Abstract
1. The activation kinetics of the IRK1 channel stably expressed in L cells (a murine fibroblast cell line) were studied under the whole-cell voltage clamp. Without polyamines or Mg2+ in the pipettes, inward currents showed an exponential activation on hyperpolarization. The steep inward rectification of the currents around the reversal potential (Erev) could be described by the open-close transition of the channel with first-order kinetics. 2. When the tetravalent organic cation spermine (Spm) was added in the pipettes, the activation kinetics changed; this was explicable by the increase in the closing rate constant. The activation of the currents observed without Spm or Mg2+ in the pipettes was ascribed to the unblocking of the 'endogenous-Spm block'. 3. In the presence of the divalent cation putrescine (Put) or of Mg2+ in the pipettes, a different non-conductive state suppressed the outward currents on depolarization; the channels instantaneously changed to the open state on repolarization. As the depolarization was prolonged, this non-conductive state was replaced by the non-conductive state that shows an exponential activation on repolarization. This phenomenon was attributed to the redistribution of the channels from the Put- or Mg(2+)-blocked state to the 'endogenous Spm-blocked state' during depolarization. 4. In the presence of the trivalent cation spermidine (Spd) in the pipettes, two different non-conductive states occurred, showing a faster and a slower activation on repolarization. The rectification around Erev was mainly due to the non-conductive state showing a faster activation, which appeared to be the Spd-blocked state. During depolarization, redistribution of the channels to the 'endogenous Spm-blocked state' also occurred. 5. In the presence of Spd, Put or Mg2+ in the pipettes, the voltage dependence of the activation time constant reflecting the unblocking of the 'endogenous Spm' was shifted in the hyperpolarizing direction. 6. Our results suggest that the 'intrinsic gating' that shows the time-dependent activation on repolarization, and that is responsible for the inward rectification around Erev, reflects the blocking kinetics of the tetravalent Spm.
Full text
PDF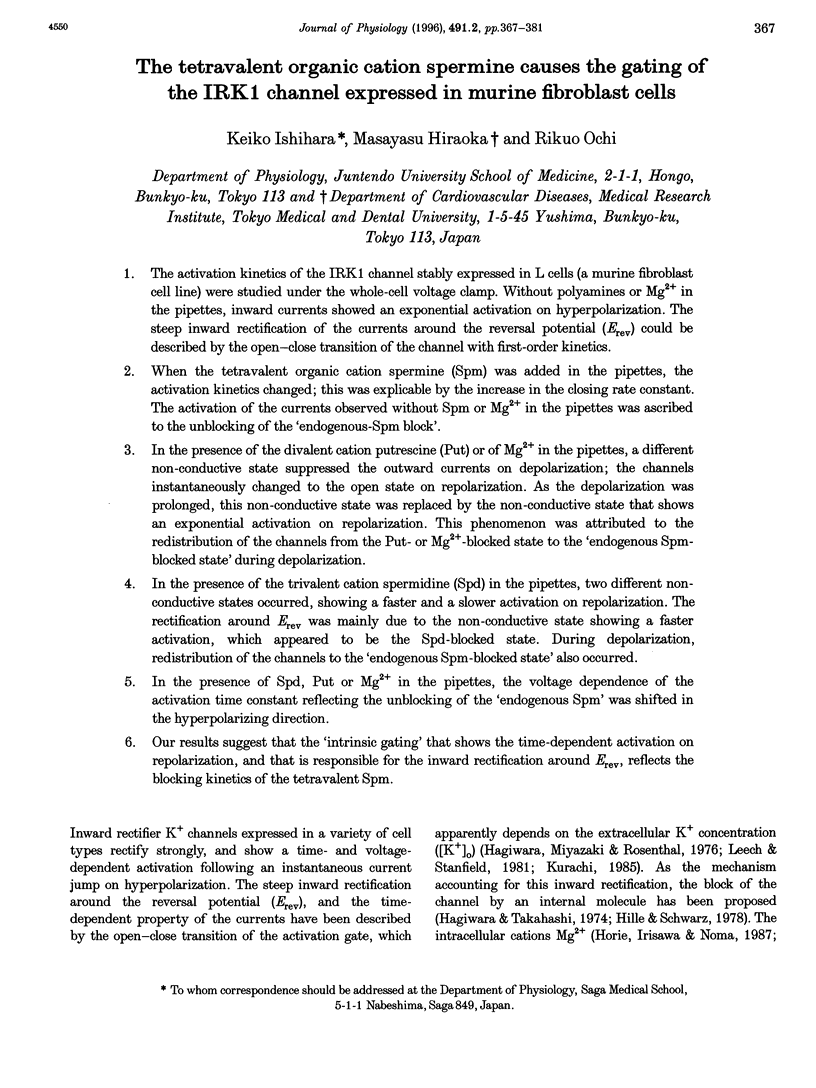
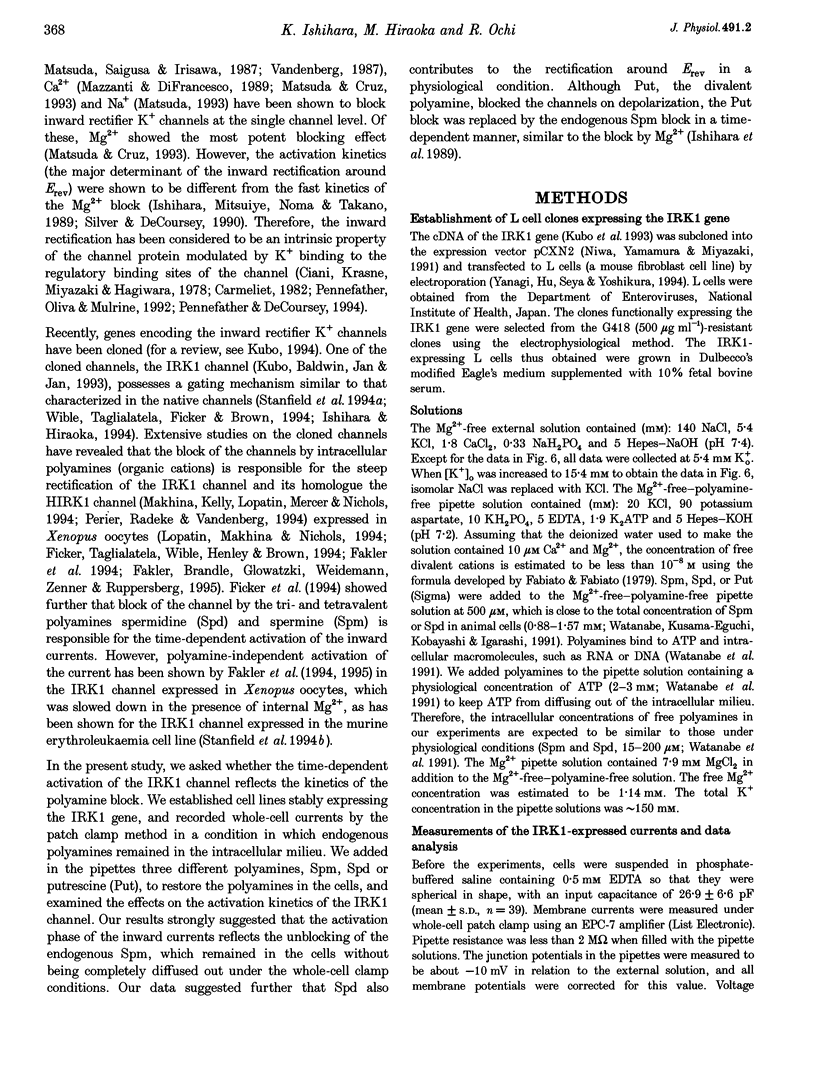
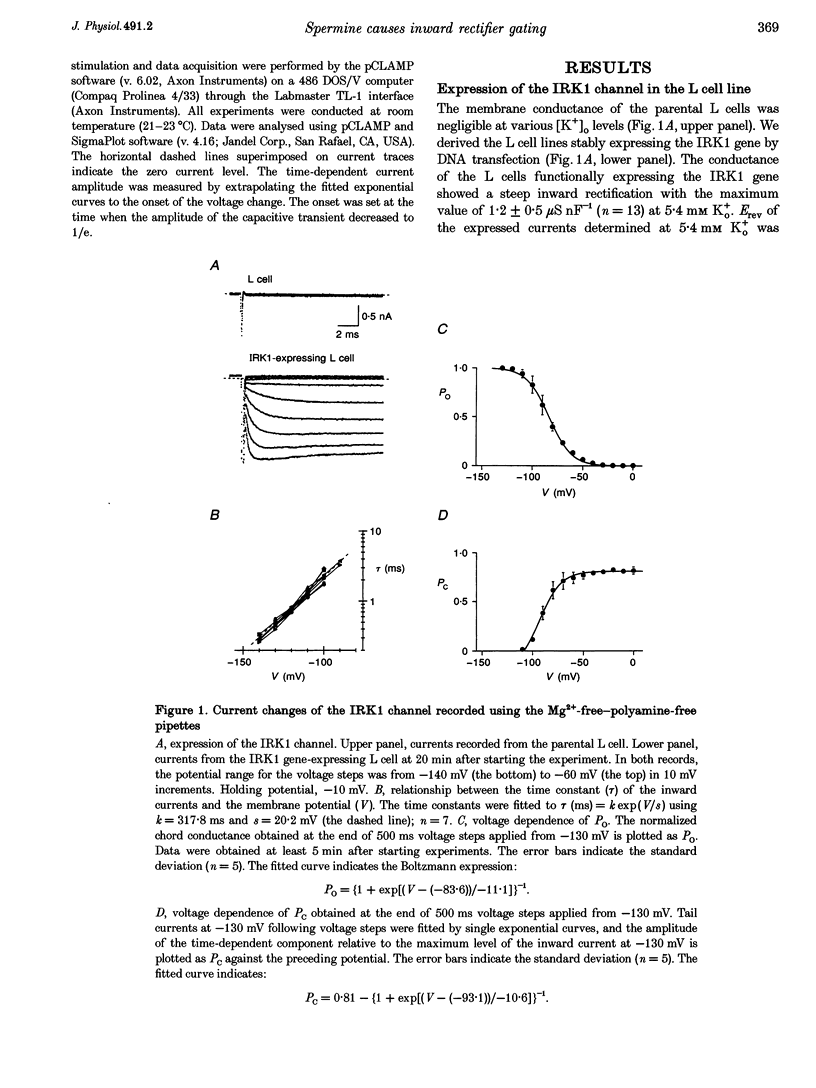
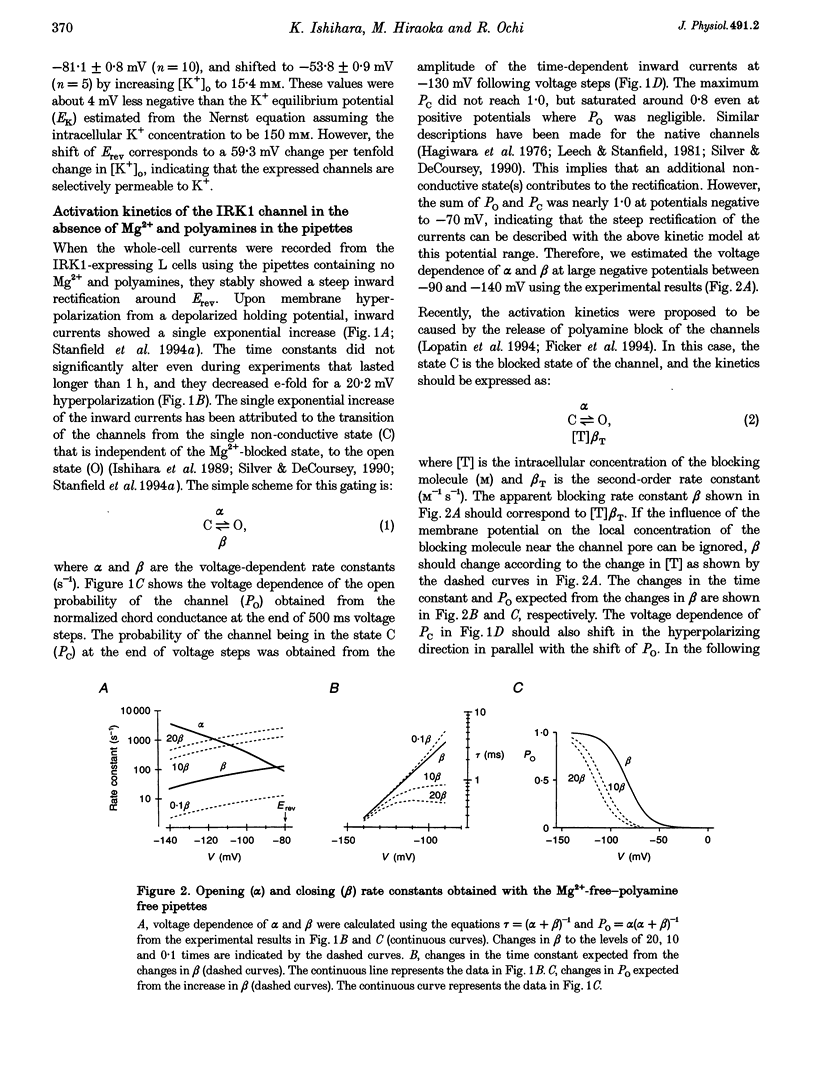
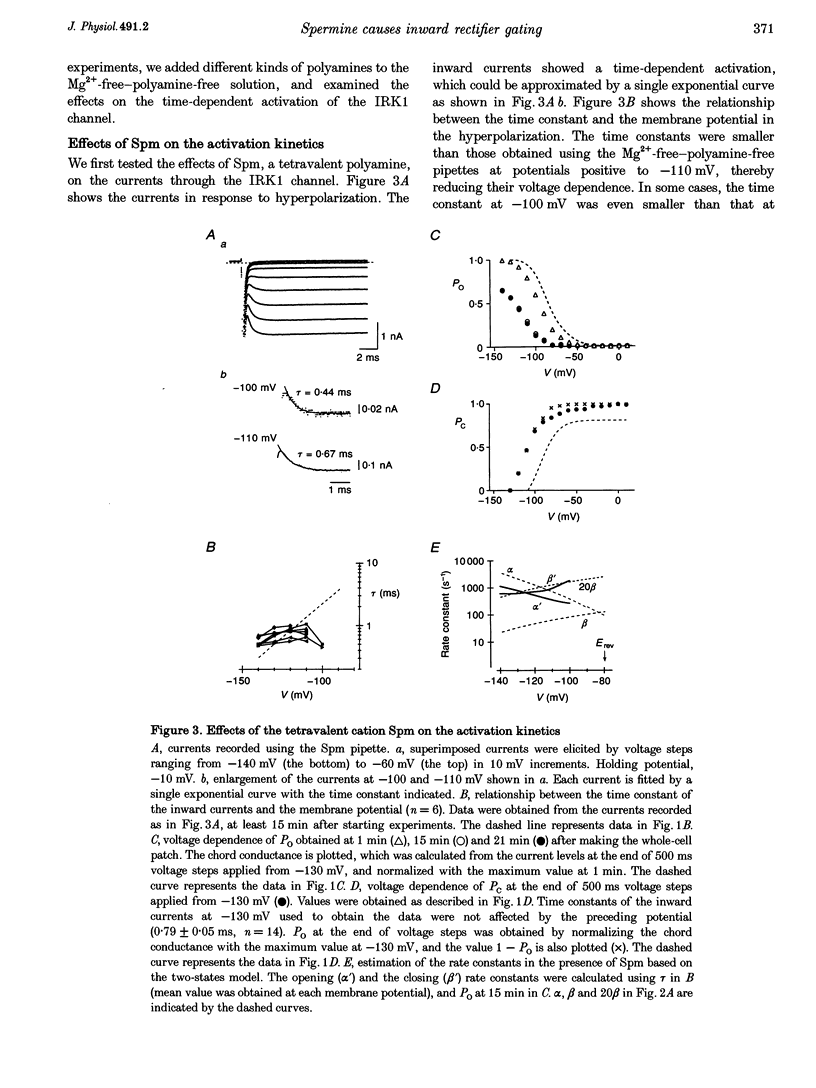
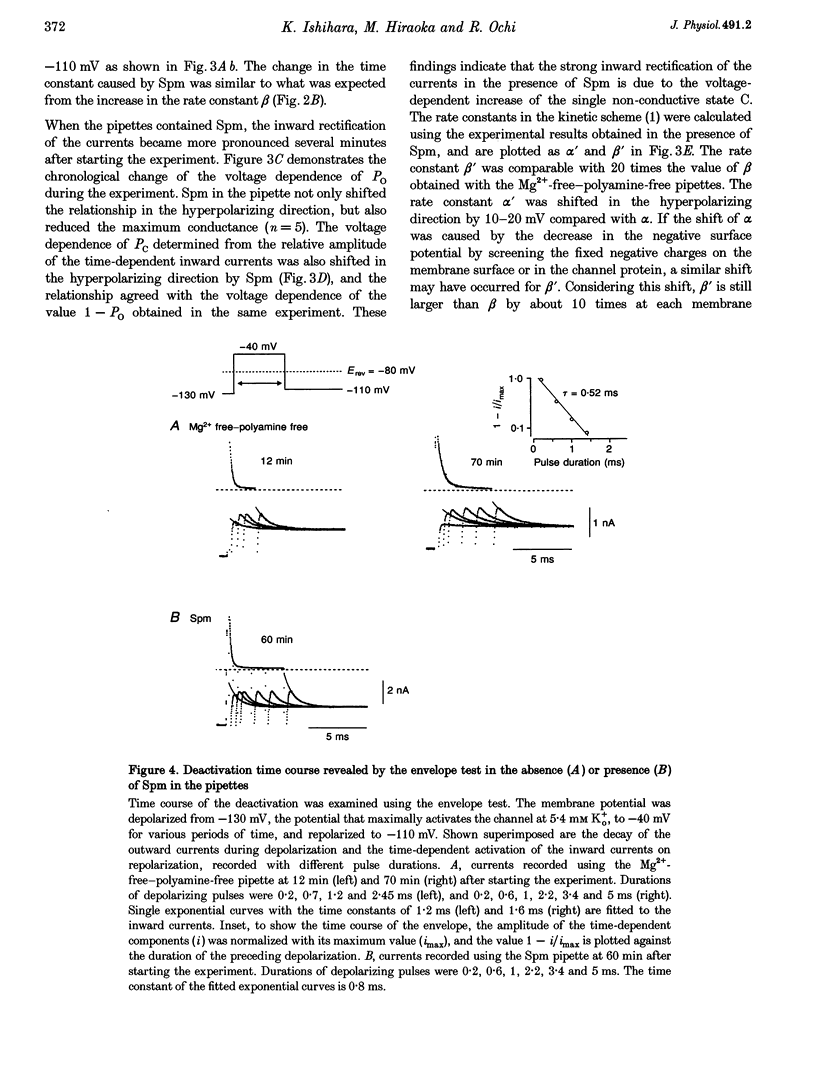
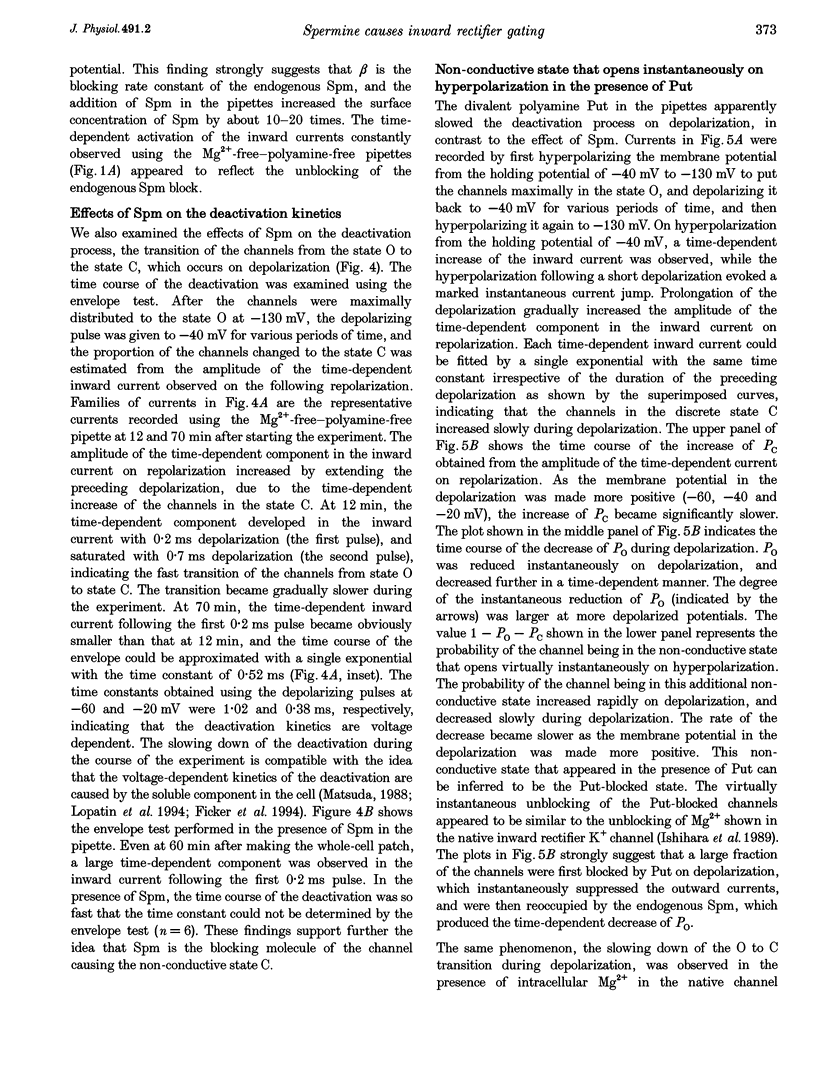
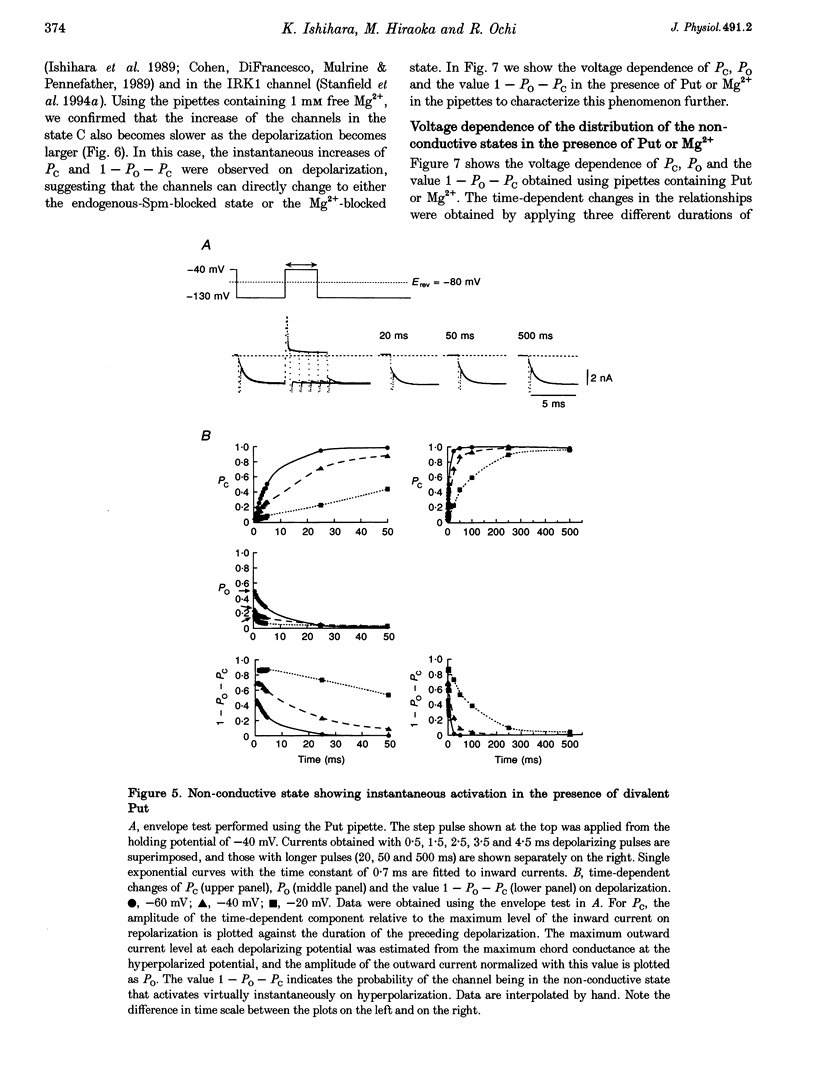
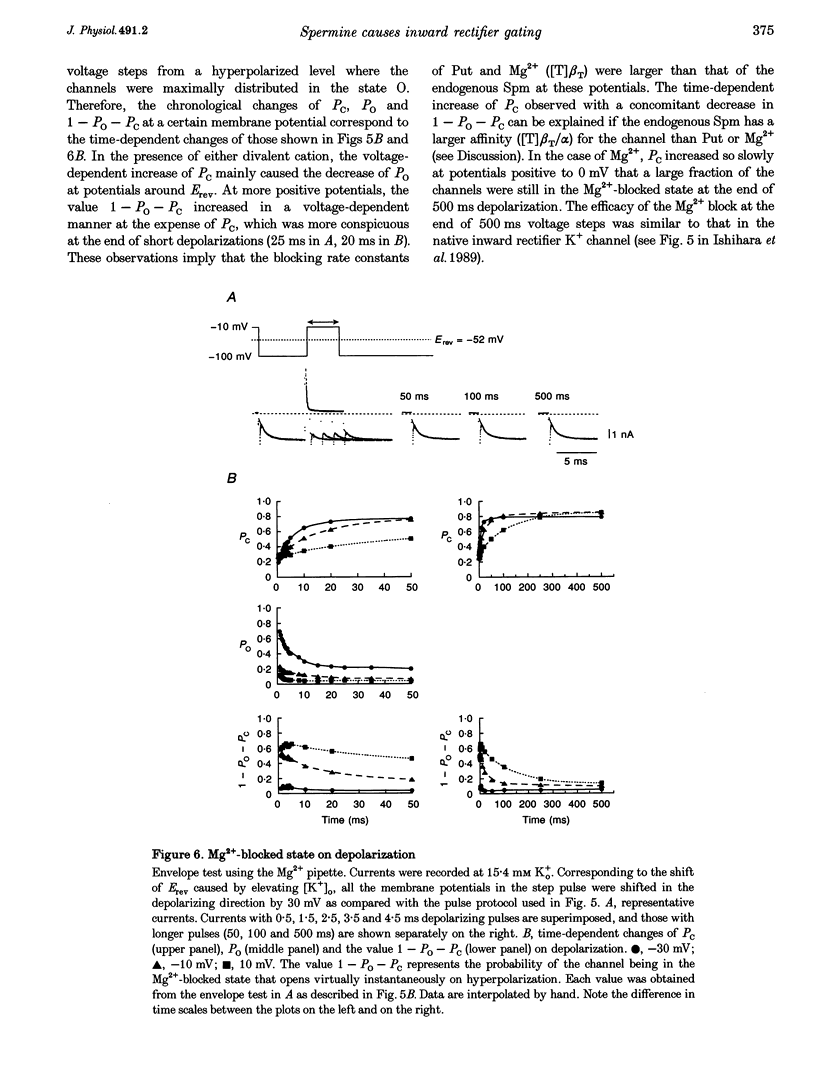
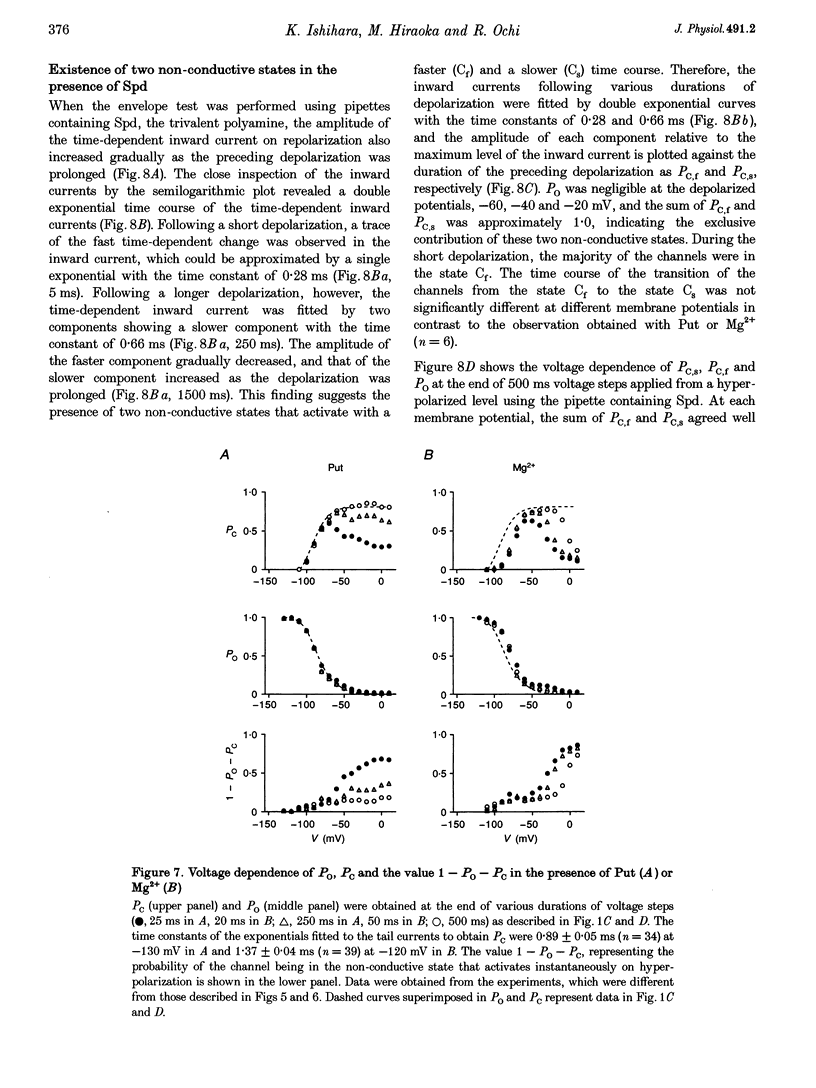
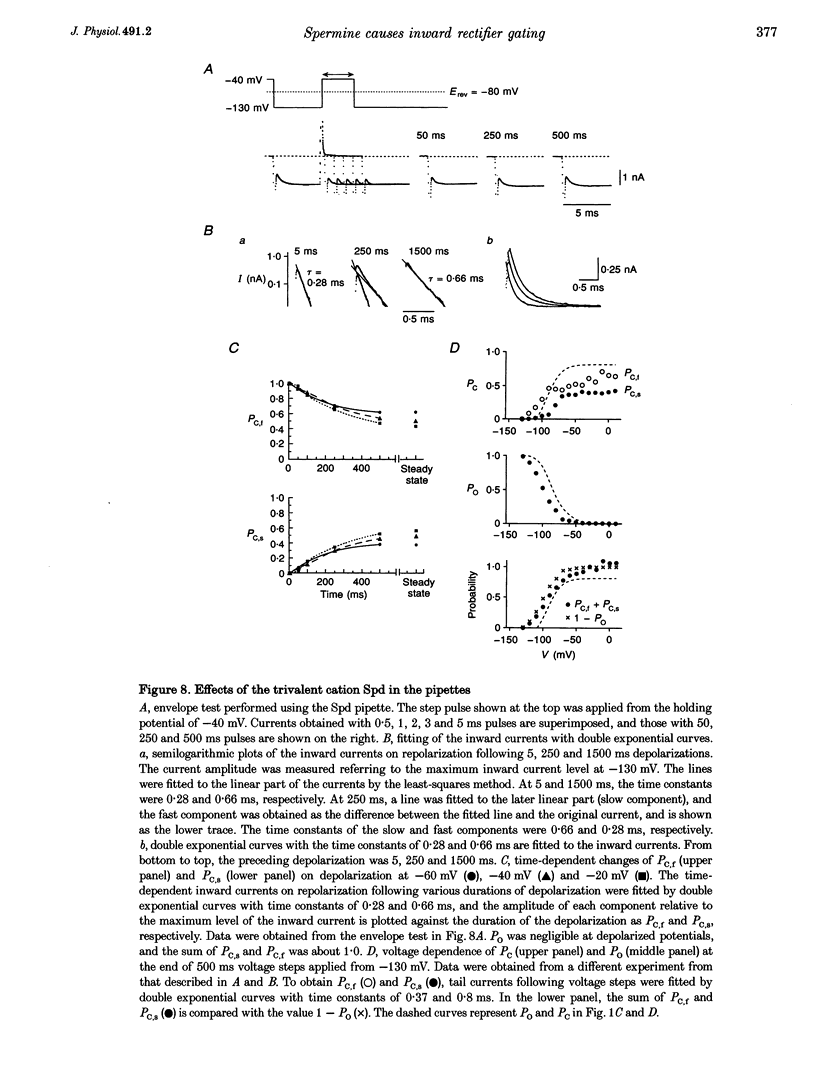
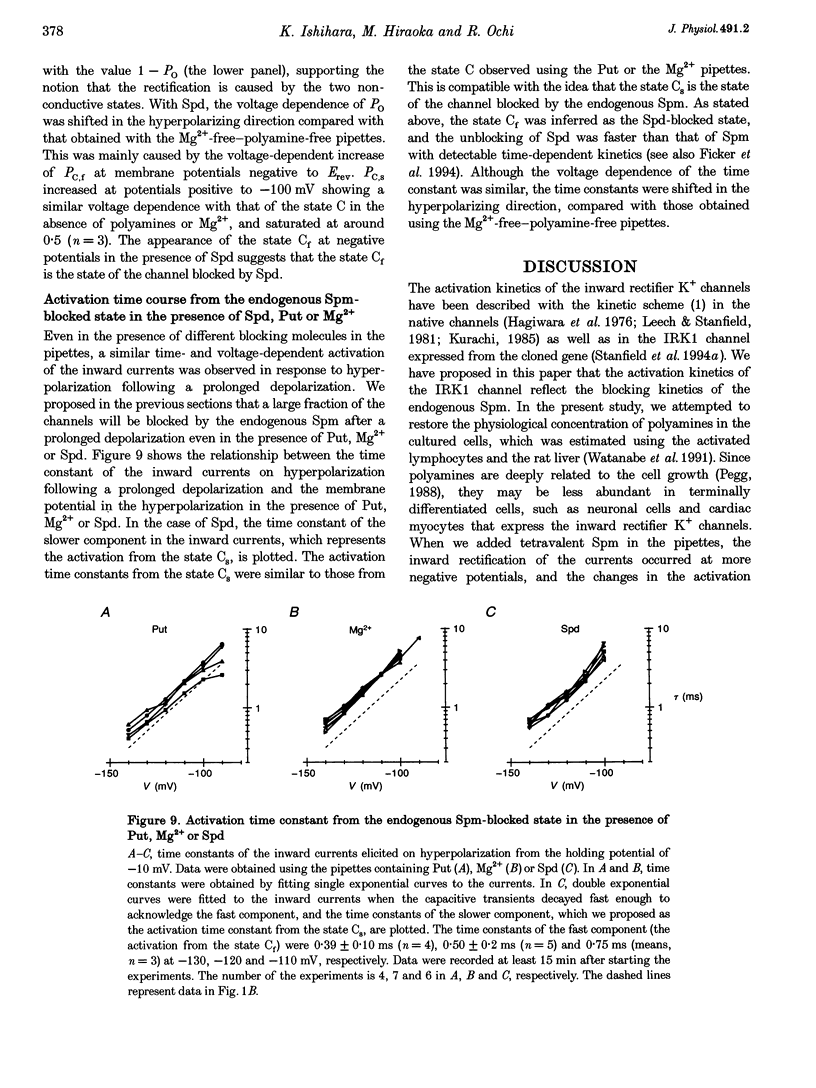
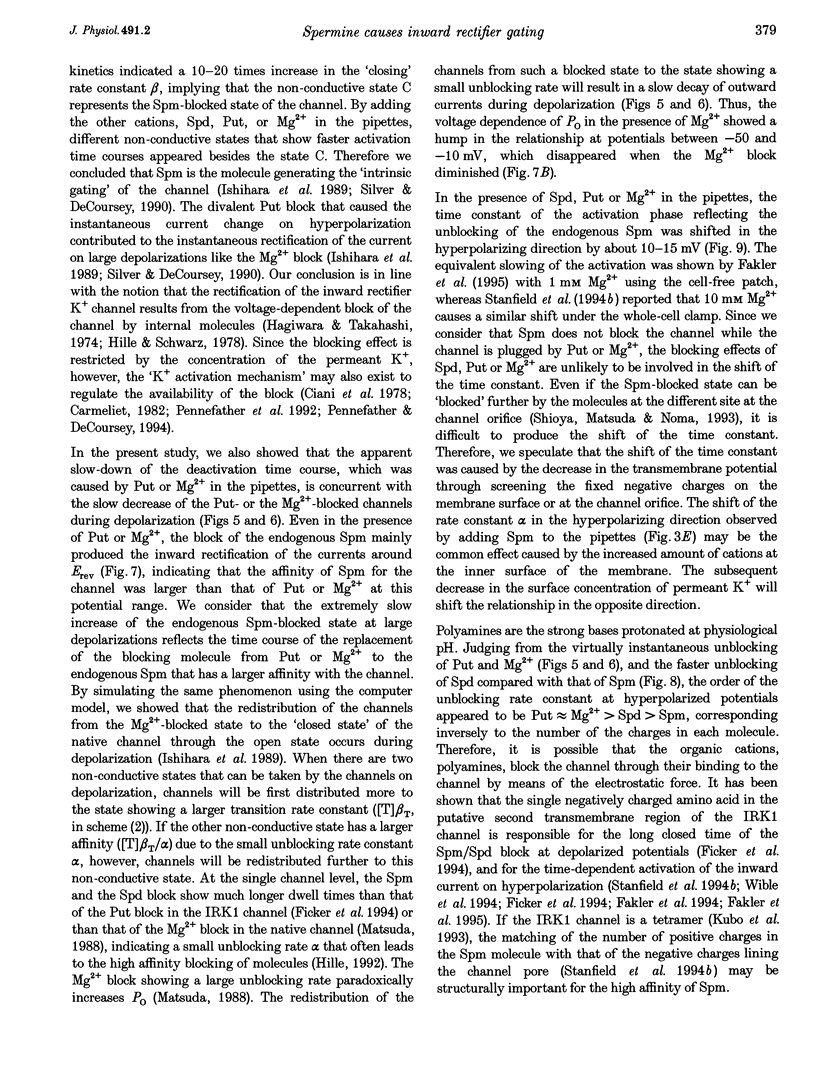
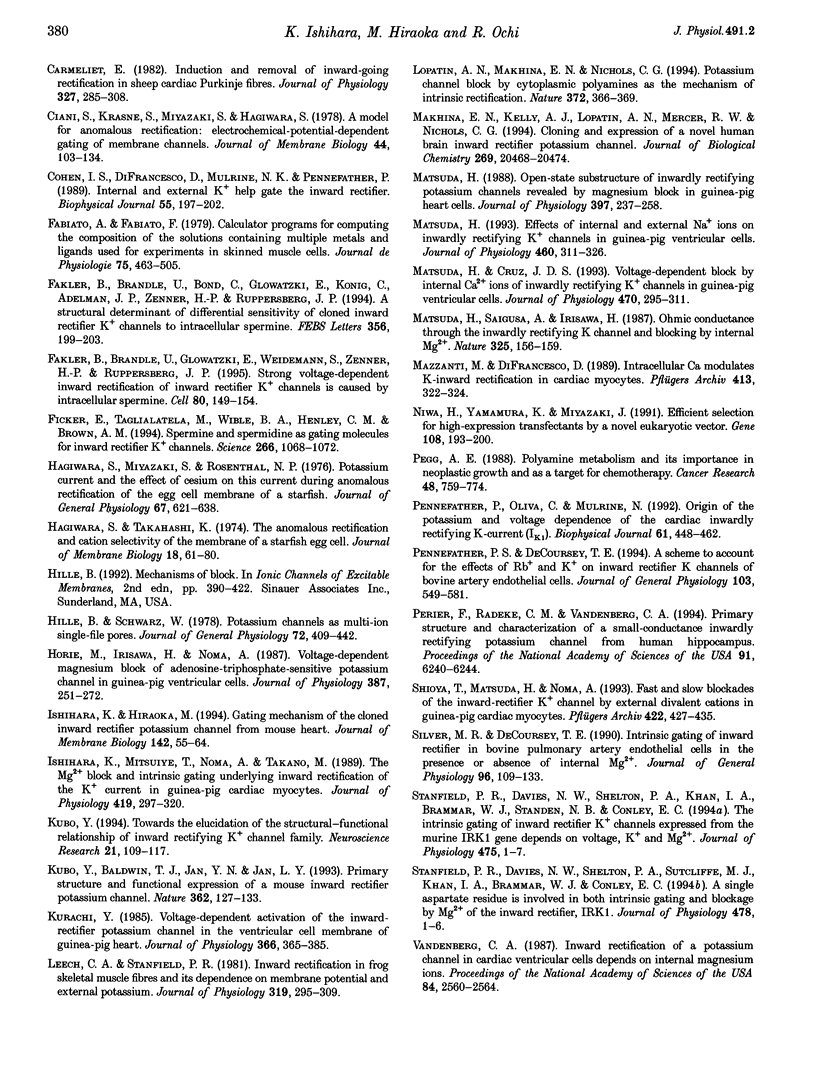
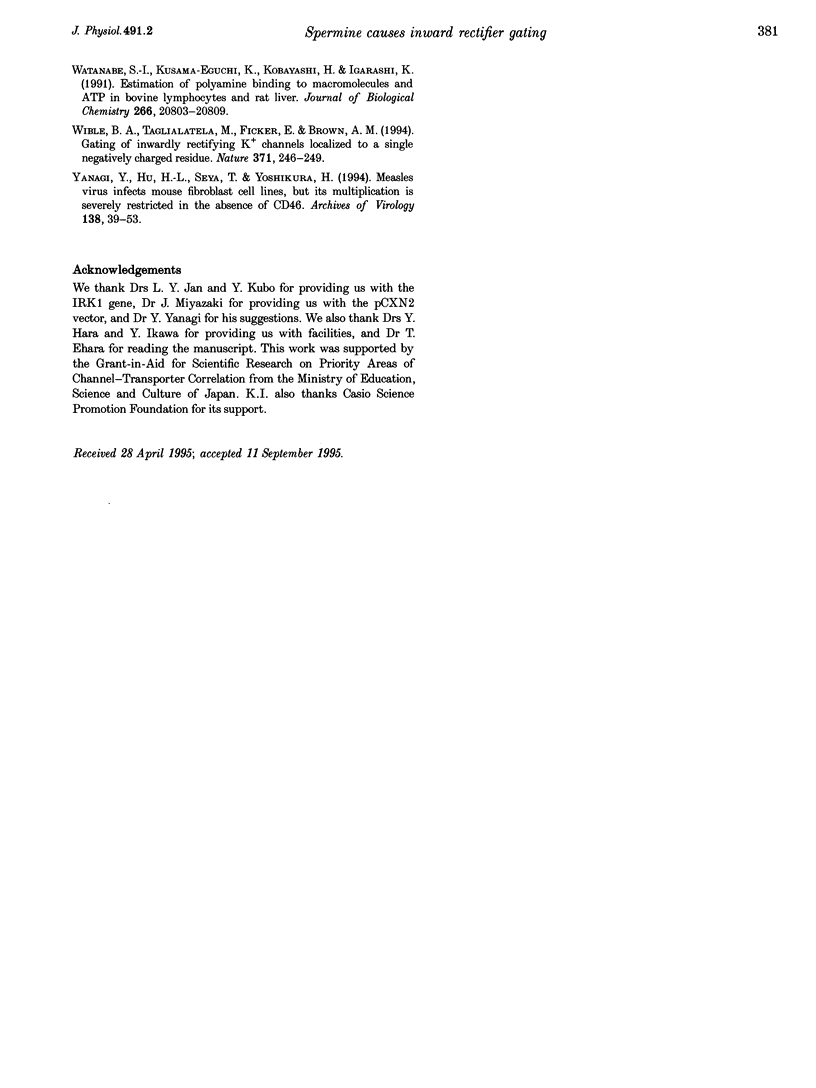
Selected References
These references are in PubMed. This may not be the complete list of references from this article.
- Carmeliet E. Induction and removal of inward-going rectification in sheep cardiac Purkinje fibres. J Physiol. 1982 Jun;327:285–308. doi: 10.1113/jphysiol.1982.sp014232. [DOI] [PMC free article] [PubMed] [Google Scholar]
- Ciani S., Krasne S., Miyazaki S., Hagiwara S. A model for anomalous rectification: electrochemical-potential-dependent gating of membrane channels. J Membr Biol. 1978 Dec 15;44(2):103–134. doi: 10.1007/BF01976035. [DOI] [PubMed] [Google Scholar]
- Cohen I. S., DiFrancesco D., Mulrine N. K., Pennefather P. Internal and external K+ help gate the inward rectifier. Biophys J. 1989 Jan;55(1):197–202. doi: 10.1016/S0006-3495(89)82792-4. [DOI] [PMC free article] [PubMed] [Google Scholar]
- Fabiato A., Fabiato F. Calculator programs for computing the composition of the solutions containing multiple metals and ligands used for experiments in skinned muscle cells. J Physiol (Paris) 1979;75(5):463–505. [PubMed] [Google Scholar]
- Fakler B., Brändle U., Bond C., Glowatzki E., König C., Adelman J. P., Zenner H. P., Ruppersberg J. P. A structural determinant of differential sensitivity of cloned inward rectifier K+ channels to intracellular spermine. FEBS Lett. 1994 Dec 19;356(2-3):199–203. doi: 10.1016/0014-5793(94)01258-x. [DOI] [PubMed] [Google Scholar]
- Fakler B., Brändle U., Glowatzki E., Weidemann S., Zenner H. P., Ruppersberg J. P. Strong voltage-dependent inward rectification of inward rectifier K+ channels is caused by intracellular spermine. Cell. 1995 Jan 13;80(1):149–154. doi: 10.1016/0092-8674(95)90459-x. [DOI] [PubMed] [Google Scholar]
- Ficker E., Taglialatela M., Wible B. A., Henley C. M., Brown A. M. Spermine and spermidine as gating molecules for inward rectifier K+ channels. Science. 1994 Nov 11;266(5187):1068–1072. doi: 10.1126/science.7973666. [DOI] [PubMed] [Google Scholar]
- Hagiwara S., Miyazaki S., Rosenthal N. P. Potassium current and the effect of cesium on this current during anomalous rectification of the egg cell membrane of a starfish. J Gen Physiol. 1976 Jun;67(6):621–638. doi: 10.1085/jgp.67.6.621. [DOI] [PMC free article] [PubMed] [Google Scholar]
- Hagiwara S., Takahashi K. The anomalous rectification and cation selectivity of the membrane of a starfish egg cell. J Membr Biol. 1974;18(1):61–80. doi: 10.1007/BF01870103. [DOI] [PubMed] [Google Scholar]
- Hille B., Schwarz W. Potassium channels as multi-ion single-file pores. J Gen Physiol. 1978 Oct;72(4):409–442. doi: 10.1085/jgp.72.4.409. [DOI] [PMC free article] [PubMed] [Google Scholar]
- Horie M., Irisawa H., Noma A. Voltage-dependent magnesium block of adenosine-triphosphate-sensitive potassium channel in guinea-pig ventricular cells. J Physiol. 1987 Jun;387:251–272. doi: 10.1113/jphysiol.1987.sp016572. [DOI] [PMC free article] [PubMed] [Google Scholar]
- Ishihara K., Hiraoka M. Gating mechanism of the cloned inward rectifier potassium channel from mouse heart. J Membr Biol. 1994 Oct;142(1):55–64. doi: 10.1007/BF00233383. [DOI] [PubMed] [Google Scholar]
- Ishihara K., Mitsuiye T., Noma A., Takano M. The Mg2+ block and intrinsic gating underlying inward rectification of the K+ current in guinea-pig cardiac myocytes. J Physiol. 1989 Dec;419:297–320. doi: 10.1113/jphysiol.1989.sp017874. [DOI] [PMC free article] [PubMed] [Google Scholar]
- Kubo Y., Baldwin T. J., Jan Y. N., Jan L. Y. Primary structure and functional expression of a mouse inward rectifier potassium channel. Nature. 1993 Mar 11;362(6416):127–133. doi: 10.1038/362127a0. [DOI] [PubMed] [Google Scholar]
- Kubo Y. Towards the elucidation of the structural-functional relationship of inward rectifying K+ channel family. Neurosci Res. 1994 Dec;21(2):109–117. doi: 10.1016/0168-0102(94)90152-x. [DOI] [PubMed] [Google Scholar]
- Kurachi Y. Voltage-dependent activation of the inward-rectifier potassium channel in the ventricular cell membrane of guinea-pig heart. J Physiol. 1985 Sep;366:365–385. doi: 10.1113/jphysiol.1985.sp015803. [DOI] [PMC free article] [PubMed] [Google Scholar]
- Leech C. A., Stanfield P. R. Inward rectification in frog skeletal muscle fibres and its dependence on membrane potential and external potassium. J Physiol. 1981;319:295–309. doi: 10.1113/jphysiol.1981.sp013909. [DOI] [PMC free article] [PubMed] [Google Scholar]
- Lopatin A. N., Makhina E. N., Nichols C. G. Potassium channel block by cytoplasmic polyamines as the mechanism of intrinsic rectification. Nature. 1994 Nov 24;372(6504):366–369. doi: 10.1038/372366a0. [DOI] [PubMed] [Google Scholar]
- Makhina E. N., Kelly A. J., Lopatin A. N., Mercer R. W., Nichols C. G. Cloning and expression of a novel human brain inward rectifier potassium channel. J Biol Chem. 1994 Aug 12;269(32):20468–20474. [PubMed] [Google Scholar]
- Matsuda H., Cruz J. dos S. Voltage-dependent block by internal Ca2+ ions of inwardly rectifying K+ channels in guinea-pig ventricular cells. J Physiol. 1993 Oct;470:295–311. doi: 10.1113/jphysiol.1993.sp019859. [DOI] [PMC free article] [PubMed] [Google Scholar]
- Matsuda H. Effects of internal and external Na+ ions on inwardly rectifying K+ channels in guinea-pig ventricular cells. J Physiol. 1993 Jan;460:311–326. doi: 10.1113/jphysiol.1993.sp019473. [DOI] [PMC free article] [PubMed] [Google Scholar]
- Matsuda H. Open-state substructure of inwardly rectifying potassium channels revealed by magnesium block in guinea-pig heart cells. J Physiol. 1988 Mar;397:237–258. doi: 10.1113/jphysiol.1988.sp016998. [DOI] [PMC free article] [PubMed] [Google Scholar]
- Matsuda H., Saigusa A., Irisawa H. Ohmic conductance through the inwardly rectifying K channel and blocking by internal Mg2+. Nature. 1987 Jan 8;325(7000):156–159. doi: 10.1038/325156a0. [DOI] [PubMed] [Google Scholar]
- Mazzanti M., DiFrancesco D. Intracellular Ca modulates K-inward rectification in cardiac myocytes. Pflugers Arch. 1989 Jan;413(3):322–324. doi: 10.1007/BF00583549. [DOI] [PubMed] [Google Scholar]
- Niwa H., Yamamura K., Miyazaki J. Efficient selection for high-expression transfectants with a novel eukaryotic vector. Gene. 1991 Dec 15;108(2):193–199. doi: 10.1016/0378-1119(91)90434-d. [DOI] [PubMed] [Google Scholar]
- Pegg A. E. Polyamine metabolism and its importance in neoplastic growth and a target for chemotherapy. Cancer Res. 1988 Feb 15;48(4):759–774. [PubMed] [Google Scholar]
- Pennefather P. S., DeCoursey T. E. A scheme to account for the effects of Rb+ and K+ on inward rectifier K channels of bovine artery endothelial cells. J Gen Physiol. 1994 Apr;103(4):549–581. doi: 10.1085/jgp.103.4.549. [DOI] [PMC free article] [PubMed] [Google Scholar]
- Pennefather P., Oliva C., Mulrine N. Origin of the potassium and voltage dependence of the cardiac inwardly rectifying K-current (IK1). Biophys J. 1992 Feb;61(2):448–462. doi: 10.1016/S0006-3495(92)81850-7. [DOI] [PMC free article] [PubMed] [Google Scholar]
- Périer F., Radeke C. M., Vandenberg C. A. Primary structure and characterization of a small-conductance inwardly rectifying potassium channel from human hippocampus. Proc Natl Acad Sci U S A. 1994 Jun 21;91(13):6240–6244. doi: 10.1073/pnas.91.13.6240. [DOI] [PMC free article] [PubMed] [Google Scholar]
- Shioya T., Matsuda H., Noma A. Fast and slow blockades of the inward-rectifier K+ channel by external divalent cations in guinea-pig cardiac myocytes. Pflugers Arch. 1993 Feb;422(5):427–435. doi: 10.1007/BF00375067. [DOI] [PubMed] [Google Scholar]
- Silver M. R., DeCoursey T. E. Intrinsic gating of inward rectifier in bovine pulmonary artery endothelial cells in the presence or absence of internal Mg2+. J Gen Physiol. 1990 Jul;96(1):109–133. doi: 10.1085/jgp.96.1.109. [DOI] [PMC free article] [PubMed] [Google Scholar]
- Stanfield P. R., Davies N. W., Shelton P. A., Khan I. A., Brammar W. J., Standen N. B., Conley E. C. The intrinsic gating of inward rectifier K+ channels expressed from the murine IRK1 gene depends on voltage, K+ and Mg2+. J Physiol. 1994 Feb 15;475(1):1–7. doi: 10.1113/jphysiol.1994.sp020044. [DOI] [PMC free article] [PubMed] [Google Scholar]
- Stanfield P. R., Davies N. W., Shelton P. A., Sutcliffe M. J., Khan I. A., Brammar W. J., Conley E. C. A single aspartate residue is involved in both intrinsic gating and blockage by Mg2+ of the inward rectifier, IRK1. J Physiol. 1994 Jul 1;478(Pt 1):1–6. doi: 10.1113/jphysiol.1994.sp020225. [DOI] [PMC free article] [PubMed] [Google Scholar]
- Vandenberg C. A. Inward rectification of a potassium channel in cardiac ventricular cells depends on internal magnesium ions. Proc Natl Acad Sci U S A. 1987 Apr;84(8):2560–2564. doi: 10.1073/pnas.84.8.2560. [DOI] [PMC free article] [PubMed] [Google Scholar]
- Watanabe S., Kusama-Eguchi K., Kobayashi H., Igarashi K. Estimation of polyamine binding to macromolecules and ATP in bovine lymphocytes and rat liver. J Biol Chem. 1991 Nov 5;266(31):20803–20809. [PubMed] [Google Scholar]
- Wible B. A., Taglialatela M., Ficker E., Brown A. M. Gating of inwardly rectifying K+ channels localized to a single negatively charged residue. Nature. 1994 Sep 15;371(6494):246–249. doi: 10.1038/371246a0. [DOI] [PubMed] [Google Scholar]
- Yanagi Y., Hu H. L., Seya T., Yoshikura H. Measles virus infects mouse fibroblast cell lines, but its multiplication is severely restricted in the absence of CD46. Arch Virol. 1994;138(1-2):39–53. doi: 10.1007/BF01310037. [DOI] [PubMed] [Google Scholar]