Abstract
A mutant of Arabidopsis thaliana (L.) Heyn. (a small plant in the crucifer family) that lacks glycine decarboxylase activity owing to a recessive nuclear mutation has been isolated on the basis of a growth requirement for high concentrations of atmospheric CO2. Mitochondria isolated from leaves of the mutant did not exhibit glycine-dependent O2 consumption, did not release 14CO2 from [14C]glycine, and did not catalyse the glycine-bicarbonate exchange reaction that is considered to be the first partial reaction associated with glycine cleavage. Photosynthesis in the mutant was decreased after illumination under atmospheric conditions that promote partitioning of carbon into intermediates of the photorespiratory pathway, but was not impaired under non-photorespiratory conditions. Thus glycine decarboxylase activity is not required for any essential function unrelated to photorespiration. The photosynthetic response of the mutant in photorespiratory conditions is probably caused by an increased rate of glyoxylate oxidation, which results from the sequestering of all readily transferable amino groups in a metabolically inactive glycine pool, and by a depletion of intermediates from the photosynthesis cycle. The rate of release of 14CO2 from exogenously applied [14C]glycollate was 14-fold lower in the mutant than in the wild type, suggesting that glycine decarboxylation is the only significant source of photorespiratory CO2.
Full text
PDF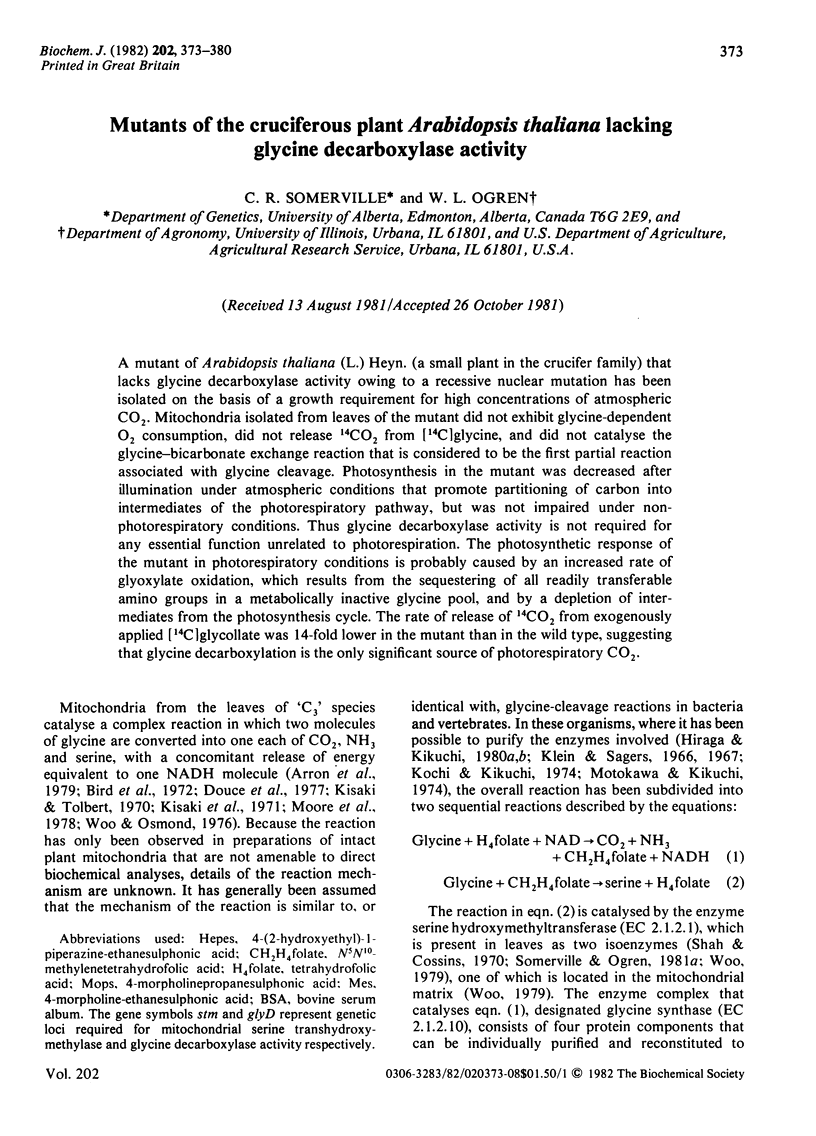
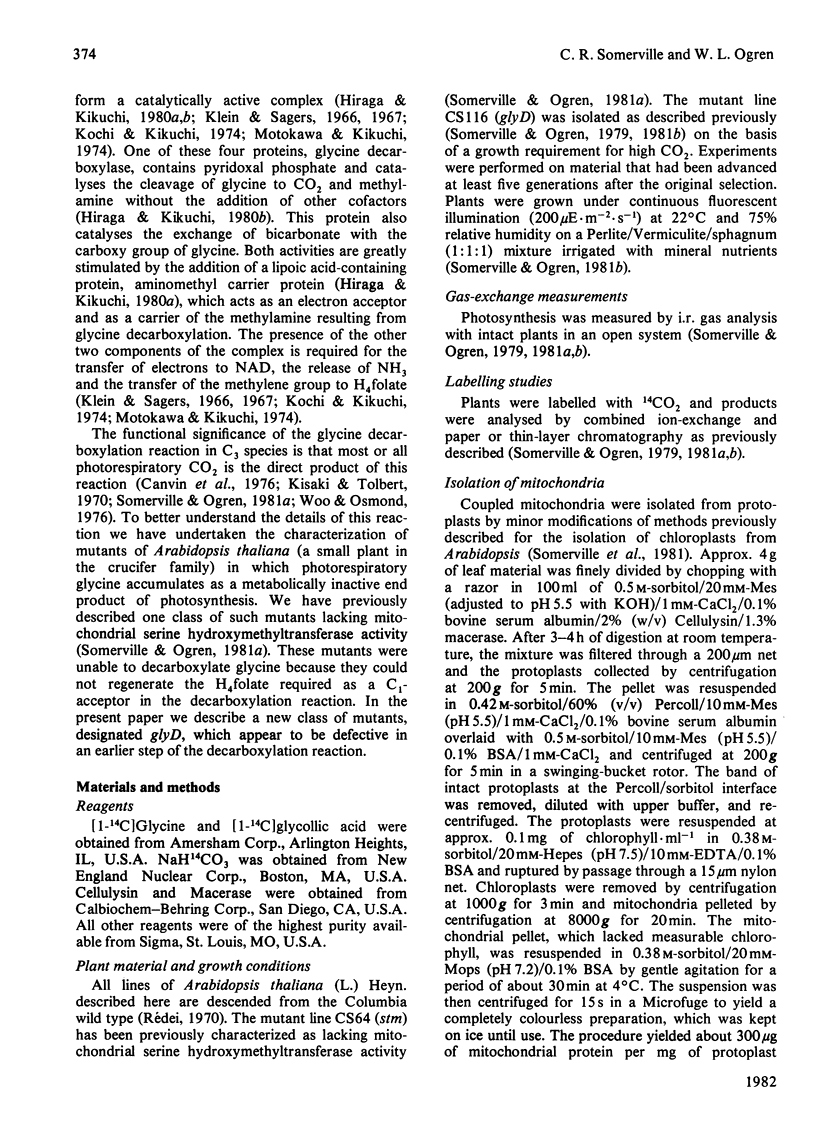
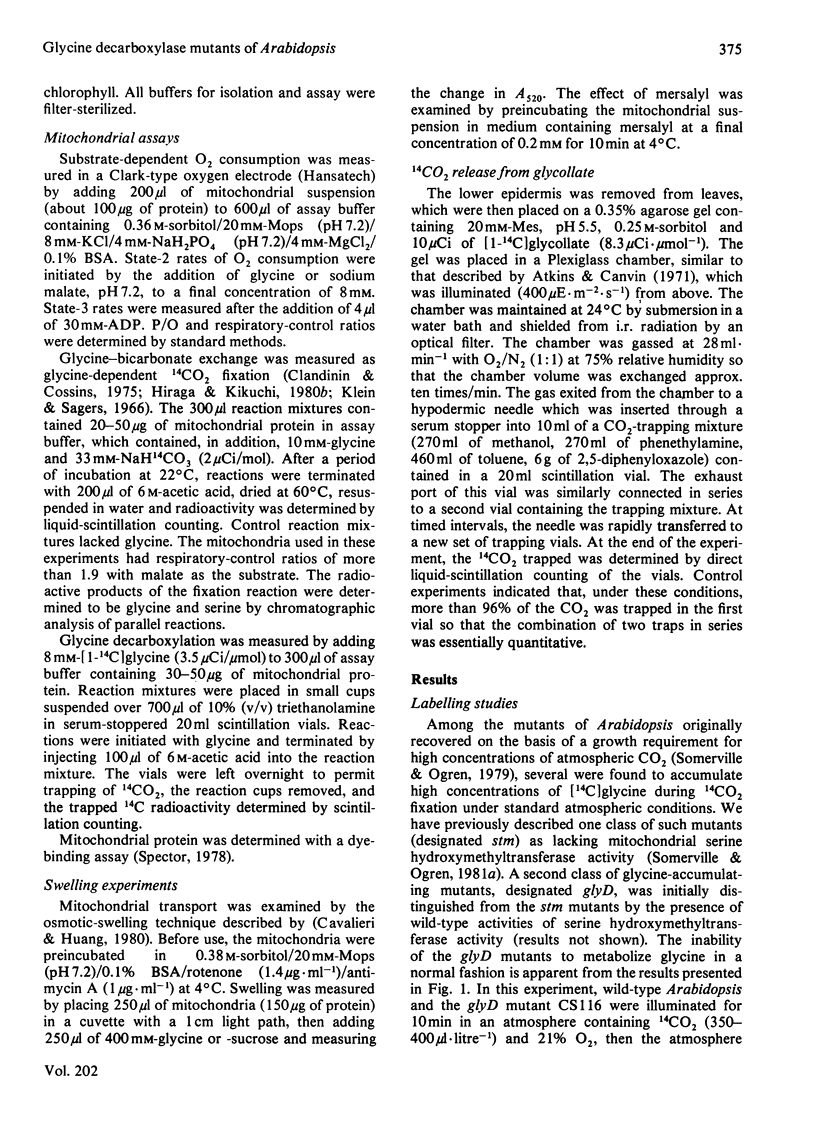
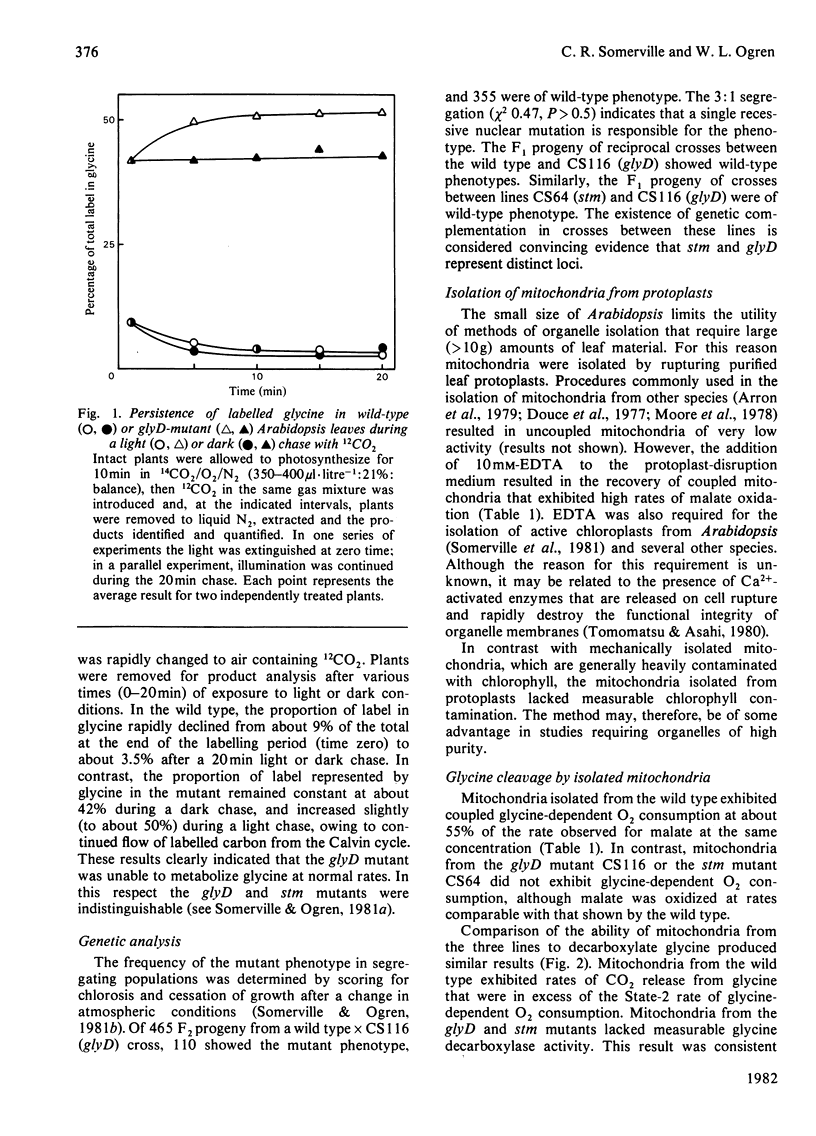
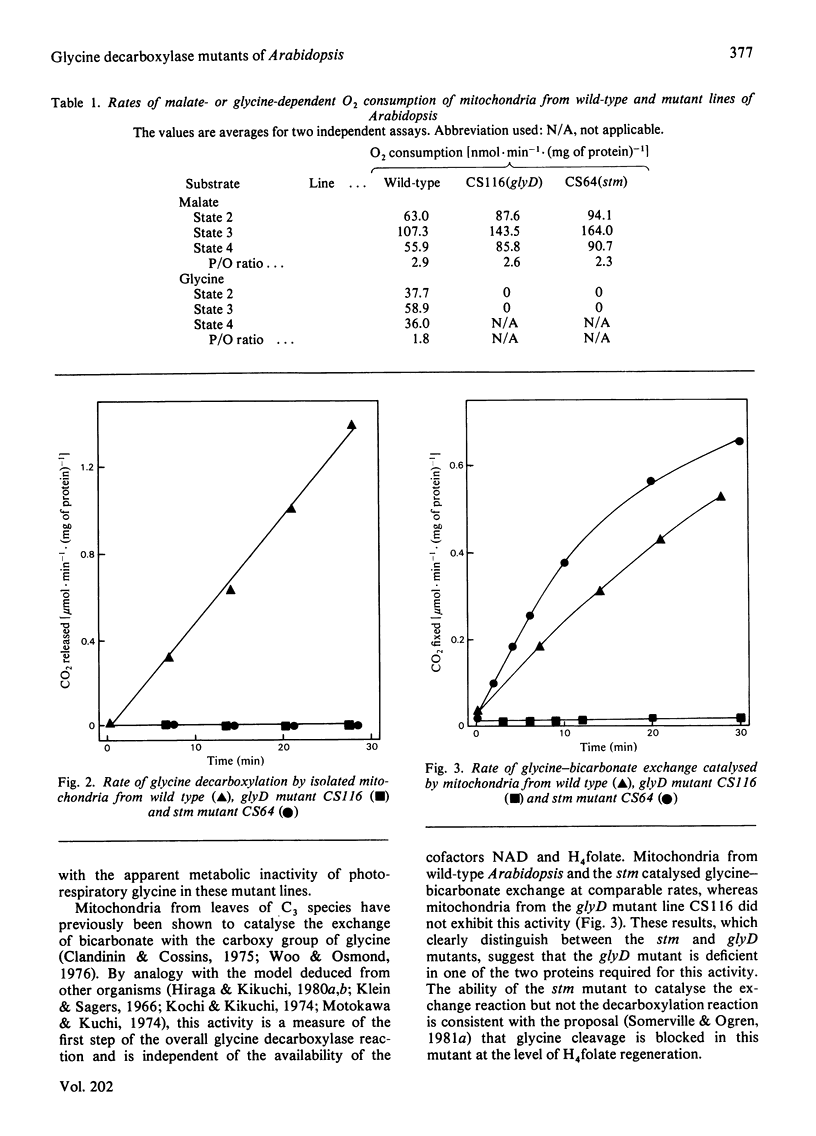
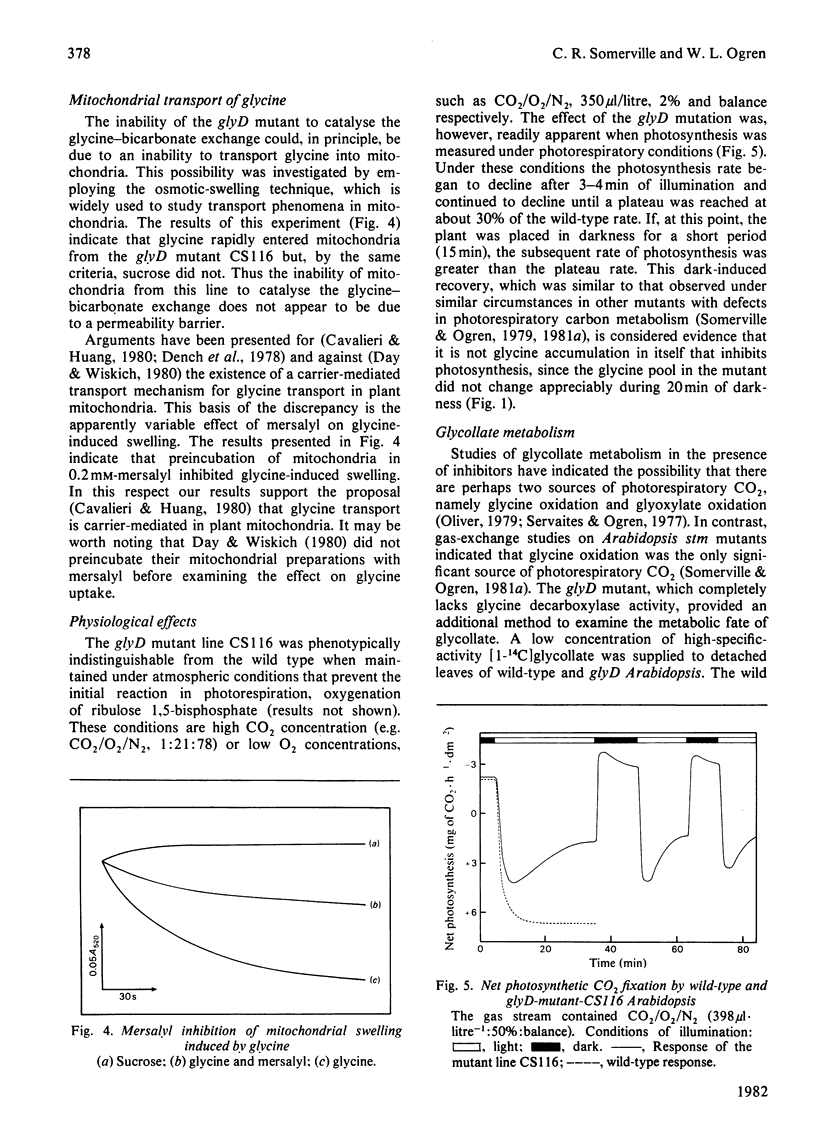
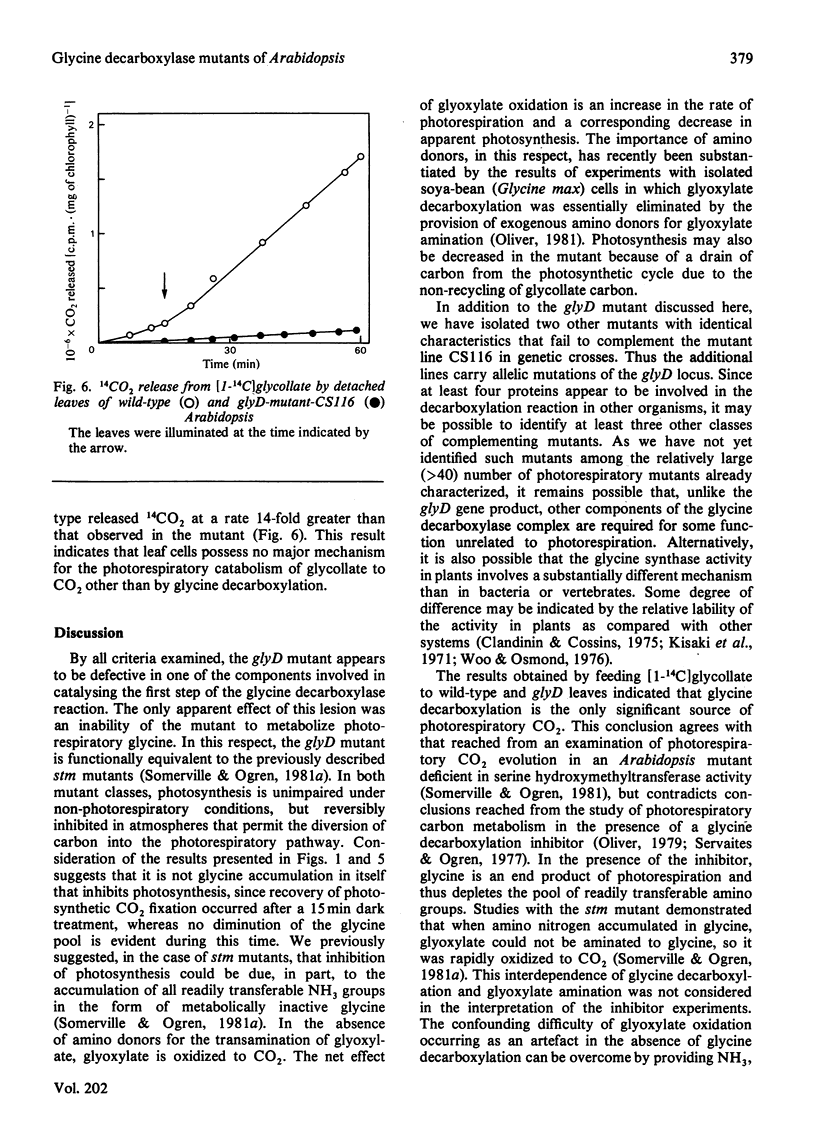
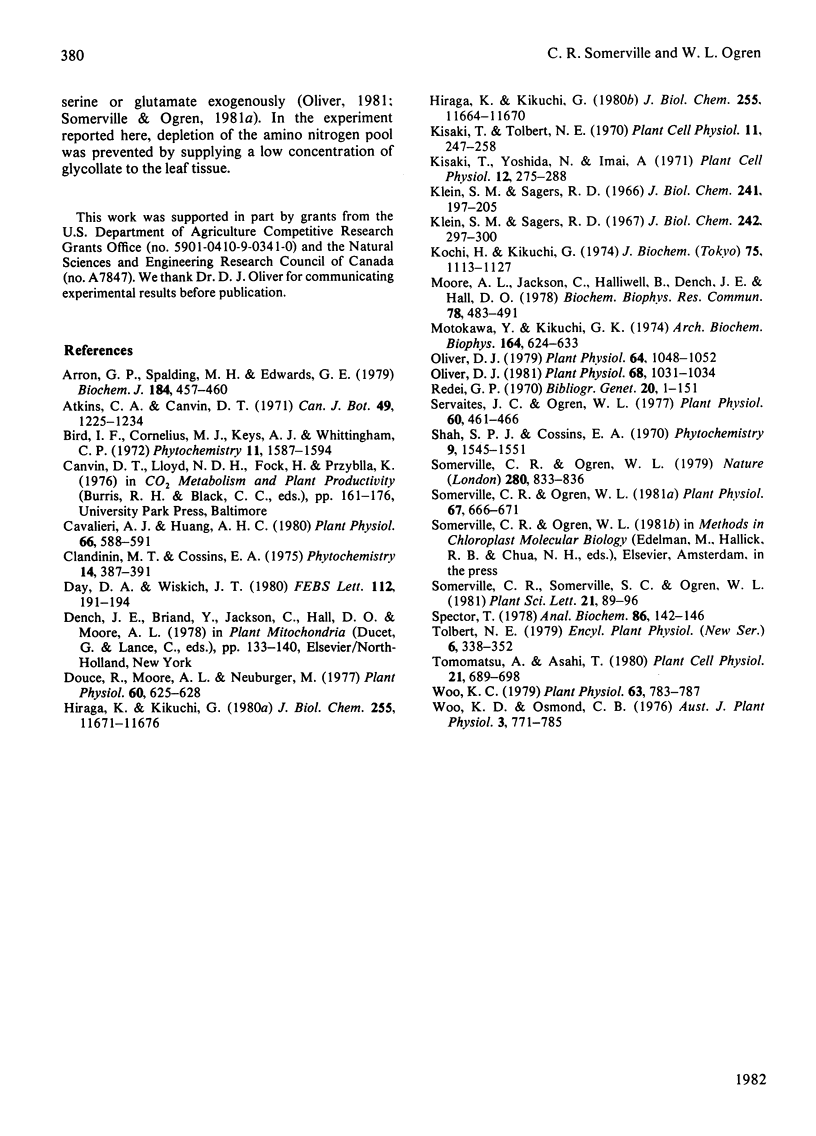
Selected References
These references are in PubMed. This may not be the complete list of references from this article.
- Cadenas E., Garland P. B. Synthesis of cytoplasmic membrane during growth and division of Escherichia coli. Dispersive behaviour of respiratory nitrate reductase. Biochem J. 1979 Oct 15;184(1):45–50. doi: 10.1042/bj1840045. [DOI] [PMC free article] [PubMed] [Google Scholar]
- Cavalieri A. J., Huang A. H. Carrier Protein-mediated Transport of Neutral Amino Acids into Mung Bean Mitochondria. Plant Physiol. 1980 Oct;66(4):588–591. doi: 10.1104/pp.66.4.588. [DOI] [PMC free article] [PubMed] [Google Scholar]
- Douce R., Moore A. L., Neuburger M. Isolation and oxidative properties of intact mitochondria isolated from spinach leaves. Plant Physiol. 1977 Oct;60(4):625–628. doi: 10.1104/pp.60.4.625. [DOI] [PMC free article] [PubMed] [Google Scholar]
- Hiraga K., Kikuchi G. The mitochondrial glycine cleavage system. Functional association of glycine decarboxylase and aminomethyl carrier protein. J Biol Chem. 1980 Dec 25;255(24):11671–11676. [PubMed] [Google Scholar]
- Hiraga K., Kikuchi G. The mitochondrial glycine cleavage system. Purification and properties of glycine decarboxylase from chicken liver mitochondria. J Biol Chem. 1980 Dec 25;255(24):11664–11670. [PubMed] [Google Scholar]
- Klein S. M., Sagers R. D. Glycine metabolism. 3. A flavin-linked dehydrogenase associated with the glycine cleavage system in Peptococcus glycinophilus. J Biol Chem. 1967 Jan 25;242(2):297–300. [PubMed] [Google Scholar]
- Klein S. M., Sagers R. D. Glycine metabolism. I. Properties of the system catalyzing the exchange of bicarbonate with the carboxyl group of glycine in Peptococcus glycinophilus. J Biol Chem. 1966 Jan 10;241(1):197–205. [PubMed] [Google Scholar]
- Kochi H., Kikuchi G. Mechanism of the reversible glycine cleavage reaction in Arthrobacter globiformis. I. Purification and function of protein components required for the reaction. J Biochem. 1974 May;75(5):1113–1127. doi: 10.1093/oxfordjournals.jbchem.a130483. [DOI] [PubMed] [Google Scholar]
- Moore A. L., Jackson C., Halliwell B., Dench J. E., Hall D. O. Intramitochondrial localisation of glycine decarboxylase in spinach leaves. Biochem Biophys Res Commun. 1977 Sep 23;78(2):483–491. doi: 10.1016/0006-291x(77)90204-2. [DOI] [PubMed] [Google Scholar]
- Motokawa Y., Kikuchi G. Glycine metabolism by rat liver mitochondria. Reconstruction of the reversible glycine cleavage system with partially purified protein components. Arch Biochem Biophys. 1974 Oct;164(2):624–633. doi: 10.1016/0003-9861(74)90074-5. [DOI] [PubMed] [Google Scholar]
- Oliver D. J. Mechanism of decarboxylation of glycine and glycolate by isolated soybean cells. Plant Physiol. 1979 Dec;64(6):1048–1052. doi: 10.1104/pp.64.6.1048. [DOI] [PMC free article] [PubMed] [Google Scholar]
- Oliver D. J. Role of Glycine and Glyoxylate Decarboxylation in Photorespiratory CO(2) Release. Plant Physiol. 1981 Nov;68(5):1031–1034. doi: 10.1104/pp.68.5.1031. [DOI] [PMC free article] [PubMed] [Google Scholar]
- Servaites J. C. Chemical inhibition of the glycolate pathway in soybean leaf cells. Plant Physiol. 1977 Oct;60(4):461–466. doi: 10.1104/pp.60.4.461. [DOI] [PMC free article] [PubMed] [Google Scholar]
- Somerville C. R., Ogren W. L. Photorespiration-deficient Mutants of Arabidopsis thaliana Lacking Mitochondrial Serine Transhydroxymethylase Activity. Plant Physiol. 1981 Apr;67(4):666–671. doi: 10.1104/pp.67.4.666. [DOI] [PMC free article] [PubMed] [Google Scholar]
- Spector T. Refinement of the coomassie blue method of protein quantitation. A simple and linear spectrophotometric assay for less than or equal to 0.5 to 50 microgram of protein. Anal Biochem. 1978 May;86(1):142–146. doi: 10.1016/0003-2697(78)90327-5. [DOI] [PubMed] [Google Scholar]
- Woo K. C. Properties and intramitochondrial localization of serine hydroxymethyltransferase in leaves of higher plants. Plant Physiol. 1979 Apr;63(4):783–787. doi: 10.1104/pp.63.4.783. [DOI] [PMC free article] [PubMed] [Google Scholar]