Abstract
1. The kinetic properties of the internal Na+ (Na+i)- dependent 45Ca2+ influx and external Na+ (Na+o)-dependent 45Ca2+ efflux were determined in isolated rat brain nerve terminals (synaptosomes) under conditions which the concentrations of internal Na+ ([Na+]i), external Na+ ([Na+]o), external Ca2+ (Ca2+]o), and external K+ ([K+]o) were varied. Both fluxes are manifestations of Na(+)-Ca2+ exchange. 2. Ca2+ uptake was augmented by raising [Na+]i and / or lowering [Na+]o. The increase in Ca2+ uptake induced by removing external Na+ was, in most instances, quantitatively equal to the Na+i-dependent Ca2+ uptake. 3. The Na+i-dependent Ca2+ uptake (measured at 1 s) was activated with an apparent half-maximal [Ca2+]o (KCa(o)) of about 0.23 mM. External Na+ inhibited the uptake in a non- competitive manner: increasing [Na+]o from 4.7 to 96 mM reduced the maximal Na+(i)-dependent Ca2+ uptake but did not affect KCa(o). 4. The inhibition of Ca2+ uptake by Na+o was proportional to ([Na+]o)2, and had a Hill coefficient (nH) of approximately 2.0. The mean apparent half-maximal [Na+]o for inhibition (KI(Na)) was about 60mM, and was independent of [Ca2+]o between 0.1 and 1.2mM; this, too, is indicative of non-competitive inhibition. 5. Low concentrations of alkali metal ions (M+) in the medium, including Na+, stimulated the Na+i-dependent uptake. The external Na+ and K+ concentrations required for apparent half-maximal activation (KM(Na) and KM(K), respectively) were 0.12 and 0.10mM. Thus, the relationship between Ca2+ uptake and [Na+]o was biphasic: uptake was stimulated by [Na+]o < or = 10 mM, and inhibited by higher [Na+]o. 6. The calculated maximal Na+i-dependent Ca2+ uptake (Jmax) was about 1530 pmol (mg protein) -1s-1 at 30 degrees C saturating [Ca2+]o and external M+ concentration ([M+]o), and with negligible inhibition by external Na+. 7. Internal Na+ activated the Ca2+ uptake with an apparent half-maximal concentration (KNa(i)) of about 20 mM and a Hill coefficient, nH, of approximately 3.0. 8. The Jmax for the Na+o-dependent efflux of Ca2+ from 45Ca(2+)-loaded synaptosomes treated with carbonyl cyanide p-trifluormethoxy-phenylhydrazone (FCCP) and caffeine (to release stored Ca2+ and raise the internal Ca2+ concentration ([Ca2+]i) was about 1800-2000 pmol (mg protein -1s-1 at 37 degrees C. 9. When the membrane potential (Vm) was reduced (depolarized) by increasing [K+]o, the Na+i-dependent Ca2+ influx increased, and the Na+o-dependent Ca2+ efflux declined. Both fluxes changed about 2-fold per 60 mV change in Vm. This voltage sensitivity corresponds to the movement of one elementary charge through about 60% of the membrane electric field. The symmetry suggests that the voltage-sensitive step is reversible. 10. The Jmax values for both Ca2P influx and efflux correspond to a Na+-Ca2+ exchange-mediated flux of about 425-575 jumol Ca2P (1 cell water)-' s-' or a turnover of about one quarter of the total synaptosome Ca2P in 1 s. We conclude that the Na+-Ca2P exchanger may contribute to Ca2P entry during nerve terminal depolarization; it is likely to be a major mechanism mediating Ca2P extrusion during subsequent repolarization and recovery.
Full text
PDF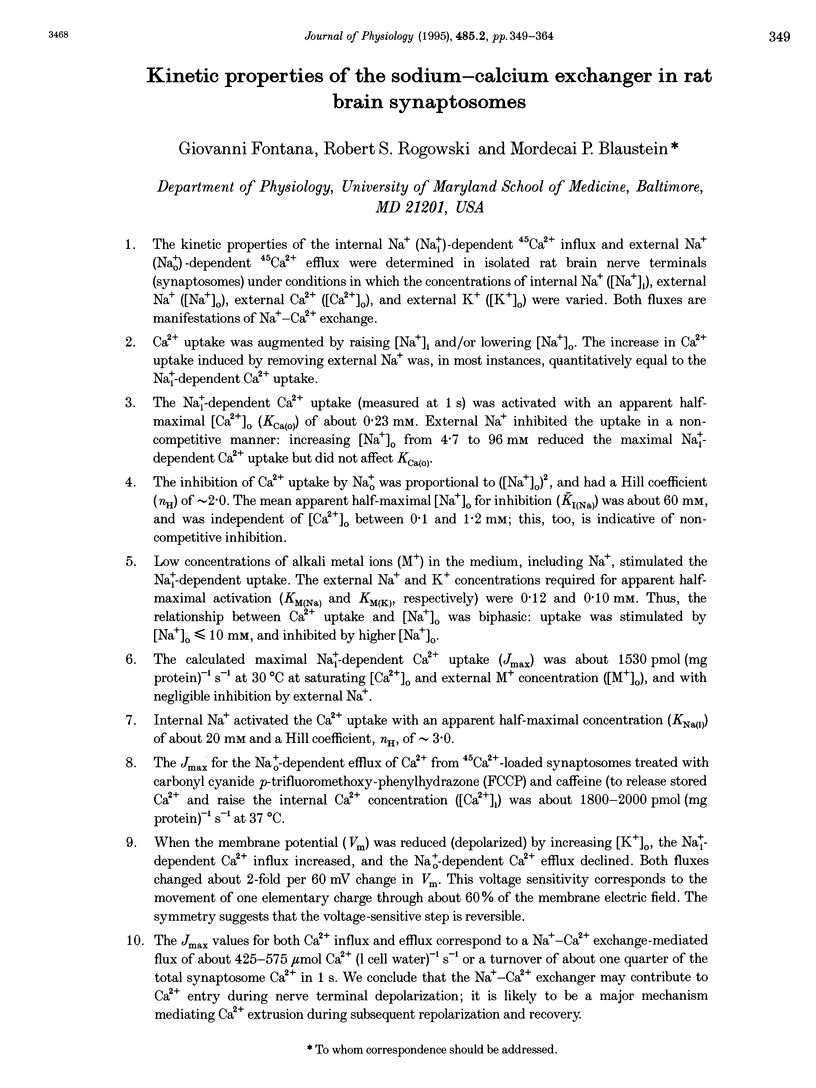
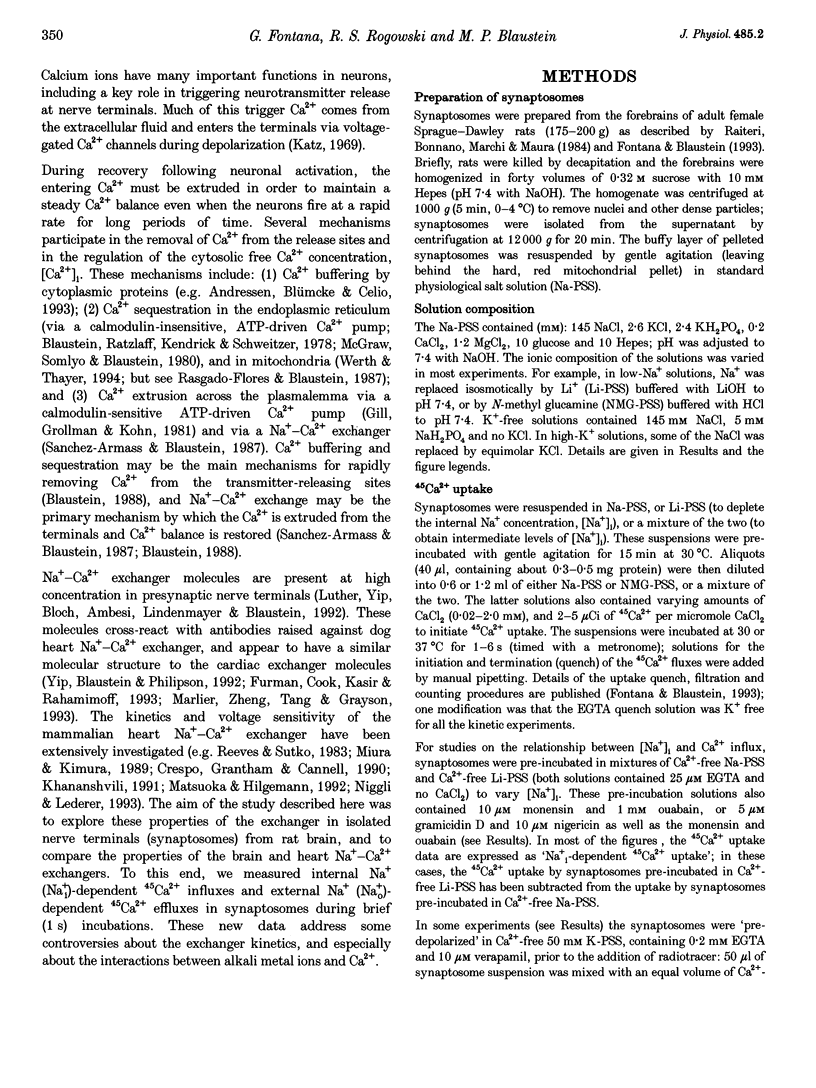
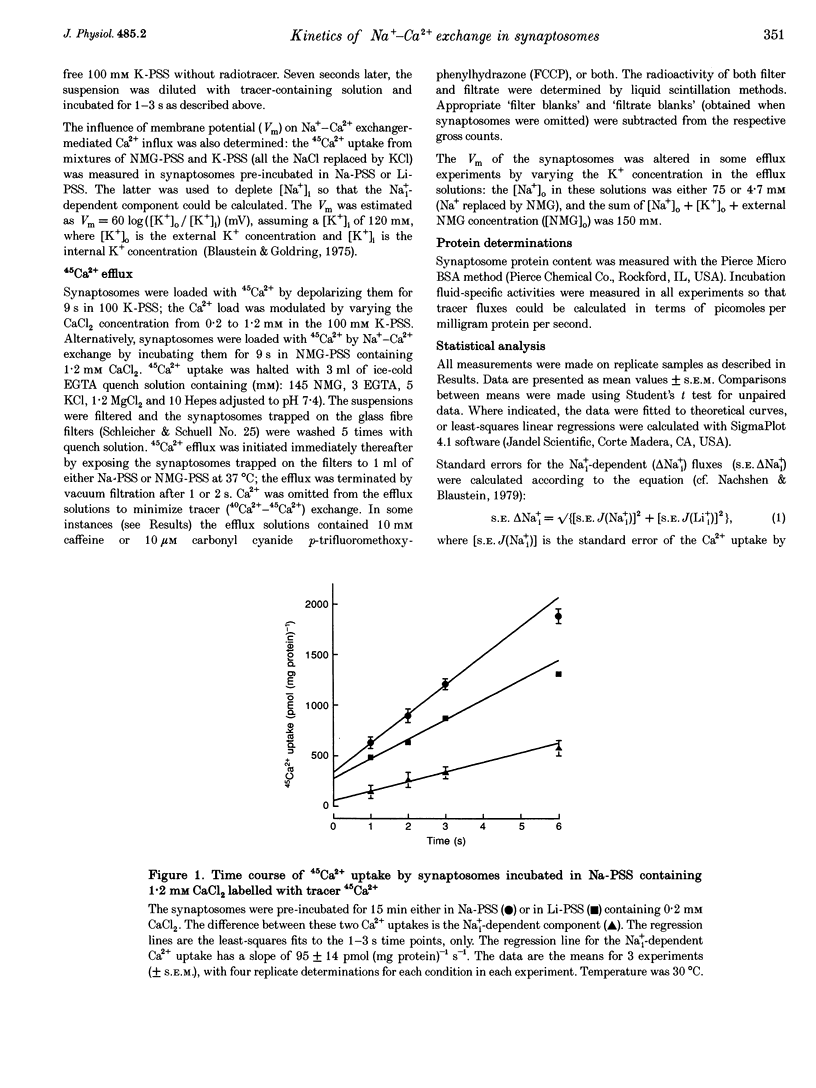
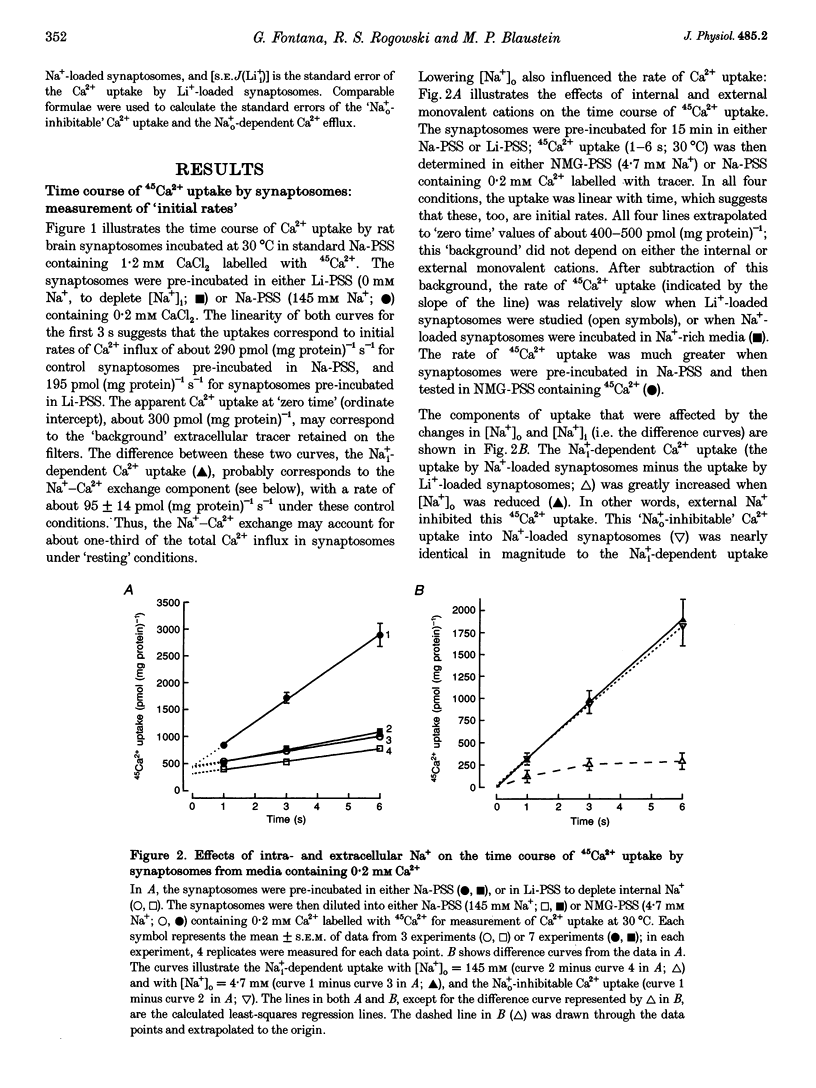
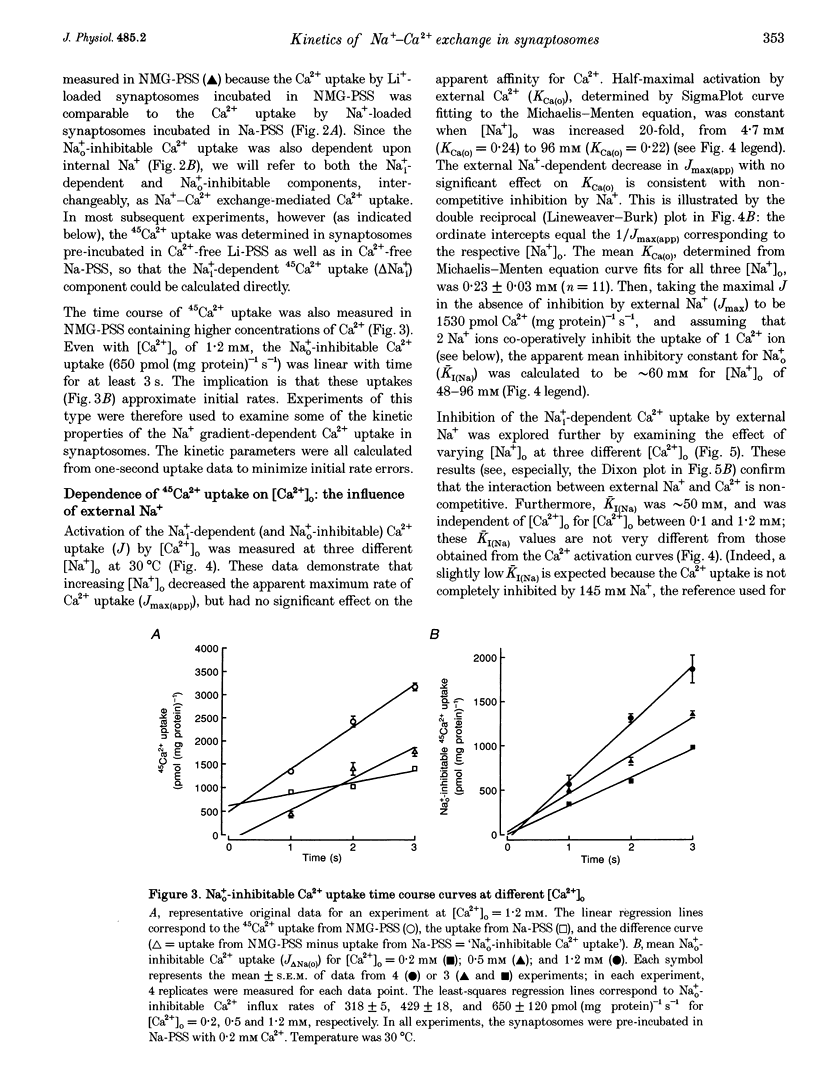
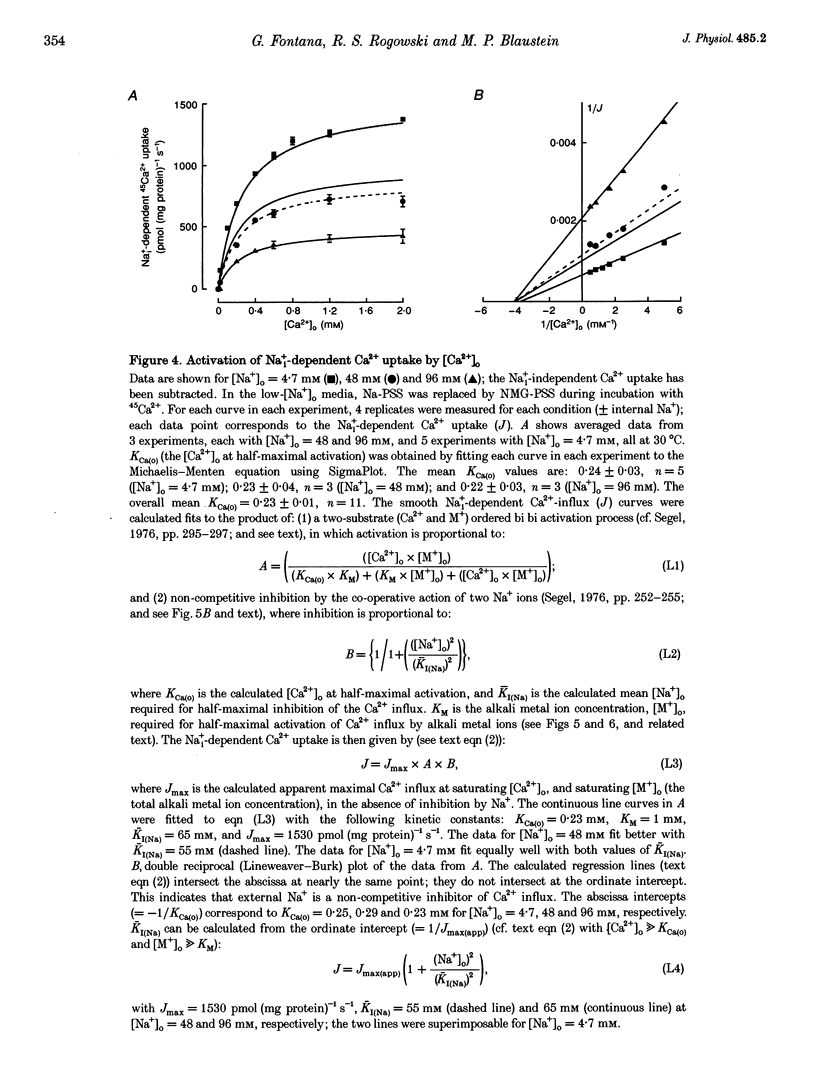
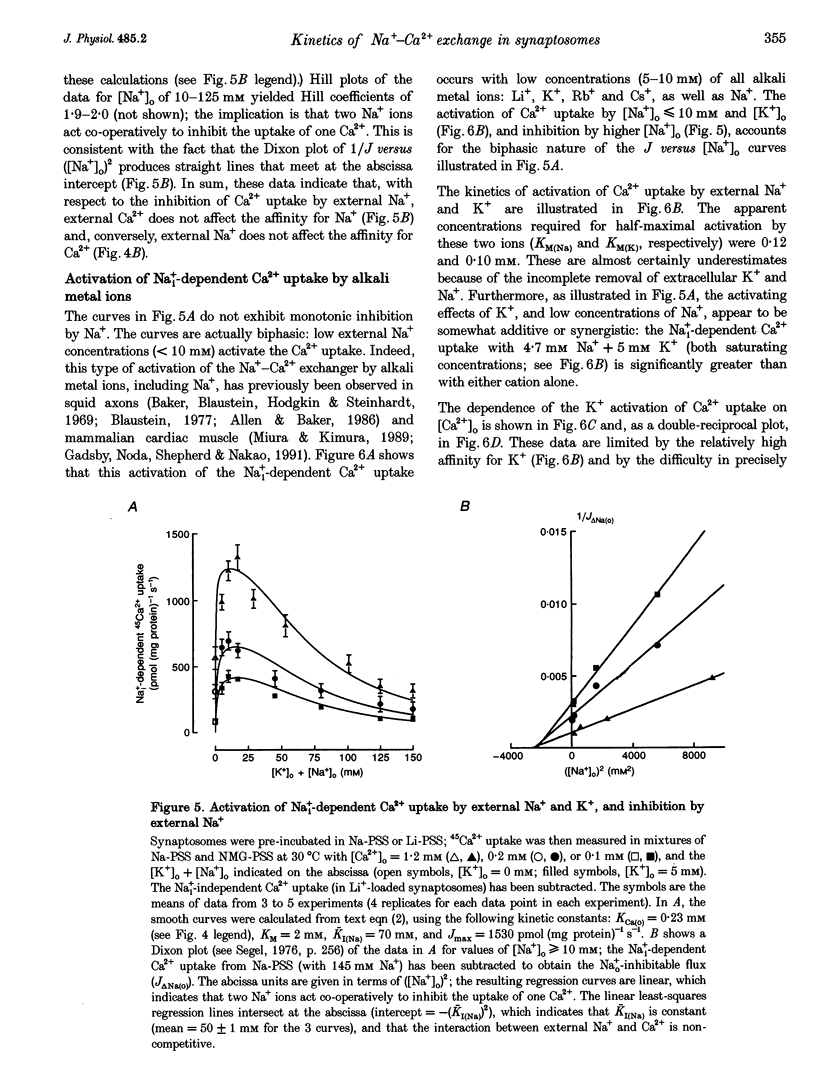
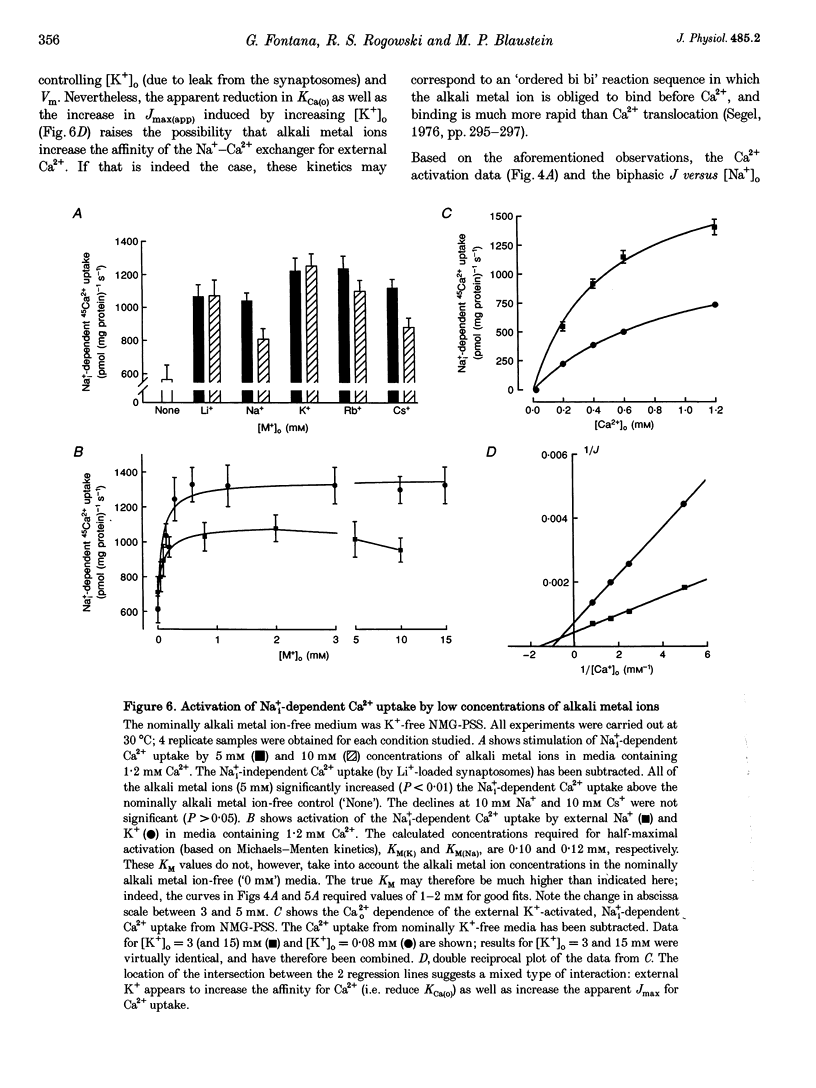
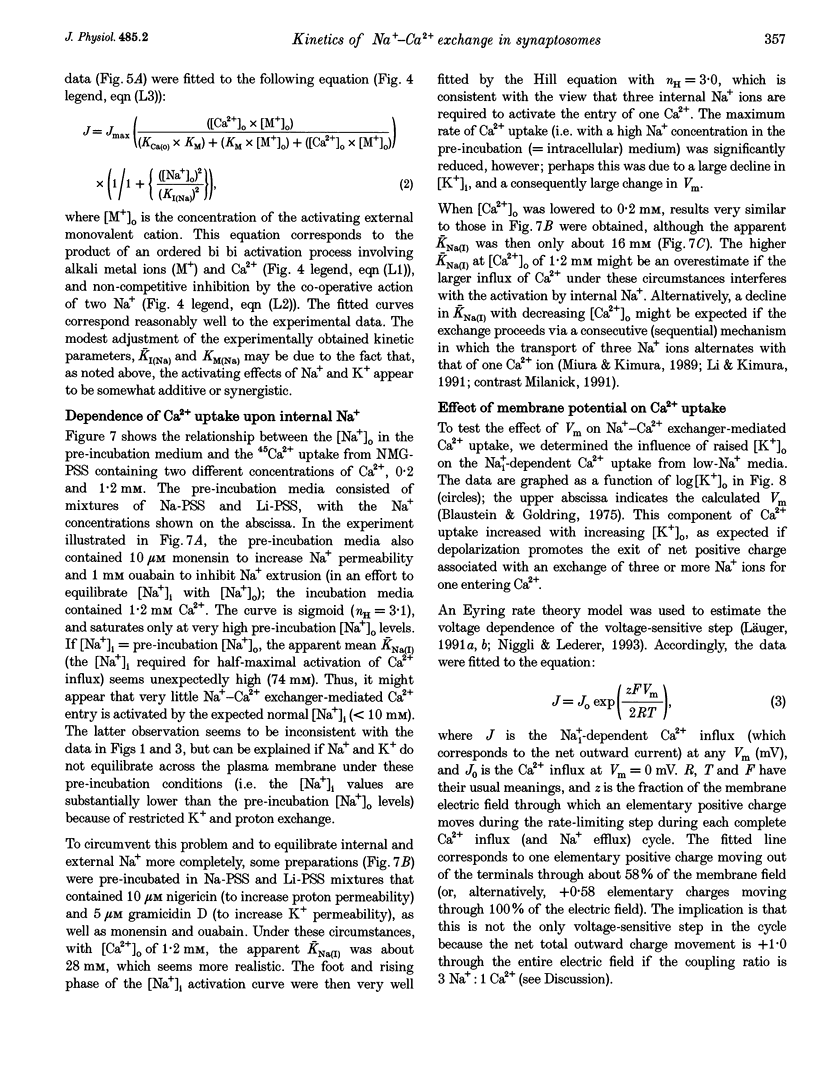
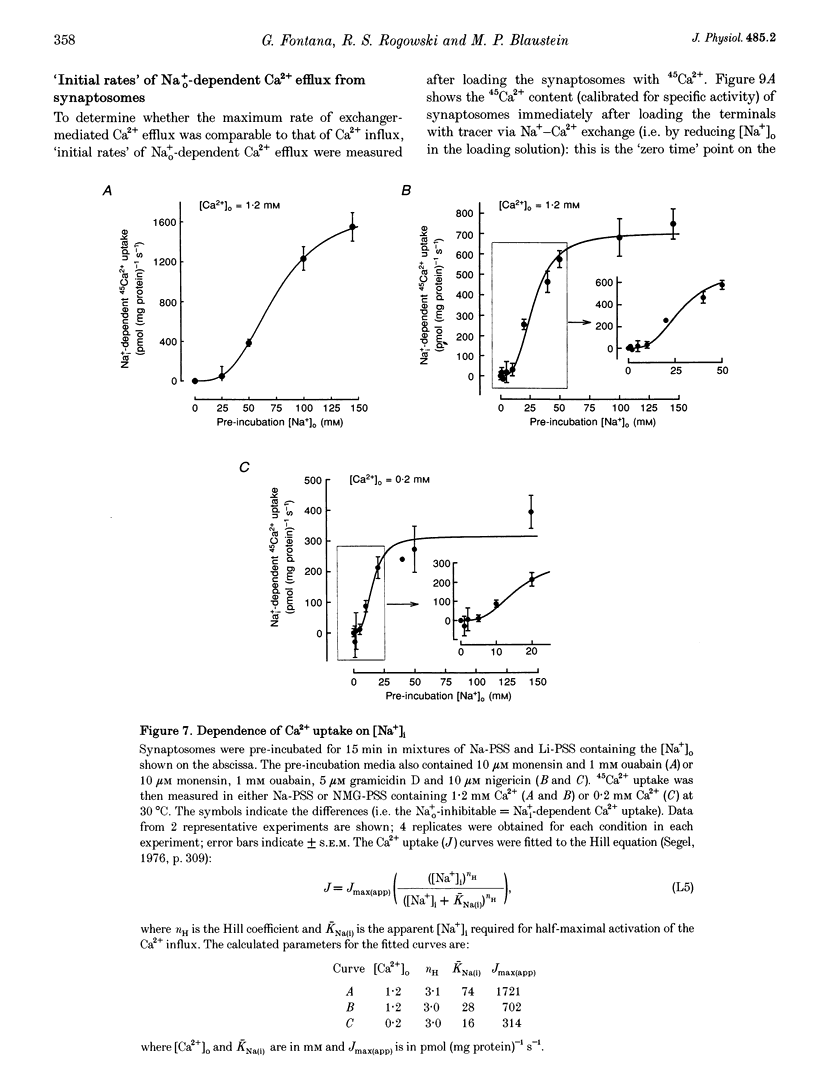
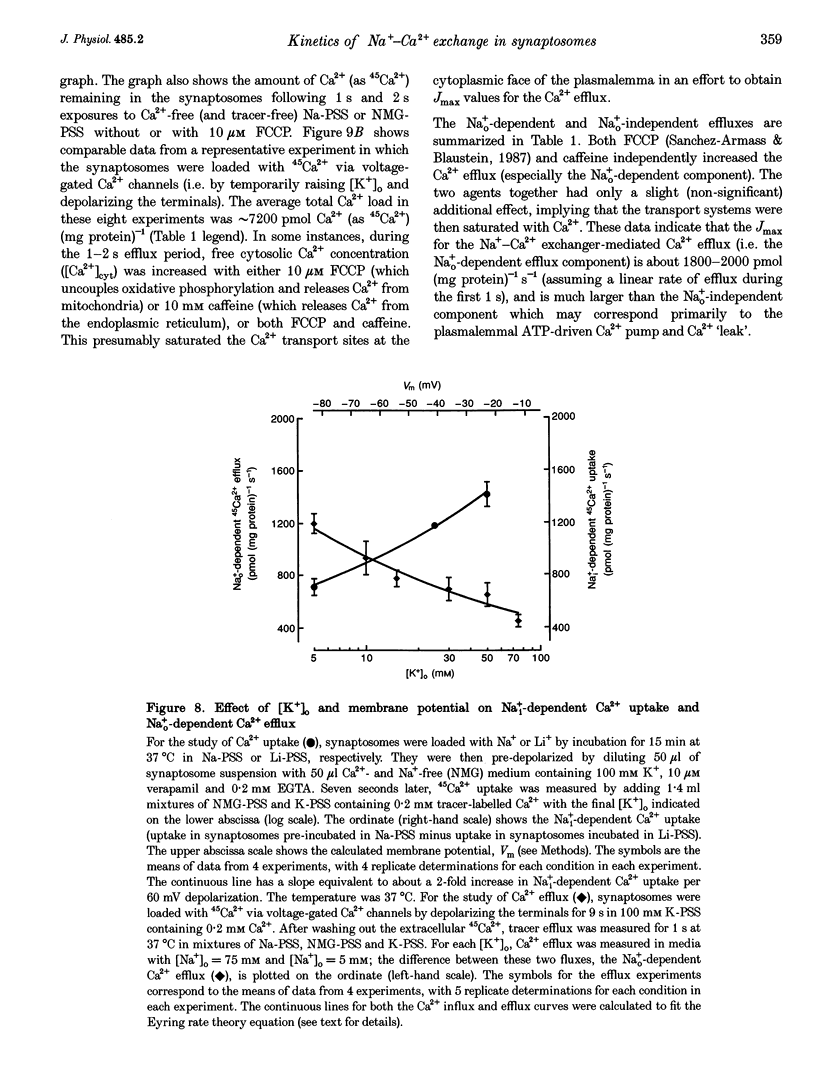
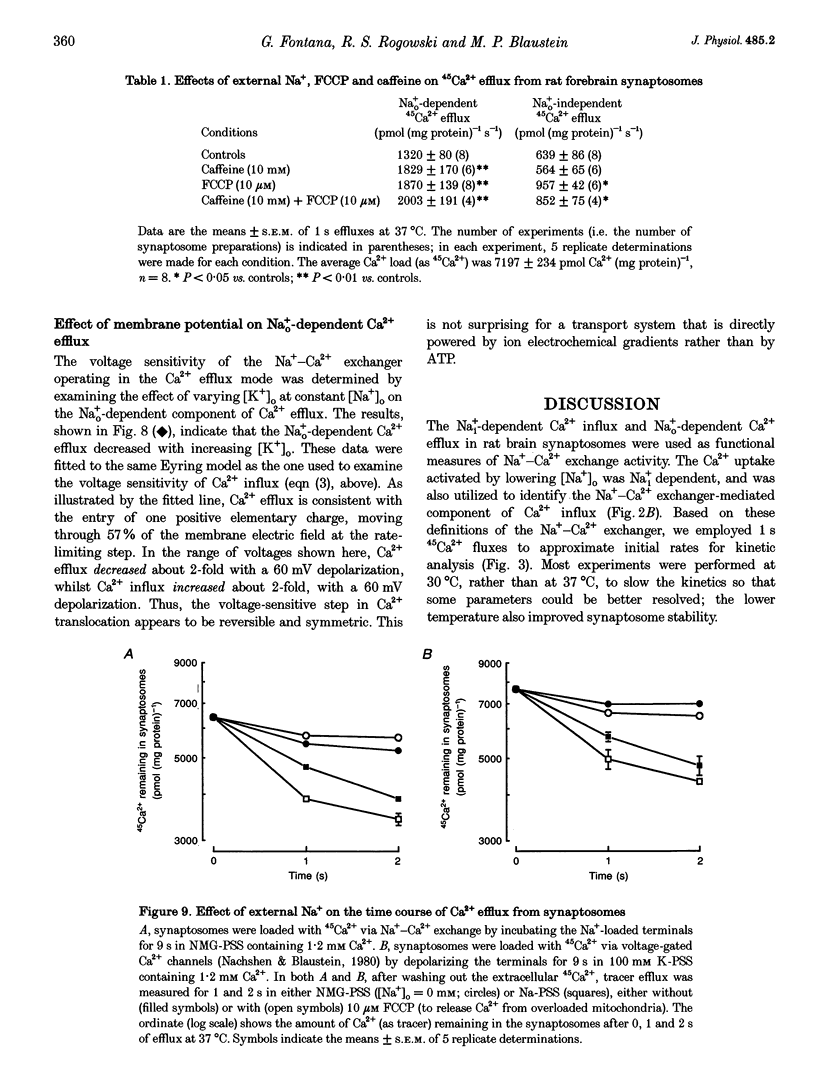
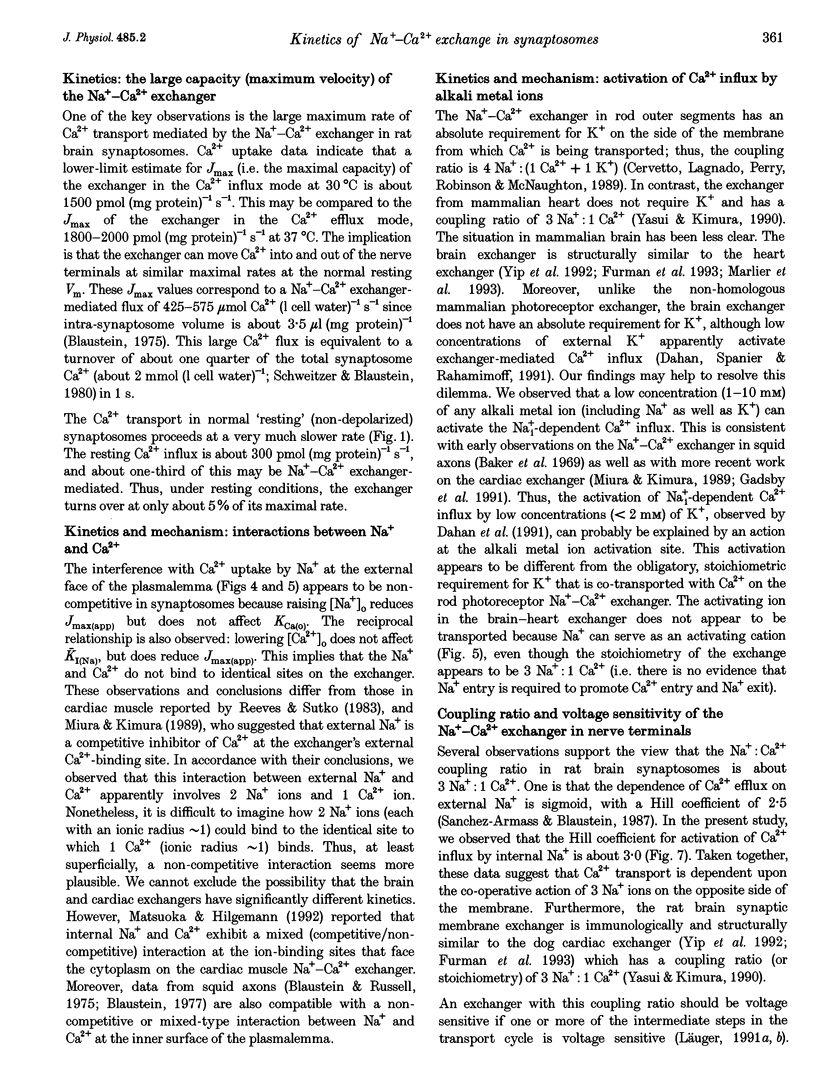
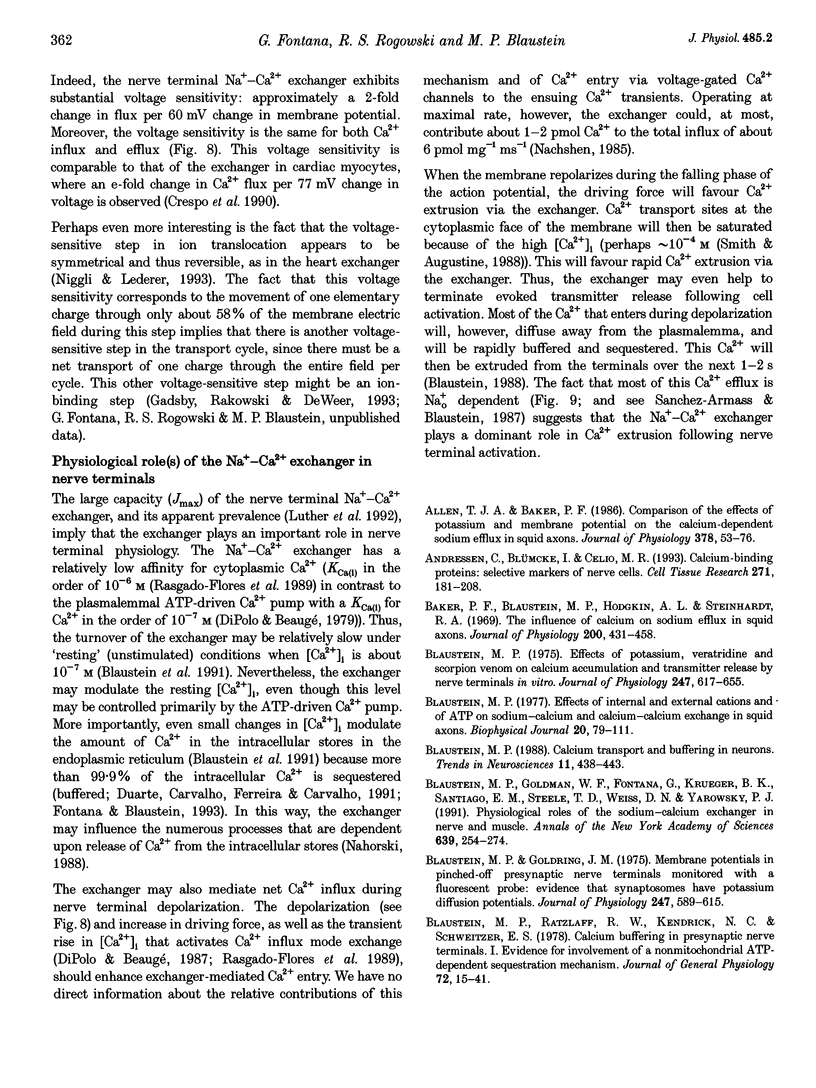
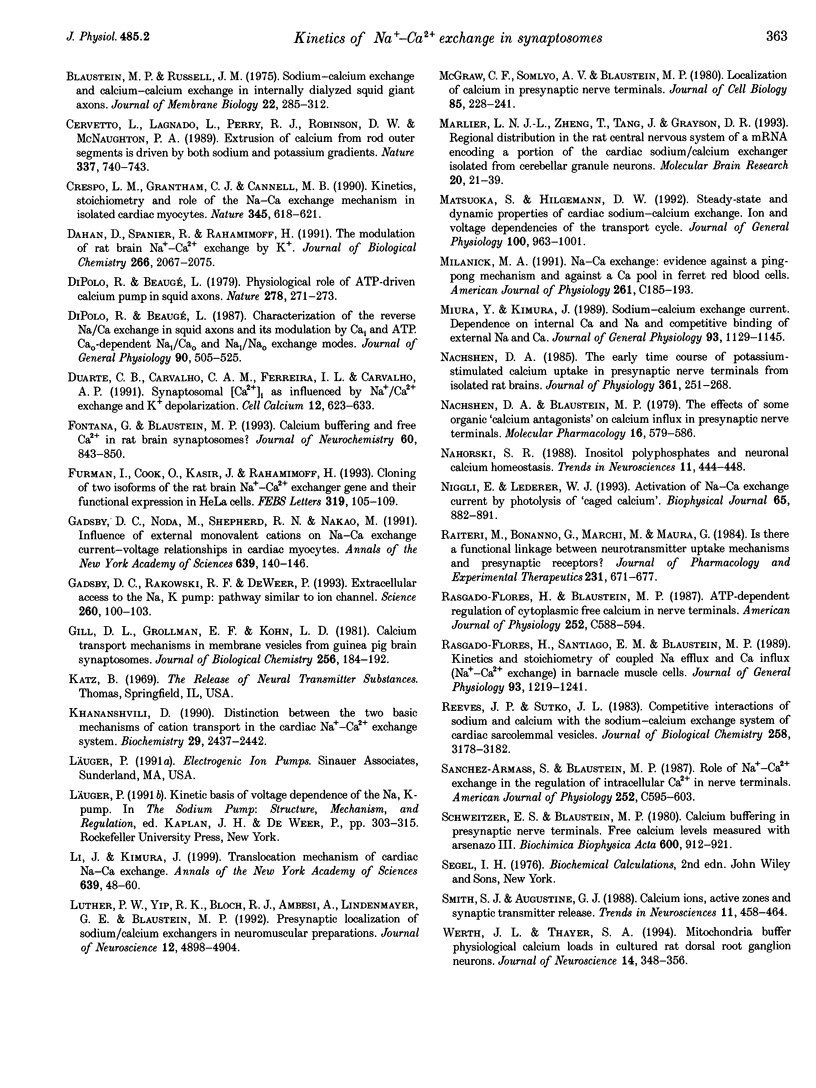
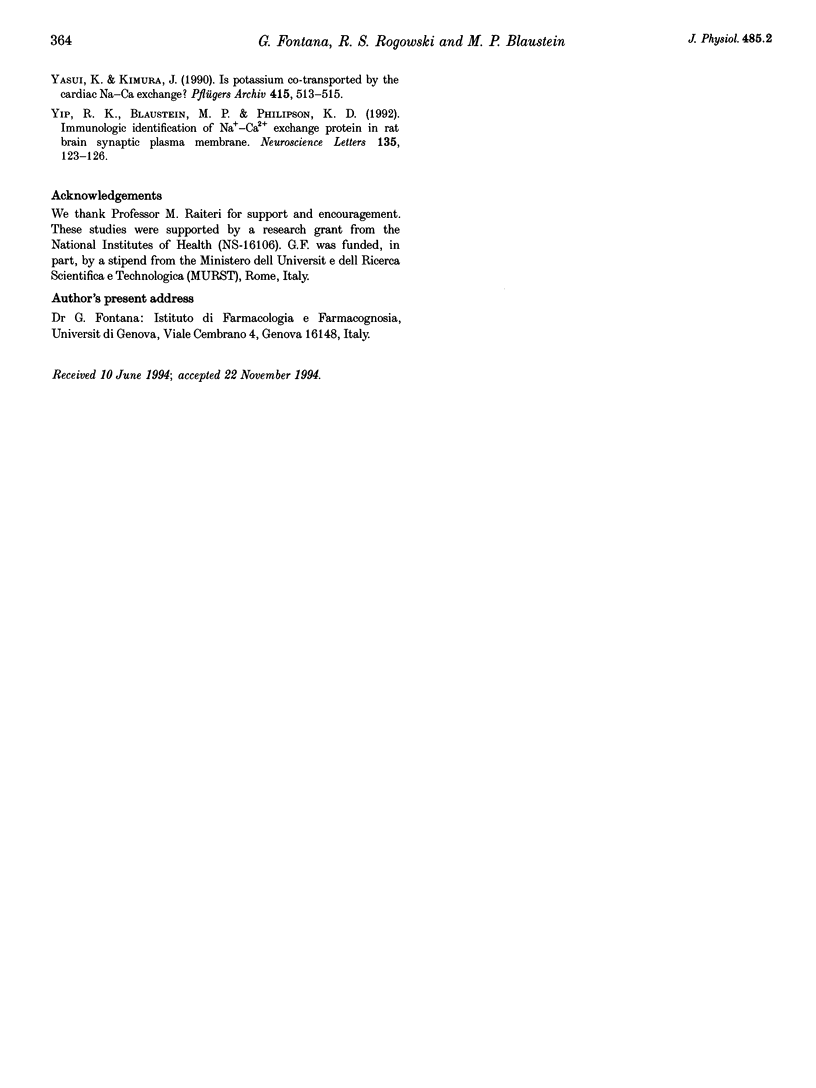
Selected References
These references are in PubMed. This may not be the complete list of references from this article.
- Allen T. J., Baker P. F. Comparison of the effects of potassium and membrane potential on the calcium-dependent sodium efflux in squid axons. J Physiol. 1986 Sep;378:53–76. doi: 10.1113/jphysiol.1986.sp016207. [DOI] [PMC free article] [PubMed] [Google Scholar]
- Andressen C., Blümcke I., Celio M. R. Calcium-binding proteins: selective markers of nerve cells. Cell Tissue Res. 1993 Feb;271(2):181–208. doi: 10.1007/BF00318606. [DOI] [PubMed] [Google Scholar]
- Baker P. F., Blaustein M. P., Hodgkin A. L., Steinhardt R. A. The influence of calcium on sodium efflux in squid axons. J Physiol. 1969 Feb;200(2):431–458. doi: 10.1113/jphysiol.1969.sp008702. [DOI] [PMC free article] [PubMed] [Google Scholar]
- Blaustein M. P. Calcium transport and buffering in neurons. Trends Neurosci. 1988 Oct;11(10):438–443. doi: 10.1016/0166-2236(88)90195-6. [DOI] [PubMed] [Google Scholar]
- Blaustein M. P. Effects of potassium, veratridine, and scorpion venom on calcium accumulation and transmitter release by nerve terminals in vitro. J Physiol. 1975 Jun;247(3):617–655. doi: 10.1113/jphysiol.1975.sp010950. [DOI] [PMC free article] [PubMed] [Google Scholar]
- Blaustein M. P., Goldman W. F., Fontana G., Krueger B. K., Santiago E. M., Steele T. D., Weiss D. N., Yarowsky P. J. Physiological roles of the sodium-calcium exchanger in nerve and muscle. Ann N Y Acad Sci. 1991;639:254–274. doi: 10.1111/j.1749-6632.1991.tb17315.x. [DOI] [PubMed] [Google Scholar]
- Blaustein M. P., Goldring J. M. Membrane potentials in pinched-off presynaptic nerve ternimals monitored with a fluorescent probe: evidence that synaptosomes have potassium diffusion potentials. J Physiol. 1975 Jun;247(3):589–615. doi: 10.1113/jphysiol.1975.sp010949. [DOI] [PMC free article] [PubMed] [Google Scholar]
- Blaustein M. P., Ratzlaff R. W., Kendrick N. C., Schweitzer E. S. Calcium buffering in presynaptic nerve terminals. I. Evidence for involvement of a nonmitochondrial ATP-dependent sequestration mechanism. J Gen Physiol. 1978 Jul;72(1):15–41. doi: 10.1085/jgp.72.1.15. [DOI] [PMC free article] [PubMed] [Google Scholar]
- Blaustein M. P., Russell J. M. Sodium-calcium exchange and calcium-calcium exchange in internally dialyzed squid giant axons. J Membr Biol. 1975 Jul 24;22(3-4):285–312. doi: 10.1007/BF01868176. [DOI] [PubMed] [Google Scholar]
- Blaustein M. P., Santiago E. M. Effects of internal and external cations and of ATP on sodium-calcium and calcium-calcium exchange in squid axons. Biophys J. 1977 Oct;20(1):79–111. doi: 10.1016/S0006-3495(77)85538-0. [DOI] [PMC free article] [PubMed] [Google Scholar]
- Cervetto L., Lagnado L., Perry R. J., Robinson D. W., McNaughton P. A. Extrusion of calcium from rod outer segments is driven by both sodium and potassium gradients. Nature. 1989 Feb 23;337(6209):740–743. doi: 10.1038/337740a0. [DOI] [PubMed] [Google Scholar]
- Crespo L. M., Grantham C. J., Cannell M. B. Kinetics, stoichiometry and role of the Na-Ca exchange mechanism in isolated cardiac myocytes. Nature. 1990 Jun 14;345(6276):618–621. doi: 10.1038/345618a0. [DOI] [PubMed] [Google Scholar]
- Dahan D., Spanier R., Rahamimoff H. The modulation of rat brain Na(+)-Ca2+ exchange by K+. J Biol Chem. 1991 Feb 5;266(4):2067–2075. [PubMed] [Google Scholar]
- DiPolo R., Beaugé L. Characterization of the reverse Na/Ca exchange in squid axons and its modulation by Cai and ATP. Cai-dependent Nai/Cao and Nai/Nao exchange modes. J Gen Physiol. 1987 Oct;90(4):505–525. doi: 10.1085/jgp.90.4.505. [DOI] [PMC free article] [PubMed] [Google Scholar]
- DiPolo R., Beaugé L. Physiological role of ATP-driven calcium pump in squid axon. Nature. 1979 Mar 15;278(5701):271–273. doi: 10.1038/278271a0. [DOI] [PubMed] [Google Scholar]
- Duarte C. B., Carvalho C. A., Ferreira I. L., Carvalho A. P. Synaptosomal [Ca2+]i as influenced by Na+/Ca2+ exchange and K+ depolarization. Cell Calcium. 1991 Oct;12(9):623–633. doi: 10.1016/0143-4160(91)90059-n. [DOI] [PubMed] [Google Scholar]
- Fontana G., Blaustein M. P. Calcium buffering and free Ca2+ in rat brain synaptosomes. J Neurochem. 1993 Mar;60(3):843–850. doi: 10.1111/j.1471-4159.1993.tb03228.x. [DOI] [PubMed] [Google Scholar]
- Furman I., Cook O., Kasir J., Rahamimoff H. Cloning of two isoforms of the rat brain Na(+)-Ca2+ exchanger gene and their functional expression in HeLa cells. FEBS Lett. 1993 Mar 15;319(1-2):105–109. doi: 10.1016/0014-5793(93)80046-w. [DOI] [PubMed] [Google Scholar]
- Gadsby D. C., Noda M., Shepherd R. N., Nakao M. Influence of external monovalent cations on Na-Ca exchange current-voltage relationships in cardiac myocytes. Ann N Y Acad Sci. 1991;639:140–146. doi: 10.1111/j.1749-6632.1991.tb17297.x. [DOI] [PubMed] [Google Scholar]
- Gadsby D. C., Rakowski R. F., De Weer P. Extracellular access to the Na,K pump: pathway similar to ion channel. Science. 1993 Apr 2;260(5104):100–103. doi: 10.1126/science.7682009. [DOI] [PubMed] [Google Scholar]
- Gill D. L., Grollman E. F., Kohn L. D. Calcium transport mechanisms in membrane vesicles from guinea pig brain synaptosomes. J Biol Chem. 1981 Jan 10;256(1):184–192. [PubMed] [Google Scholar]
- Khananshvili D. Distinction between the two basic mechanisms of cation transport in the cardiac Na(+)-Ca2+ exchange system. Biochemistry. 1990 Mar 13;29(10):2437–2442. doi: 10.1021/bi00462a001. [DOI] [PubMed] [Google Scholar]
- Li J. M., Kimura J. Translocation mechanism of cardiac Na-Ca exchange. Ann N Y Acad Sci. 1991;639:48–60. doi: 10.1111/j.1749-6632.1991.tb17288.x. [DOI] [PubMed] [Google Scholar]
- Luther P. W., Yip R. K., Bloch R. J., Ambesi A., Lindenmayer G. E., Blaustein M. P. Presynaptic localization of sodium/calcium exchangers in neuromuscular preparations. J Neurosci. 1992 Dec;12(12):4898–4904. doi: 10.1523/JNEUROSCI.12-12-04898.1992. [DOI] [PMC free article] [PubMed] [Google Scholar]
- Marlier L. N., Zheng T., Tang J., Grayson D. R. Regional distribution in the rat central nervous system of a mRNA encoding a portion of the cardiac sodium/calcium exchanger isolated from cerebellar granule neurons. Brain Res Mol Brain Res. 1993 Oct;20(1-2):21–39. doi: 10.1016/0169-328x(93)90107-z. [DOI] [PubMed] [Google Scholar]
- Matsuoka S., Hilgemann D. W. Steady-state and dynamic properties of cardiac sodium-calcium exchange. Ion and voltage dependencies of the transport cycle. J Gen Physiol. 1992 Dec;100(6):963–1001. doi: 10.1085/jgp.100.6.963. [DOI] [PMC free article] [PubMed] [Google Scholar]
- McGraw C. F., Somlyo A. V., Blaustein M. P. Localization of calcium in presynaptic nerve terminals. An ultrastructural and electron microprobe analysis. J Cell Biol. 1980 May;85(2):228–241. doi: 10.1083/jcb.85.2.228. [DOI] [PMC free article] [PubMed] [Google Scholar]
- Milanick M. A. Na-Ca exchange: evidence against a ping-pong mechanism and against a Ca pool in ferret red blood cells. Am J Physiol. 1991 Jul;261(1 Pt 1):C185–C193. doi: 10.1152/ajpcell.1991.261.1.C185. [DOI] [PubMed] [Google Scholar]
- Miura Y., Kimura J. Sodium-calcium exchange current. Dependence on internal Ca and Na and competitive binding of external Na and Ca. J Gen Physiol. 1989 Jun;93(6):1129–1145. doi: 10.1085/jgp.93.6.1129. [DOI] [PMC free article] [PubMed] [Google Scholar]
- Nachshen D. A., Blaustein M. P. The effects of some organic "calcium antagonists" on calcium influx in presynaptic nerve terminals. Mol Pharmacol. 1979 Sep;16(2):576–586. [PubMed] [Google Scholar]
- Nachshen D. A. The early time course of potassium-stimulated calcium uptake in presynaptic nerve terminals isolated from rat brain. J Physiol. 1985 Apr;361:251–268. doi: 10.1113/jphysiol.1985.sp015644. [DOI] [PMC free article] [PubMed] [Google Scholar]
- Nahorski S. R. Inositol polyphosphates and neuronal calcium homeostasis. Trends Neurosci. 1988 Oct;11(10):444–448. doi: 10.1016/0166-2236(88)90196-8. [DOI] [PubMed] [Google Scholar]
- Niggli E., Lederer W. J. Activation of Na-Ca exchange current by photolysis of "caged calcium". Biophys J. 1993 Aug;65(2):882–891. doi: 10.1016/S0006-3495(93)81105-6. [DOI] [PMC free article] [PubMed] [Google Scholar]
- Raiteri M., Bonanno G., Marchi M., Maura G. Is there a functional linkage between neurotransmitter uptake mechanisms and presynaptic receptors? J Pharmacol Exp Ther. 1984 Dec;231(3):671–677. [PubMed] [Google Scholar]
- Rasgado-Flores H., Blaustein M. P. ATP-dependent regulation of cytoplasmic free calcium in nerve terminals. Am J Physiol. 1987 Jun;252(6 Pt 1):C588–C594. doi: 10.1152/ajpcell.1987.252.6.C588. [DOI] [PubMed] [Google Scholar]
- Rasgado-Flores H., Santiago E. M., Blaustein M. P. Kinetics and stoichiometry of coupled Na efflux and Ca influx (Na/Ca exchange) in barnacle muscle cells. J Gen Physiol. 1989 Jun;93(6):1219–1241. doi: 10.1085/jgp.93.6.1219. [DOI] [PMC free article] [PubMed] [Google Scholar]
- Reeves J. P., Sutko J. L. Competitive interactions of sodium and calcium with the sodium-calcium exchange system of cardiac sarcolemmal vesicles. J Biol Chem. 1983 Mar 10;258(5):3178–3182. [PubMed] [Google Scholar]
- Sanchez-Armass S., Blaustein M. P. Role of sodium-calcium exchange in regulation of intracellular calcium in nerve terminals. Am J Physiol. 1987 Jun;252(6 Pt 1):C595–C603. doi: 10.1152/ajpcell.1987.252.6.C595. [DOI] [PubMed] [Google Scholar]
- Schweitzer E. S., Blaustein M. P. Calcium buffering in presynaptic nerve terminals. Free calcium levels measured with arsenazo III. Biochim Biophys Acta. 1980 Aug 14;600(3):912–921. doi: 10.1016/0005-2736(80)90493-9. [DOI] [PubMed] [Google Scholar]
- Smith S. J., Augustine G. J. Calcium ions, active zones and synaptic transmitter release. Trends Neurosci. 1988 Oct;11(10):458–464. doi: 10.1016/0166-2236(88)90199-3. [DOI] [PubMed] [Google Scholar]
- Werth J. L., Thayer S. A. Mitochondria buffer physiological calcium loads in cultured rat dorsal root ganglion neurons. J Neurosci. 1994 Jan;14(1):348–356. doi: 10.1523/JNEUROSCI.14-01-00348.1994. [DOI] [PMC free article] [PubMed] [Google Scholar]
- Yasui K., Kimura J. Is potassium co-transported by the cardiac Na-Ca exchange? Pflugers Arch. 1990 Jan;415(4):513–515. doi: 10.1007/BF00373636. [DOI] [PubMed] [Google Scholar]
- Yip R. K., Blaustein M. P., Philipson K. D. Immunologic identification of Na/Ca exchange protein in rat brain synaptic plasma membrane. Neurosci Lett. 1992 Feb 17;136(1):123–126. doi: 10.1016/0304-3940(92)90663-r. [DOI] [PubMed] [Google Scholar]