Abstract
1. The effects of metabolic poisoning, intracellular Mg(2+) and pH on ATP-dependent K+ (K+ATP) channels were examined in adult mouse isolated skeletal muscle fibres using the patch clamp technique. 2. In cell-attached membrane patches, while openings of one kind of channel could only rarely be detected under control conditions, cell poisoning with fluorodinitrobenzene (FDNB), dinitrophenol (DNP) and cyanide (CN) induced a strong and partially reversible increase in channel activity. 3. Slope conductance and glibenclamide sensitivity of this outward current indicated that the channel activated during poisoning was the K+ATP channel. 4. Single channel current amplitude was reduced during poisoning, but remained unchanged when activation of the K+ATP channel was induced by cromakalim. 5. In inside-out membrane patches, in the absence of intracellular ATP, intracellular application of Mg2+ decreased channel activity and single channel current amplitude. Inhibition of K+ATP channels by ATP was also reduced. 6. In the absence of intracellular ATP, a decrease in intracellular pH induced a reduction in channel activity and single channel current amplitude. Inhibition of K+ATP channels by ATP was also reduced. 7. The reduction of single channel current amplitude during poisoning was attributed to an increase in intracellular Mg2+ concentration caused by a fall in intracellular ATP concentration. These results also show that metabolic poisoning causes direct activation of K+ATP channels in skeletal muscle, and that is activation is at least partially mediated through an increase in intracellular Mg(2+) concentration and a decrease in intracellular pH.
Full text
PDF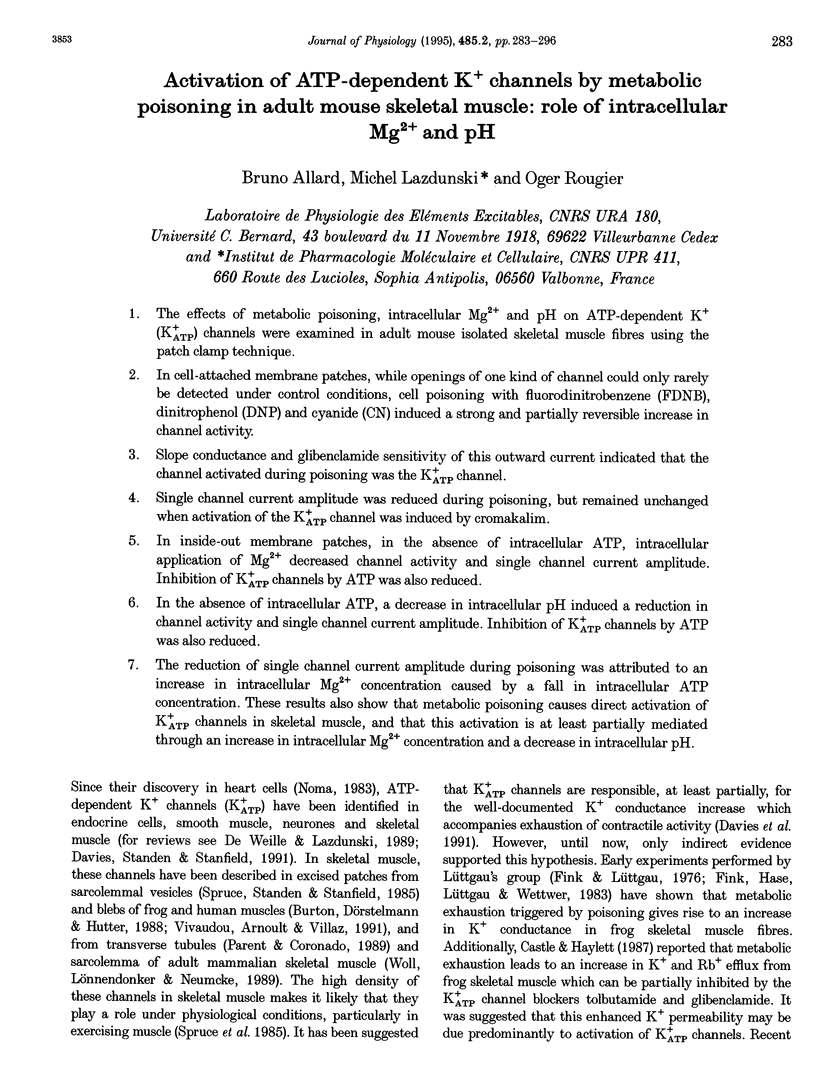
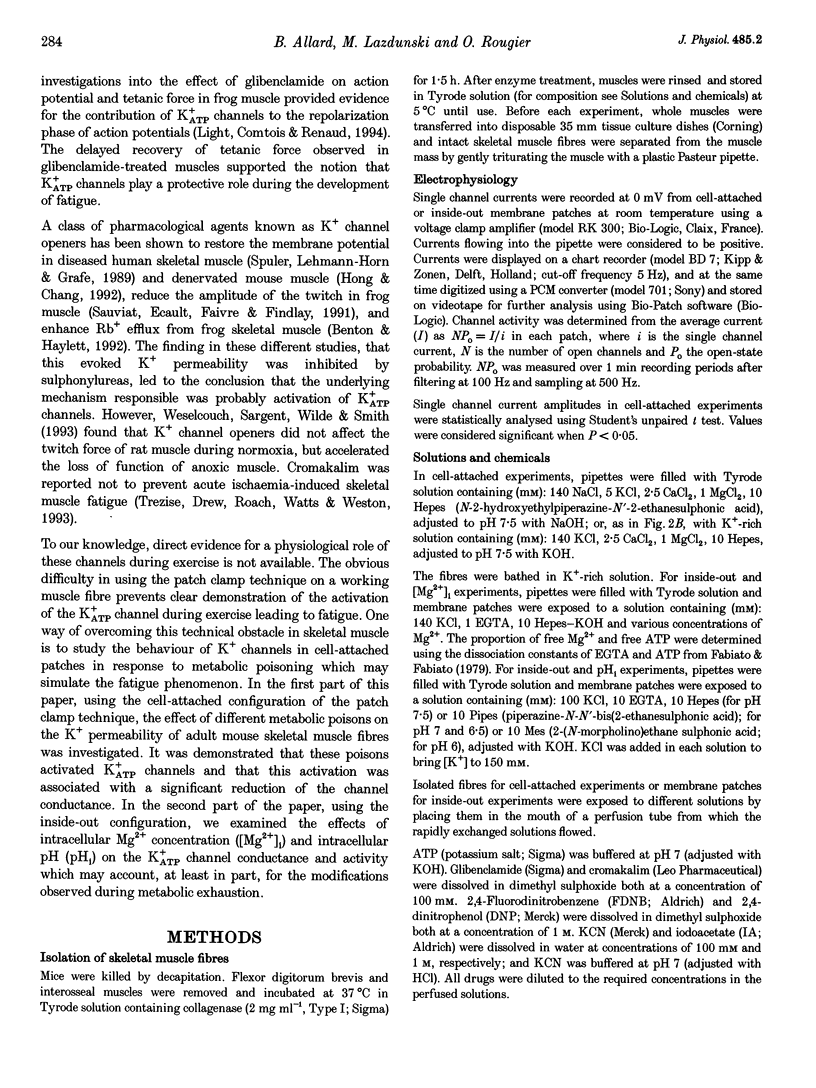
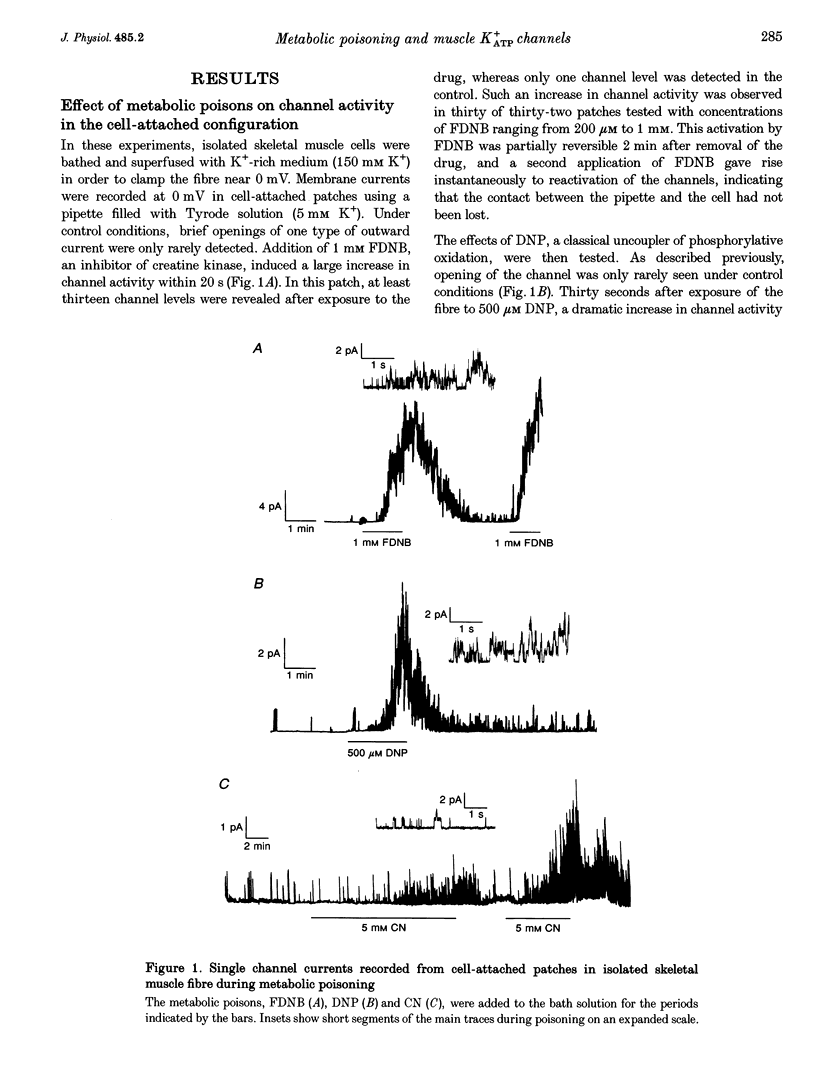
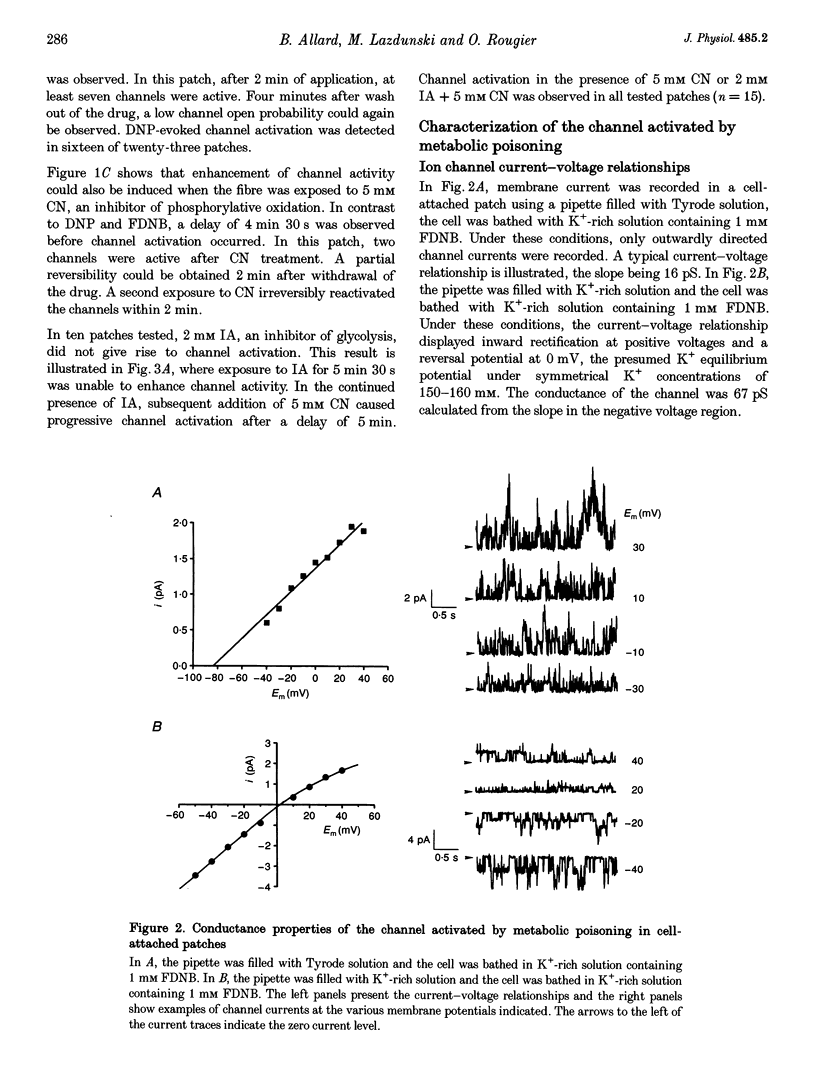
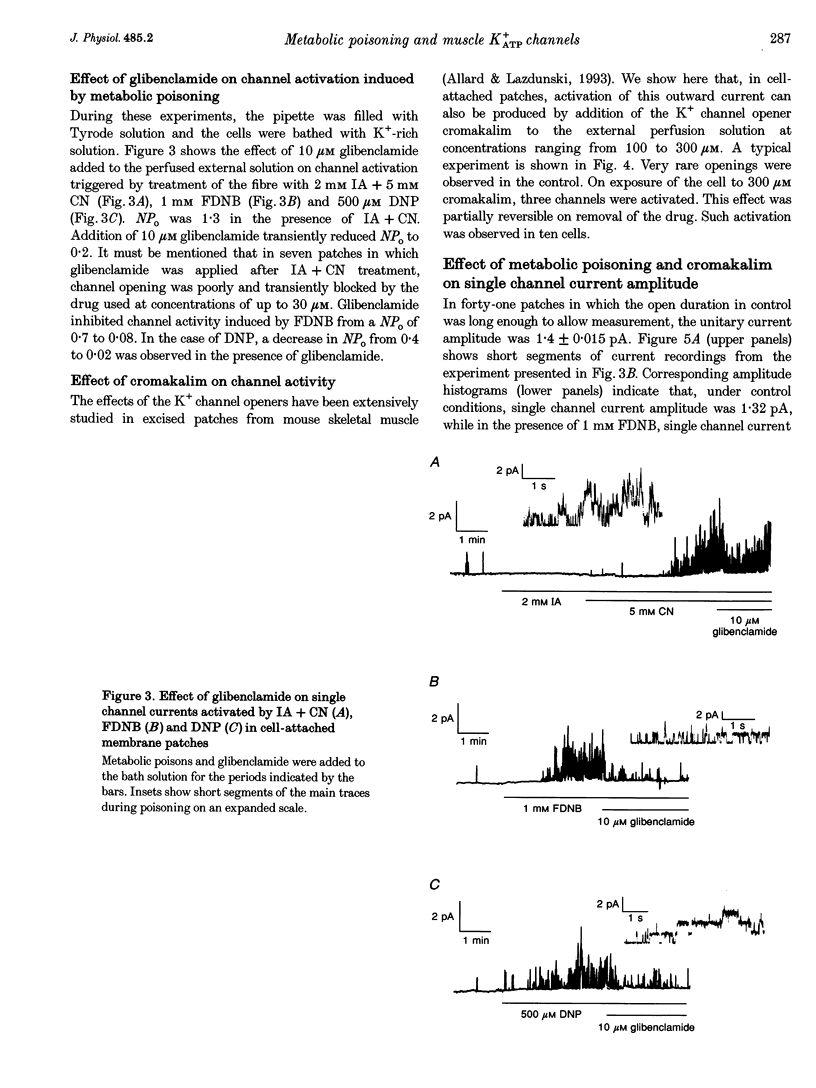
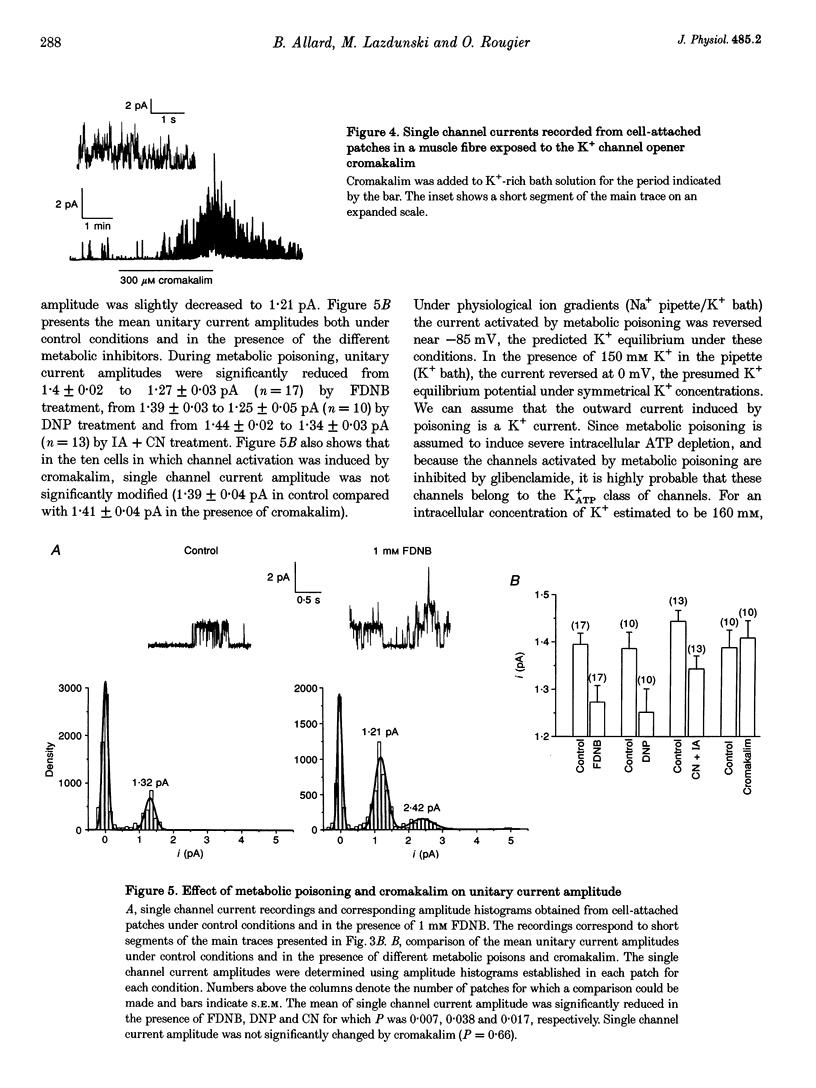
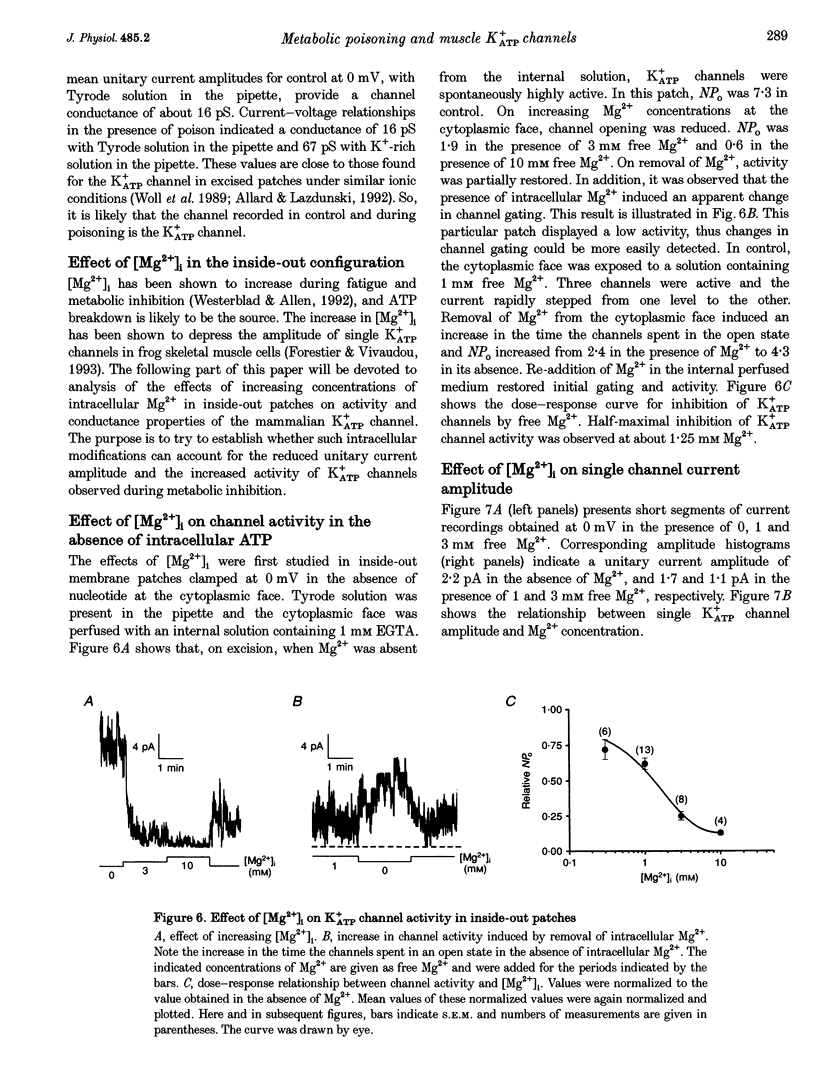
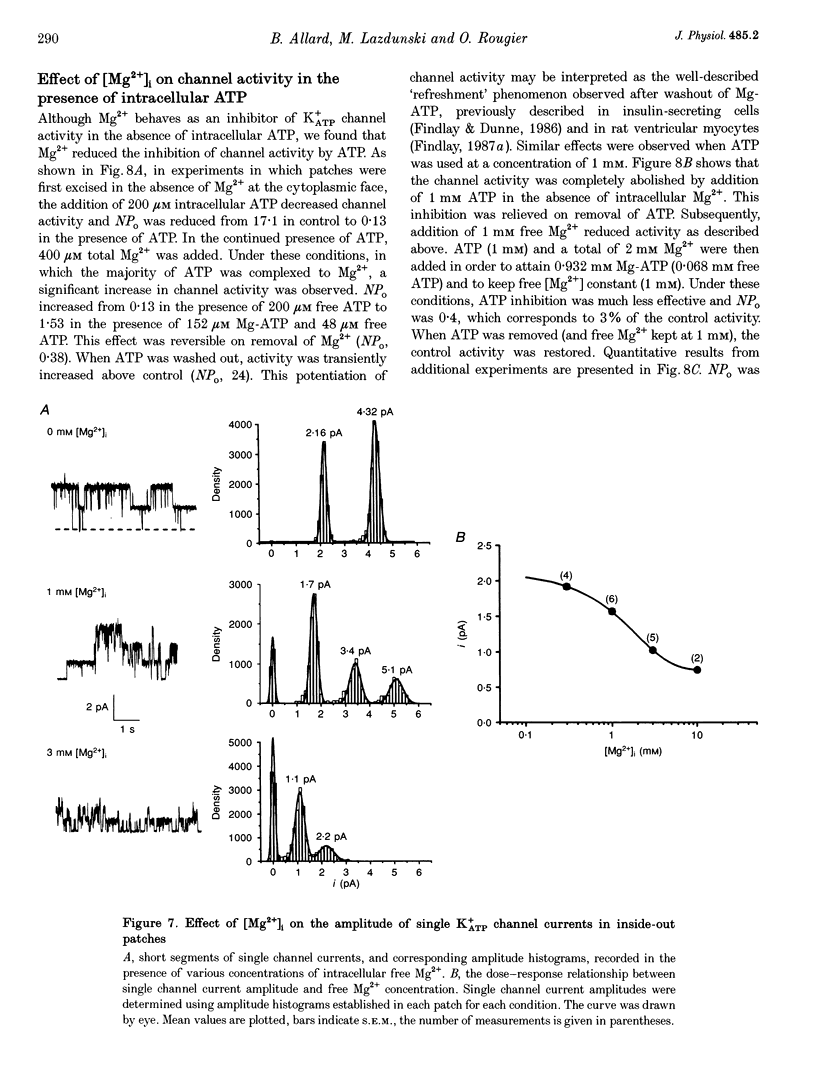
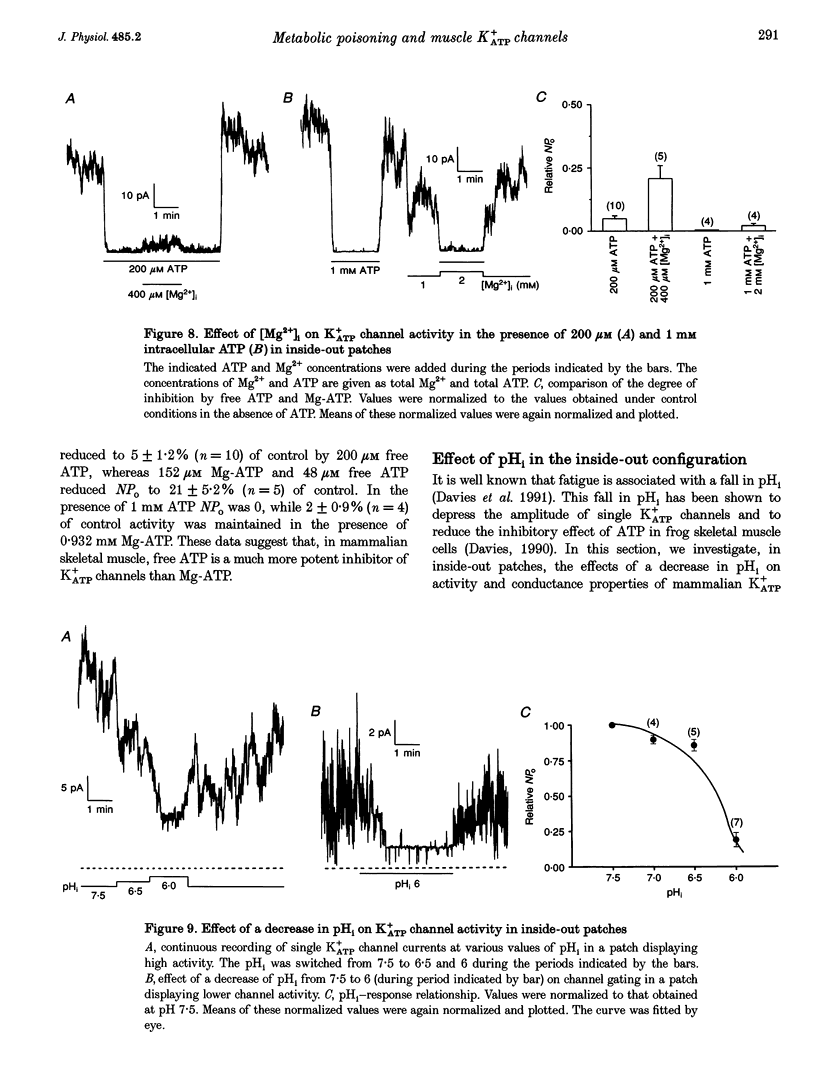
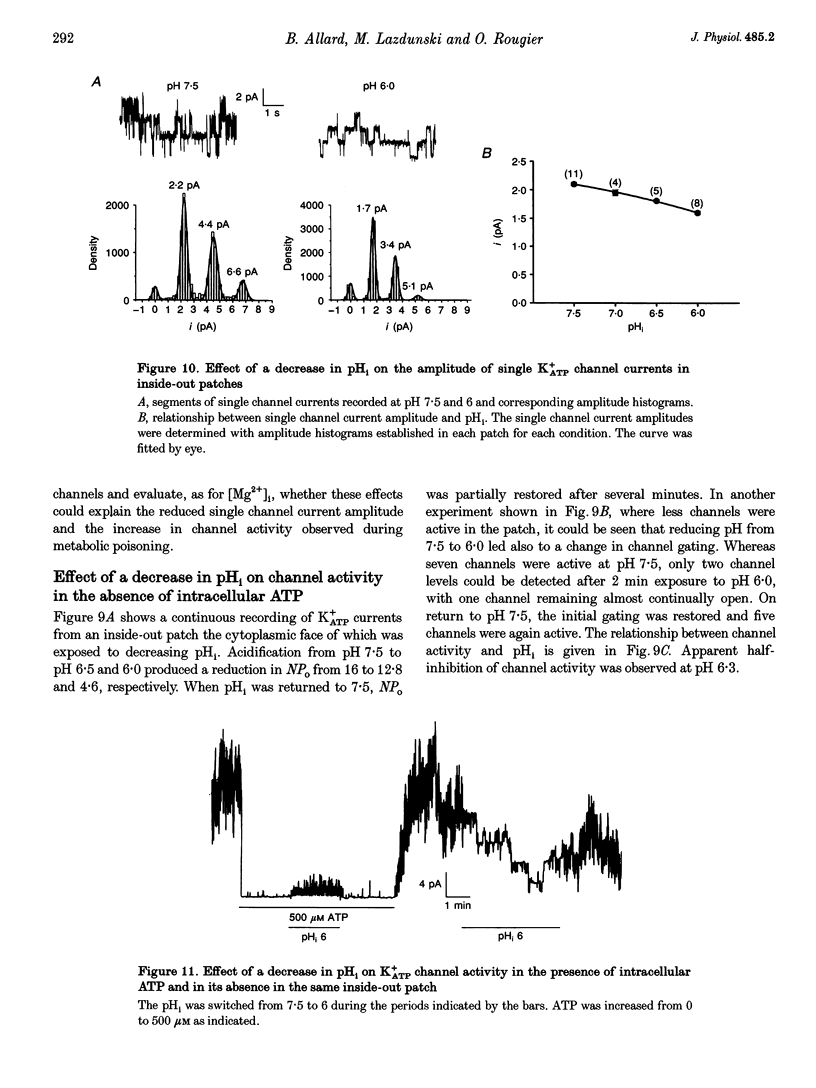
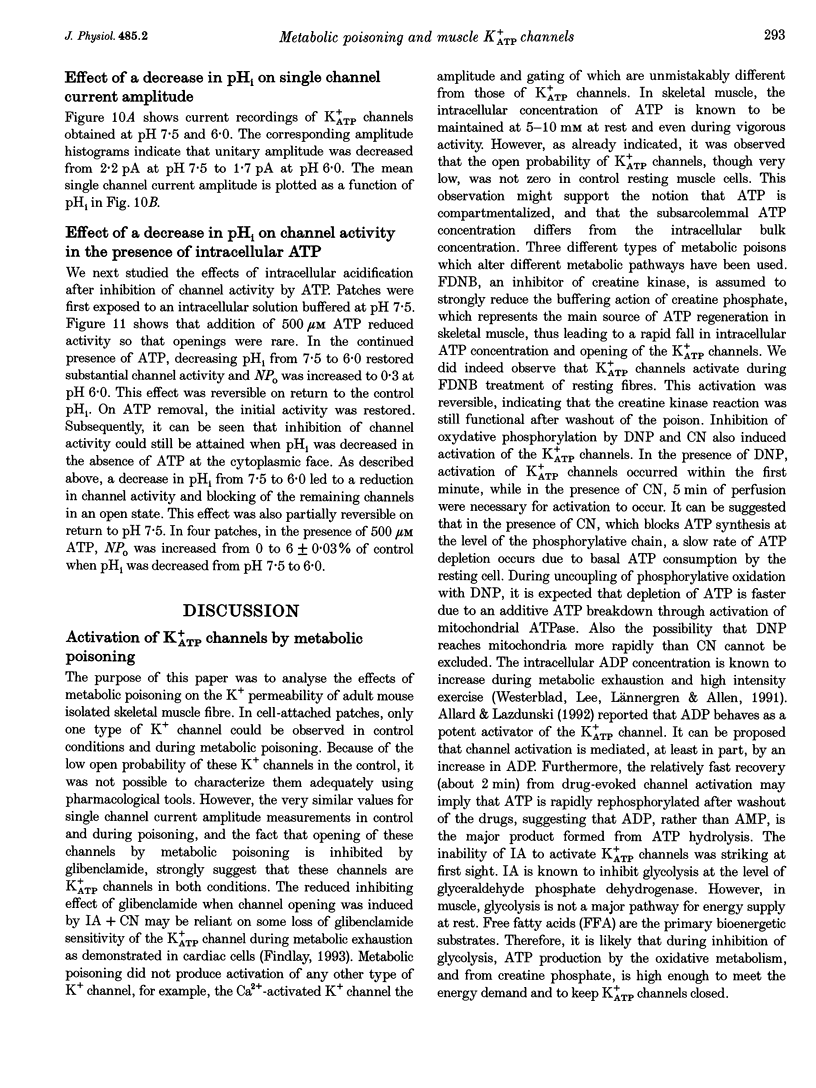
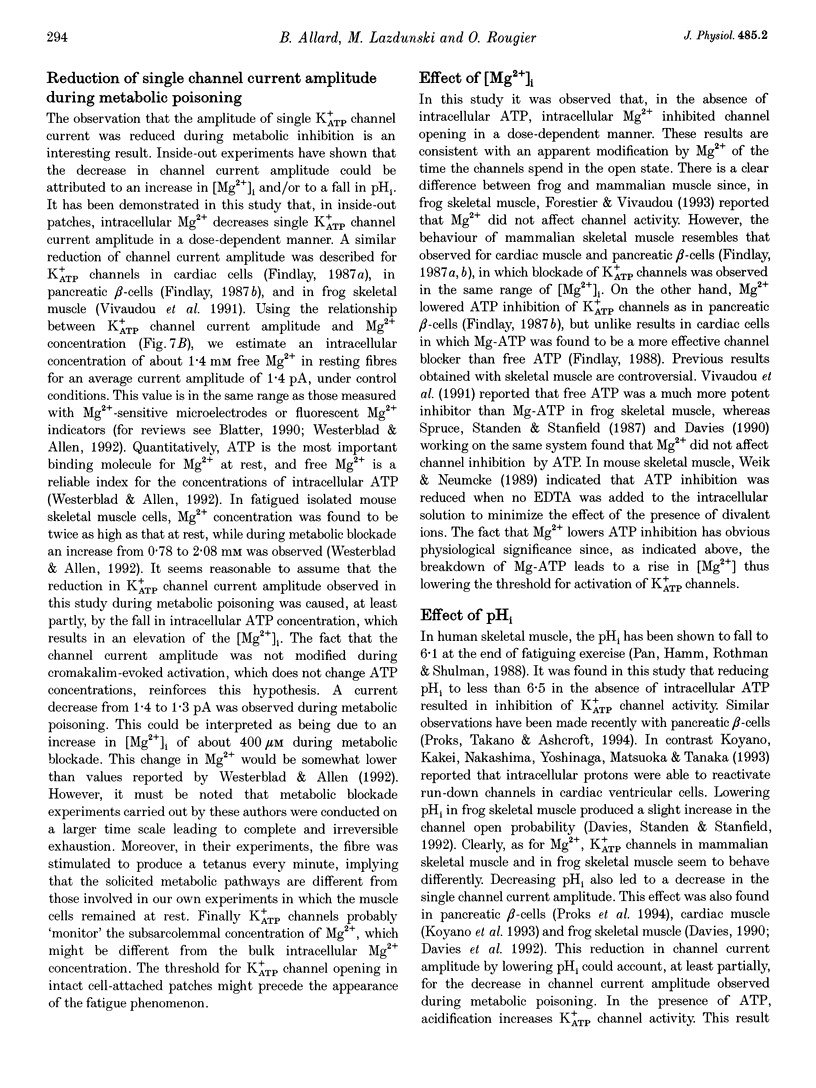
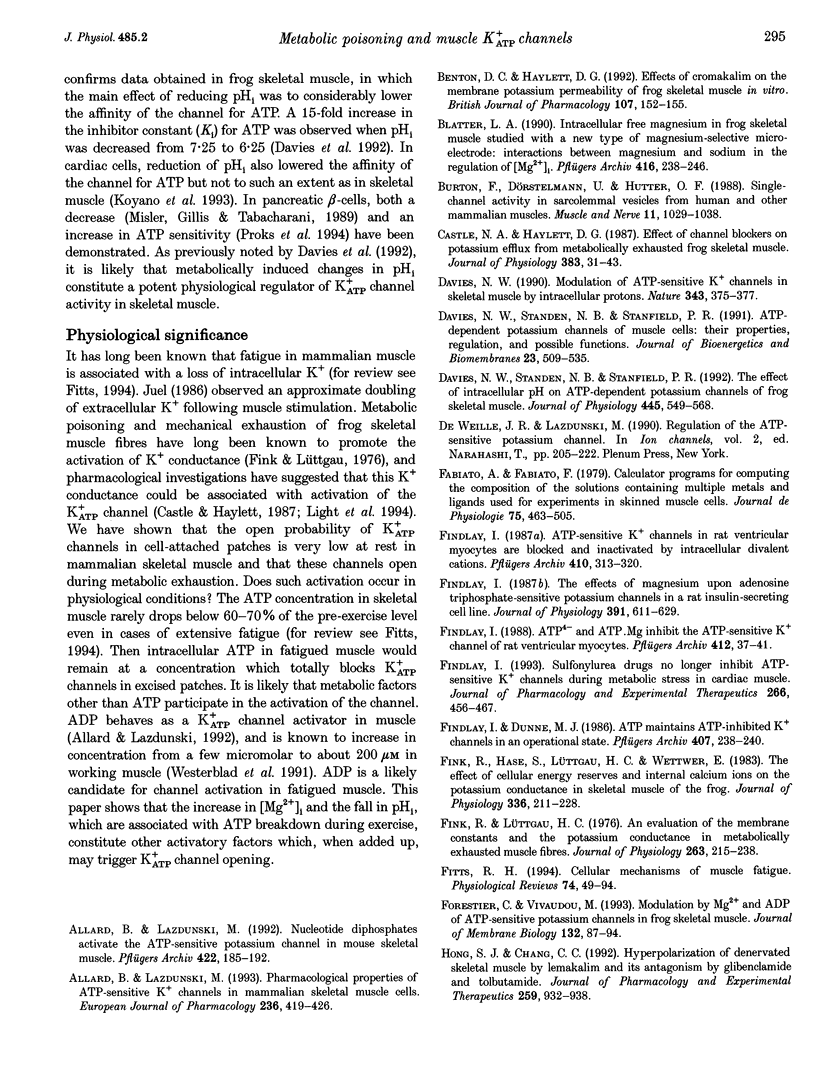
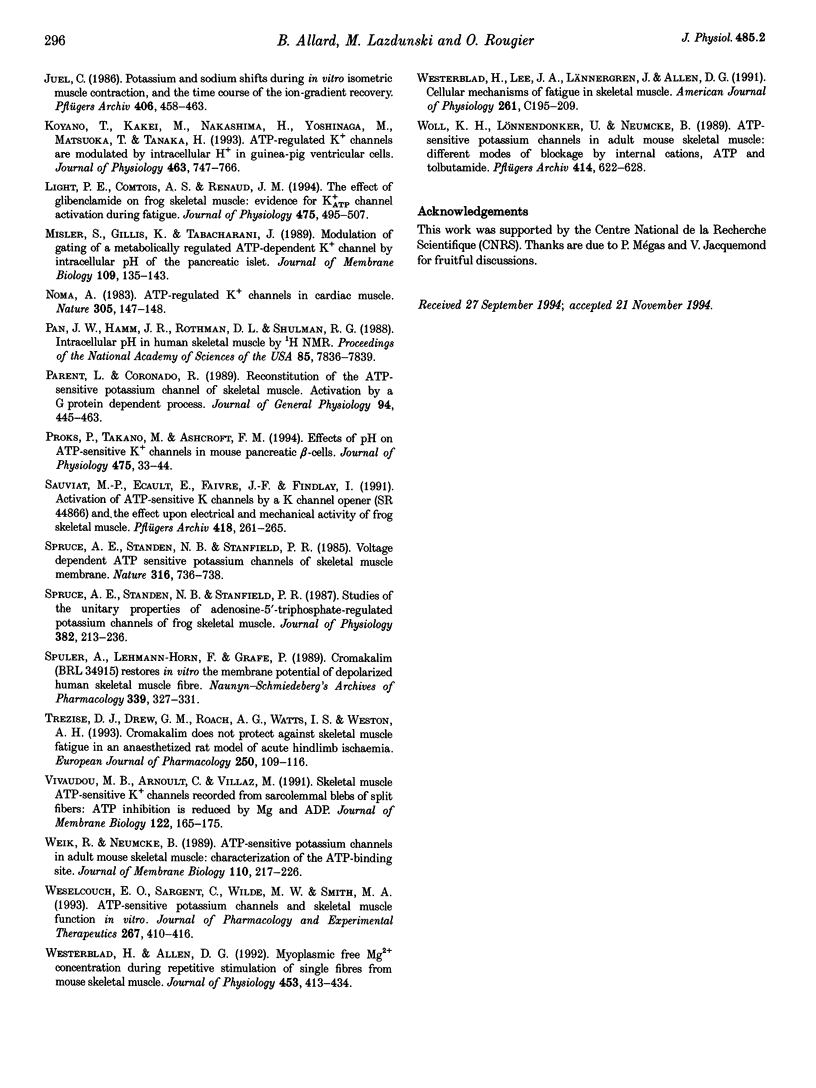
Selected References
These references are in PubMed. This may not be the complete list of references from this article.
- Allard B., Lazdunski M. Nucleotide diphosphates activate the ATP-sensitive potassium channel in mouse skeletal muscle. Pflugers Arch. 1992 Nov;422(2):185–192. doi: 10.1007/BF00370419. [DOI] [PubMed] [Google Scholar]
- Allard B., Lazdunski M. Pharmacological properties of ATP-sensitive K+ channels in mammalian skeletal muscle cells. Eur J Pharmacol. 1993 Jun 4;236(3):419–426. doi: 10.1016/0014-2999(93)90480-6. [DOI] [PubMed] [Google Scholar]
- Benton D. C., Haylett D. G. Effects of cromakalim on the membrane potassium permeability of frog skeletal muscle in vitro. Br J Pharmacol. 1992 Sep;107(1):152–155. doi: 10.1111/j.1476-5381.1992.tb14478.x. [DOI] [PMC free article] [PubMed] [Google Scholar]
- Burton F., Dörstelmann U., Hutter O. F. Single-channel activity in sarcolemmal vesicles from human and other mammalian muscles. Muscle Nerve. 1988 Oct;11(10):1029–1038. doi: 10.1002/mus.880111004. [DOI] [PubMed] [Google Scholar]
- Castle N. A., Haylett D. G. Effect of channel blockers on potassium efflux from metabolically exhausted frog skeletal muscle. J Physiol. 1987 Feb;383:31–43. doi: 10.1113/jphysiol.1987.sp016394. [DOI] [PMC free article] [PubMed] [Google Scholar]
- Davies N. W. Modulation of ATP-sensitive K+ channels in skeletal muscle by intracellular protons. Nature. 1990 Jan 25;343(6256):375–377. doi: 10.1038/343375a0. [DOI] [PubMed] [Google Scholar]
- Davies N. W., Standen N. B., Stanfield P. R. The effect of intracellular pH on ATP-dependent potassium channels of frog skeletal muscle. J Physiol. 1992 Jan;445:549–568. doi: 10.1113/jphysiol.1992.sp018939. [DOI] [PMC free article] [PubMed] [Google Scholar]
- Davis N. W., Standen N. B., Stanfield P. R. ATP-dependent potassium channels of muscle cells: their properties, regulation, and possible functions. J Bioenerg Biomembr. 1991 Aug;23(4):509–535. doi: 10.1007/BF00785809. [DOI] [PubMed] [Google Scholar]
- Fabiato A., Fabiato F. Calculator programs for computing the composition of the solutions containing multiple metals and ligands used for experiments in skinned muscle cells. J Physiol (Paris) 1979;75(5):463–505. [PubMed] [Google Scholar]
- Findlay I. ATP-sensitive K+ channels in rat ventricular myocytes are blocked and inactivated by internal divalent cations. Pflugers Arch. 1987 Oct;410(3):313–320. doi: 10.1007/BF00580282. [DOI] [PubMed] [Google Scholar]
- Findlay I. ATP4- and ATP.Mg inhibit the ATP-sensitive K+ channel of rat ventricular myocytes. Pflugers Arch. 1988 Jul;412(1-2):37–41. doi: 10.1007/BF00583729. [DOI] [PubMed] [Google Scholar]
- Findlay I., Dunne M. J. ATP maintains ATP-inhibited K+ channels in an operational state. Pflugers Arch. 1986 Aug;407(2):238–240. doi: 10.1007/BF00580683. [DOI] [PubMed] [Google Scholar]
- Findlay I. Sulphonylurea drugs no longer inhibit ATP-sensitive K+ channels during metabolic stress in cardiac muscle. J Pharmacol Exp Ther. 1993 Jul;266(1):456–467. [PubMed] [Google Scholar]
- Findlay I. The effects of magnesium upon adenosine triphosphate-sensitive potassium channels in a rat insulin-secreting cell line. J Physiol. 1987 Oct;391:611–629. doi: 10.1113/jphysiol.1987.sp016759. [DOI] [PMC free article] [PubMed] [Google Scholar]
- Fink R., Hase S., Lüttgau H. C., Wettwer E. The effect of cellular energy reserves and internal calcium ions on the potassium conductance in skeletal muscle of the frog. J Physiol. 1983 Mar;336:211–228. doi: 10.1113/jphysiol.1983.sp014577. [DOI] [PMC free article] [PubMed] [Google Scholar]
- Fink R., Lüttgau H. C. An evaluation of the membrane constants and the potassium conductance in metabolically exhausted muscle fibres. J Physiol. 1976 Dec;263(2):215–238. doi: 10.1113/jphysiol.1976.sp011629. [DOI] [PMC free article] [PubMed] [Google Scholar]
- Fitts R. H. Cellular mechanisms of muscle fatigue. Physiol Rev. 1994 Jan;74(1):49–94. doi: 10.1152/physrev.1994.74.1.49. [DOI] [PubMed] [Google Scholar]
- Forestier C., Vivaudou M. Modulation by Mg2+ and ADP of ATP-sensitive potassium channels in frog skeletal muscle. J Membr Biol. 1993 Feb;132(1):87–94. doi: 10.1007/BF00233054. [DOI] [PubMed] [Google Scholar]
- Hong S. J., Chang C. C. Hyperpolarization of denervated skeletal muscle by lemakalim and its antagonism by glybenclamide and tolbutamide. J Pharmacol Exp Ther. 1991 Nov;259(2):932–938. [PubMed] [Google Scholar]
- Juel C. Potassium and sodium shifts during in vitro isometric muscle contraction, and the time course of the ion-gradient recovery. Pflugers Arch. 1986 May;406(5):458–463. doi: 10.1007/BF00583367. [DOI] [PubMed] [Google Scholar]
- Koyano T., Kakei M., Nakashima H., Yoshinaga M., Matsuoka T., Tanaka H. ATP-regulated K+ channels are modulated by intracellular H+ in guinea-pig ventricular cells. J Physiol. 1993 Apr;463:747–766. doi: 10.1113/jphysiol.1993.sp019620. [DOI] [PMC free article] [PubMed] [Google Scholar]
- Light P. E., Comtois A. S., Renaud J. M. The effect of glibenclamide on frog skeletal muscle: evidence for K+ATP channel activation during fatigue. J Physiol. 1994 Mar 15;475(3):495–507. doi: 10.1113/jphysiol.1994.sp020088. [DOI] [PMC free article] [PubMed] [Google Scholar]
- Misler S., Gillis K., Tabcharani J. Modulation of gating of a metabolically regulated, ATP-dependent K+ channel by intracellular pH in B cells of the pancreatic islet. J Membr Biol. 1989 Jul;109(2):135–143. doi: 10.1007/BF01870852. [DOI] [PubMed] [Google Scholar]
- Noma A. ATP-regulated K+ channels in cardiac muscle. Nature. 1983 Sep 8;305(5930):147–148. doi: 10.1038/305147a0. [DOI] [PubMed] [Google Scholar]
- Pan J. W., Hamm J. R., Rothman D. L., Shulman R. G. Intracellular pH in human skeletal muscle by 1H NMR. Proc Natl Acad Sci U S A. 1988 Nov;85(21):7836–7839. doi: 10.1073/pnas.85.21.7836. [DOI] [PMC free article] [PubMed] [Google Scholar]
- Proks P., Takano M., Ashcroft F. M. Effects of intracellular pH on ATP-sensitive K+ channels in mouse pancreatic beta-cells. J Physiol. 1994 Feb 15;475(1):33–44. doi: 10.1113/jphysiol.1994.sp020047. [DOI] [PMC free article] [PubMed] [Google Scholar]
- Sauviat M. P., Ecault E., Faivre J. F., Findlay I. Activation of ATP-sensitive K channels by a K channel opener (SR 44866) and the effect upon electrical and mechanical activity of frog skeletal muscle. Pflugers Arch. 1991 Apr;418(3):261–265. doi: 10.1007/BF00370524. [DOI] [PubMed] [Google Scholar]
- Spruce A. E., Standen N. B., Stanfield P. R. Studies of the unitary properties of adenosine-5'-triphosphate-regulated potassium channels of frog skeletal muscle. J Physiol. 1987 Jan;382:213–236. doi: 10.1113/jphysiol.1987.sp016364. [DOI] [PMC free article] [PubMed] [Google Scholar]
- Spruce A. E., Standen N. B., Stanfield P. R. Voltage-dependent ATP-sensitive potassium channels of skeletal muscle membrane. Nature. 1985 Aug 22;316(6030):736–738. doi: 10.1038/316736a0. [DOI] [PubMed] [Google Scholar]
- Spuler A., Lehmann-Horn F., Grafe P. Cromakalim (BRL 34915) restores in vitro the membrane potential of depolarized human skeletal muscle fibres. Naunyn Schmiedebergs Arch Pharmacol. 1989 Mar;339(3):327–331. doi: 10.1007/BF00173587. [DOI] [PubMed] [Google Scholar]
- Trezise D. J., Drew G. M., Roach A. G., Watts I. S., Weston A. H. Cromakalim does not protect against skeletal muscle fatigue in an anaesthetized rat model of acute hindlimb ischaemia. Eur J Pharmacol. 1993 Nov 30;250(1):109–116. doi: 10.1016/0014-2999(93)90627-t. [DOI] [PubMed] [Google Scholar]
- Vivaudou M. B., Arnoult C., Villaz M. Skeletal muscle ATP-sensitive K+ channels recorded from sarcolemmal blebs of split fibers: ATP inhibition is reduced by magnesium and ADP. J Membr Biol. 1991 Jun;122(2):165–175. doi: 10.1007/BF01872639. [DOI] [PubMed] [Google Scholar]
- Weik R., Neumcke B. ATP-sensitive potassium channels in adult mouse skeletal muscle: characterization of the ATP-binding site. J Membr Biol. 1989 Sep;110(3):217–226. doi: 10.1007/BF01869152. [DOI] [PubMed] [Google Scholar]
- Weselcouch E. O., Sargent C., Wilde M. W., Smith M. A. ATP-sensitive potassium channels and skeletal muscle function in vitro. J Pharmacol Exp Ther. 1993 Oct;267(1):410–416. [PubMed] [Google Scholar]
- Westerblad H., Allen D. G. Myoplasmic free Mg2+ concentration during repetitive stimulation of single fibres from mouse skeletal muscle. J Physiol. 1992;453:413–434. doi: 10.1113/jphysiol.1992.sp019236. [DOI] [PMC free article] [PubMed] [Google Scholar]
- Westerblad H., Lee J. A., Lännergren J., Allen D. G. Cellular mechanisms of fatigue in skeletal muscle. Am J Physiol. 1991 Aug;261(2 Pt 1):C195–C209. doi: 10.1152/ajpcell.1991.261.2.C195. [DOI] [PubMed] [Google Scholar]
- Woll K. H., Lönnendonker U., Neumcke B. ATP-sensitive potassium channels in adult mouse skeletal muscle: different modes of blockage by internal cations, ATP and tolbutamide. Pflugers Arch. 1989 Sep;414(6):622–628. doi: 10.1007/BF00582126. [DOI] [PubMed] [Google Scholar]
- de Weille J. R., Lazdunski M. Regulation of the ATP-sensitive potassium channel. Ion Channels. 1990;2:205–222. doi: 10.1007/978-1-4615-7305-0_6. [DOI] [PubMed] [Google Scholar]