Abstract
1. Interspike interval distributions from human motor units of a variety of muscles were analysed to assess the role of synaptic noise in excitation. The time course of the underlying post-spike after-hyperpolarization (AHP) was deduced by applying a specially developed transform to the interval data. Different firing rates were studied both by varying the firing voluntarily, and by selecting subpopulations of spikes for a given firing rate from long recordings with slight variations in frequency. 2. At low firing rates the interval histograms had an exponential tail. Thus at long intervals, the motoneurone was randomly excited by noise and its post-spike AHP was complete. This contrasts with the firing produced by intracellular current injection in the cat, when the membrane potential increases linearly until threshold is reached. The interval histogram was therefore analysed with the aid of a model of synaptic excitation to deduce the mean 'trajectory' of membrane voltage in the last part of the interspike interval. 3. The computer model, described in the Appendix, was used to determine the effect of the mean level of membrane potential on the probability of a spike being excited, per unit time, during an on-going interspike interval. All variables were treated as voltages, with synaptic noise simulated by time-smoothed Gaussian noise. This enabled an interval distribution to be transformed into a segment of the underlying trajectory of the membrane potential; the potential was expressed in terms of the noise amplitude and the spike threshold. 4. At low firing rates, the equilibrium value of the membrane voltage trajectory lay well below threshold; the deviation typically corresponded to the standard deviation of the noise or more. The noise standard deviation was estimated to be about 2 mV. 5. With increasing mean firing rate, the near-threshold portion of the trajectory obtainable from the histogram occurred earlier, was steeper and rose to a higher level. Trajectories for different firing rates fell on the same curve after shifting them vertically by varying amounts. The curve was taken to represent the AHP of the motoneurone and was closely exponential. The shift of the trajectory gave its mean synaptic drive. The duration of the AHP varied between units and was longer than average for units from soleus muscle. 6. Further modelling showed that summation of noise with the AHP can explain the well-known changes in discharge variability that occur as firing rate increases. 7. It is concluded that synaptic noise plays a major role in the excitation of tonically firing human motoneurones and that the noiseless motoneurone with a linear trajectory provides an inadequate model for the conscious human. This is of interest in relation to various standard measures of human motor unit activity such as short-term synchronization.
Full text
PDF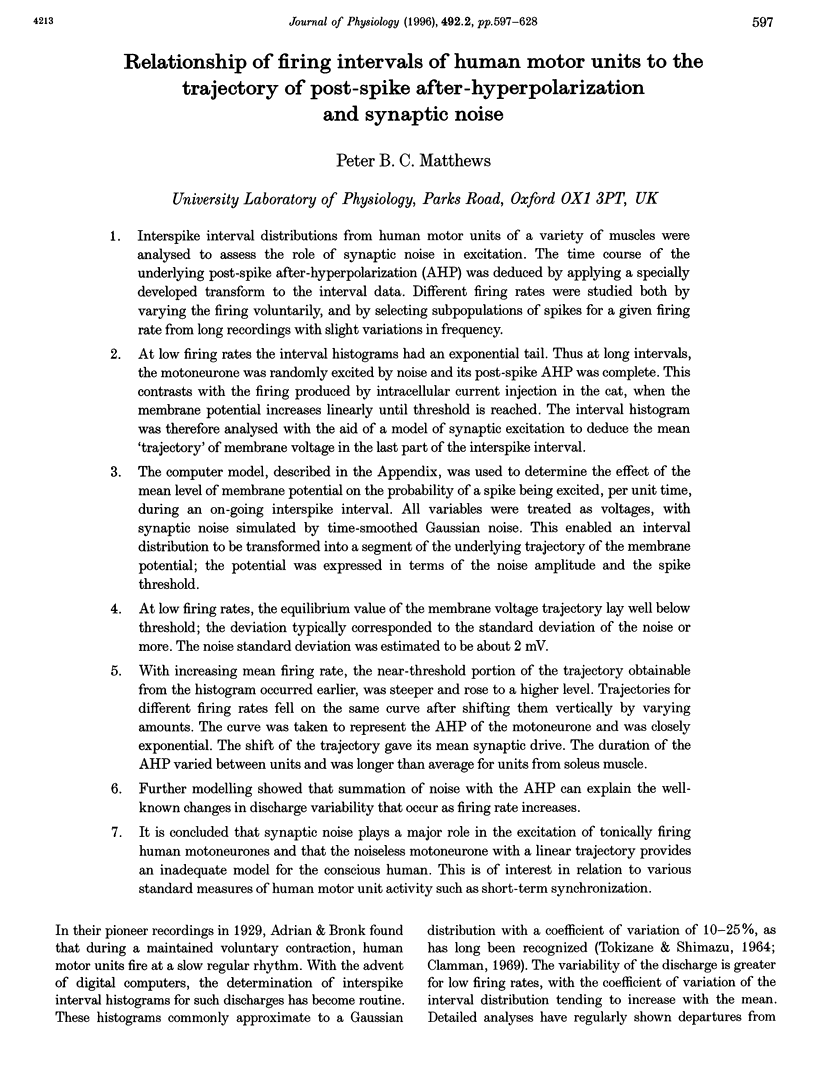
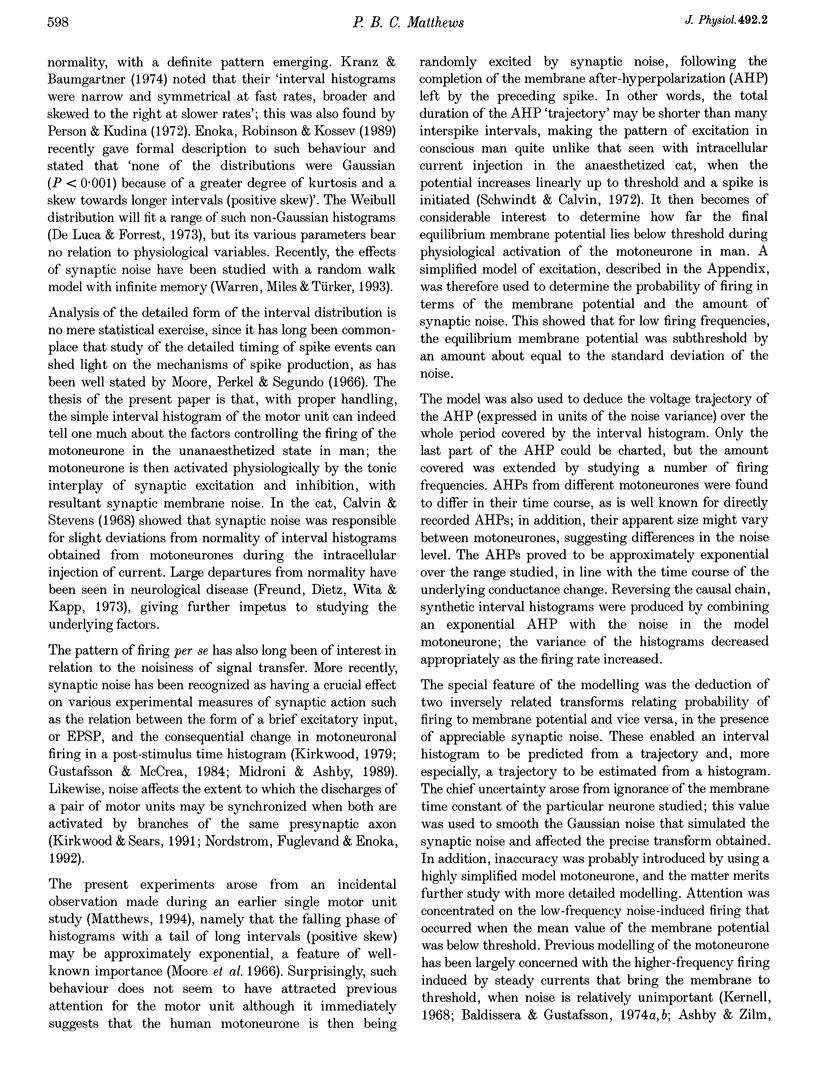
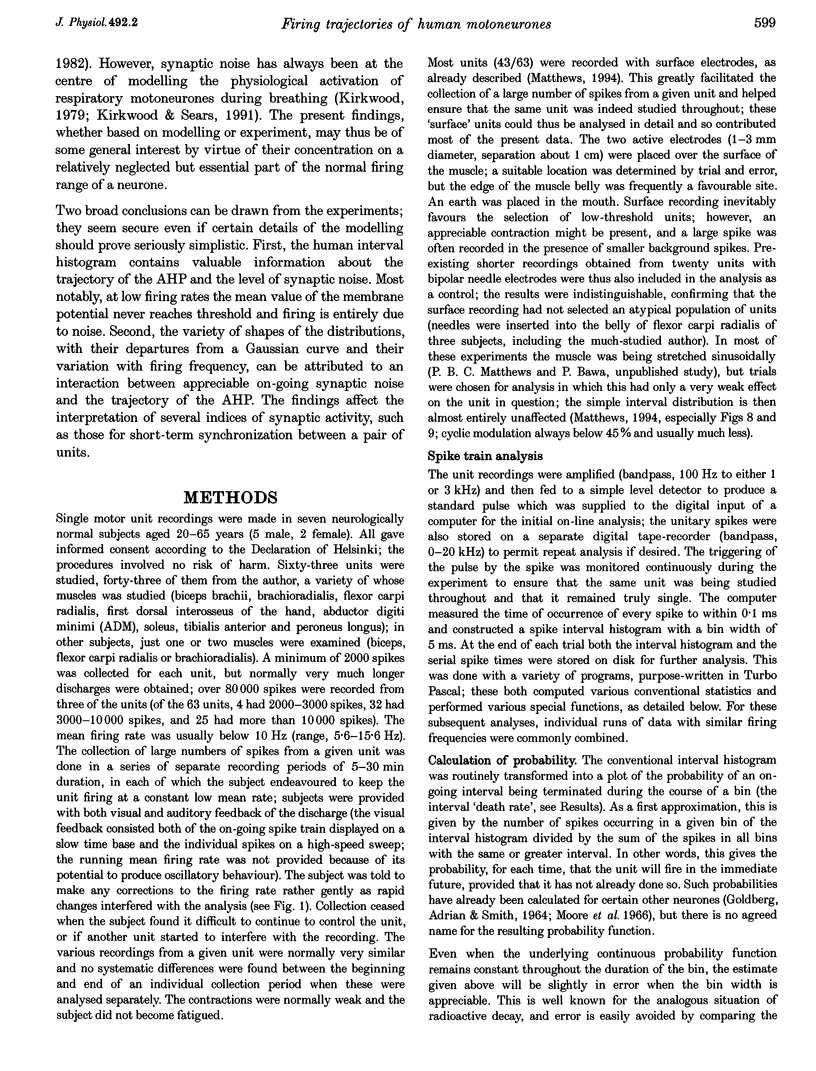
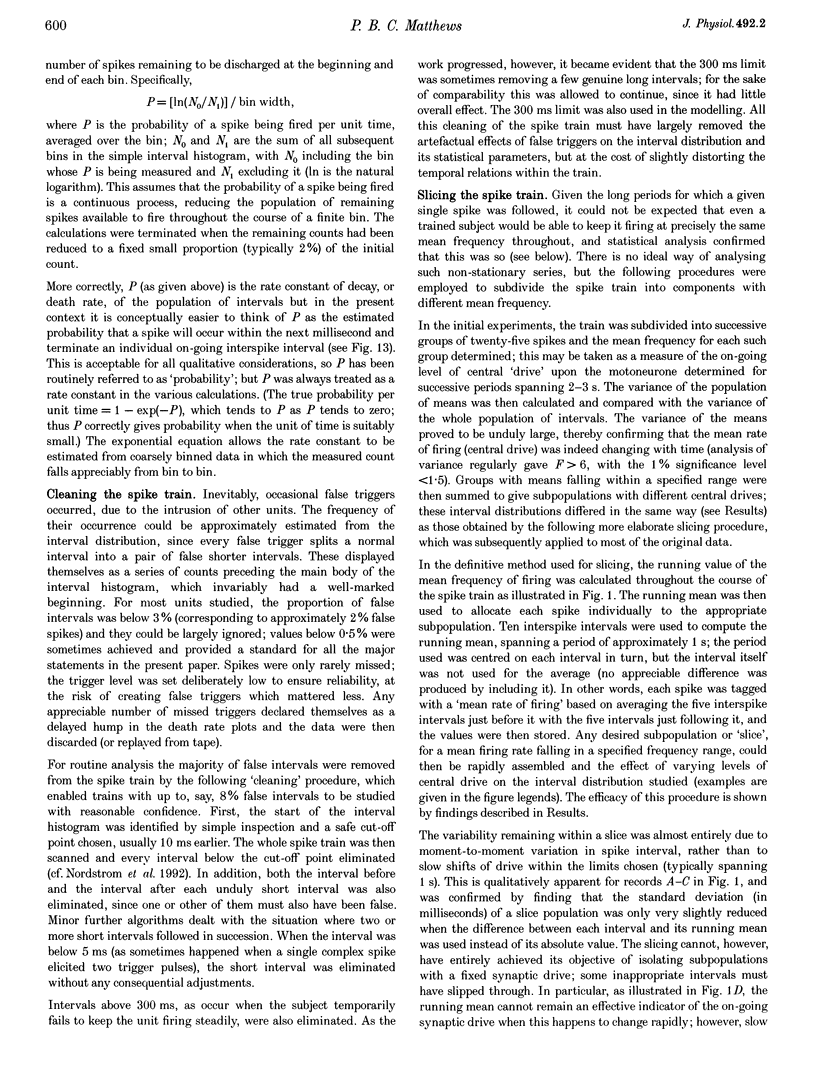
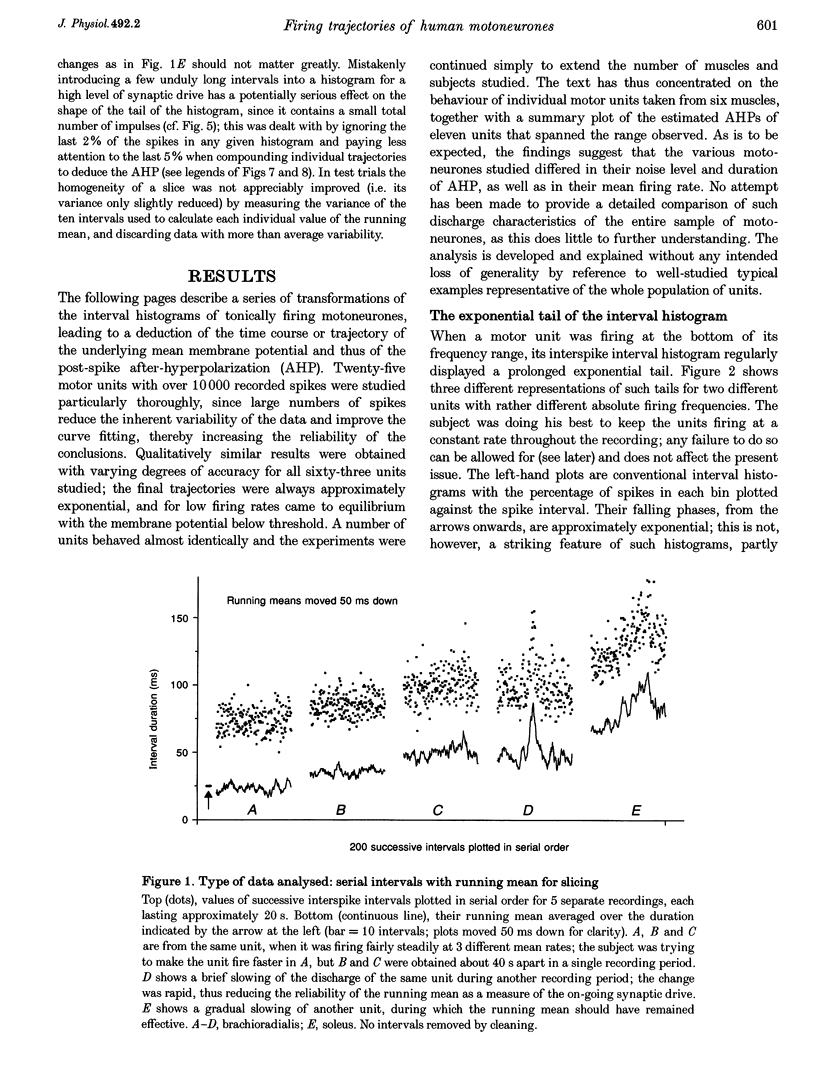
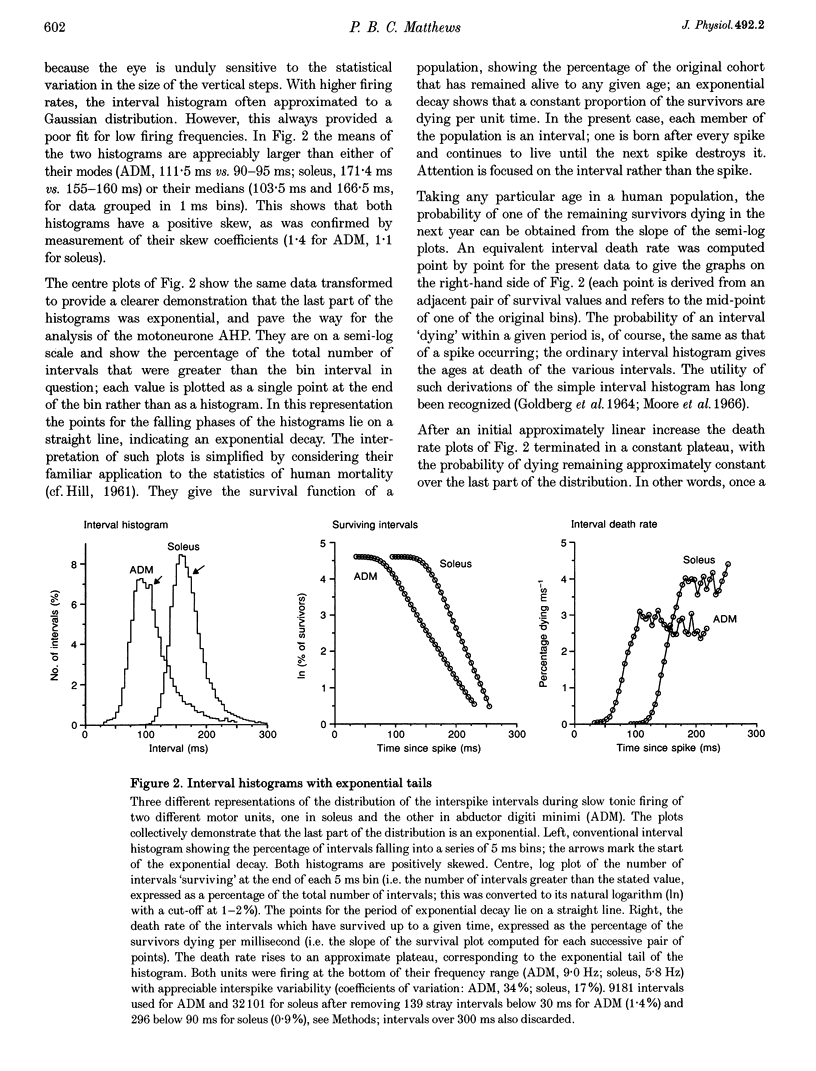
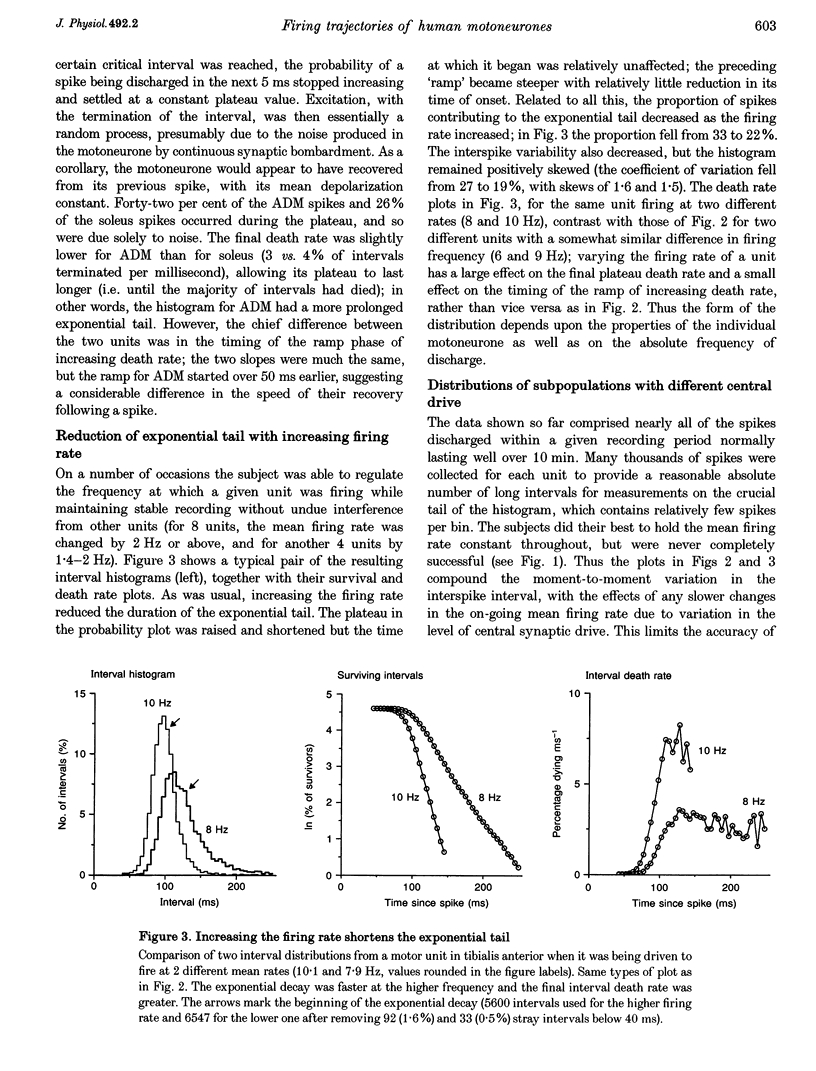
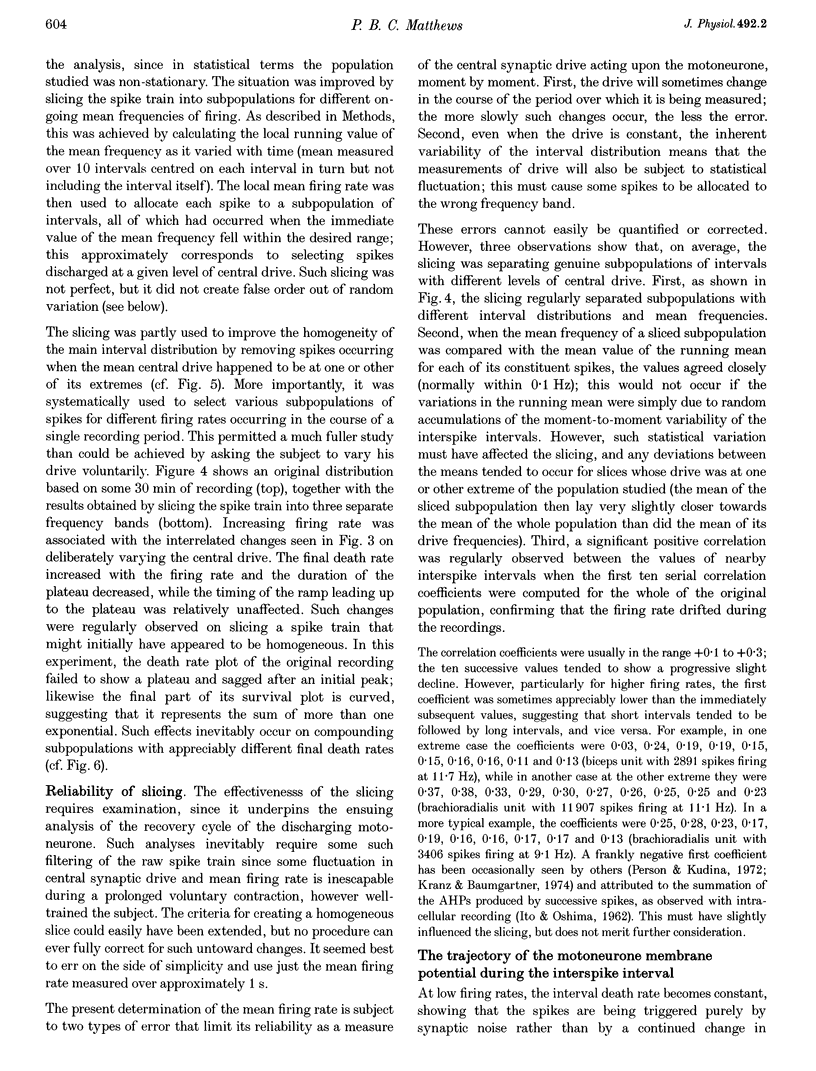
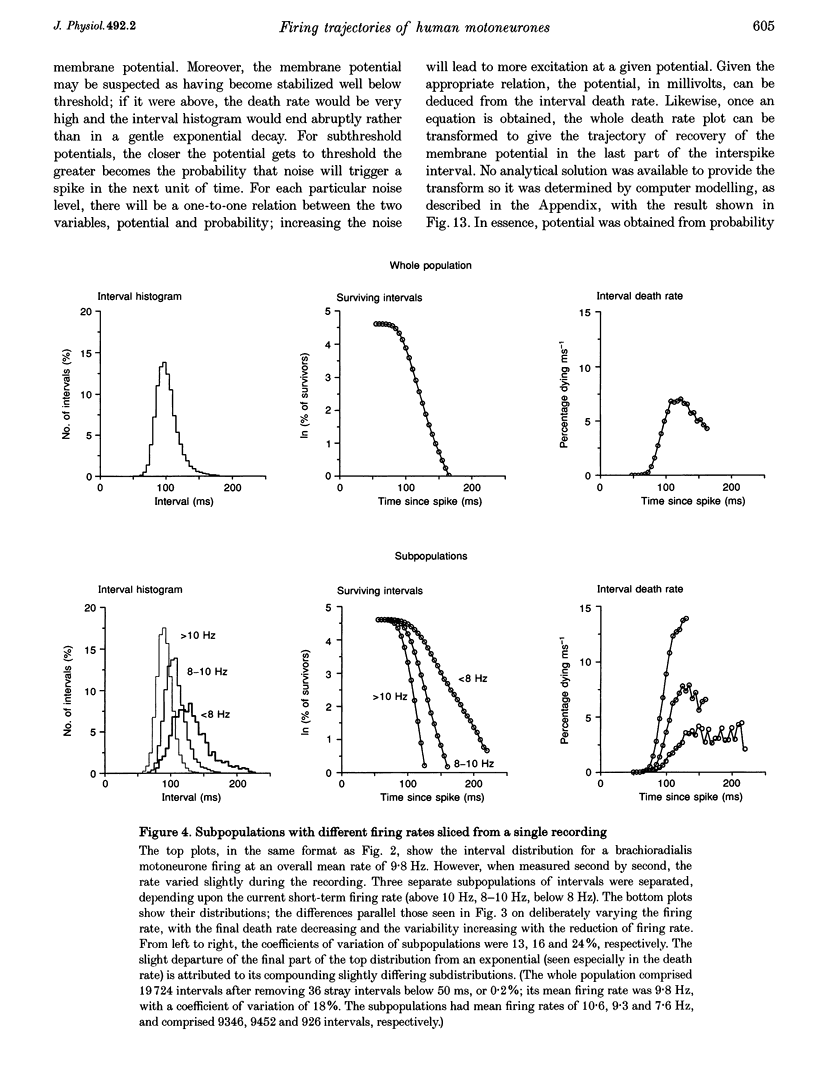
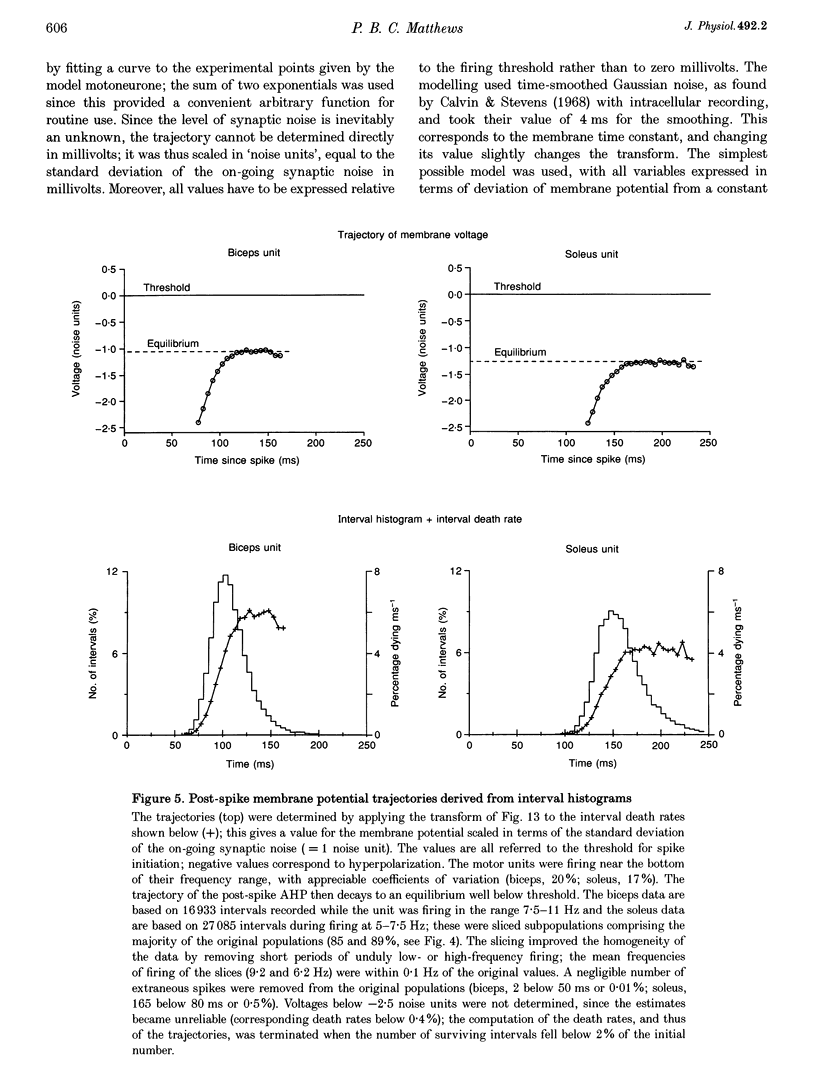
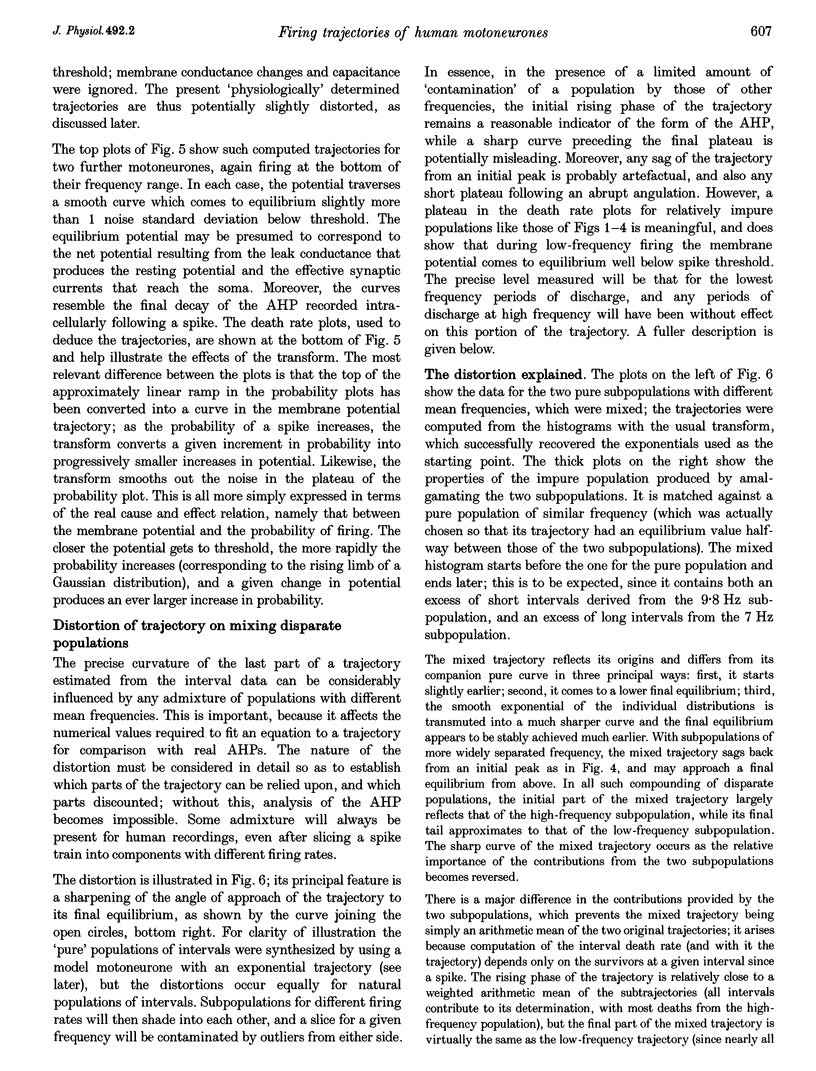
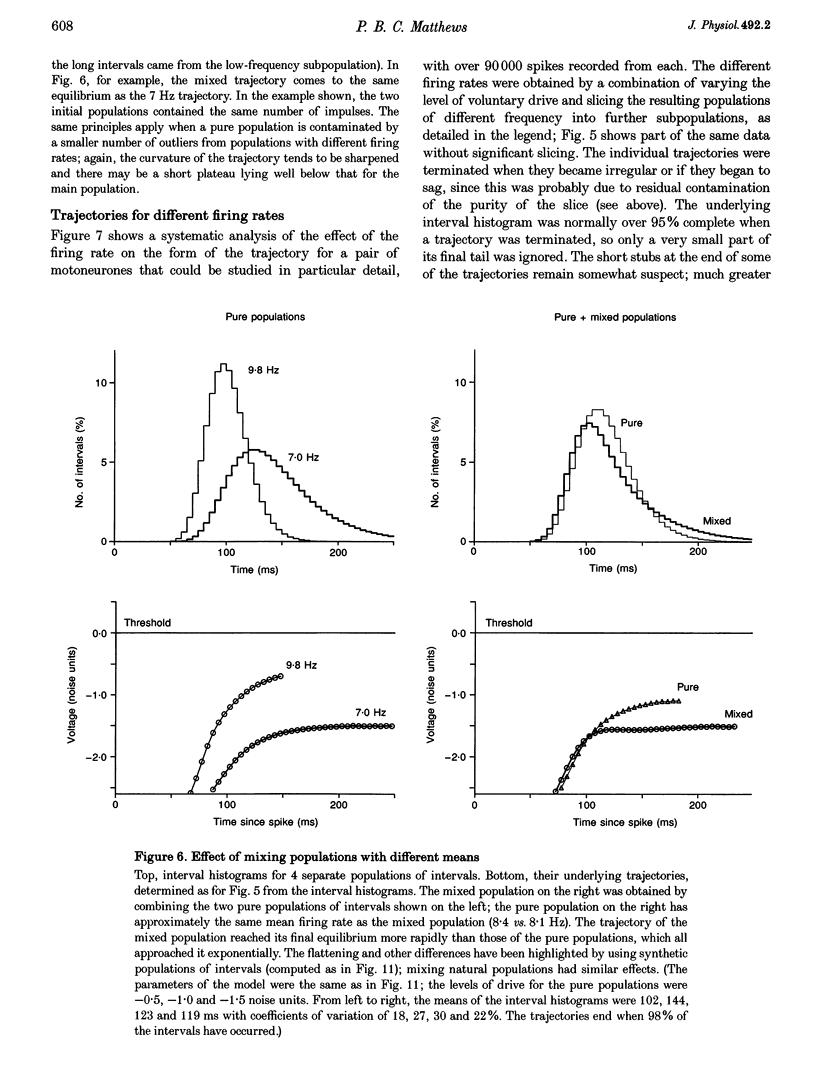
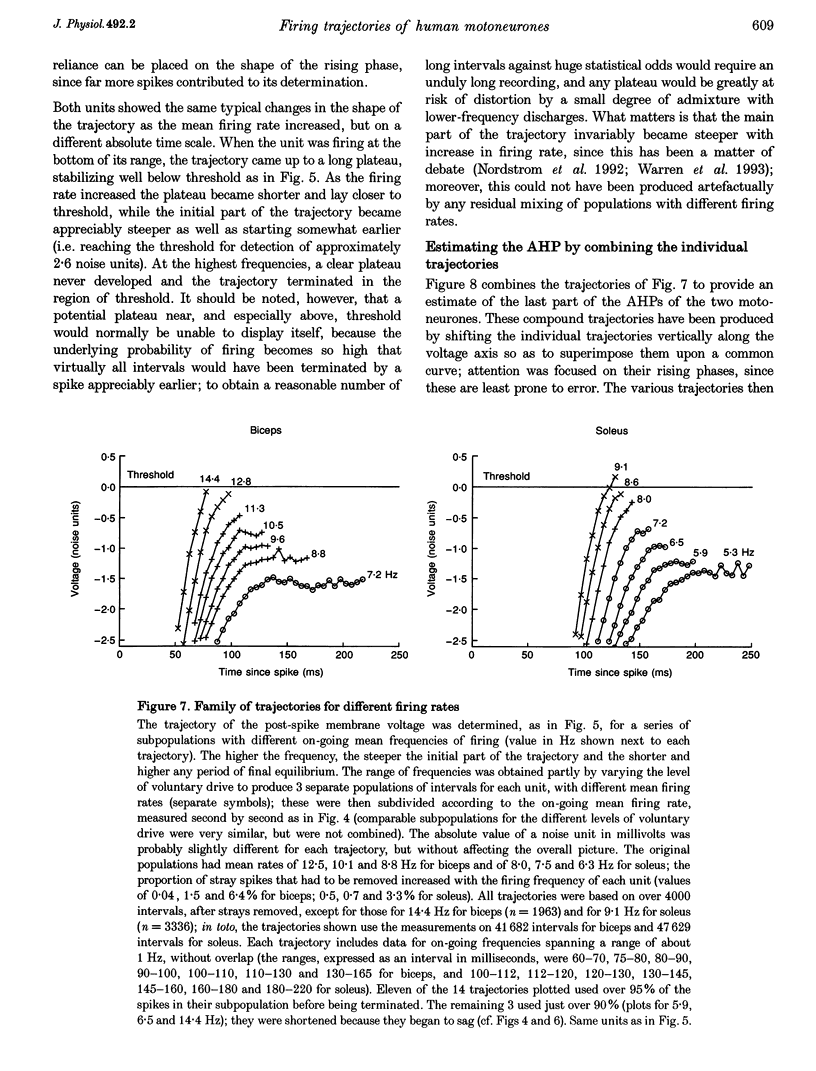
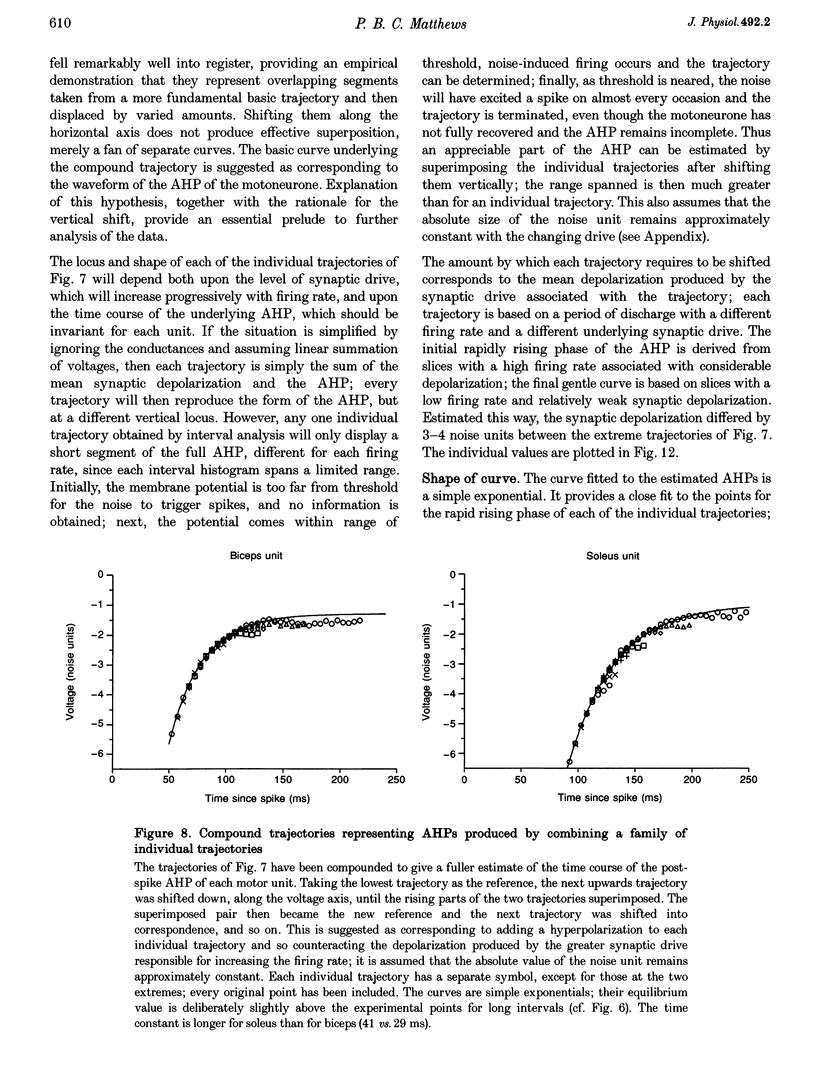
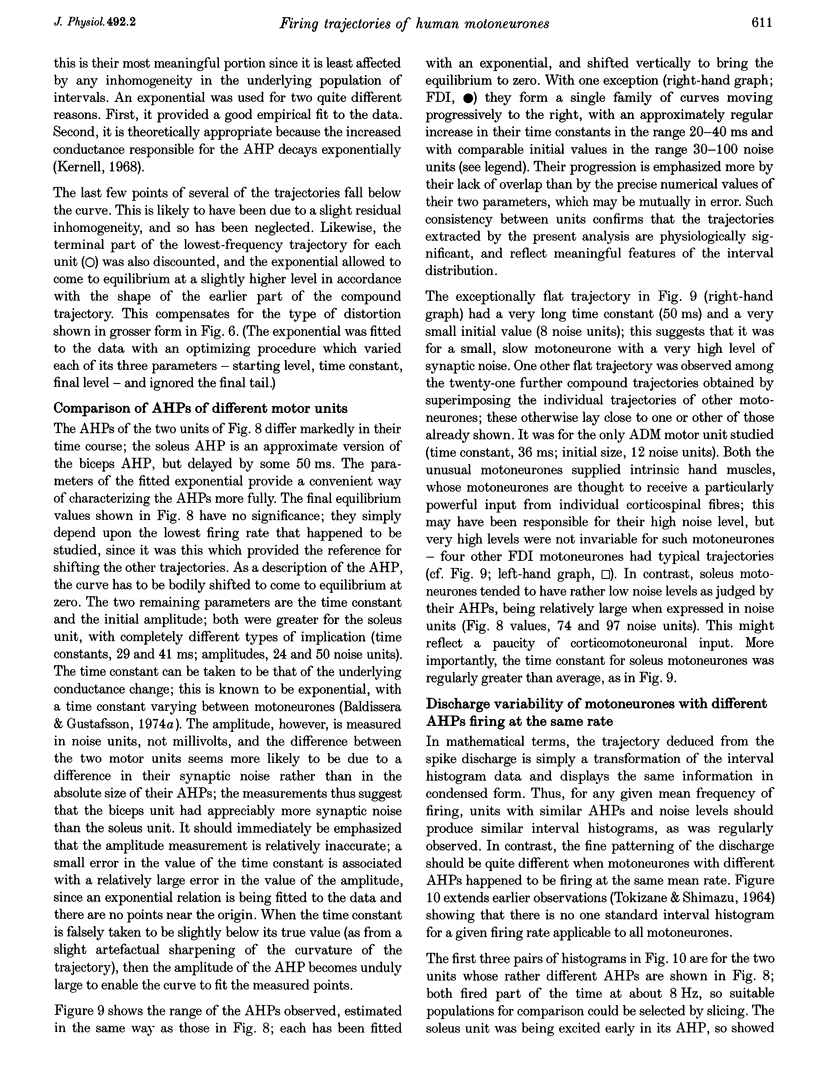
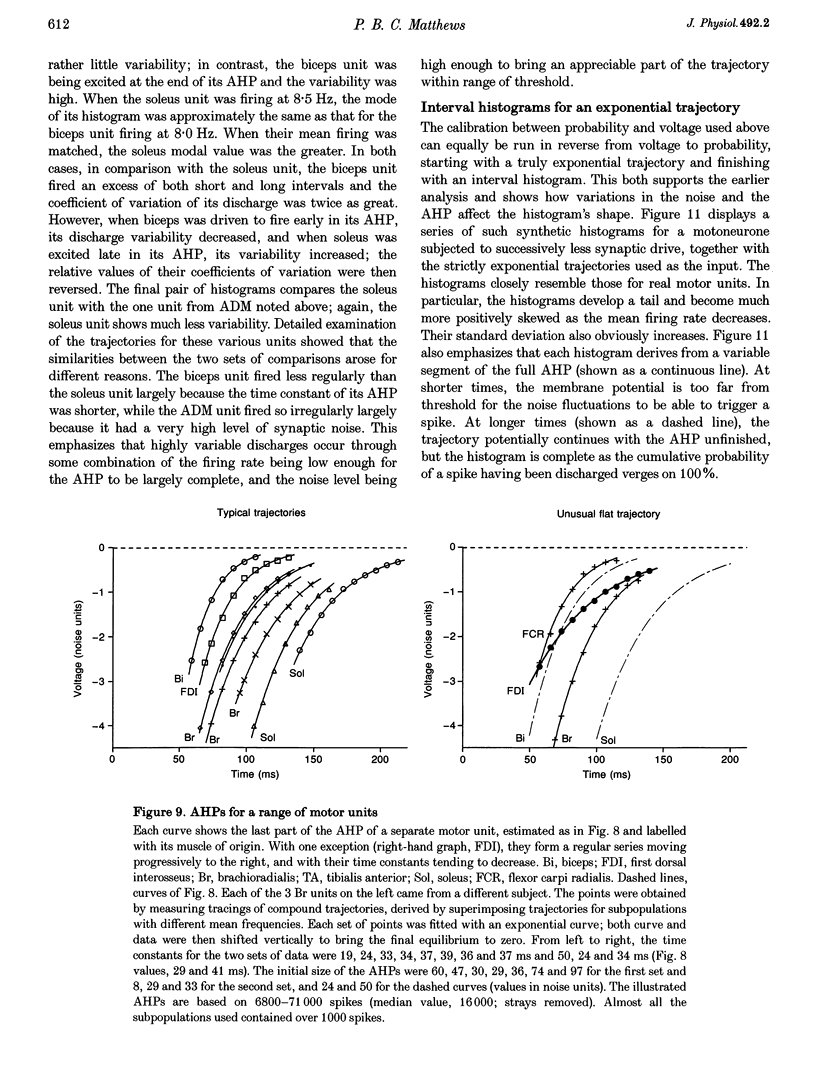
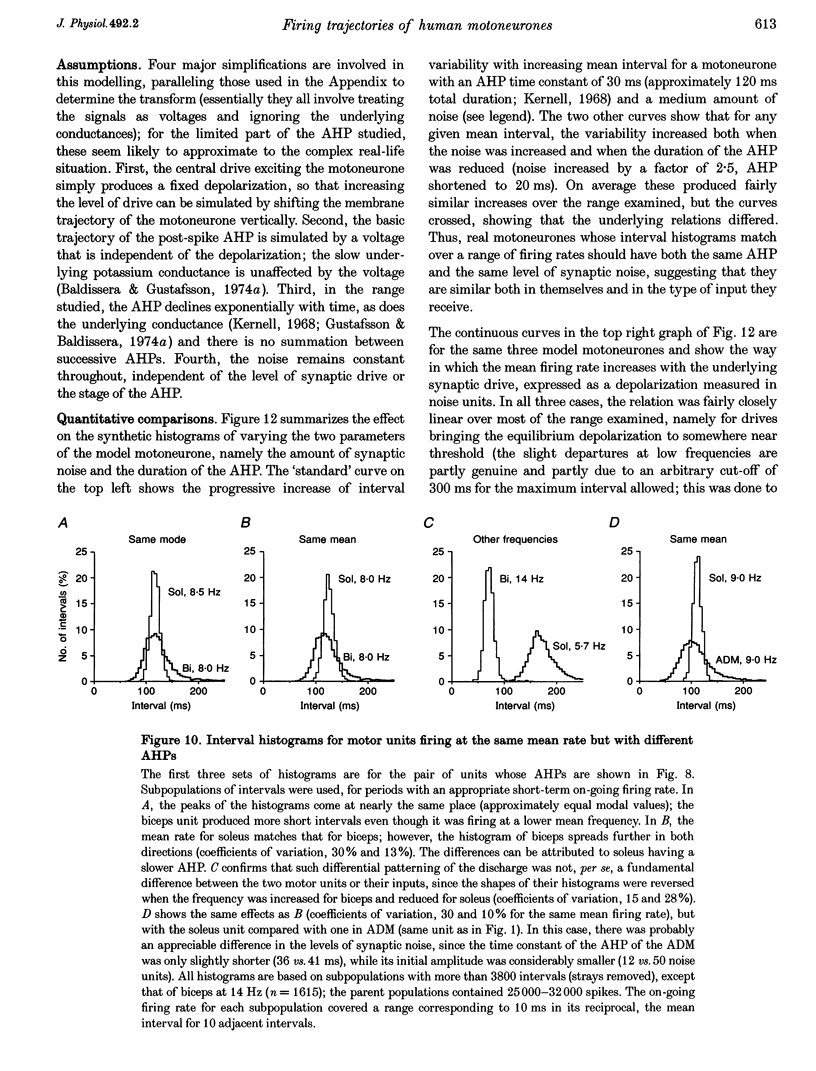
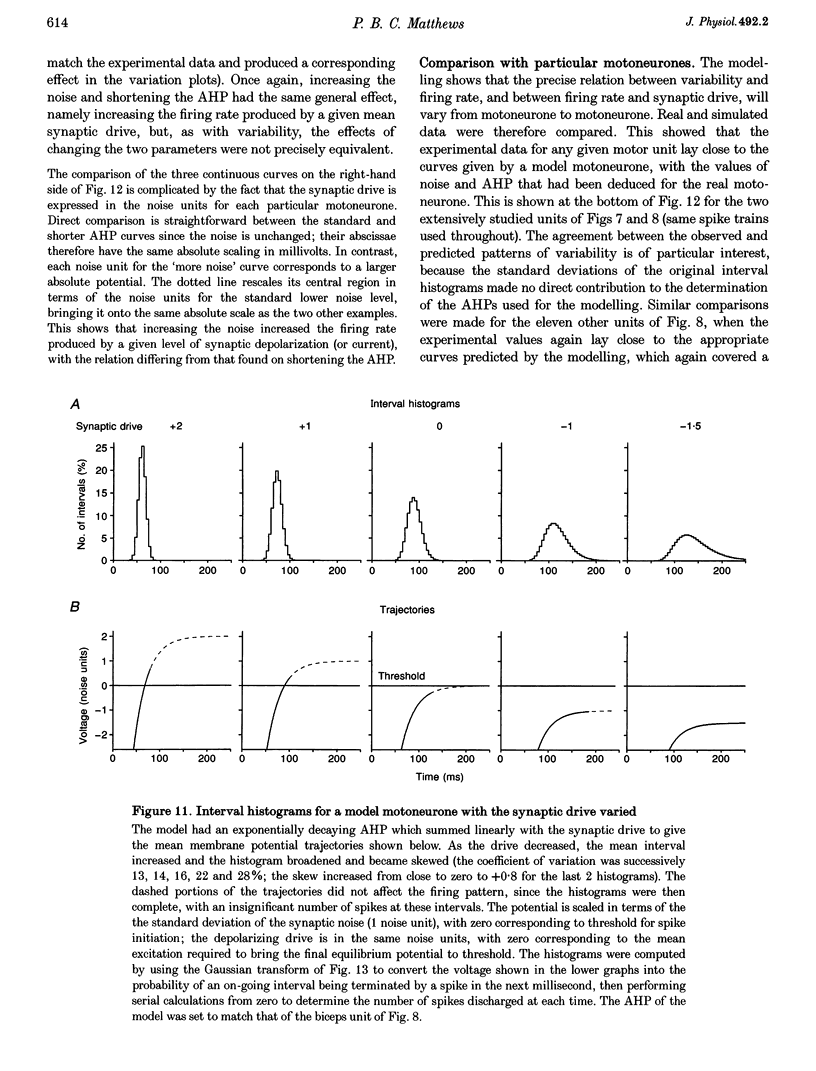
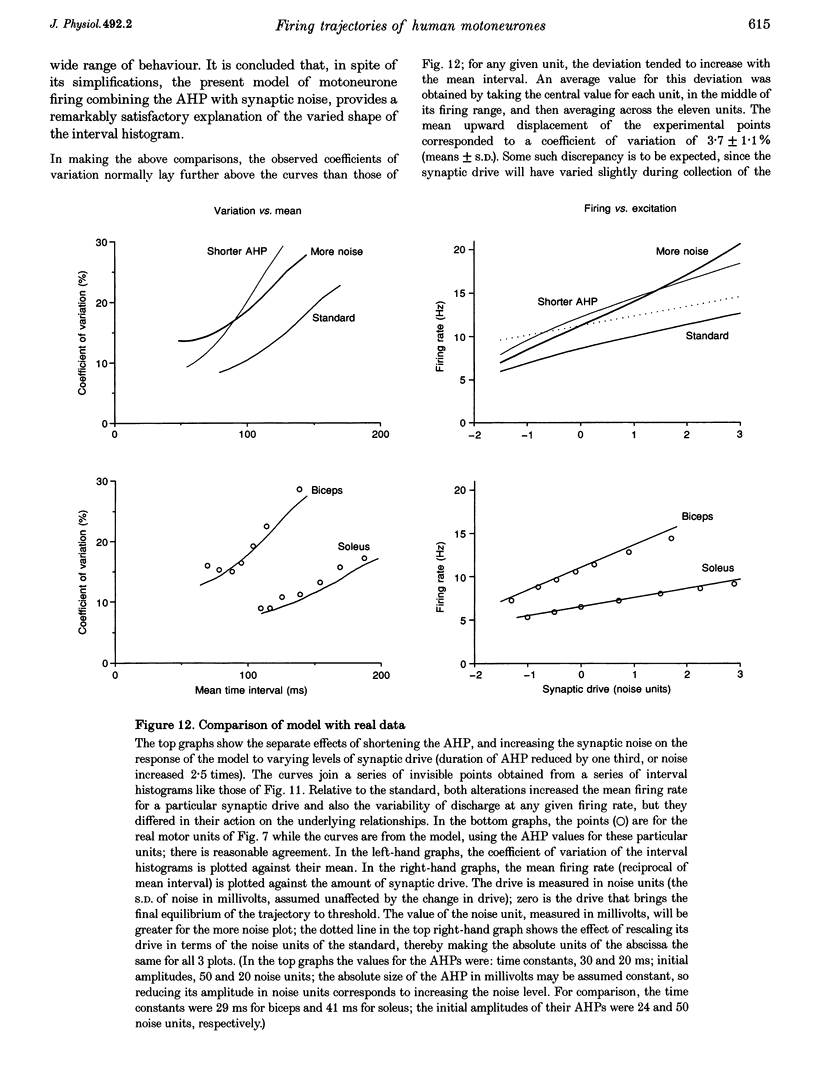
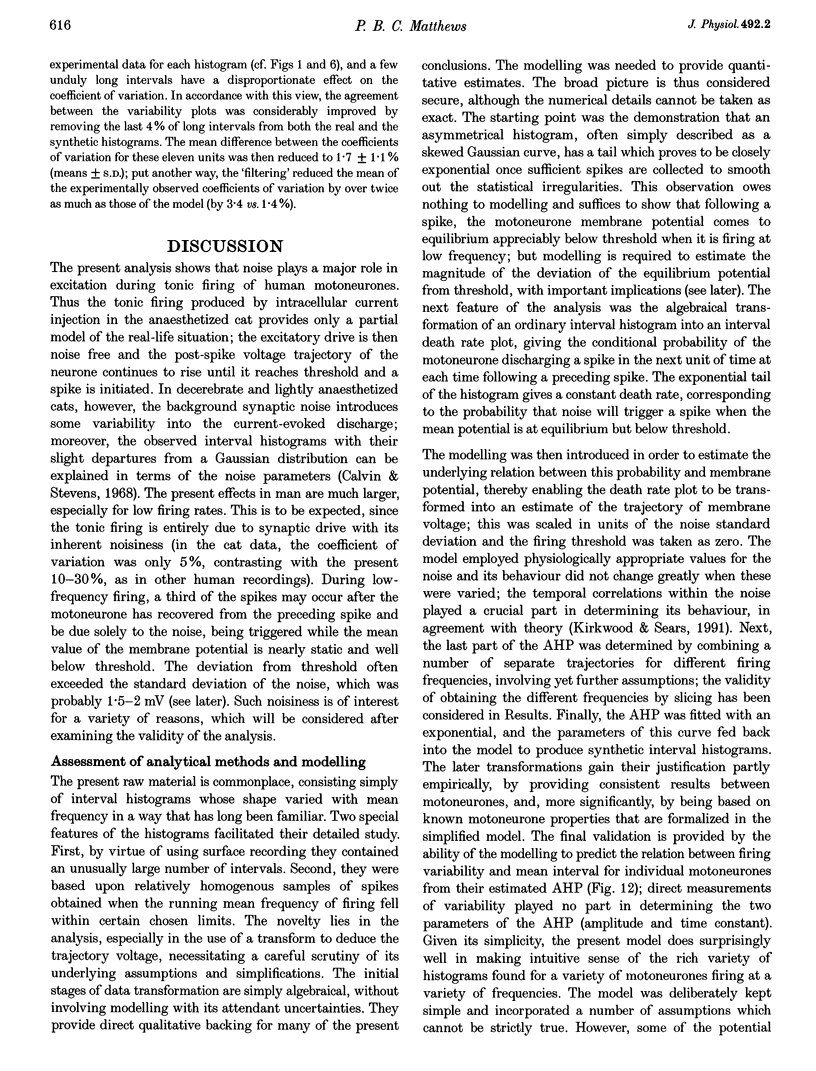
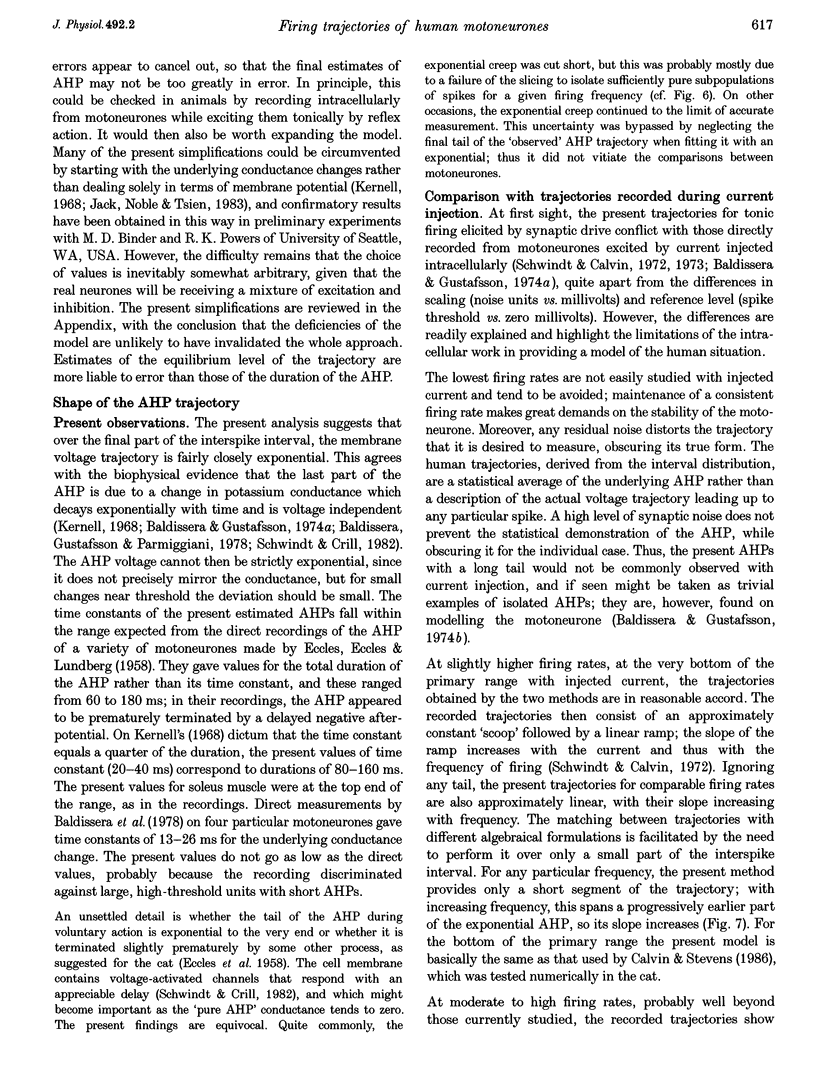
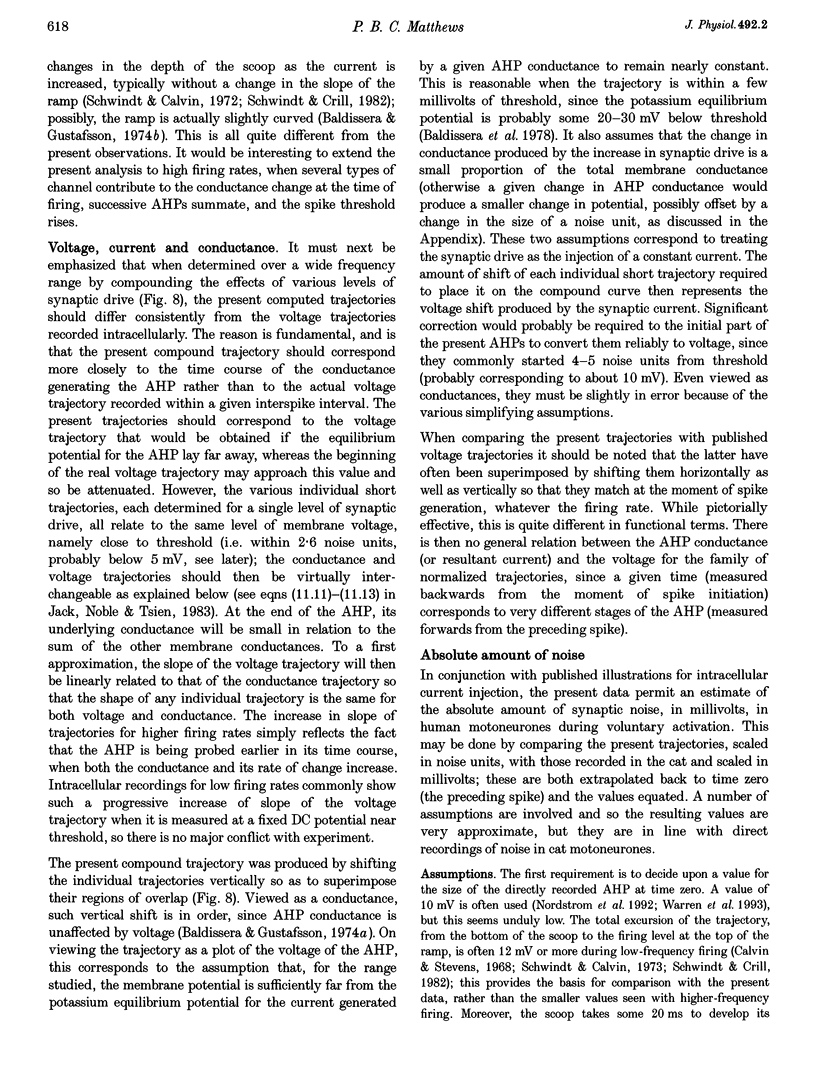
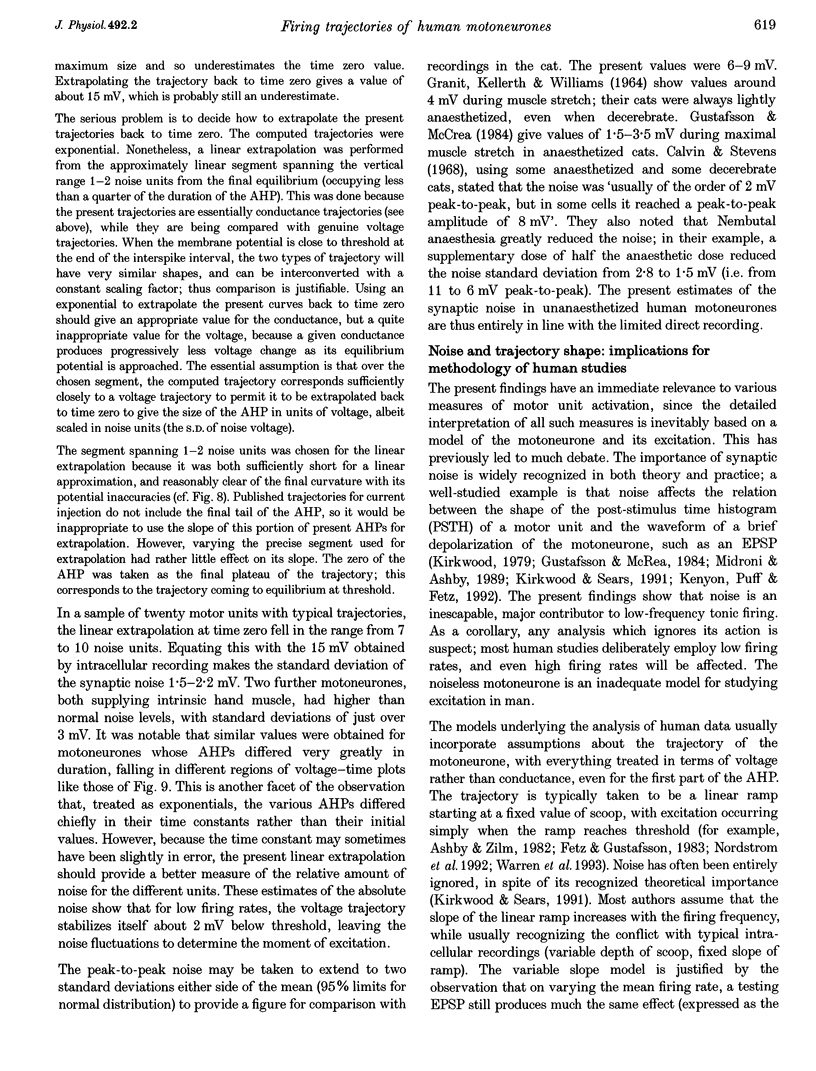
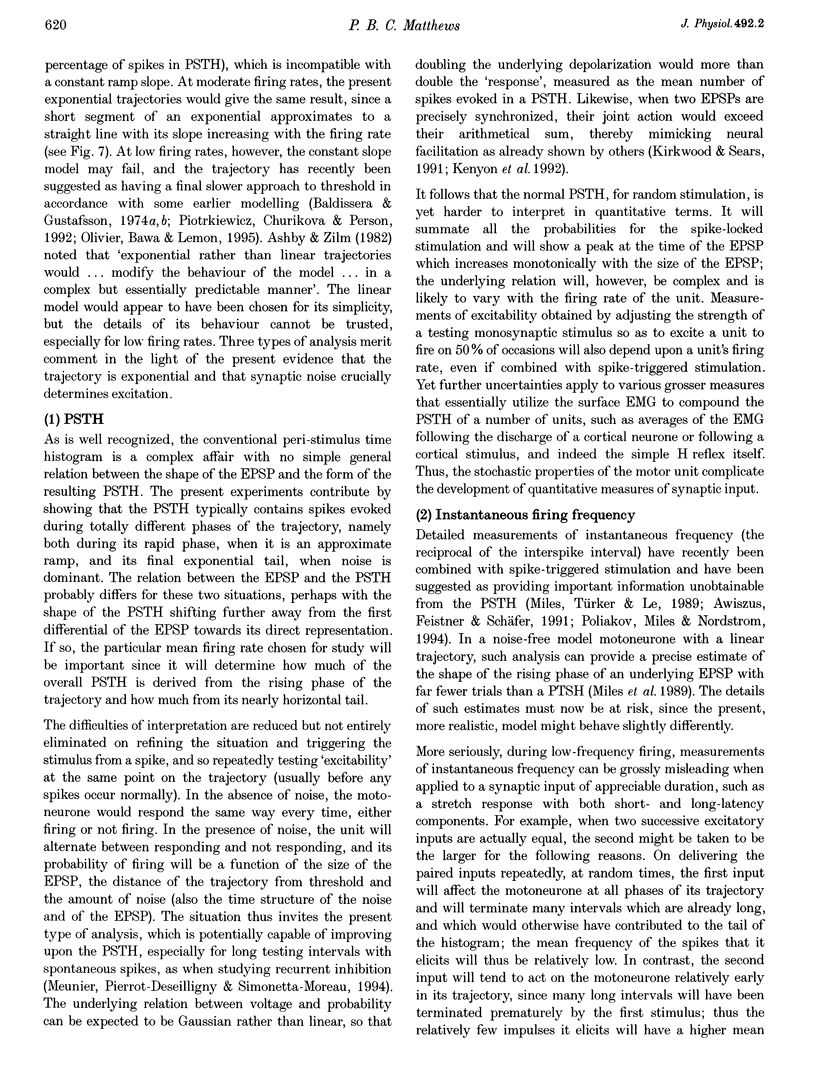
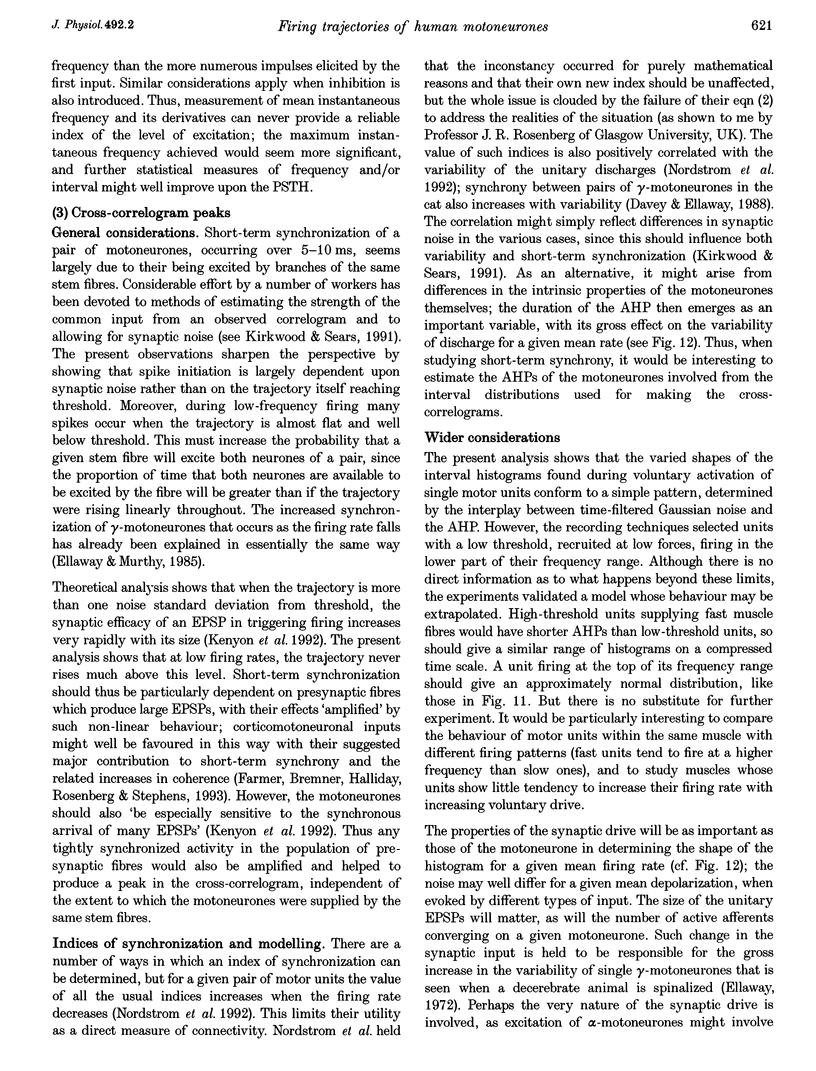
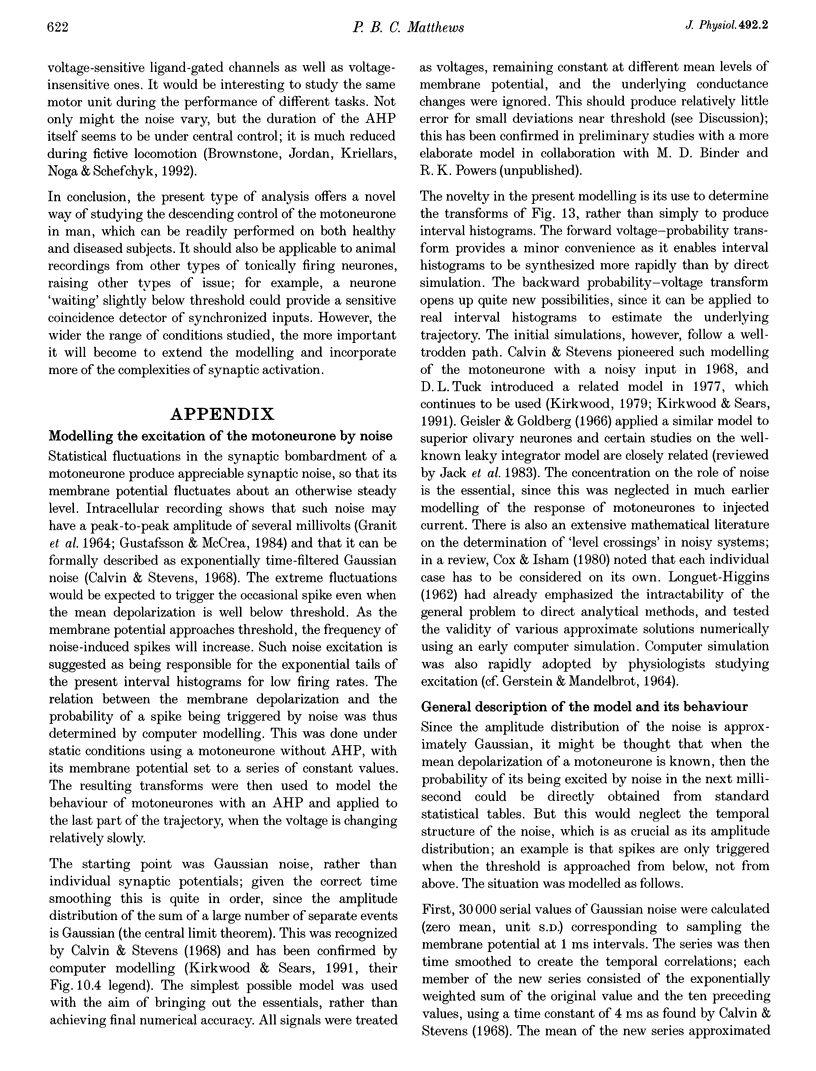
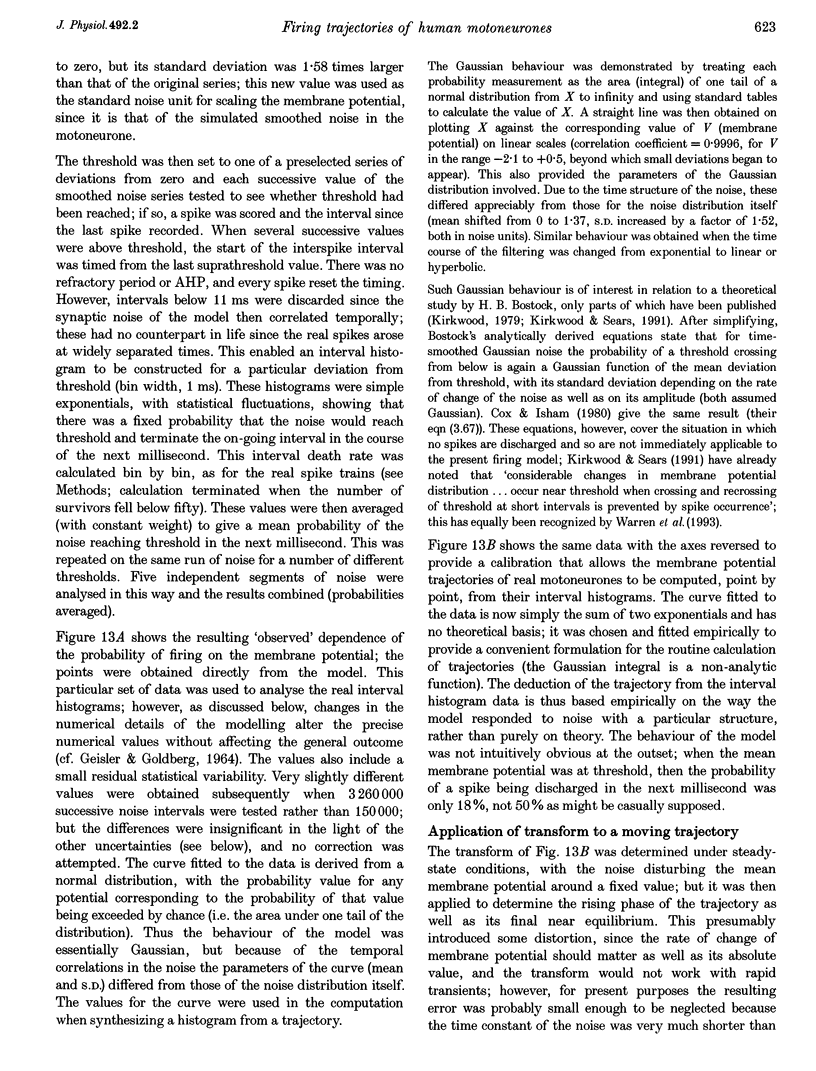
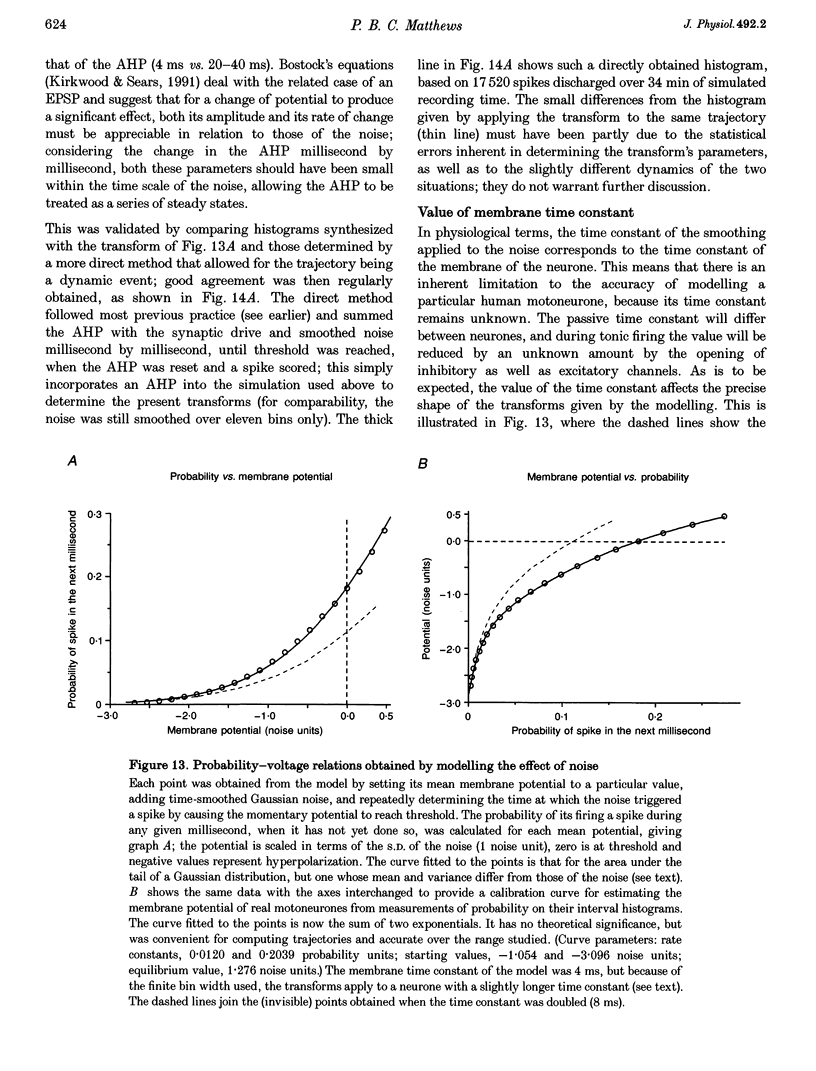
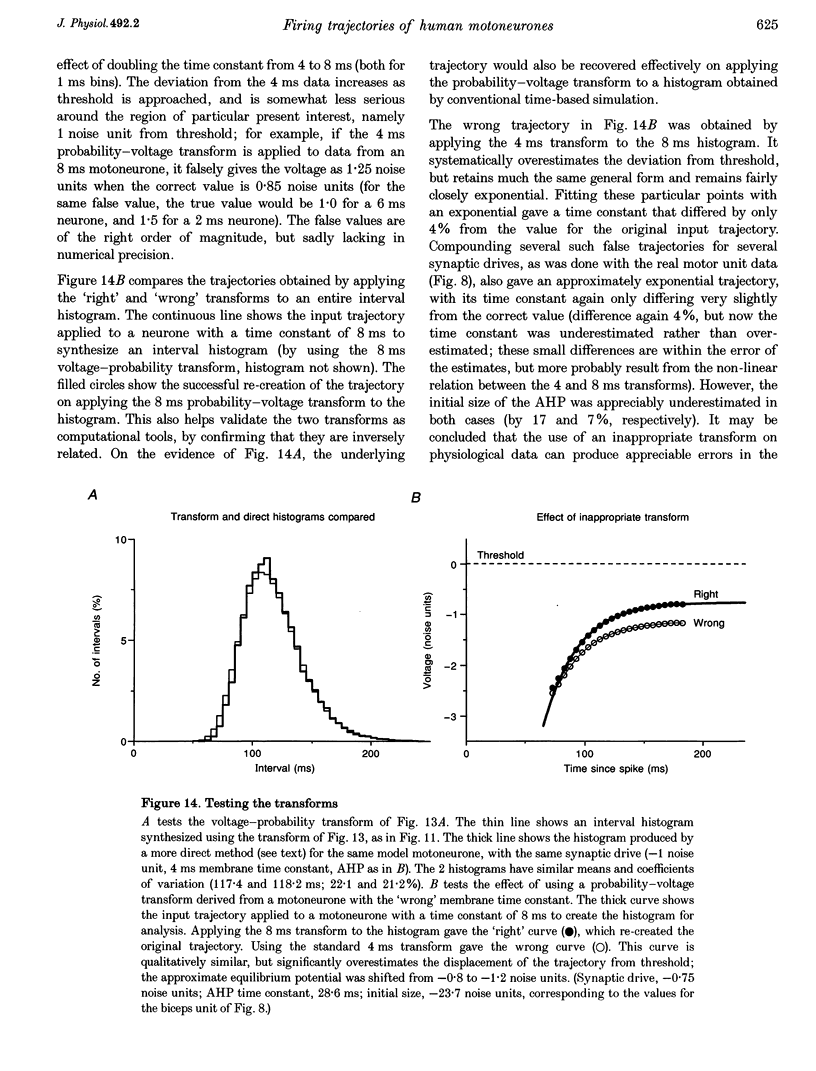
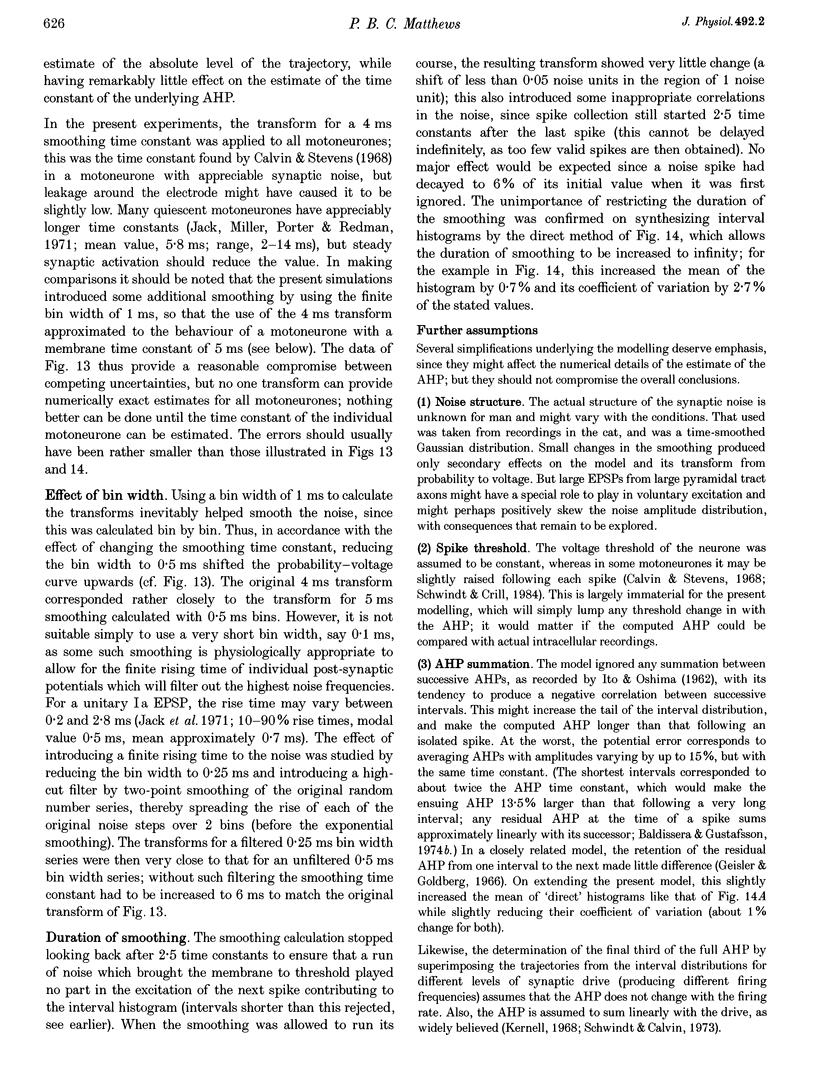
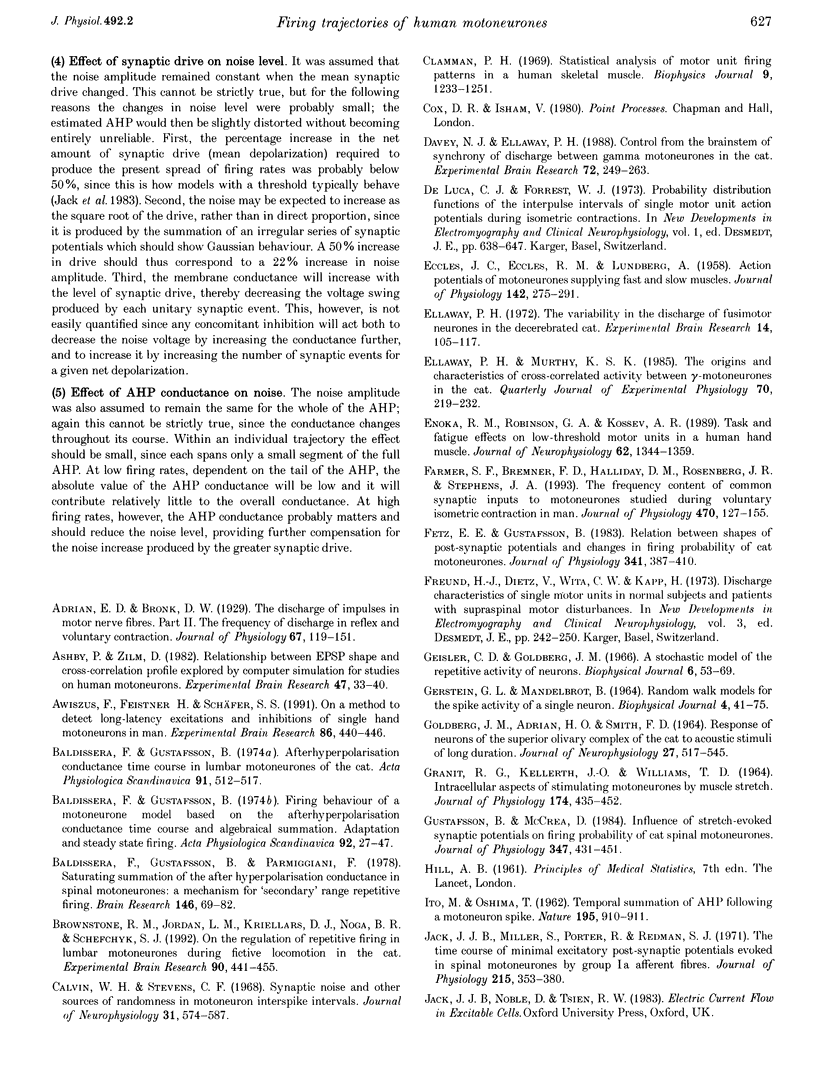
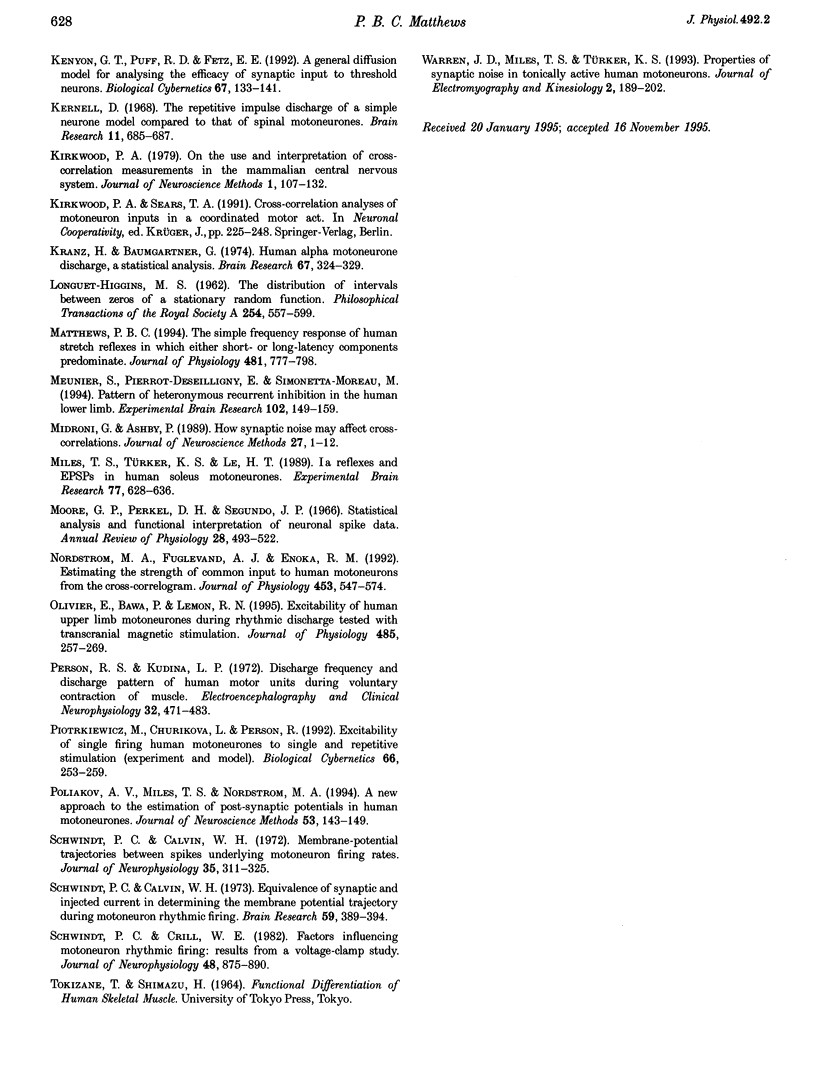
Selected References
These references are in PubMed. This may not be the complete list of references from this article.
- Adrian E. D., Bronk D. W. The discharge of impulses in motor nerve fibres: Part II. The frequency of discharge in reflex and voluntary contractions. J Physiol. 1929 Mar 20;67(2):i3–151. [PMC free article] [PubMed] [Google Scholar]
- Ashby P., Zilm D. Relationship between EPSP shape and cross-correlation profile explored by computer simulation for studies on human motoneurons. Exp Brain Res. 1982;47(1):33–40. doi: 10.1007/BF00235883. [DOI] [PubMed] [Google Scholar]
- Awiszus F., Feistner H., Schäfer S. S. On a method to detect long-latency excitations and inhibitions of single hand muscle motoneurons in man. Exp Brain Res. 1991;86(2):440–446. doi: 10.1007/BF00228970. [DOI] [PubMed] [Google Scholar]
- Baldissera F., Gustafsson B. Afterhyperpolarization conductance time course in lumbar motoneurones of the cat. Acta Physiol Scand. 1974 Aug;91(4):512–527. doi: 10.1111/j.1748-1716.1974.tb05707.x. [DOI] [PubMed] [Google Scholar]
- Baldissera F., Gustafsson B., Parmiggiani F. Saturating summation of the afterhyperpolarization conductance in spinal motoneurones: a mechanism for 'secondary range' repetitive firing. Brain Res. 1978 May 5;146(1):69–82. doi: 10.1016/0006-8993(78)90218-4. [DOI] [PubMed] [Google Scholar]
- Brownstone R. M., Jordan L. M., Kriellaars D. J., Noga B. R., Shefchyk S. J. On the regulation of repetitive firing in lumbar motoneurones during fictive locomotion in the cat. Exp Brain Res. 1992;90(3):441–455. doi: 10.1007/BF00230927. [DOI] [PubMed] [Google Scholar]
- Calvin W. H., Stevens C. F. Synaptic noise and other sources of randomness in motoneuron interspike intervals. J Neurophysiol. 1968 Jul;31(4):574–587. doi: 10.1152/jn.1968.31.4.574. [DOI] [PubMed] [Google Scholar]
- Davey N. J., Ellaway P. H. Control from the brainstem of synchrony of discharge between gamma motoneurones in the cat. Exp Brain Res. 1988;72(2):249–263. doi: 10.1007/BF00250248. [DOI] [PubMed] [Google Scholar]
- ECCLES J. C., ECCLES R. M., LUNDBERG A. The action potentials of the alpha motoneurones supplying fast and slow muscles. J Physiol. 1958 Jul 14;142(2):275–291. doi: 10.1113/jphysiol.1958.sp006015. [DOI] [PMC free article] [PubMed] [Google Scholar]
- Ellaway P. H. The variability in discharge of fusimotor neurones in the decerebrate cat. Exp Brain Res. 1972;14(2):105–117. doi: 10.1007/BF00234794. [DOI] [PubMed] [Google Scholar]
- Enoka R. M., Robinson G. A., Kossev A. R. Task and fatigue effects on low-threshold motor units in human hand muscle. J Neurophysiol. 1989 Dec;62(6):1344–1359. doi: 10.1152/jn.1989.62.6.1344. [DOI] [PubMed] [Google Scholar]
- Farmer S. F., Bremner F. D., Halliday D. M., Rosenberg J. R., Stephens J. A. The frequency content of common synaptic inputs to motoneurones studied during voluntary isometric contraction in man. J Physiol. 1993 Oct;470:127–155. doi: 10.1113/jphysiol.1993.sp019851. [DOI] [PMC free article] [PubMed] [Google Scholar]
- Fetz E. E., Gustafsson B. Relation between shapes of post-synaptic potentials and changes in firing probability of cat motoneurones. J Physiol. 1983 Aug;341:387–410. doi: 10.1113/jphysiol.1983.sp014812. [DOI] [PMC free article] [PubMed] [Google Scholar]
- GERSTEIN G. L., MANDELBROT B. RANDOM WALK MODELS FOR THE SPIKE ACTIVITY OF A SINGLE NEURON. Biophys J. 1964 Jan;4:41–68. doi: 10.1016/s0006-3495(64)86768-0. [DOI] [PMC free article] [PubMed] [Google Scholar]
- GRANIT R., KELLERTH J. O., WILLIAMS T. D. INTRACELLULAR ASPECTS OF STIMULATING MOTONEURONES BY MUSCLE STRETCH. J Physiol. 1964 Nov;174:435–452. doi: 10.1113/jphysiol.1964.sp007496. [DOI] [PMC free article] [PubMed] [Google Scholar]
- Geisler C. D., Goldberg J. M. A stochastic model of the repetitive activity of neurons. Biophys J. 1966 Jan;6(1):53–69. doi: 10.1016/S0006-3495(66)86639-0. [DOI] [PMC free article] [PubMed] [Google Scholar]
- Gustafsson B., McCrea D. Influence of stretch-evoked synaptic potentials on firing probability of cat spinal motoneurones. J Physiol. 1984 Feb;347:431–451. doi: 10.1113/jphysiol.1984.sp015074. [DOI] [PMC free article] [PubMed] [Google Scholar]
- Jack J. J., Miller S., Porter R., Redman S. J. The time course of minimal excitory post-synaptic potentials evoked in spinal motoneurones by group Ia afferent fibres. J Physiol. 1971 Jun;215(2):353–380. doi: 10.1113/jphysiol.1971.sp009474. [DOI] [PMC free article] [PubMed] [Google Scholar]
- Kenyon G. T., Puff R. D., Fetz E. E. A general diffusion model for analyzing the efficacy of synaptic input to threshold neurons. Biol Cybern. 1992;67(2):133–141. doi: 10.1007/BF00201020. [DOI] [PubMed] [Google Scholar]
- Kernell D. The repetitive impulse discharge of a simple neurone model compared to that of spinal motoneurones. Brain Res. 1968 Dec;11(3):685–687. doi: 10.1016/0006-8993(68)90157-1. [DOI] [PubMed] [Google Scholar]
- Kirkwood P. A. On the use and interpretation of cross-correlations measurements in the mammalian central nervous system. J Neurosci Methods. 1979 Aug;1(2):107–132. doi: 10.1016/0165-0270(79)90009-8. [DOI] [PubMed] [Google Scholar]
- Kranz H., Baumgartner G. Human alpha motoneurone discharge, a statistical analysis. Brain Res. 1974 Feb 22;67(2):324–329. doi: 10.1016/0006-8993(74)90282-0. [DOI] [PubMed] [Google Scholar]
- Matthews P. B. The simple frequency response of human stretch reflexes in which either short- or long-latency components predominate. J Physiol. 1994 Dec 15;481(Pt 3):777–798. doi: 10.1113/jphysiol.1994.sp020481. [DOI] [PMC free article] [PubMed] [Google Scholar]
- Meunier S., Pierrot-Deseilligny E., Simonetta-Moreau M. Pattern of heteronymous recurrent inhibition in the human lower limb. Exp Brain Res. 1994;102(1):149–159. doi: 10.1007/BF00232447. [DOI] [PubMed] [Google Scholar]
- Moore G. P., Perkel D. H., Segundo J. P. Statistical analysis and functional interpretation of neuronal spike data. Annu Rev Physiol. 1966;28:493–522. doi: 10.1146/annurev.ph.28.030166.002425. [DOI] [PubMed] [Google Scholar]
- Nordstrom M. A., Fuglevand A. J., Enoka R. M. Estimating the strength of common input to human motoneurons from the cross-correlogram. J Physiol. 1992;453:547–574. doi: 10.1113/jphysiol.1992.sp019244. [DOI] [PMC free article] [PubMed] [Google Scholar]
- Olivier E., Bawa P., Lemon R. N. Excitability of human upper limb motoneurones during rhythmic discharge tested with transcranial magnetic stimulation. J Physiol. 1995 May 15;485(Pt 1):257–269. doi: 10.1113/jphysiol.1995.sp020728. [DOI] [PMC free article] [PubMed] [Google Scholar]
- POGGIO G. F., VIERNSTEIN L. J. TIME SERIES ANALYSIS OF IMPULSE SEQUENCES OF THALAMIC SOMATIC SENSORY NEURONS. J Neurophysiol. 1964 Jul;27:517–545. doi: 10.1152/jn.1964.27.4.517. [DOI] [PubMed] [Google Scholar]
- Person R. S., Kudina L. P. Discharge frequency and discharge pattern of human motor units during voluntary contraction of muscle. Electroencephalogr Clin Neurophysiol. 1972 May;32(5):471–483. doi: 10.1016/0013-4694(72)90058-2. [DOI] [PubMed] [Google Scholar]
- Piotrkiewicz M., Churikova L., Person R. Excitability of single firing human motoneurones to single and repetitive stimulation (experiment and model). Biol Cybern. 1992;66(3):253–259. doi: 10.1007/BF00198478. [DOI] [PubMed] [Google Scholar]
- Poliakov A. V., Miles T. S., Nordstrom M. A. A new approach to the estimation of post-synaptic potentials in human motoneurones. J Neurosci Methods. 1994 Aug;53(2):143–149. doi: 10.1016/0165-0270(94)90171-6. [DOI] [PubMed] [Google Scholar]
- Schwindt P. C., Calvin W. H. Equivalence of synaptic and injected current in determining the membrane potential trajectory during motoneuron rhythmic firing. Brain Res. 1973 Sep 14;59:389–394. doi: 10.1016/0006-8993(73)90278-3. [DOI] [PubMed] [Google Scholar]
- Schwindt P. C., Calvin W. H. Membrane-potential trajectories between spikes underlying motoneuron firing rates. J Neurophysiol. 1972 May;35(3):311–325. doi: 10.1152/jn.1972.35.3.311. [DOI] [PubMed] [Google Scholar]
- Schwindt P. C., Crill W. E. Factors influencing motoneuron rhythmic firing: results from a voltage-clamp study. J Neurophysiol. 1982 Oct;48(4):875–890. doi: 10.1152/jn.1982.48.4.875. [DOI] [PubMed] [Google Scholar]