Abstract
1. Intracellular recordings in adult rat hippocampal slices were used to investigate the properties and origins of intrinsically generated bursts in the somata of CA1 pyramidal cells (PCs). The CA1 PCs were classified as either non-bursters or bursters according to the firing patterns evoked by intrasomatically applied long ( > or = 100 ms) depolarizing current pulses. Non-bursters generated stimulus-graded trains of independent action potentials, whereas bursters generated clusters of three or more closely spaced spikes riding on a distinct depolarizing envelope. 2. In all PCs fast spike repolarization was incomplete and ended at a potential approximately 10 mV more positive than resting potential. Solitary spikes were followed by a distinct after-depolarizing potential (ADP) lasting 20-40 ms. The ADP in most non-bursters declined monotonically to baseline ('passive' ADP), whereas in most bursters it remained steady or even re-depolarized before declining to baseline ('active' ADP). 3. Active, but not passive, ADPs were associated with an apparent increase in input conductance. They were maximal in amplitude when the spike was evoked from resting potential and were reduced by mild depolarization or hyperpolarization (+/- 2 mV). 4. Evoked and spontaneous burst firing was sensitive to small changes in membrane potential. In most cases maximal bursts were generated at resting potential and were curtailed by small depolarizations or hyperpolarizations (+/- 5 mV). 5. Bursts comprising clusters of spikelets ('d-spikes') were observed in 12% of the bursters. Some of the d-spikes attained threshold for triggering full somatic spikes. Gradually hyperpolarizing these neurones blocked somatic spikes before blocking d-spikes, suggesting that the latter are generated at more remote sites. 6. The data suggest that active ADPs and intrinsic bursts in the somata of adult CA1 PCs are generated by a slow, voltage-gated inward current. Bursts arise in neurones in which this current is sufficiently large to generate suprathreshold ADPs, and thereby initiate a regenerative process of spike recruitment and slow depolarization.
Full text
PDF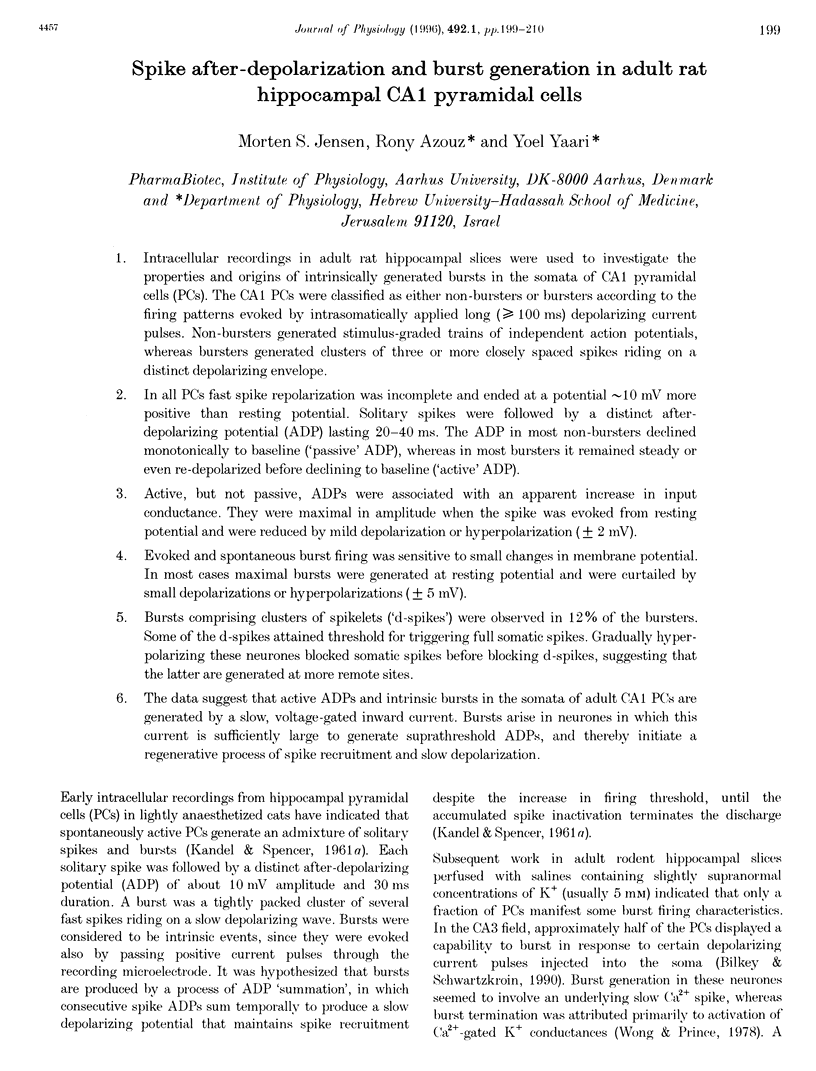
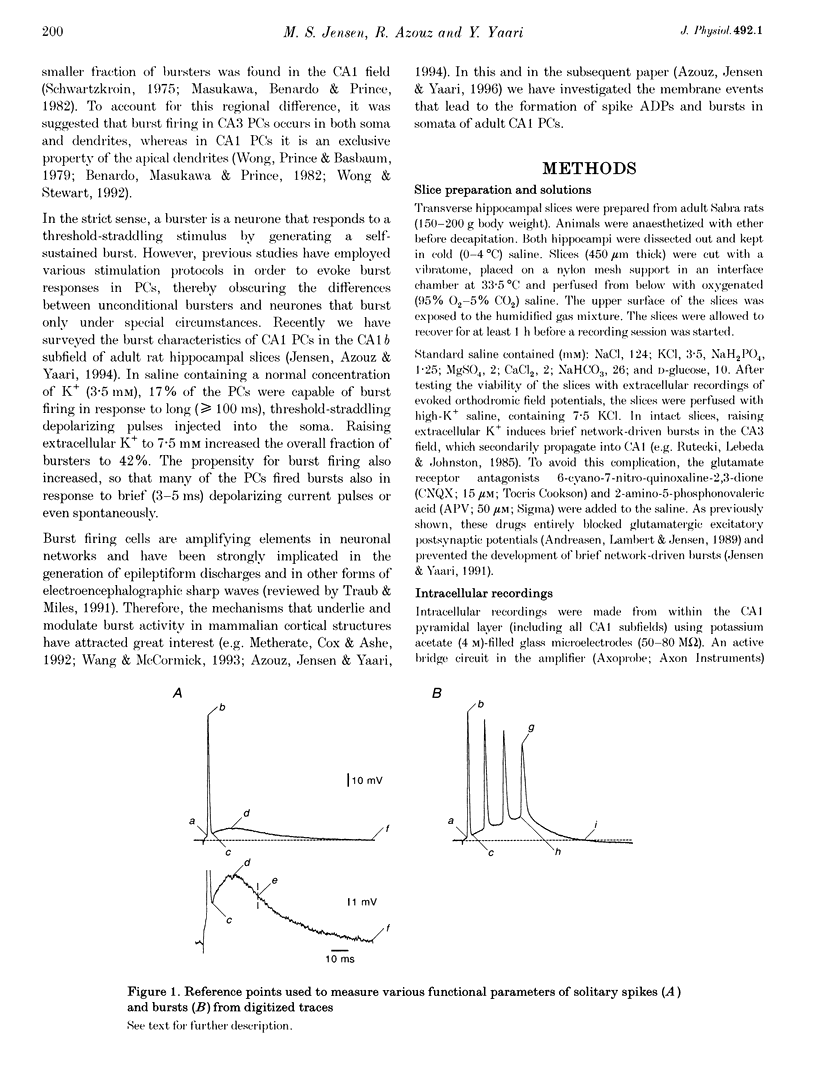
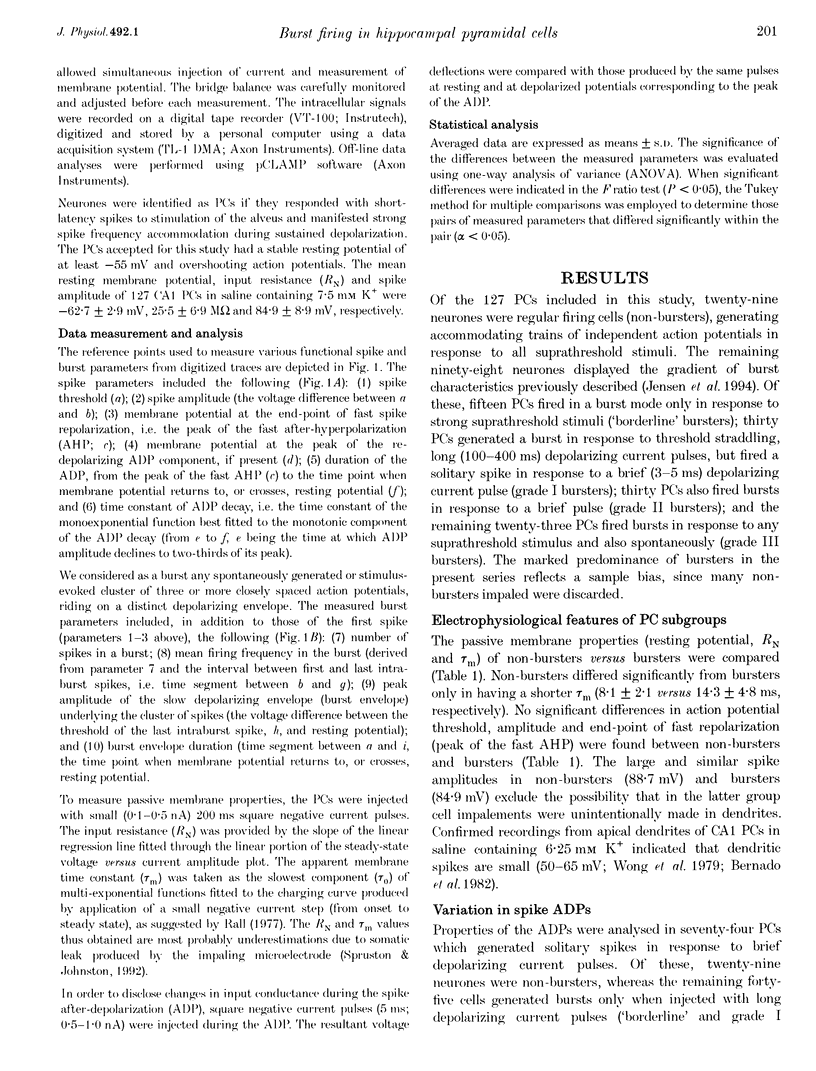
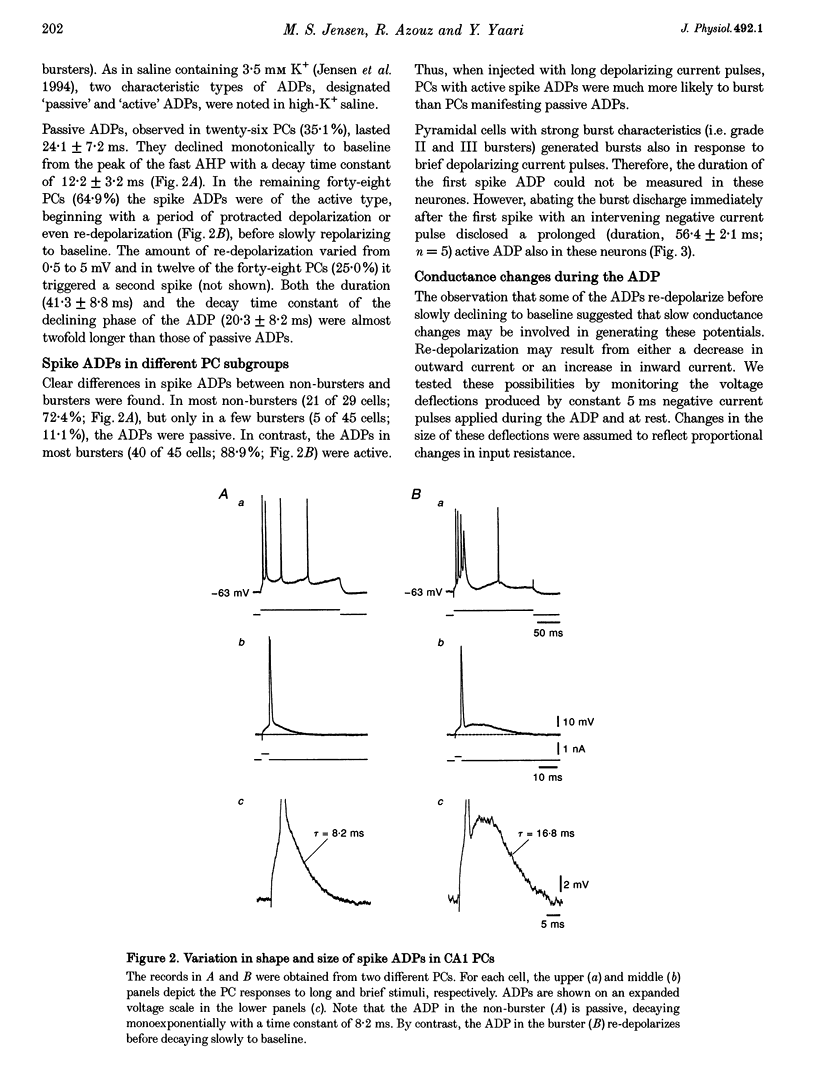
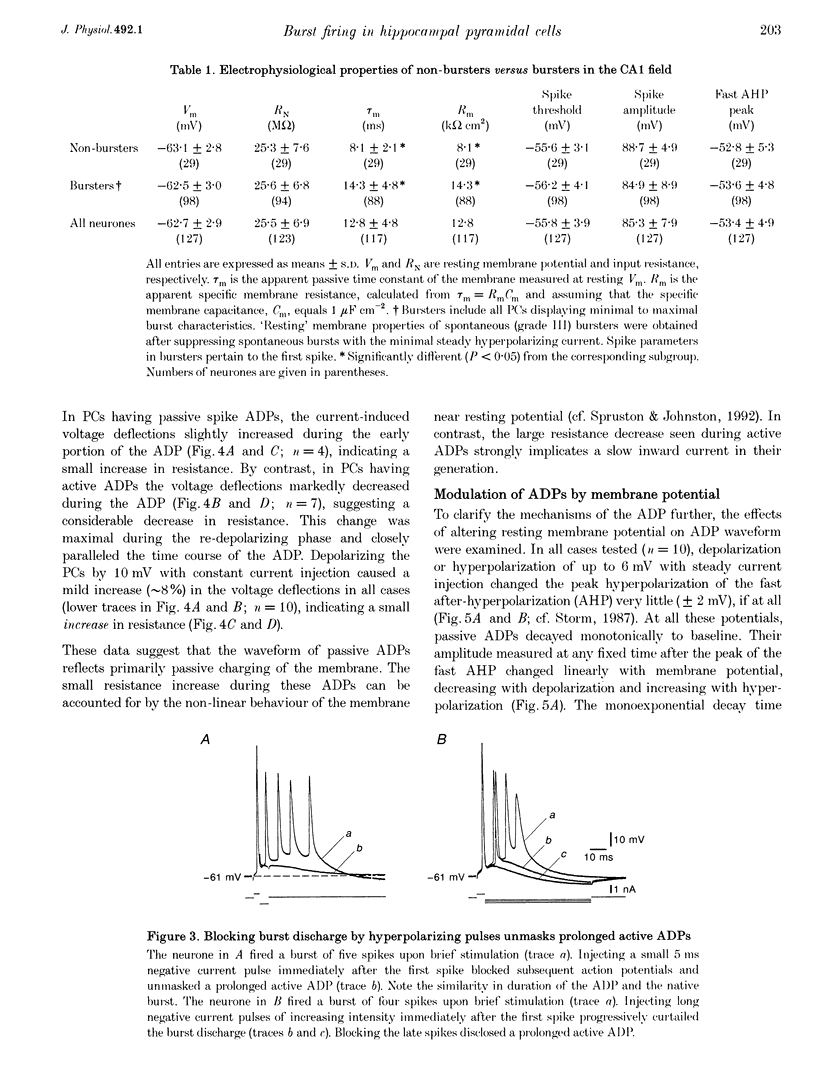
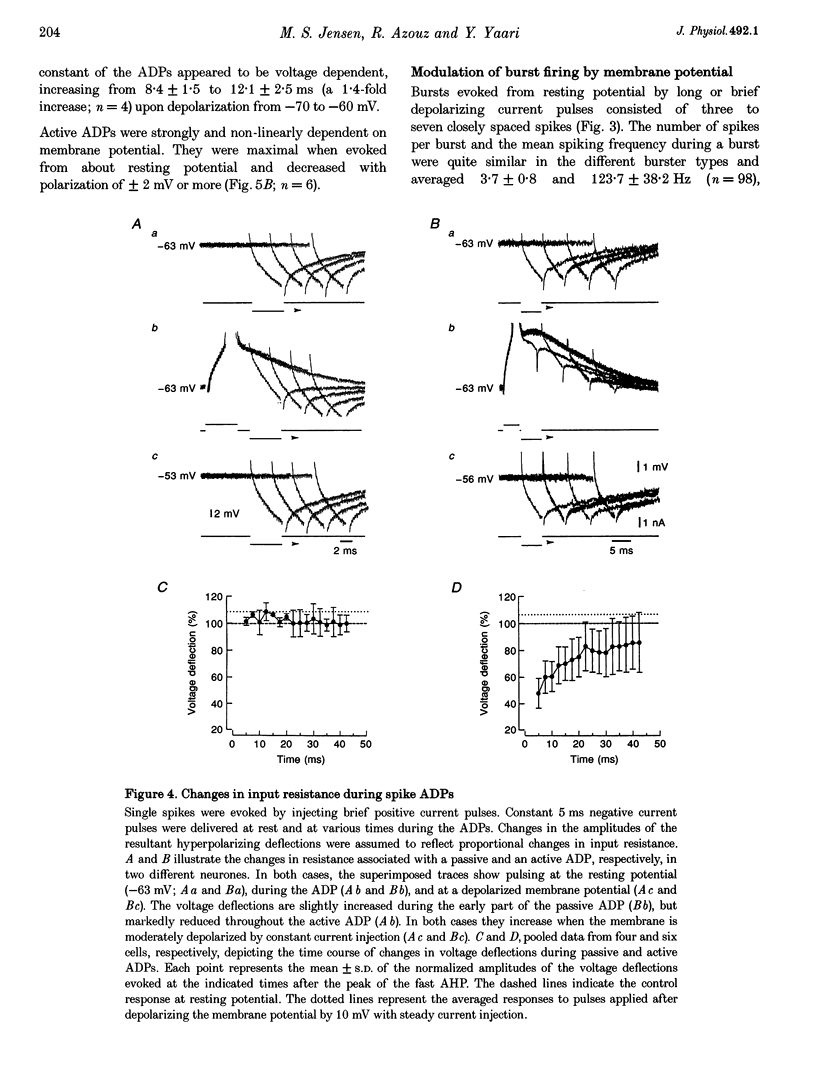
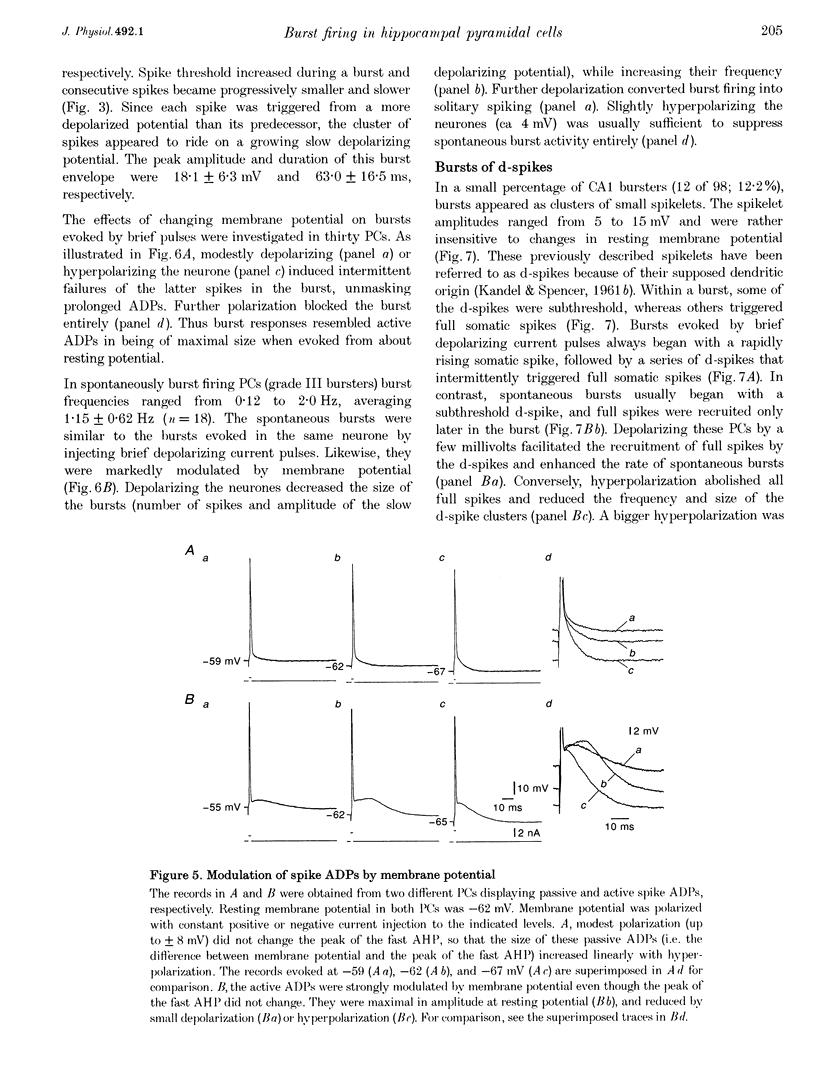
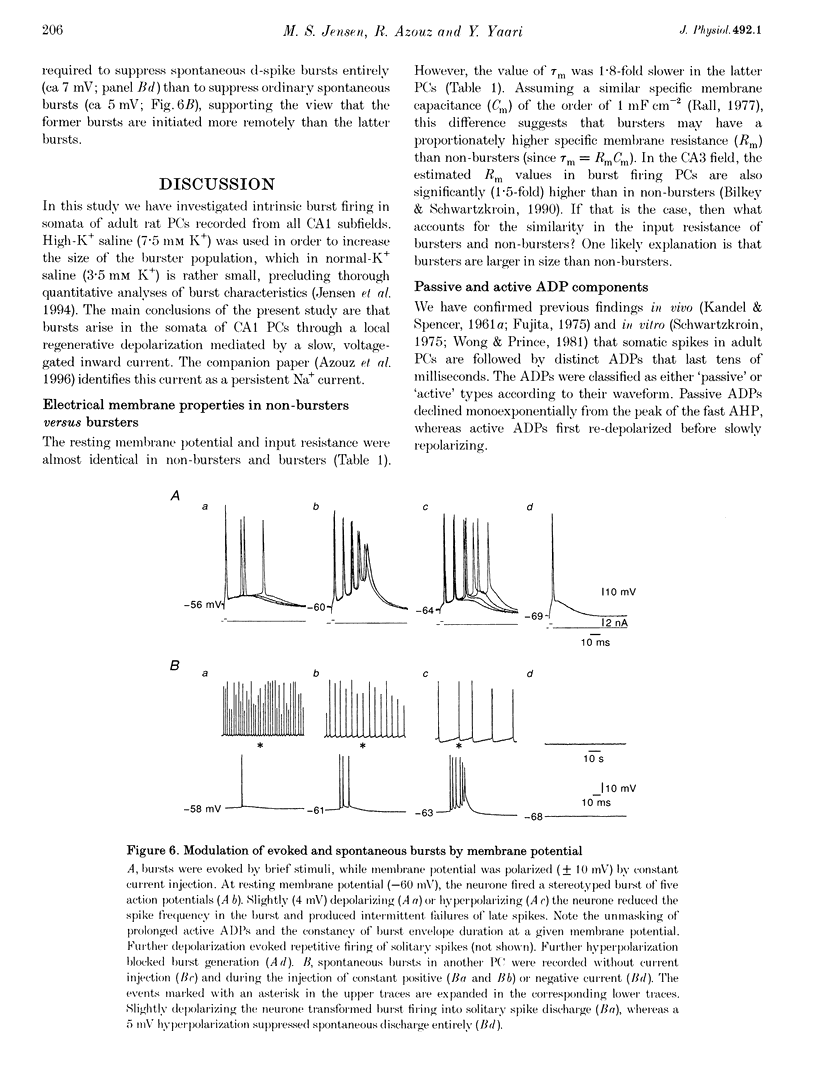
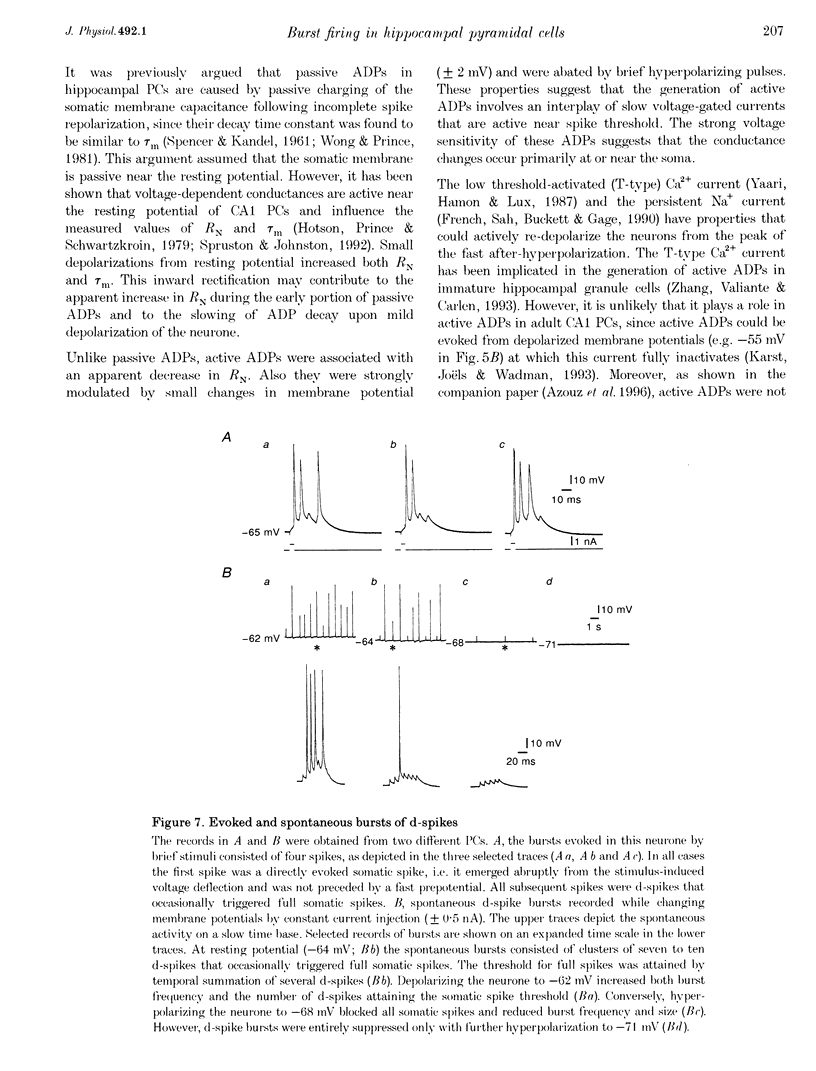
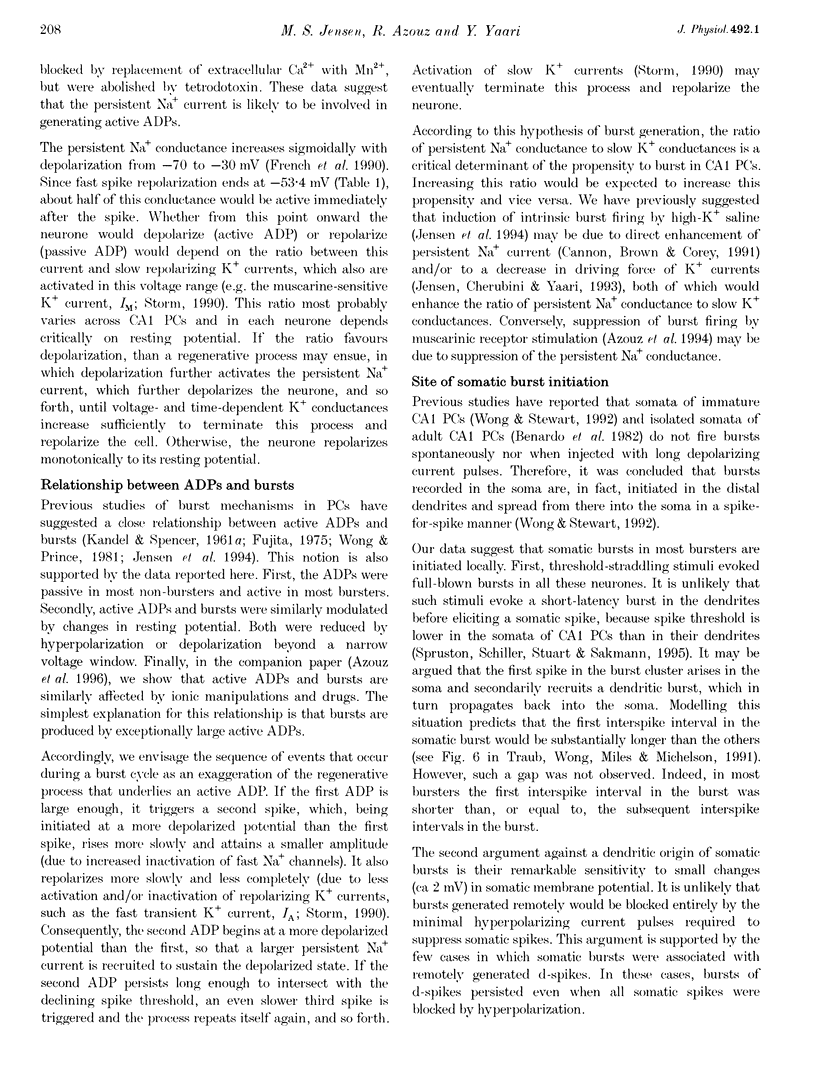
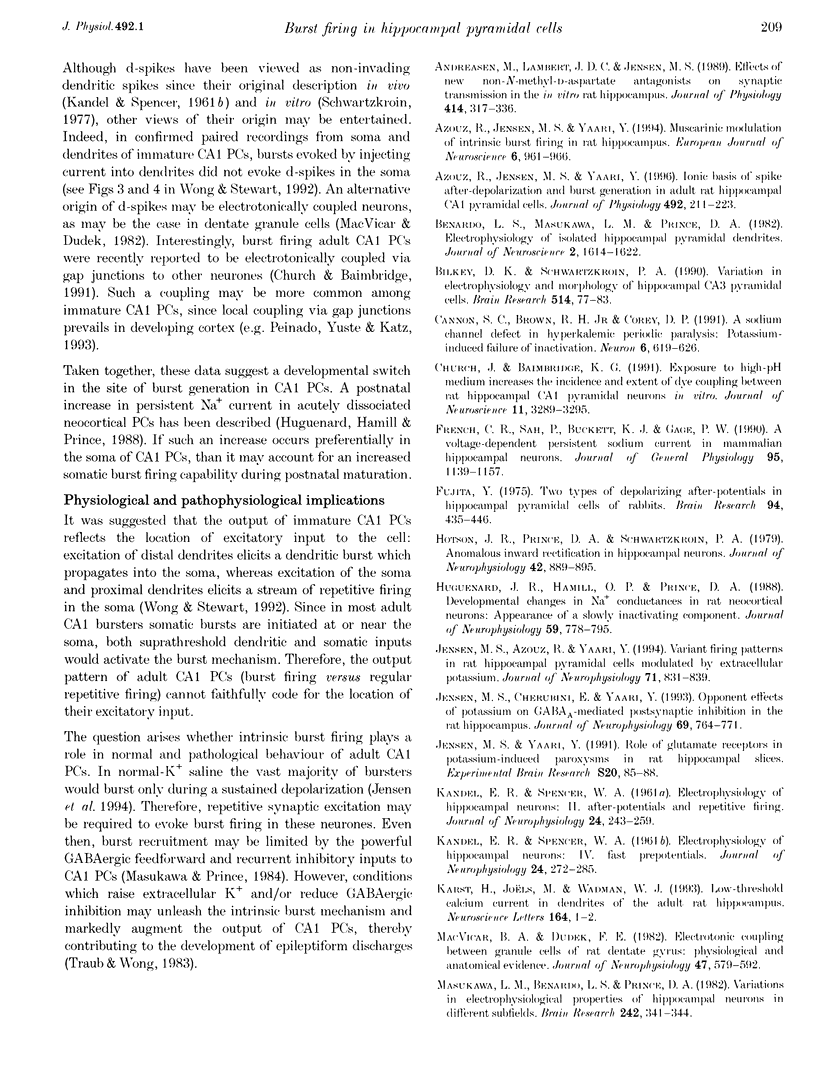
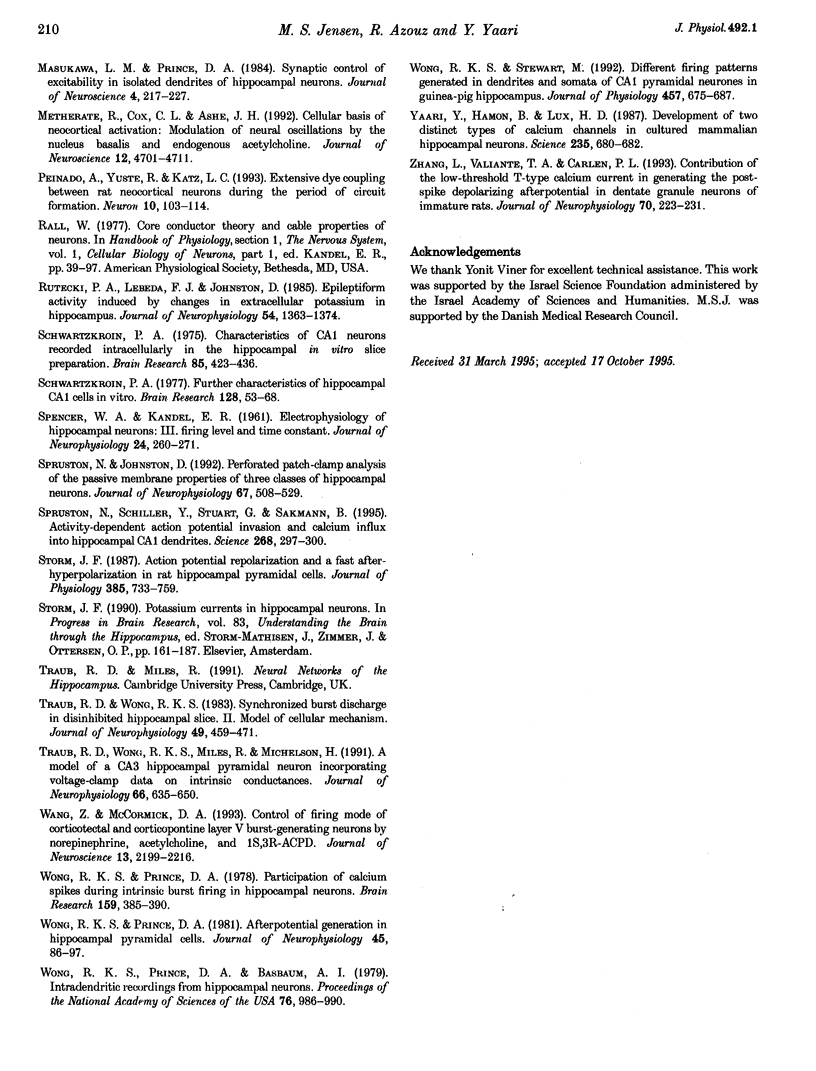
Selected References
These references are in PubMed. This may not be the complete list of references from this article.
- Masukawa L. M., Prince D. A. Synaptic control of excitability in isolated dendrites of hippocampal neurons. J Neurosci. 1984 Jan;4(1):217–227. doi: 10.1523/JNEUROSCI.04-01-00217.1984. [DOI] [PMC free article] [PubMed] [Google Scholar]
- Peinado A., Yuste R., Katz L. C. Extensive dye coupling between rat neocortical neurons during the period of circuit formation. Neuron. 1993 Jan;10(1):103–114. doi: 10.1016/0896-6273(93)90246-n. [DOI] [PubMed] [Google Scholar]
- Rutecki P. A., Lebeda F. J., Johnston D. Epileptiform activity induced by changes in extracellular potassium in hippocampus. J Neurophysiol. 1985 Nov;54(5):1363–1374. doi: 10.1152/jn.1985.54.5.1363. [DOI] [PubMed] [Google Scholar]
- Schwartzkroin P. A. Further characteristics of hippocampal CA1 cells in vitro. Brain Res. 1977 Jun 3;128(1):53–68. doi: 10.1016/0006-8993(77)90235-9. [DOI] [PubMed] [Google Scholar]
- Storm J. F. Action potential repolarization and a fast after-hyperpolarization in rat hippocampal pyramidal cells. J Physiol. 1987 Apr;385:733–759. doi: 10.1113/jphysiol.1987.sp016517. [DOI] [PMC free article] [PubMed] [Google Scholar]
- Storm J. F. Potassium currents in hippocampal pyramidal cells. Prog Brain Res. 1990;83:161–187. doi: 10.1016/s0079-6123(08)61248-0. [DOI] [PubMed] [Google Scholar]
- Traub R. D., Wong R. K., Miles R., Michelson H. A model of a CA3 hippocampal pyramidal neuron incorporating voltage-clamp data on intrinsic conductances. J Neurophysiol. 1991 Aug;66(2):635–650. doi: 10.1152/jn.1991.66.2.635. [DOI] [PubMed] [Google Scholar]
- Wong R. K., Prince D. A. Afterpotential generation in hippocampal pyramidal cells. J Neurophysiol. 1981 Jan;45(1):86–97. doi: 10.1152/jn.1981.45.1.86. [DOI] [PubMed] [Google Scholar]
- Wong R. K., Prince D. A., Basbaum A. I. Intradendritic recordings from hippocampal neurons. Proc Natl Acad Sci U S A. 1979 Feb;76(2):986–990. doi: 10.1073/pnas.76.2.986. [DOI] [PMC free article] [PubMed] [Google Scholar]
- Wong R. K., Prince D. A. Participation of calcium spikes during intrinsic burst firing in hippocampal neurons. Brain Res. 1978 Dec 29;159(2):385–390. doi: 10.1016/0006-8993(78)90544-9. [DOI] [PubMed] [Google Scholar]
- Wong R. K., Stewart M. Different firing patterns generated in dendrites and somata of CA1 pyramidal neurones in guinea-pig hippocampus. J Physiol. 1992 Nov;457:675–687. doi: 10.1113/jphysiol.1992.sp019401. [DOI] [PMC free article] [PubMed] [Google Scholar]
- Yaari Y., Hamon B., Lux H. D. Development of two types of calcium channels in cultured mammalian hippocampal neurons. Science. 1987 Feb 6;235(4789):680–682. doi: 10.1126/science.2433765. [DOI] [PubMed] [Google Scholar]
- Zhang L., Valiante T. A., Carlen P. L. Contribution of the low-threshold T-type calcium current in generating the post-spike depolarizing afterpotential in dentate granule neurons of immature rats. J Neurophysiol. 1993 Jul;70(1):223–231. doi: 10.1152/jn.1993.70.1.223. [DOI] [PubMed] [Google Scholar]