Abstract
1. Long-term potentiation (LTP) was induced in EPSCs evoked in CA1 pyramidal neurones of young rats in vitro by extracellular stimulation of stratum radiatum. Low frequency stimulation was paired with postsynaptic depolarization to induce LTP, using whole-cell recording techniques. 2. Sufficient control and potentiated records were obtained under stable recording conditions to allow a quantal analysis of eleven EPSCs. The fluctuations in amplitude of all eleven EPSCs were quantized before conditioning stimulation, and they remained quantized after LTP induction, usually with an increased quantal variance. 3. Quantal current was increased by conditioning for nine out of eleven EPSCs. The increase in quantal current was correlated with the percentage increase in the EPSC. For only two EPSCs could the entire potentiation be attributed to an increase in quantal current. 4. The amplitude fluctuations of five control EPSCs could be described by binomial statistics, but after conditioning the binomial description held for only one of these EPSCs. For this EPSC, conditioning caused the release probability to increase from 0.39 +/- 0.05 to 0.47 +/- 0.02. 5. Quantal content was increased by conditioning stimulation for ten out of eleven EPSCs. The increase in quantal content was correlated with the percentage increase in the EPSC. However, for only four EPSCs could the entire potentiation be attributed to an increase in quantal content. 6. Most EPSCs were evoked with a high proportion of response failures. The probability of response failures decreased in eight out of eleven EPSCs following the induction of LTP. There was a negative correlation between the change in the probability of response failures and the amount of LTP. 7. The minimal number of sites at which transmission occurred increased for ten out of eleven EPSCs following LTP induction. Increases in the minimal number of active sites following conditioning were associated with decreases in the probability of response failures for seven out of eleven EPSCs. 8. The induction of LTP usually resulted in changes in the time course of the EPSCs. Cable analysis using a passive compartmental model of a CA1 pyramidal cell suggested that these time course changes were associated with shifts in the average electrotonic location of the active sites following LTP induction, rather than being caused by an increased duration of synaptic current. 9. LTP expression involves postsynaptic modifications to enhance the synaptic current at active sites. New sites are recruited, and our data cannot be used to determine if this is a result of a pre- or a postsynaptic change. Evidence for an increase in release probability was found for one EPSC.
Full text
PDF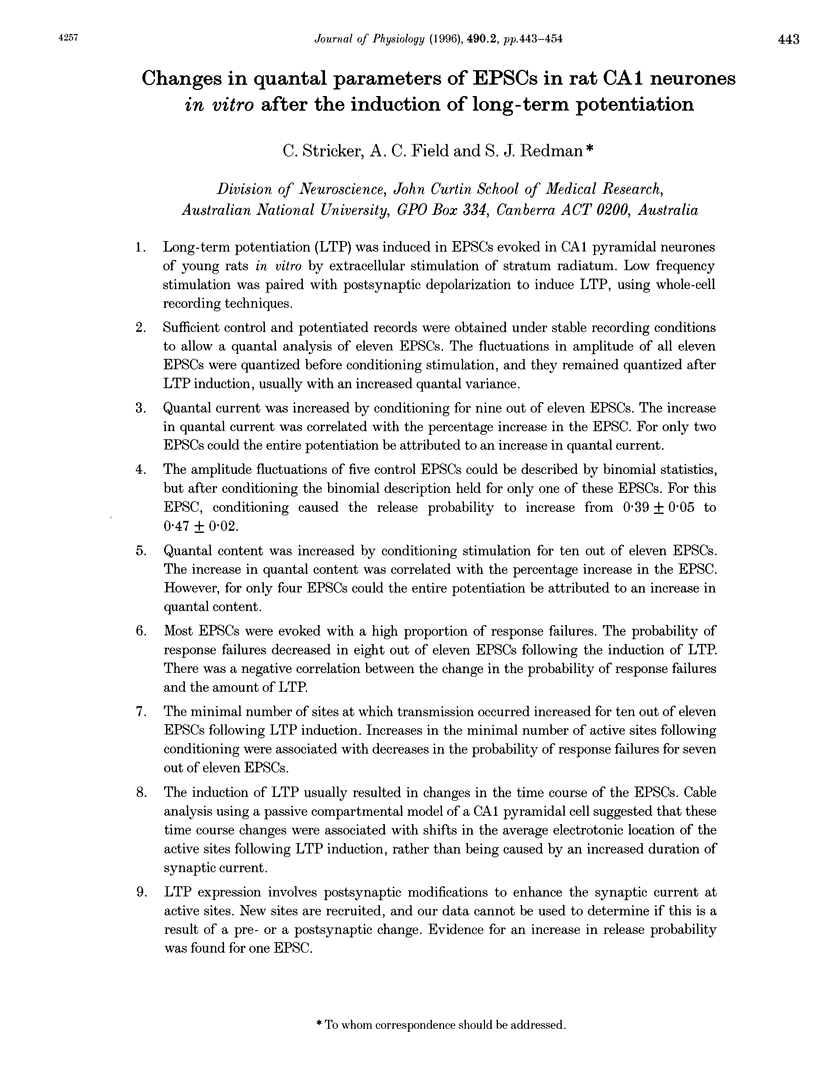
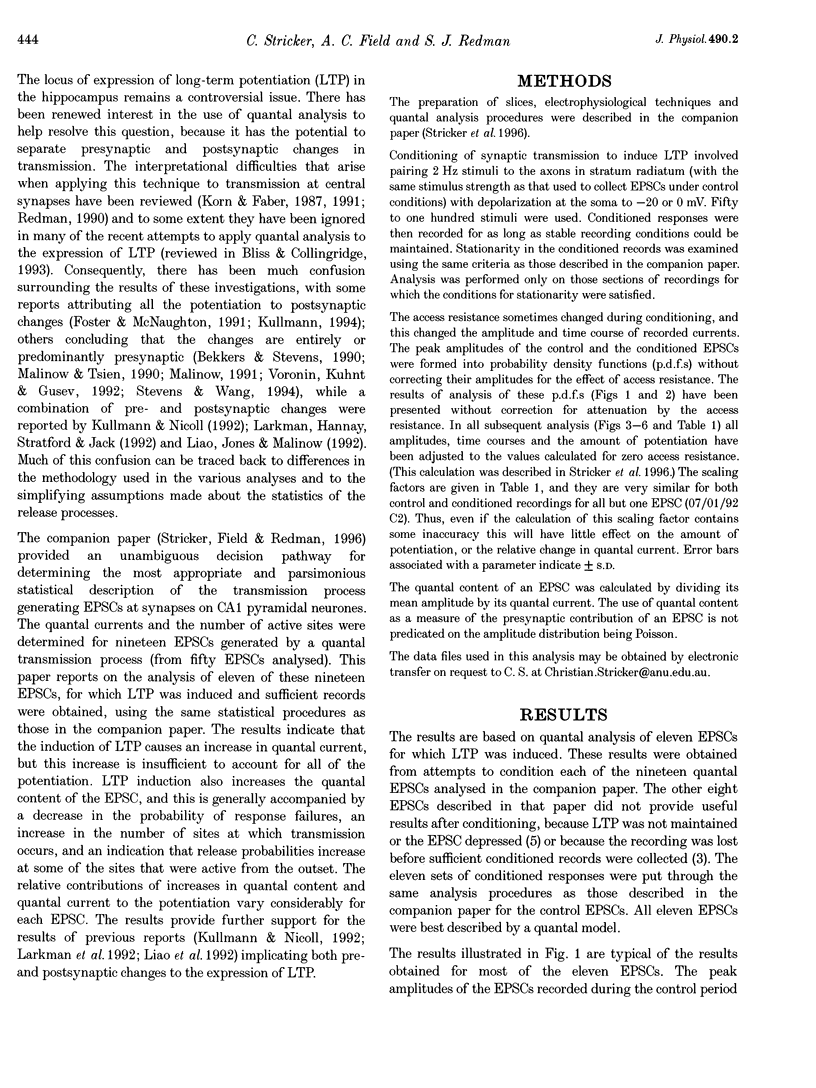
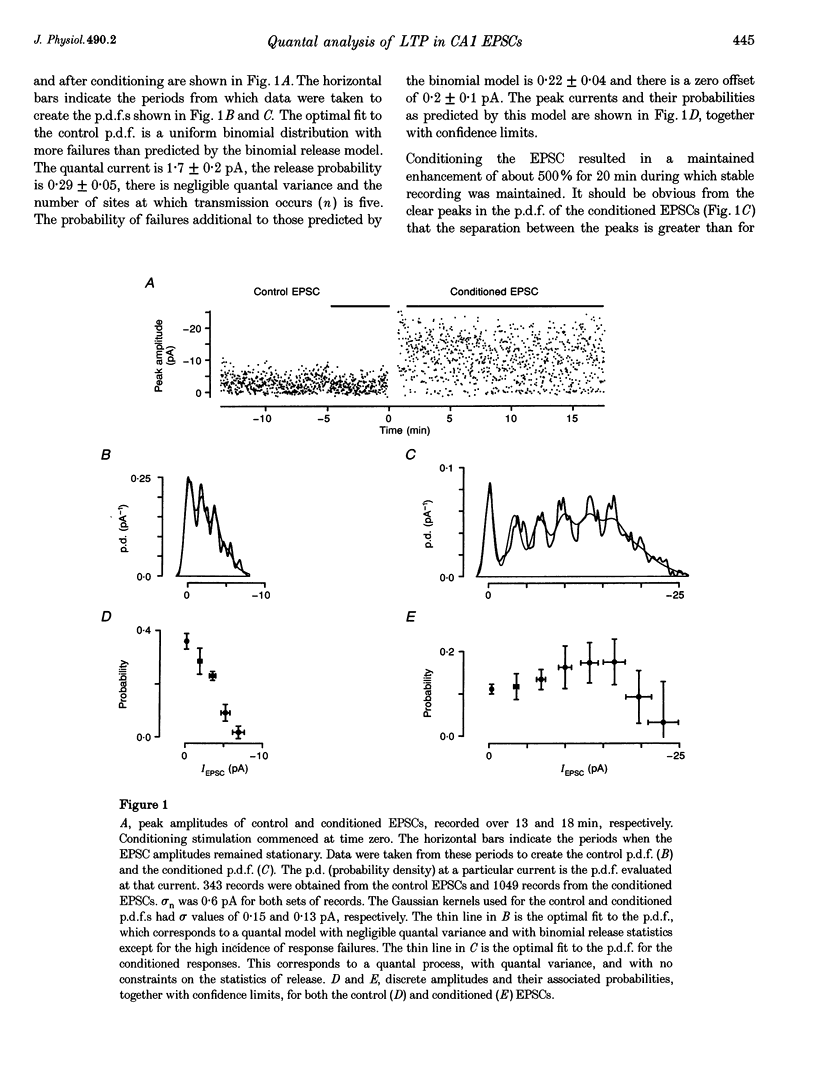
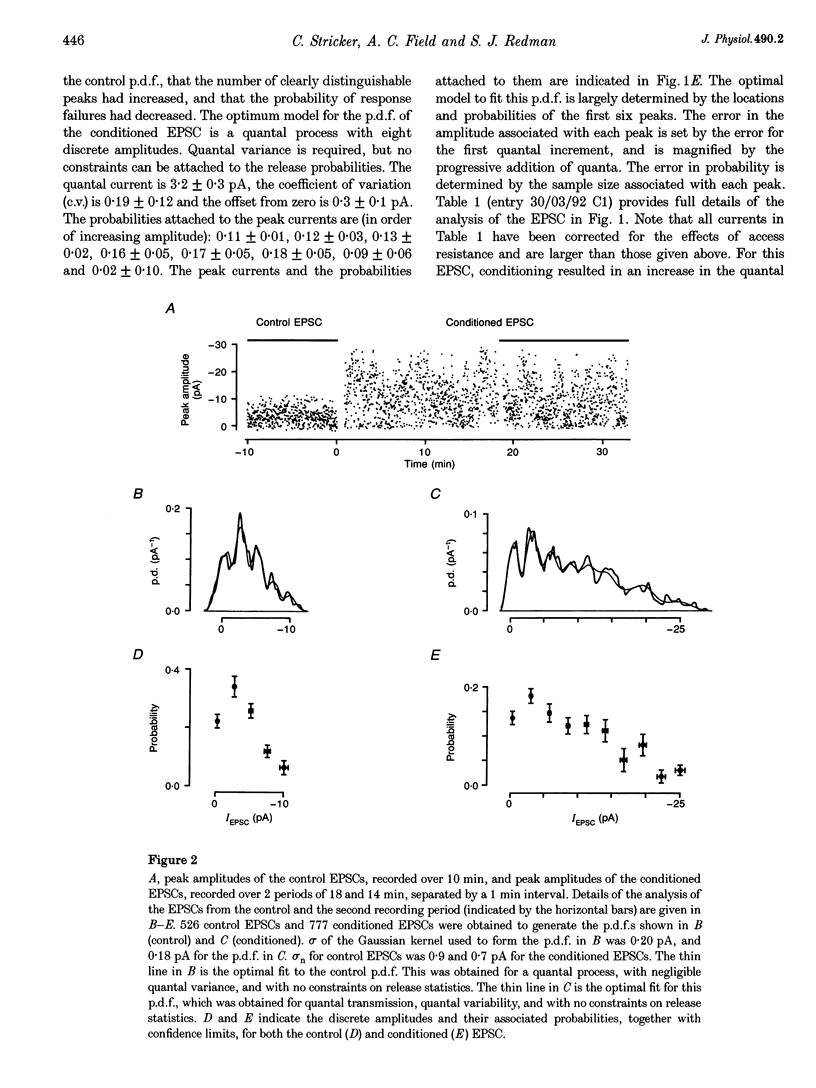
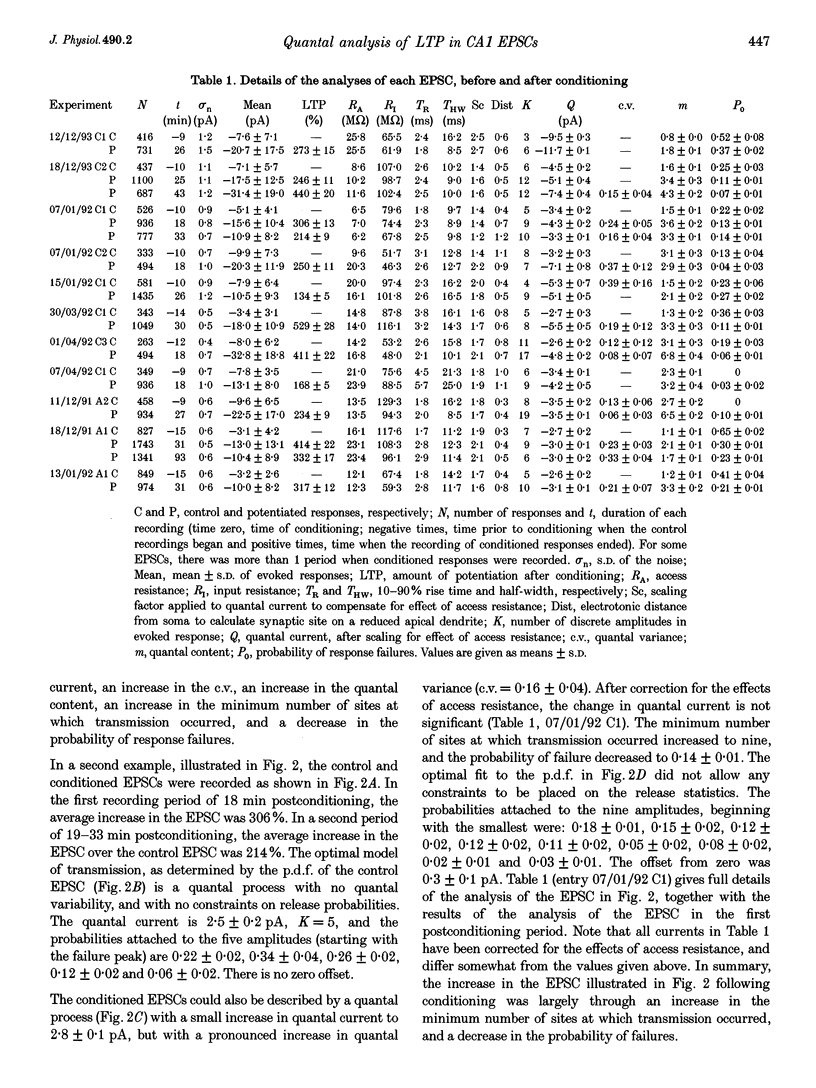
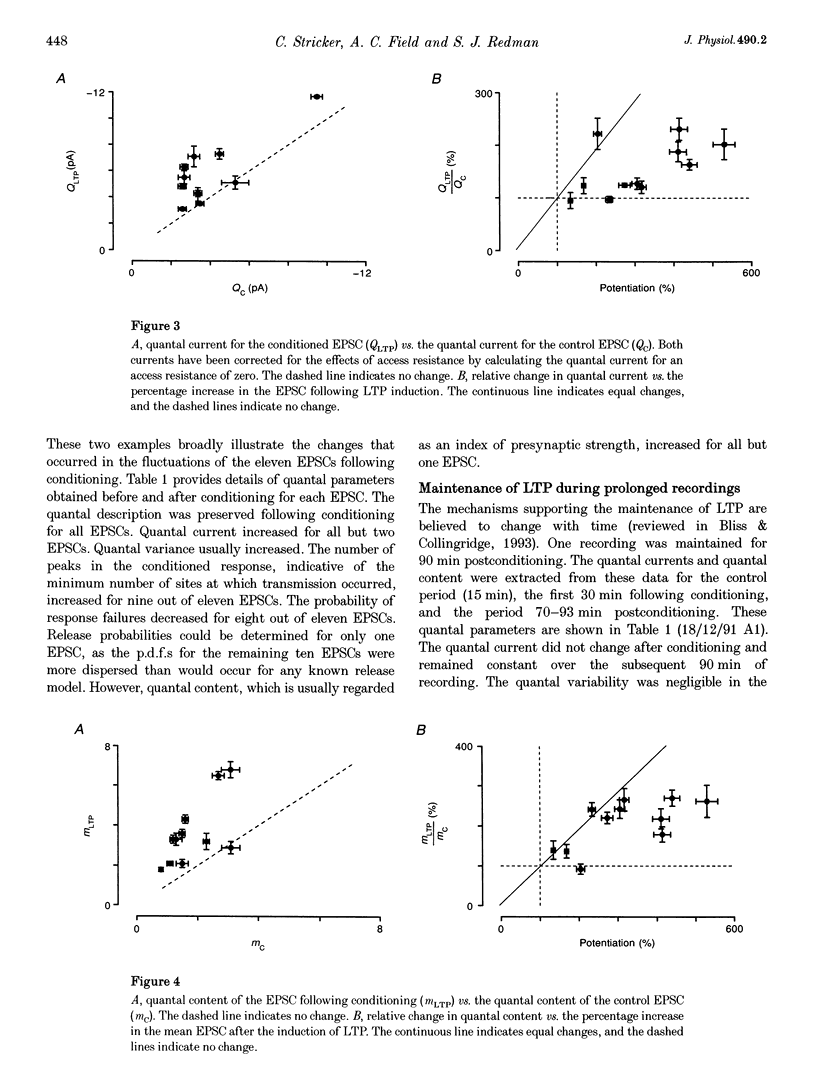
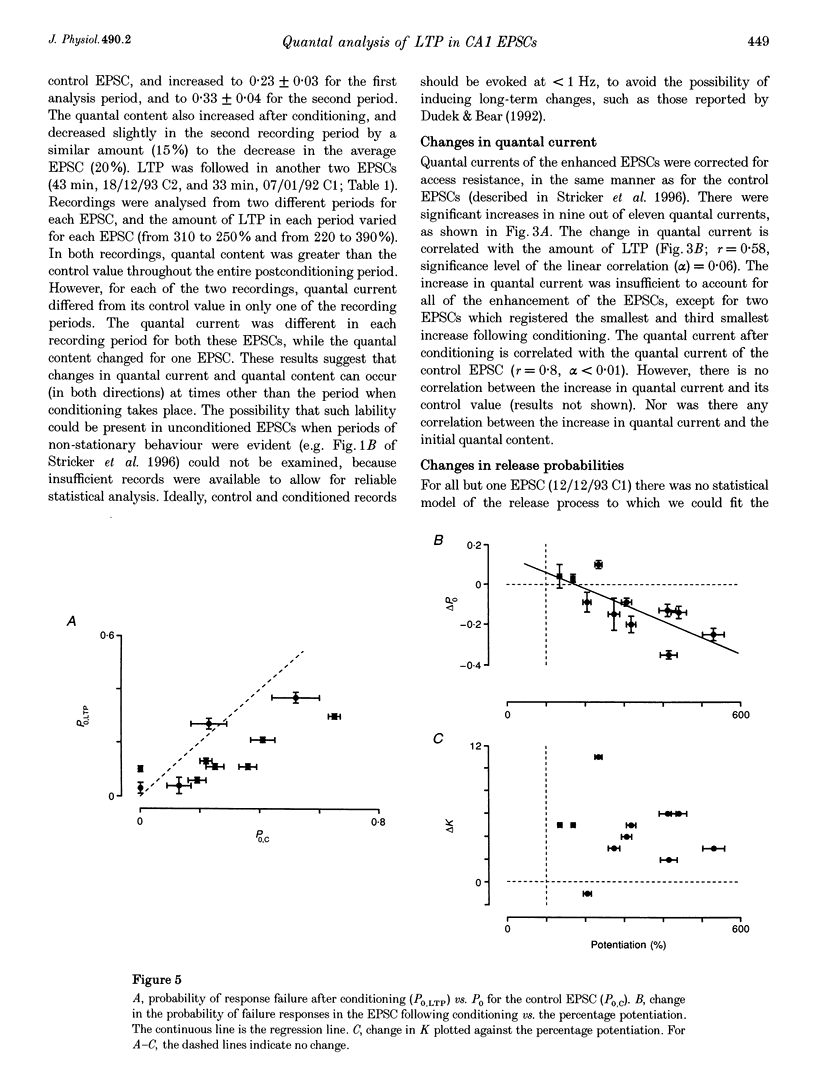
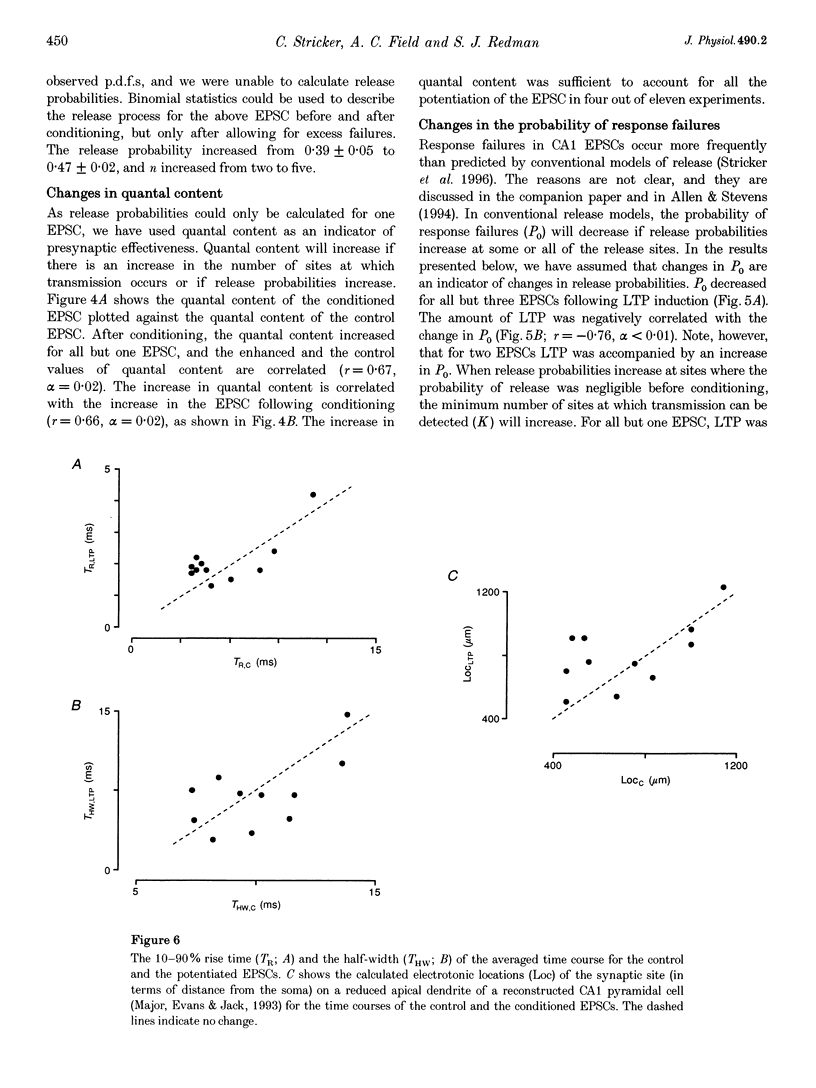
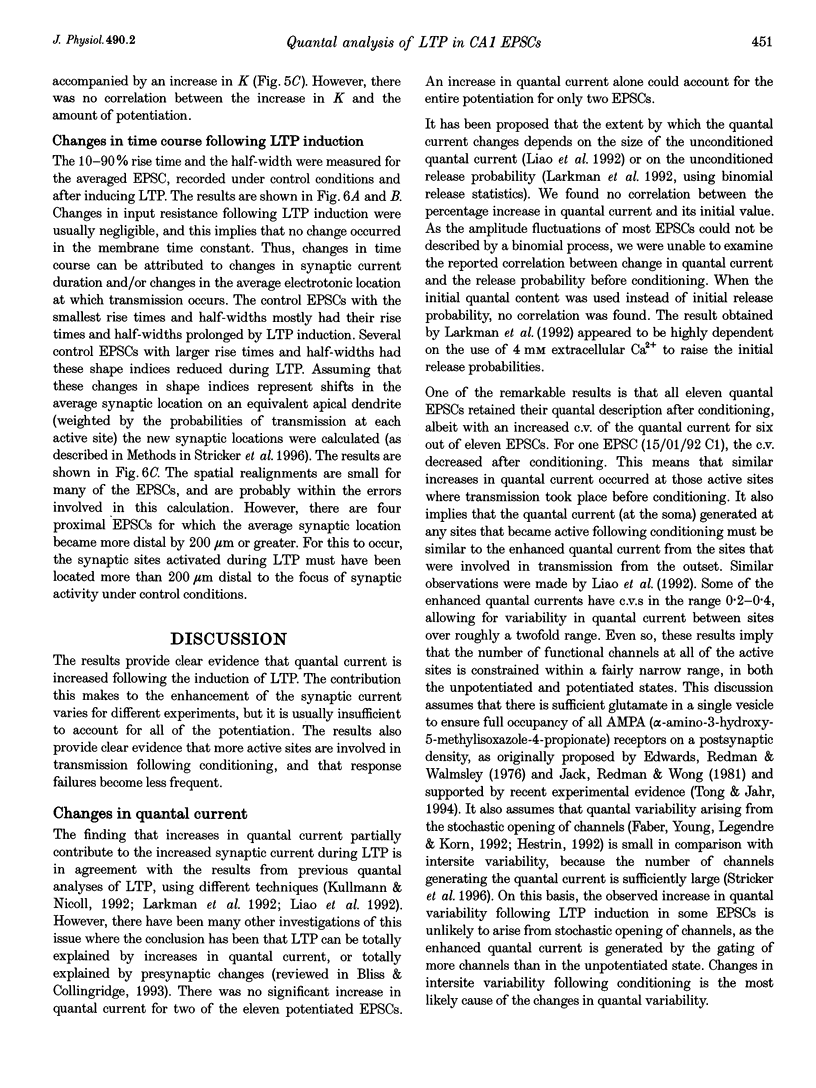
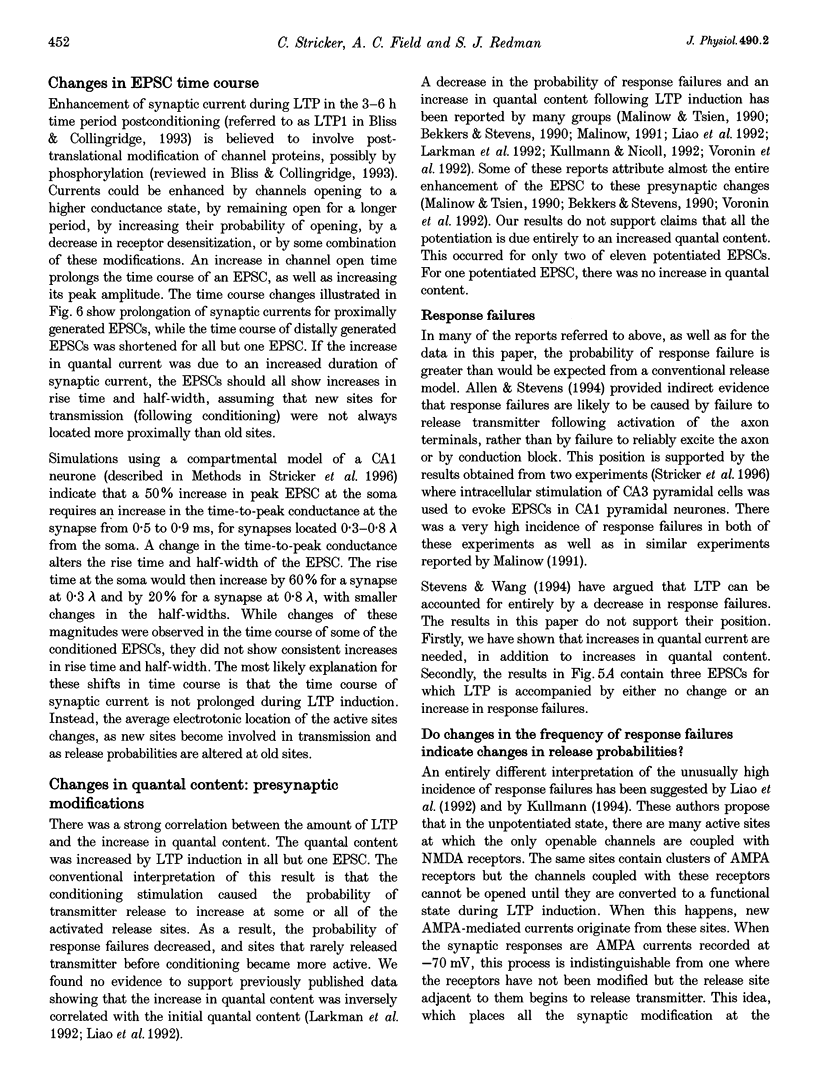
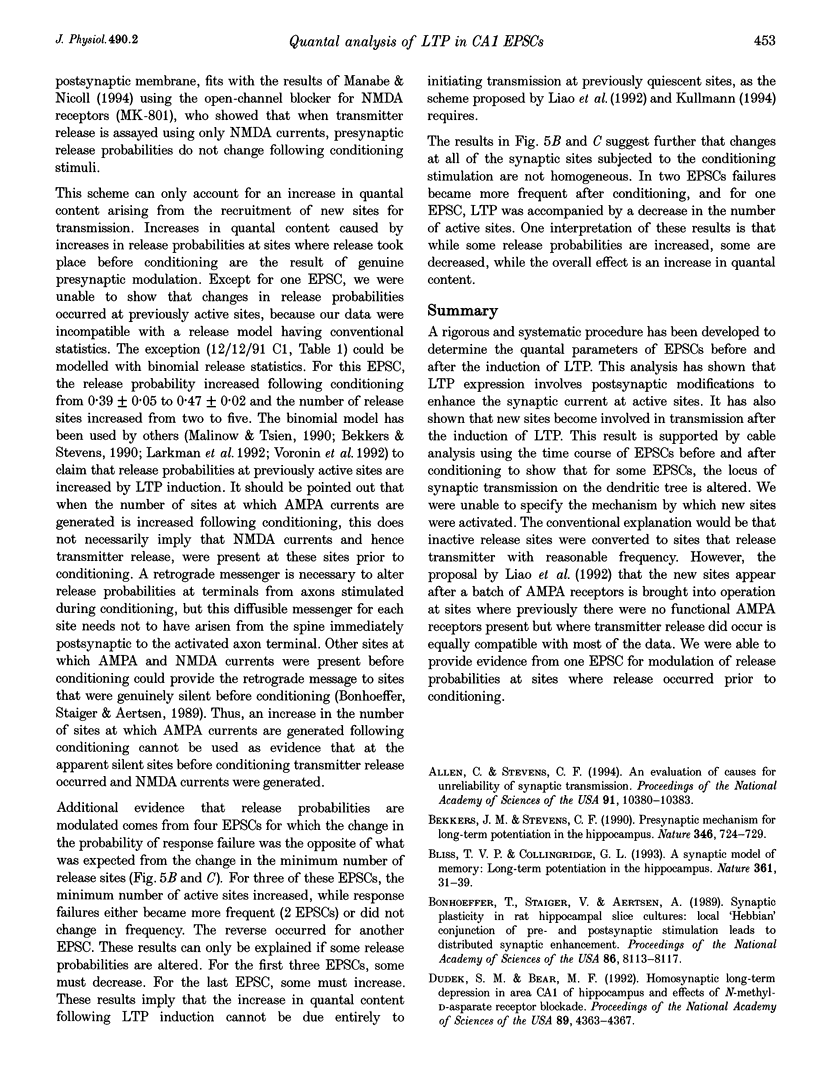
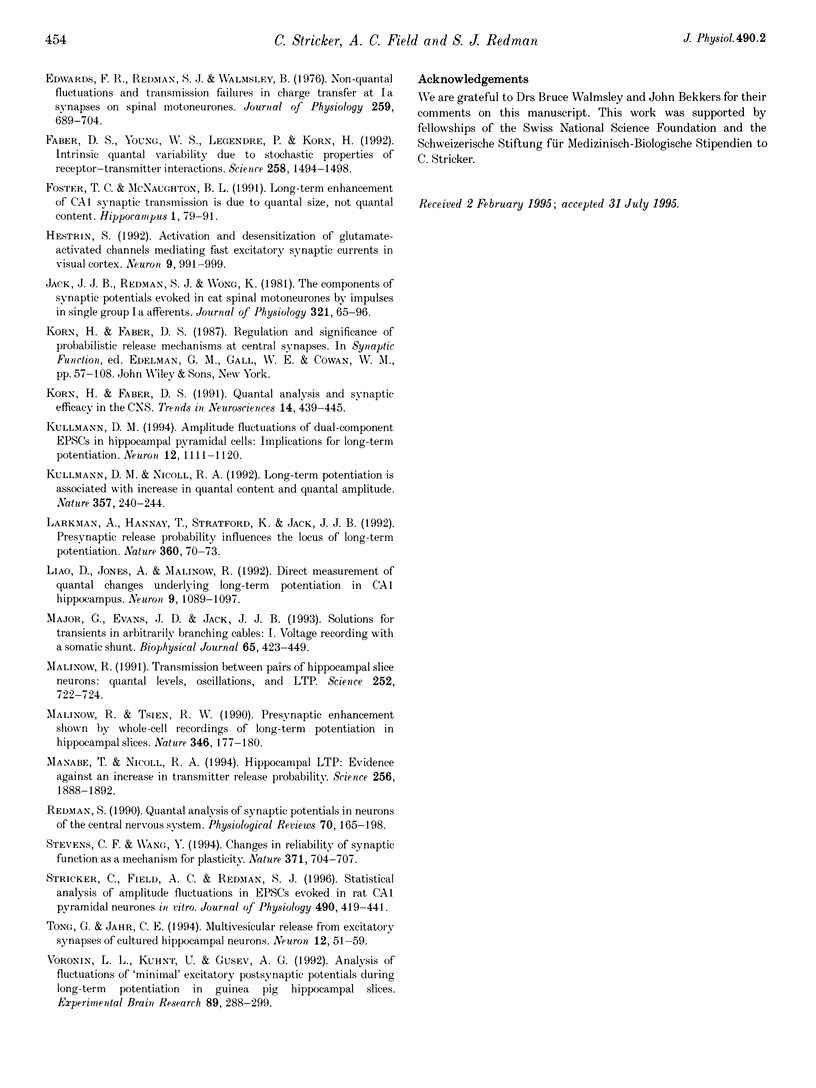
Selected References
These references are in PubMed. This may not be the complete list of references from this article.
- Allen C., Stevens C. F. An evaluation of causes for unreliability of synaptic transmission. Proc Natl Acad Sci U S A. 1994 Oct 25;91(22):10380–10383. doi: 10.1073/pnas.91.22.10380. [DOI] [PMC free article] [PubMed] [Google Scholar]
- Bekkers J. M., Stevens C. F. Presynaptic mechanism for long-term potentiation in the hippocampus. Nature. 1990 Aug 23;346(6286):724–729. doi: 10.1038/346724a0. [DOI] [PubMed] [Google Scholar]
- Bliss T. V., Collingridge G. L. A synaptic model of memory: long-term potentiation in the hippocampus. Nature. 1993 Jan 7;361(6407):31–39. doi: 10.1038/361031a0. [DOI] [PubMed] [Google Scholar]
- Bonhoeffer T., Staiger V., Aertsen A. Synaptic plasticity in rat hippocampal slice cultures: local "Hebbian" conjunction of pre- and postsynaptic stimulation leads to distributed synaptic enhancement. Proc Natl Acad Sci U S A. 1989 Oct;86(20):8113–8117. doi: 10.1073/pnas.86.20.8113. [DOI] [PMC free article] [PubMed] [Google Scholar]
- Dudek S. M., Bear M. F. Homosynaptic long-term depression in area CA1 of hippocampus and effects of N-methyl-D-aspartate receptor blockade. Proc Natl Acad Sci U S A. 1992 May 15;89(10):4363–4367. doi: 10.1073/pnas.89.10.4363. [DOI] [PMC free article] [PubMed] [Google Scholar]
- Edwards F. R., Redman S. J., Walmsley B. Non-quantal fluctuations and transmission failures in charge transfer at Ia synapses on spinal motoneurones. J Physiol. 1976 Aug;259(3):689–704. doi: 10.1113/jphysiol.1976.sp011489. [DOI] [PMC free article] [PubMed] [Google Scholar]
- Faber D. S., Young W. S., Legendre P., Korn H. Intrinsic quantal variability due to stochastic properties of receptor-transmitter interactions. Science. 1992 Nov 27;258(5087):1494–1498. doi: 10.1126/science.1279813. [DOI] [PubMed] [Google Scholar]
- Foster T. C., McNaughton B. L. Long-term enhancement of CA1 synaptic transmission is due to increased quantal size, not quantal content. Hippocampus. 1991 Jan;1(1):79–91. doi: 10.1002/hipo.450010108. [DOI] [PubMed] [Google Scholar]
- Hestrin S. Activation and desensitization of glutamate-activated channels mediating fast excitatory synaptic currents in the visual cortex. Neuron. 1992 Nov;9(5):991–999. doi: 10.1016/0896-6273(92)90250-h. [DOI] [PubMed] [Google Scholar]
- Jack J. J., Redman S. J., Wong K. The components of synaptic potentials evoked in cat spinal motoneurones by impulses in single group Ia afferents. J Physiol. 1981 Dec;321:65–96. doi: 10.1113/jphysiol.1981.sp013972. [DOI] [PMC free article] [PubMed] [Google Scholar]
- Korn H., Faber D. S. Quantal analysis and synaptic efficacy in the CNS. Trends Neurosci. 1991 Oct;14(10):439–445. doi: 10.1016/0166-2236(91)90042-s. [DOI] [PubMed] [Google Scholar]
- Kullmann D. M. Amplitude fluctuations of dual-component EPSCs in hippocampal pyramidal cells: implications for long-term potentiation. Neuron. 1994 May;12(5):1111–1120. doi: 10.1016/0896-6273(94)90318-2. [DOI] [PubMed] [Google Scholar]
- Kullmann D. M., Nicoll R. A. Long-term potentiation is associated with increases in quantal content and quantal amplitude. Nature. 1992 May 21;357(6375):240–244. doi: 10.1038/357240a0. [DOI] [PubMed] [Google Scholar]
- Larkman A., Hannay T., Stratford K., Jack J. Presynaptic release probability influences the locus of long-term potentiation. Nature. 1992 Nov 5;360(6399):70–73. doi: 10.1038/360070a0. [DOI] [PubMed] [Google Scholar]
- Liao D., Jones A., Malinow R. Direct measurement of quantal changes underlying long-term potentiation in CA1 hippocampus. Neuron. 1992 Dec;9(6):1089–1097. doi: 10.1016/0896-6273(92)90068-o. [DOI] [PubMed] [Google Scholar]
- Malinow R. Transmission between pairs of hippocampal slice neurons: quantal levels, oscillations, and LTP. Science. 1991 May 3;252(5006):722–724. doi: 10.1126/science.1850871. [DOI] [PubMed] [Google Scholar]
- Malinow R., Tsien R. W. Presynaptic enhancement shown by whole-cell recordings of long-term potentiation in hippocampal slices. Nature. 1990 Jul 12;346(6280):177–180. doi: 10.1038/346177a0. [DOI] [PubMed] [Google Scholar]
- Manabe T., Nicoll R. A. Long-term potentiation: evidence against an increase in transmitter release probability in the CA1 region of the hippocampus. Science. 1994 Sep 23;265(5180):1888–1892. doi: 10.1126/science.7916483. [DOI] [PubMed] [Google Scholar]
- Stevens C. F., Wang Y. Changes in reliability of synaptic function as a mechanism for plasticity. Nature. 1994 Oct 20;371(6499):704–707. doi: 10.1038/371704a0. [DOI] [PubMed] [Google Scholar]
- Stricker C., Field A. C., Redman S. J. Statistical analysis of amplitude fluctuations in EPSCs evoked in rat CA1 pyramidal neurones in vitro. J Physiol. 1996 Jan 15;490(Pt 2):419–441. doi: 10.1113/jphysiol.1996.sp021155. [DOI] [PMC free article] [PubMed] [Google Scholar]
- Tong G., Jahr C. E. Multivesicular release from excitatory synapses of cultured hippocampal neurons. Neuron. 1994 Jan;12(1):51–59. doi: 10.1016/0896-6273(94)90151-1. [DOI] [PubMed] [Google Scholar]
- Voronin L. L., Kuhnt U., Gusev A. G. Analysis of fluctuations of "minimal" excitatory postsynaptic potentials during long-term potentiation in guinea pig hippocampal slices. Exp Brain Res. 1992;89(2):288–299. doi: 10.1007/BF00228245. [DOI] [PubMed] [Google Scholar]