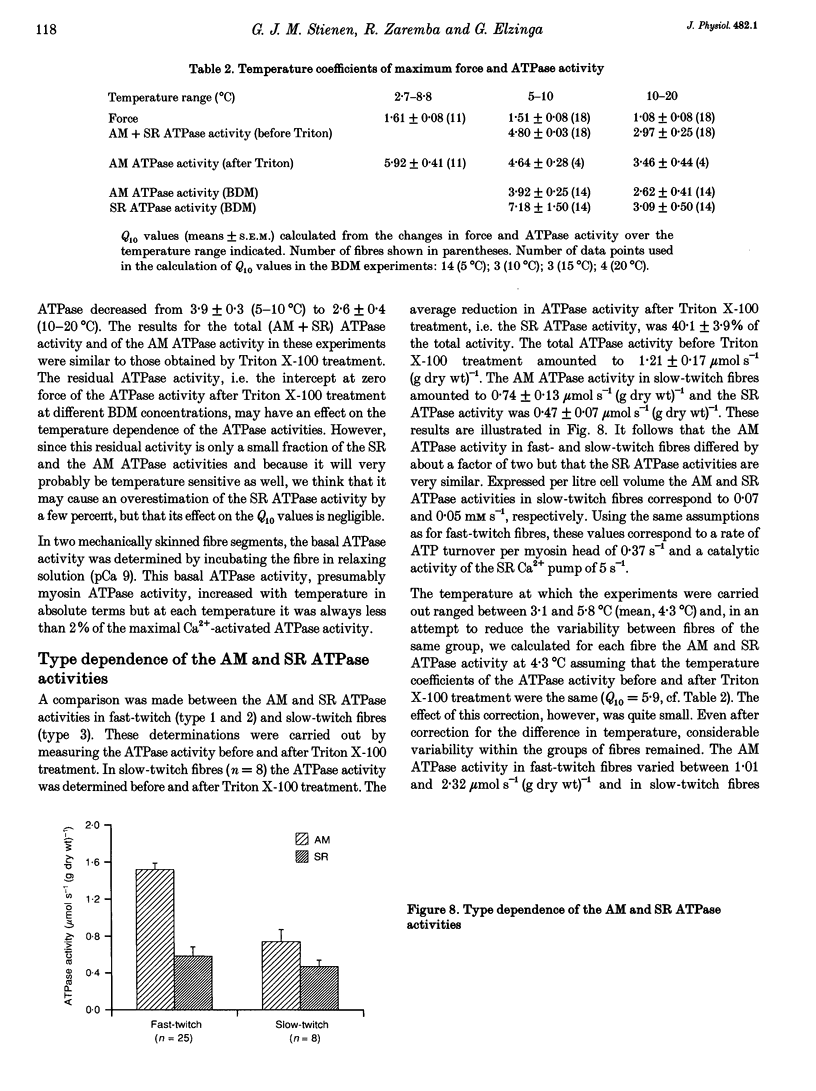
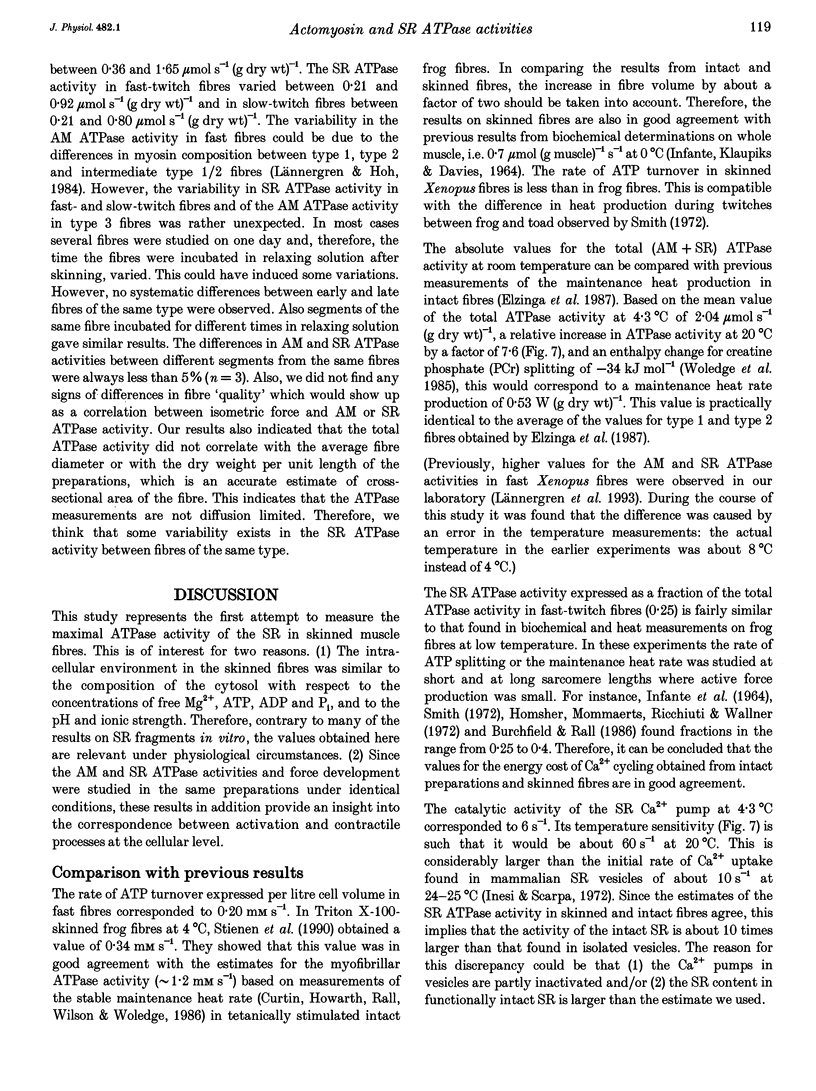
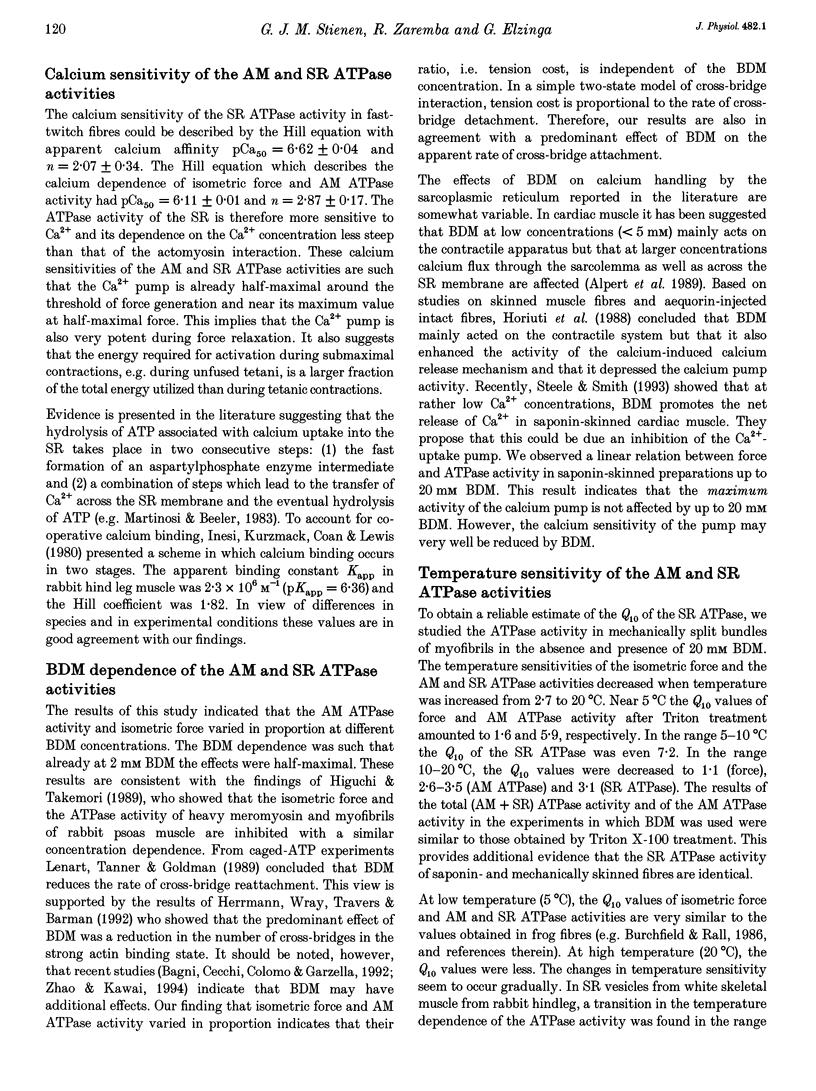
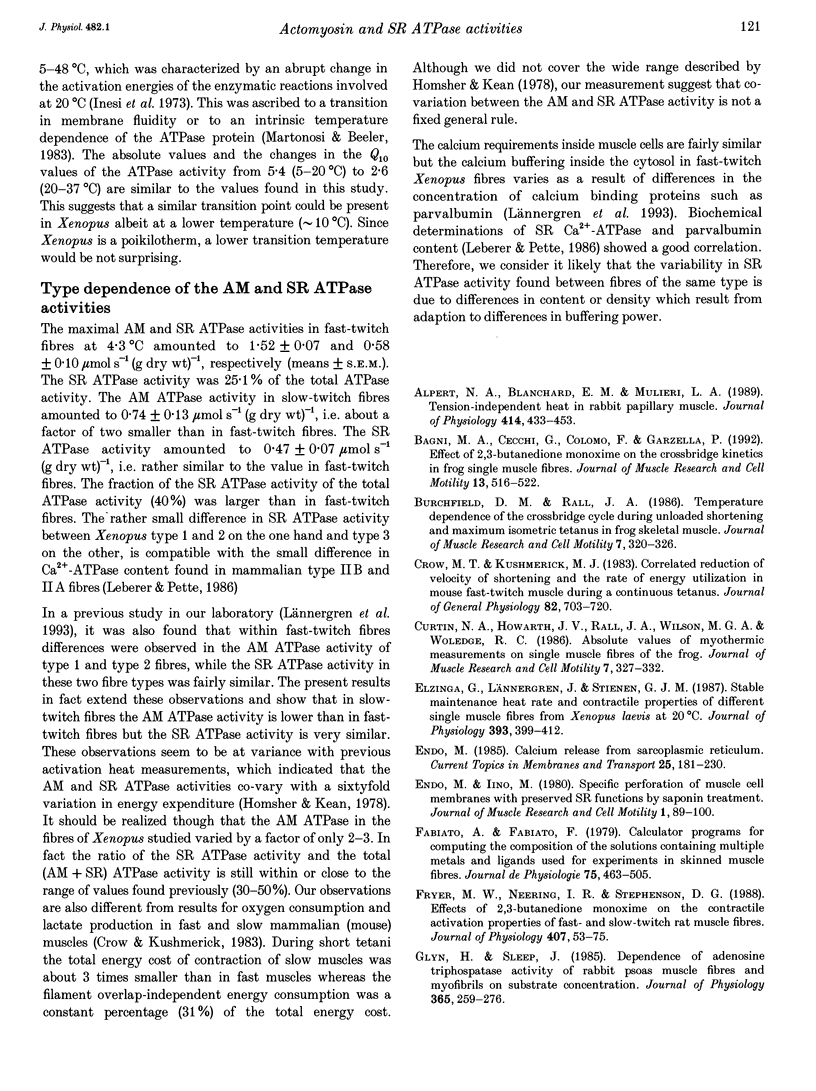
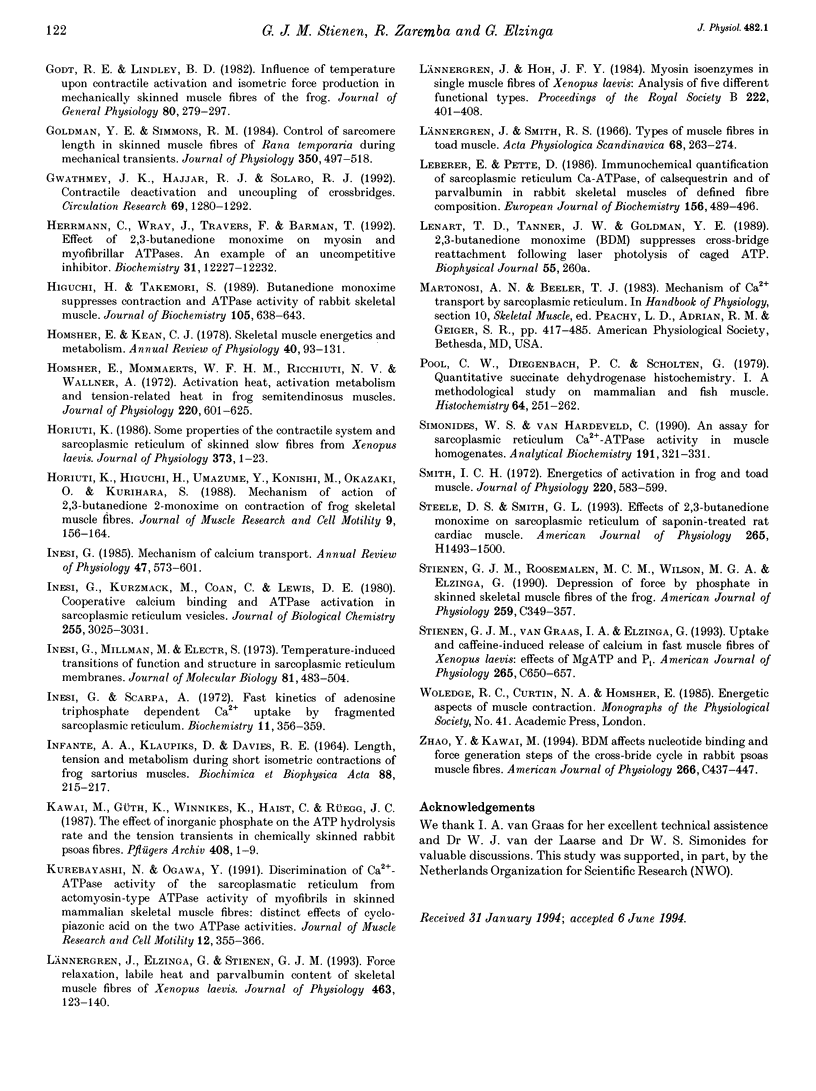
Selected References
These references are in PubMed. This may not be the complete list of references from this article.
- Alpert N. R., Blanchard E. M., Mulieri L. A. Tension-independent heat in rabbit papillary muscle. J Physiol. 1989 Jul;414:433–453. doi: 10.1113/jphysiol.1989.sp017697. [DOI] [PMC free article] [PubMed] [Google Scholar]
- Bagni M. A., Cecchi G., Colomo F., Garzella P. Effects of 2,3-butanedione monoxime on the crossbridge kinetics in frog single muscle fibres. J Muscle Res Cell Motil. 1992 Oct;13(5):516–522. doi: 10.1007/BF01737994. [DOI] [PubMed] [Google Scholar]
- Burchfield D. M., Rall J. A. Temperature dependence of the crossbridge cycle during unloaded shortening and maximum isometric tetanus in frog skeletal muscle. J Muscle Res Cell Motil. 1986 Aug;7(4):320–326. doi: 10.1007/BF01753652. [DOI] [PubMed] [Google Scholar]
- Crow M. T., Kushmerick M. J. Correlated reduction of velocity of shortening and the rate of energy utilization in mouse fast-twitch muscle during a continuous tetanus. J Gen Physiol. 1983 Nov;82(5):703–720. doi: 10.1085/jgp.82.5.703. [DOI] [PMC free article] [PubMed] [Google Scholar]
- Curtin N. A., Howarth J. V., Rall J. A., Wilson M. G., Woledge R. C. Absolute values of myothermic measurements on single muscle fibres from frog. J Muscle Res Cell Motil. 1986 Aug;7(4):327–332. doi: 10.1007/BF01753653. [DOI] [PubMed] [Google Scholar]
- Elzinga G., Lännergren J., Stienen G. J. Stable maintenance heat rate and contractile properties of different single muscle fibres from Xenopus laevis at 20 degrees C. J Physiol. 1987 Dec;393:399–412. doi: 10.1113/jphysiol.1987.sp016829. [DOI] [PMC free article] [PubMed] [Google Scholar]
- Endo M., Iino M. Specific perforation of muscle cell membranes with preserved SR functions by saponin treatment. J Muscle Res Cell Motil. 1980 Mar;1(1):89–100. doi: 10.1007/BF00711927. [DOI] [PubMed] [Google Scholar]
- Fabiato A., Fabiato F. Calculator programs for computing the composition of the solutions containing multiple metals and ligands used for experiments in skinned muscle cells. J Physiol (Paris) 1979;75(5):463–505. [PubMed] [Google Scholar]
- Fryer M. W., Neering I. R., Stephenson D. G. Effects of 2,3-butanedione monoxime on the contractile activation properties of fast- and slow-twitch rat muscle fibres. J Physiol. 1988 Dec;407:53–75. doi: 10.1113/jphysiol.1988.sp017403. [DOI] [PMC free article] [PubMed] [Google Scholar]
- Glyn H., Sleep J. Dependence of adenosine triphosphatase activity of rabbit psoas muscle fibres and myofibrils on substrate concentration. J Physiol. 1985 Aug;365:259–276. doi: 10.1113/jphysiol.1985.sp015770. [DOI] [PMC free article] [PubMed] [Google Scholar]
- Godt R. E., Lindley B. D. Influence of temperature upon contractile activation and isometric force production in mechanically skinned muscle fibers of the frog. J Gen Physiol. 1982 Aug;80(2):279–297. doi: 10.1085/jgp.80.2.279. [DOI] [PMC free article] [PubMed] [Google Scholar]
- Goldman Y. E., Simmons R. M. Control of sarcomere length in skinned muscle fibres of Rana temporaria during mechanical transients. J Physiol. 1984 May;350:497–518. doi: 10.1113/jphysiol.1984.sp015215. [DOI] [PMC free article] [PubMed] [Google Scholar]
- Gwathmey J. K., Hajjar R. J., Solaro R. J. Contractile deactivation and uncoupling of crossbridges. Effects of 2,3-butanedione monoxime on mammalian myocardium. Circ Res. 1991 Nov;69(5):1280–1292. doi: 10.1161/01.res.69.5.1280. [DOI] [PubMed] [Google Scholar]
- Herrmann C., Wray J., Travers F., Barman T. Effect of 2,3-butanedione monoxime on myosin and myofibrillar ATPases. An example of an uncompetitive inhibitor. Biochemistry. 1992 Dec 8;31(48):12227–12232. doi: 10.1021/bi00163a036. [DOI] [PubMed] [Google Scholar]
- Higuchi H., Takemori S. Butanedione monoxime suppresses contraction and ATPase activity of rabbit skeletal muscle. J Biochem. 1989 Apr;105(4):638–643. doi: 10.1093/oxfordjournals.jbchem.a122717. [DOI] [PubMed] [Google Scholar]
- Homsher E., Kean C. J. Skeletal muscle energetics and metabolism. Annu Rev Physiol. 1978;40:93–131. doi: 10.1146/annurev.ph.40.030178.000521. [DOI] [PubMed] [Google Scholar]
- Homsher E., Mommaerts W. F., Ricchiuti N. V., Wallner A. Activation heat, activation metabolism and tension-related heat in frog semitendinosus muscles. J Physiol. 1972 Feb;220(3):601–625. doi: 10.1113/jphysiol.1972.sp009725. [DOI] [PMC free article] [PubMed] [Google Scholar]
- Horiuti K., Higuchi H., Umazume Y., Konishi M., Okazaki O., Kurihara S. Mechanism of action of 2, 3-butanedione 2-monoxime on contraction of frog skeletal muscle fibres. J Muscle Res Cell Motil. 1988 Apr;9(2):156–164. doi: 10.1007/BF01773737. [DOI] [PubMed] [Google Scholar]
- Horiuti K. Some properties of the contractile system and sarcoplasmic reticulum of skinned slow fibres from Xenopus muscle. J Physiol. 1986 Apr;373:1–23. doi: 10.1113/jphysiol.1986.sp016032. [DOI] [PMC free article] [PubMed] [Google Scholar]
- INFANTE A. A., KLAUPIKS D., DAVIES R. E. LENGTH, TENSION AND METABOLISM DURING SHORT ISOMETRIC CONTRACTIONS OF FROG SARTORIUS MUSCLES. Biochim Biophys Acta. 1964 Jul 29;88:215–217. doi: 10.1016/0926-6577(64)90171-8. [DOI] [PubMed] [Google Scholar]
- Inesi G., Kurzmack M., Coan C., Lewis D. E. Cooperative calcium binding and ATPase activation in sarcoplasmic reticulum vesicles. J Biol Chem. 1980 Apr 10;255(7):3025–3031. [PubMed] [Google Scholar]
- Inesi G. Mechanism of calcium transport. Annu Rev Physiol. 1985;47:573–601. doi: 10.1146/annurev.ph.47.030185.003041. [DOI] [PubMed] [Google Scholar]
- Inesi G., Millman M., Eletr S. Temperature-induced transitions of function and structure in sarcoplasmic reticulum membranes. J Mol Biol. 1973 Dec 25;81(4):483–504. doi: 10.1016/0022-2836(73)90518-4. [DOI] [PubMed] [Google Scholar]
- Inesi G., Scarpa A. [Fast kinetics of adenosine triphosphate dependent Ca 2+ uptake by fragmented sarcoplasmic reticulum]. Biochemistry. 1972 Feb 1;11(3):356–359. doi: 10.1021/bi00753a008. [DOI] [PubMed] [Google Scholar]
- Kurebayashi N., Ogawa Y. Discrimination of Ca(2+)-ATPase activity of the sarcoplasmic reticulum from actomyosin-type ATPase activity of myofibrils in skinned mammalian skeletal muscle fibres: distinct effects of cyclopiazonic acid on the two ATPase activities. J Muscle Res Cell Motil. 1991 Aug;12(4):355–365. doi: 10.1007/BF01738590. [DOI] [PubMed] [Google Scholar]
- Leberer E., Pette D. Immunochemical quantification of sarcoplasmic reticulum Ca-ATPase, of calsequestrin and of parvalbumin in rabbit skeletal muscles of defined fiber composition. Eur J Biochem. 1986 May 2;156(3):489–496. doi: 10.1111/j.1432-1033.1986.tb09607.x. [DOI] [PubMed] [Google Scholar]
- Lännergren J., Elzinga G., Stienen G. J. Force relaxation, labile heat and parvalbumin content of skeletal muscle fibres of Xenopus laevis. J Physiol. 1993 Apr;463:123–140. doi: 10.1113/jphysiol.1993.sp019587. [DOI] [PMC free article] [PubMed] [Google Scholar]
- Lännergren J., Hoh J. F. Myosin isoenzymes in single muscle fibres of Xenopus laevis: analysis of five different functional types. Proc R Soc Lond B Biol Sci. 1984 Sep 22;222(1228):401–408. doi: 10.1098/rspb.1984.0072. [DOI] [PubMed] [Google Scholar]
- Pool C. W., Diegenbach P. C., Scholten G. Quantitative succinate-dehydrogenase histochemistry. I. A Methodological study on mammalian and fish muscle. Histochemistry. 1979;64(3):251–262. doi: 10.1007/BF00495025. [DOI] [PubMed] [Google Scholar]
- Simonides W. S., van Hardeveld C. An assay for sarcoplasmic reticulum Ca2(+)-ATPase activity in muscle homogenates. Anal Biochem. 1990 Dec;191(2):321–331. doi: 10.1016/0003-2697(90)90226-y. [DOI] [PubMed] [Google Scholar]
- Smith I. C. Energetics of activation in frog and toad muscle. J Physiol. 1972 Feb;220(3):583–599. doi: 10.1113/jphysiol.1972.sp009724. [DOI] [PMC free article] [PubMed] [Google Scholar]
- Steele D. S., Smith G. L. Effects of 2,3-butanedione monoxime on sarcoplasmic reticulum of saponin-treated rat cardiac muscle. Am J Physiol. 1993 Nov;265(5 Pt 2):H1493–H1500. doi: 10.1152/ajpheart.1993.265.5.H1493. [DOI] [PubMed] [Google Scholar]
- Stienen G. J., Roosemalen M. C., Wilson M. G., Elzinga G. Depression of force by phosphate in skinned skeletal muscle fibers of the frog. Am J Physiol. 1990 Aug;259(2 Pt 1):C349–C357. doi: 10.1152/ajpcell.1990.259.2.C349. [DOI] [PubMed] [Google Scholar]
- Stienen G. J., van Graas I. A., Elzinga G. Uptake and caffeine-induced release of calcium in fast muscle fibers of Xenopus laevis: effects of MgATP and P(i). Am J Physiol. 1993 Sep;265(3 Pt 1):C650–C657. doi: 10.1152/ajpcell.1993.265.3.C650. [DOI] [PubMed] [Google Scholar]
- Zhao Y., Kawai M. BDM affects nucleotide binding and force generation steps of the cross-bridge cycle in rabbit psoas muscle fibers. Am J Physiol. 1994 Feb;266(2 Pt 1):C437–C447. doi: 10.1152/ajpcell.1994.266.2.C437. [DOI] [PubMed] [Google Scholar]