Abstract
1. The Ca2+ content of single mammalian skeletal muscle fibres was determined using a novel technique. Mechanically skinned fibres were equilibrated with varying amounts of the Ca2+ buffer BAPTA and were then lysed in a detergent-paraffin oil emulsion. The subsequent myofilament force response was used to estimate the additional amount of Ca2+ bound to BAPTA following lysis of intracellular membranes. 2. The total endogenous Ca2+ content (corrected for endogenous Ca2+ buffering) of fast-twitch (FT) and slow-twitch (ST) fibres at a myoplasmic pCa (-log[Ca2+]) of 7.15 was 1.32 +/- 0.02 and 1.35 +/- 0.08 mM per fibre volume, respectively. The sarcoplasmic reticulum (SR) component of these estimates was calculated as 1.01 and 1.14 mM, respectively, which normalized to SR volume corresponds to resting SR Ca2+ contents of 11 and 21 mM, respectively. 3. Equilibration of 'resting' fibres with low myoplasmic [Ca2+] (pCa 7.67-9.00) elicited a time-dependent decrease in Ca2+ content in both fibre types. Equilibration of resting fibres with higher myoplasmic [Ca2+] (pCa 5.96-6.32) had no effect on the Ca2+ content of ST fibres but increased the Ca2+ content of FT fibres. The maximum steady-state total Ca2+ content (3.85 mM) was achieved in FT fibres after 3 min equilibration at pCa 5.96. Equilibration at higher myoplasmic [Ca2+] was less effective, probably due to Ca2(+)-induced Ca2+ release from the SR. 4. Exposure of fibres to either caffeine (30 mM, pCa approximately 8, 2 min) or low myoplasmic [Mg2+] (0.05 mM, pCa approximately 9, 1 min) released approximately 85% of the resting SR Ca2+ content. The ability of caffeine to release SR Ca2+ was dependent on the myoplasmic Ca2+ buffering conditions. 5. The results demonstrate that the SR of ST fibres is saturated with Ca2+ at resting myoplasmic [Ca2+] while the SR of FT fibres is only about one-third saturated with Ca2+ under equivalent conditions. These differences suggest that the rate of SR Ca2+ uptake in FT fibres is predominantly controlled by myoplasmic [Ca2+] while that of ST fibres is more likely to be limited by the [Ca2+] within the SR lumen.
Full text
PDF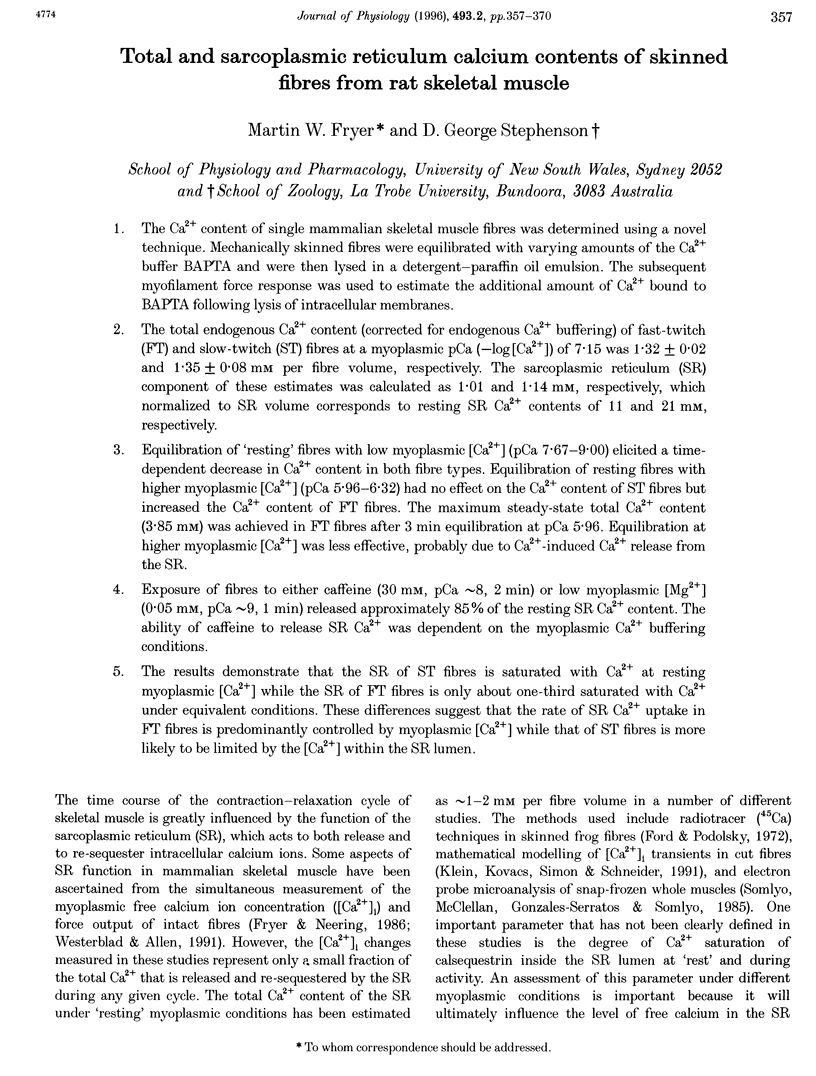
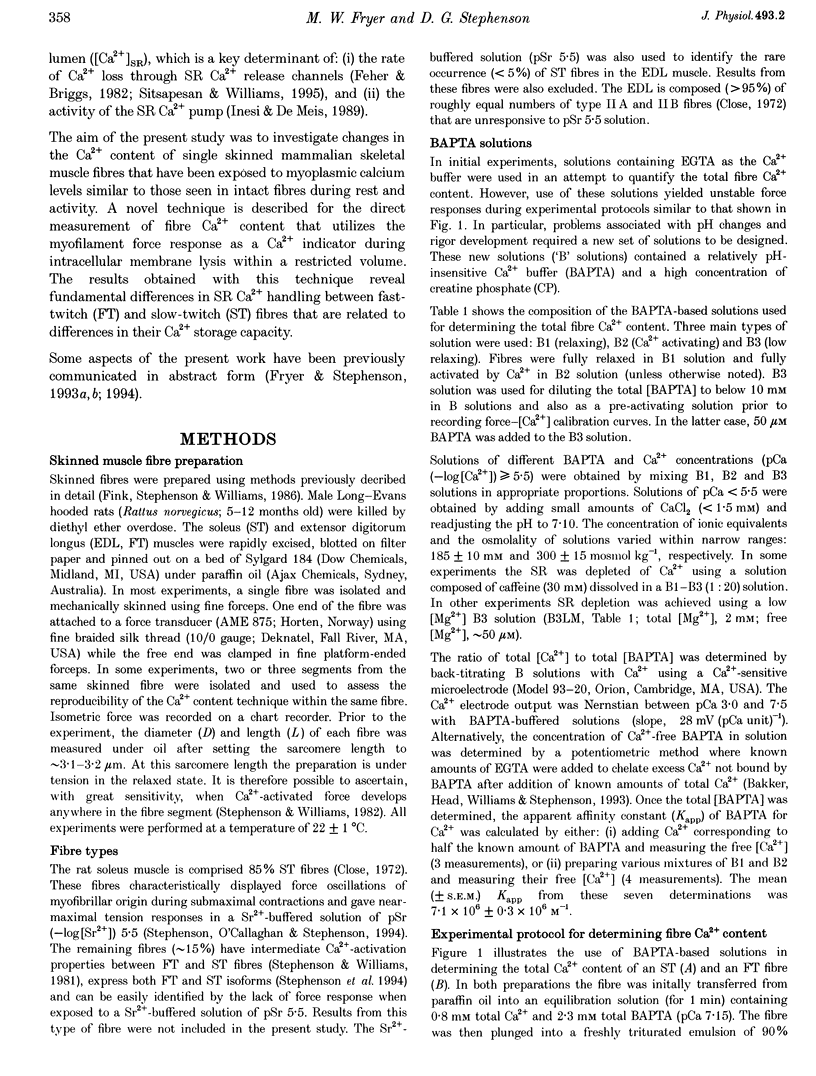
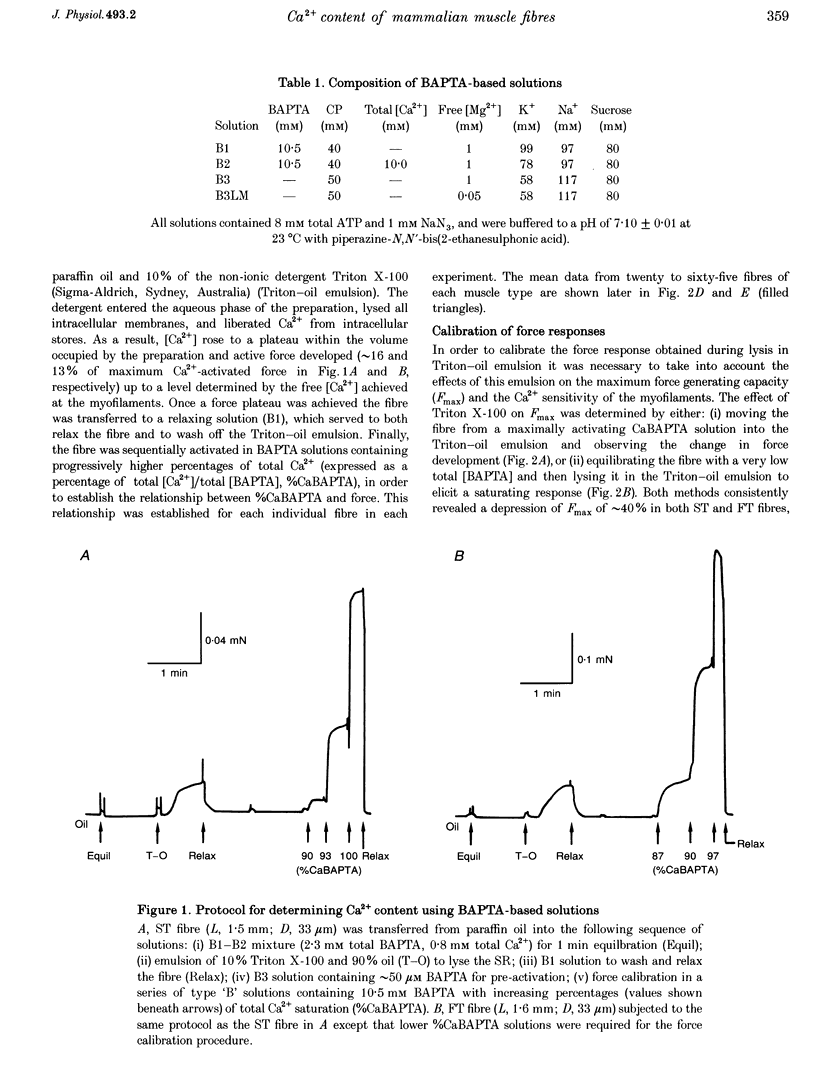
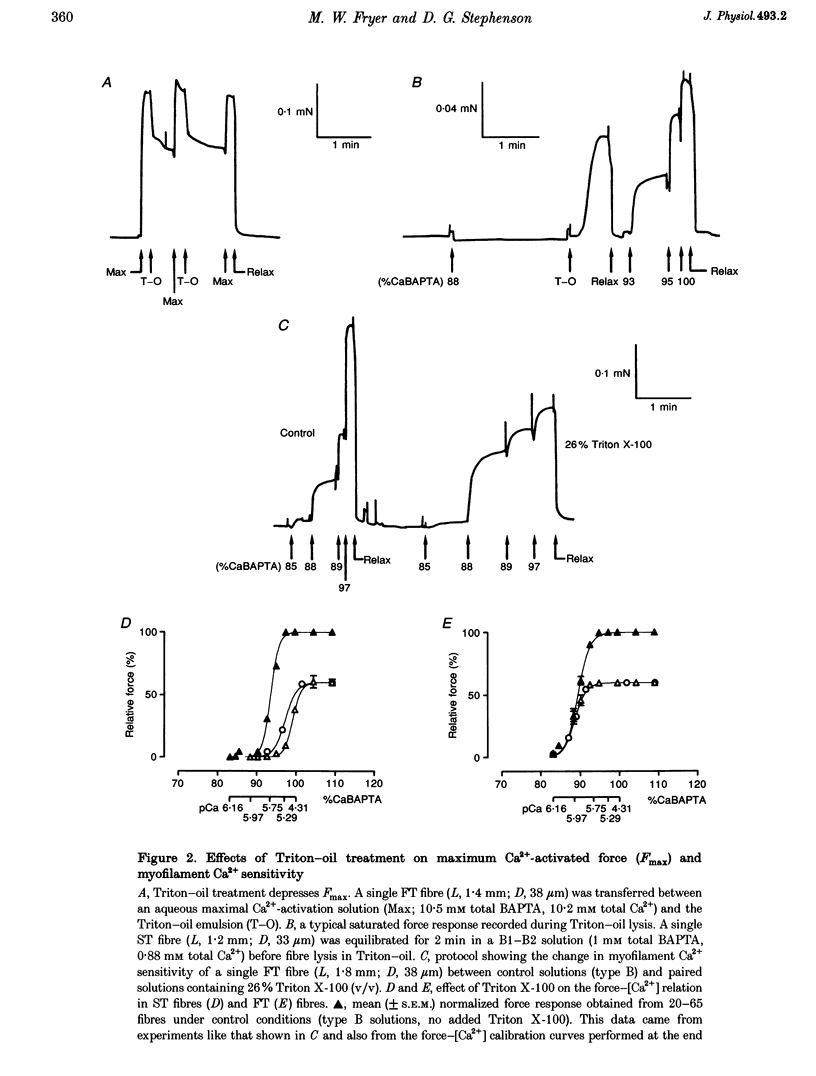
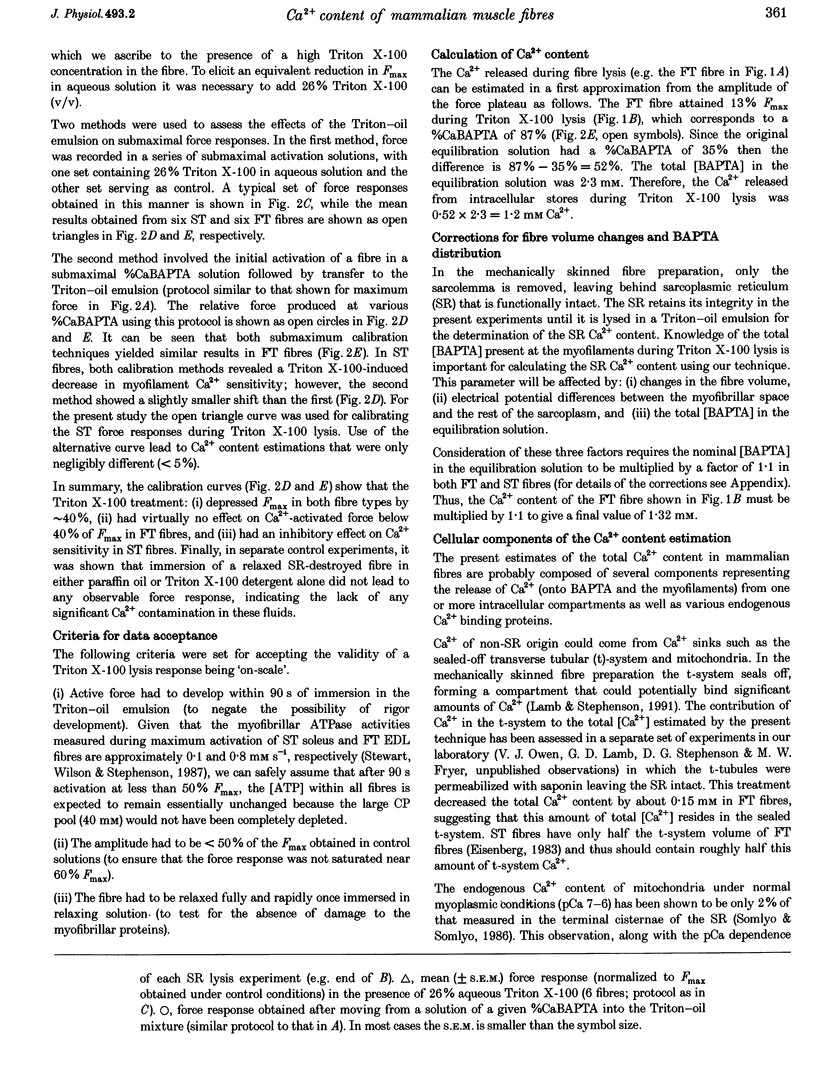
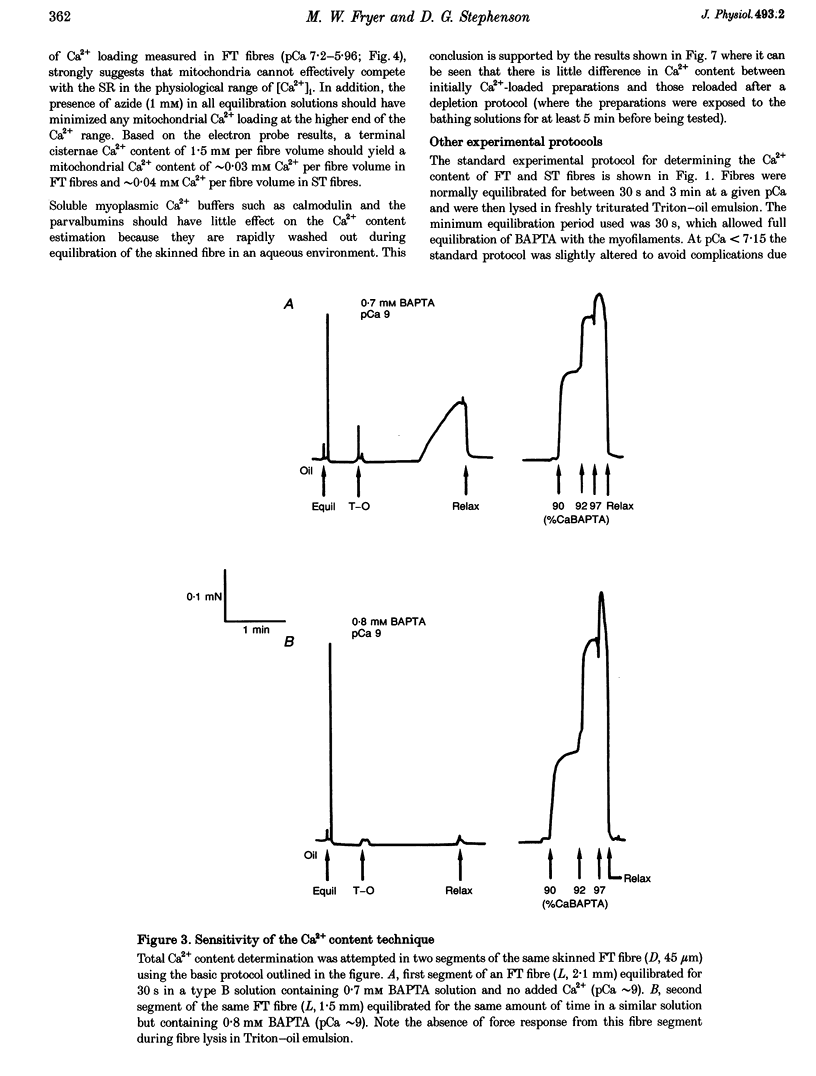
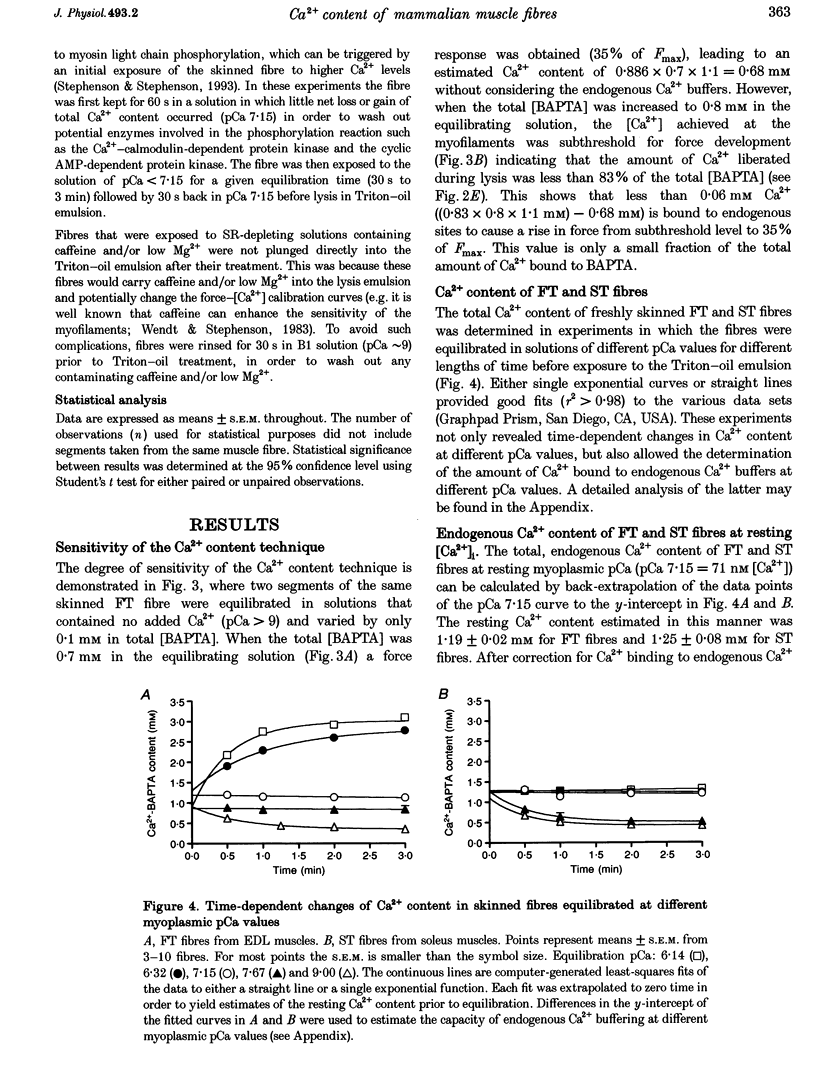
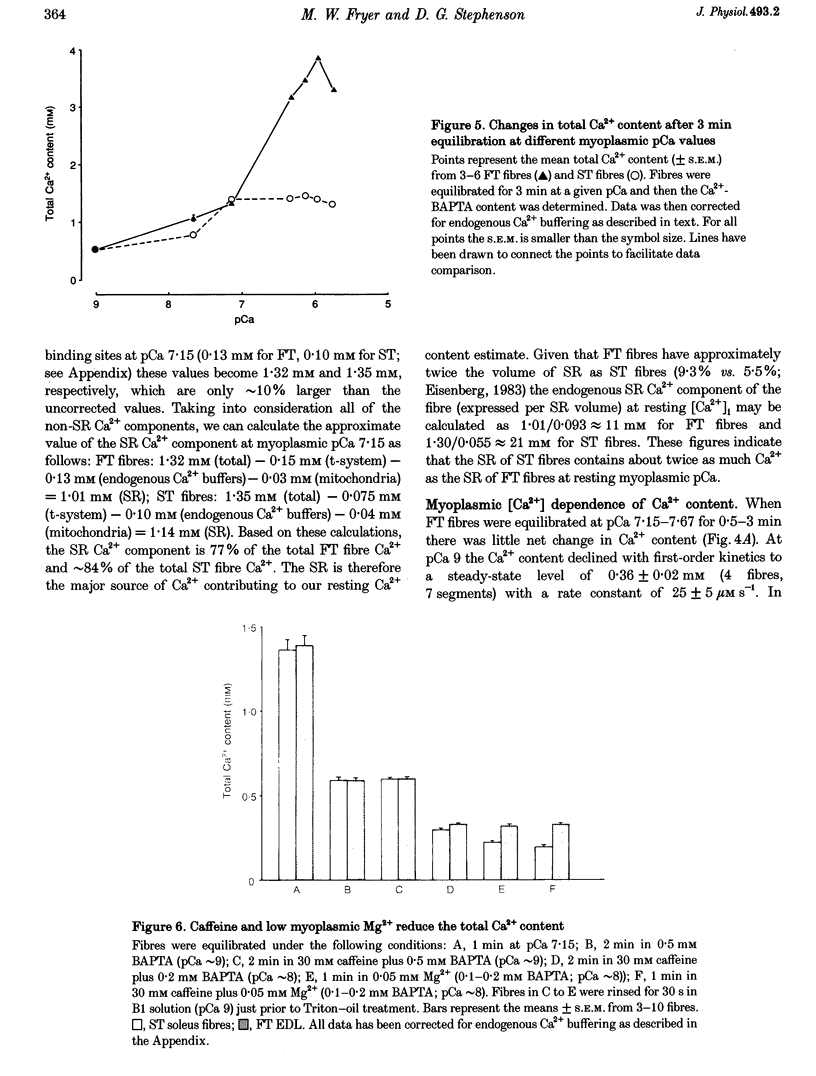
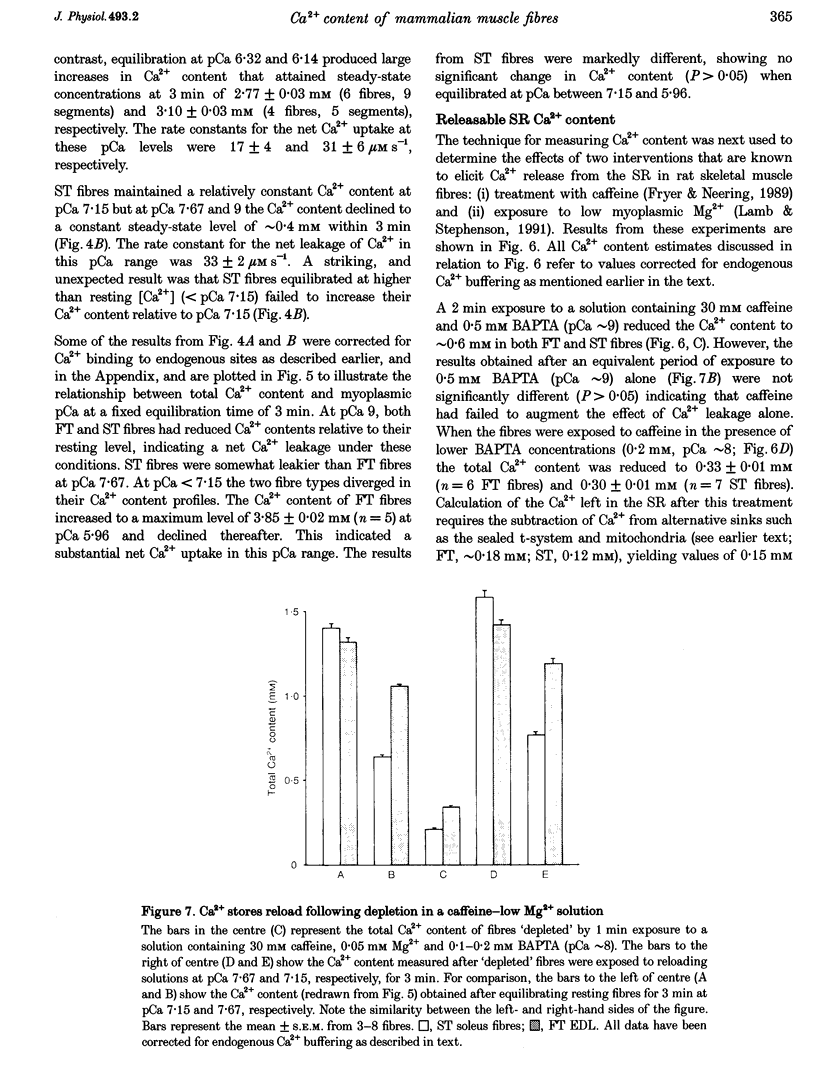
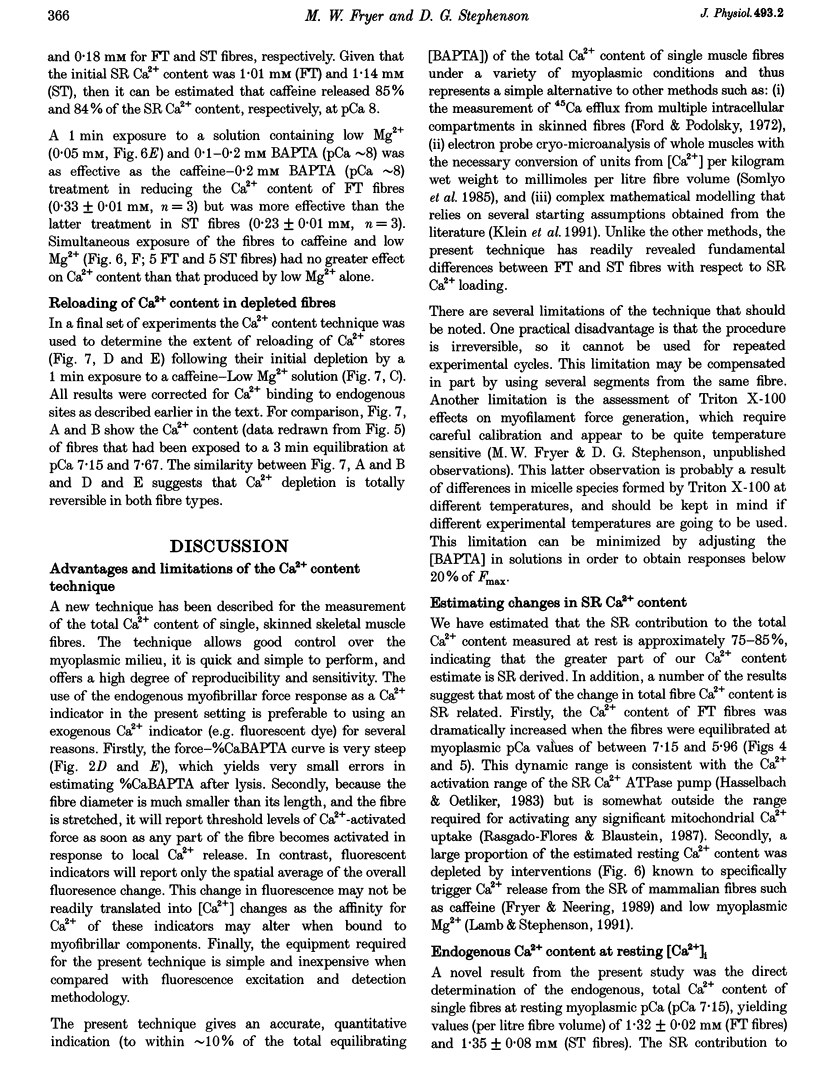
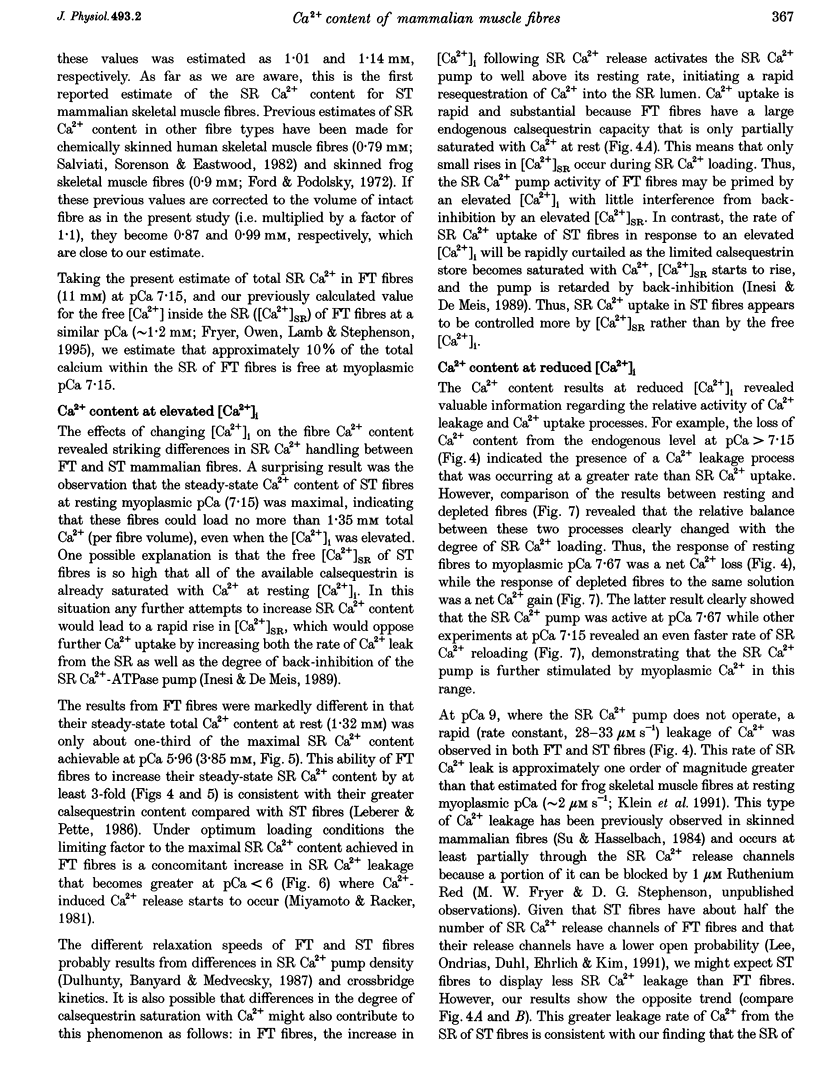
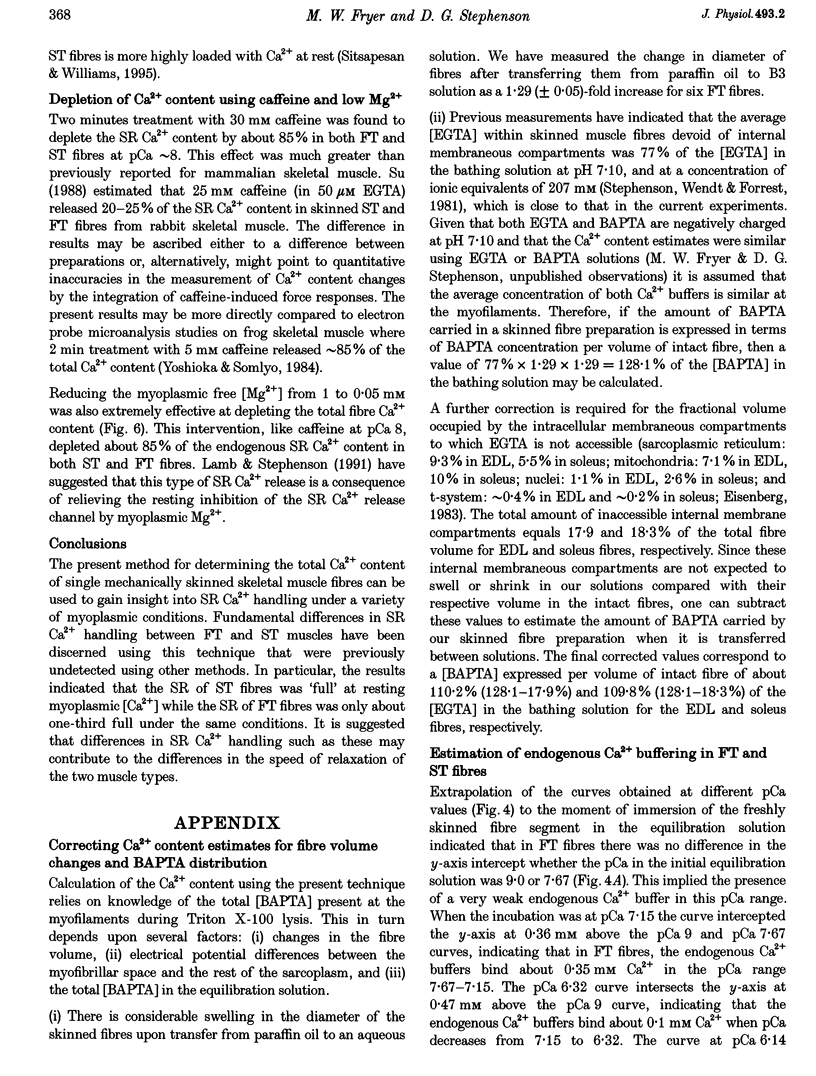
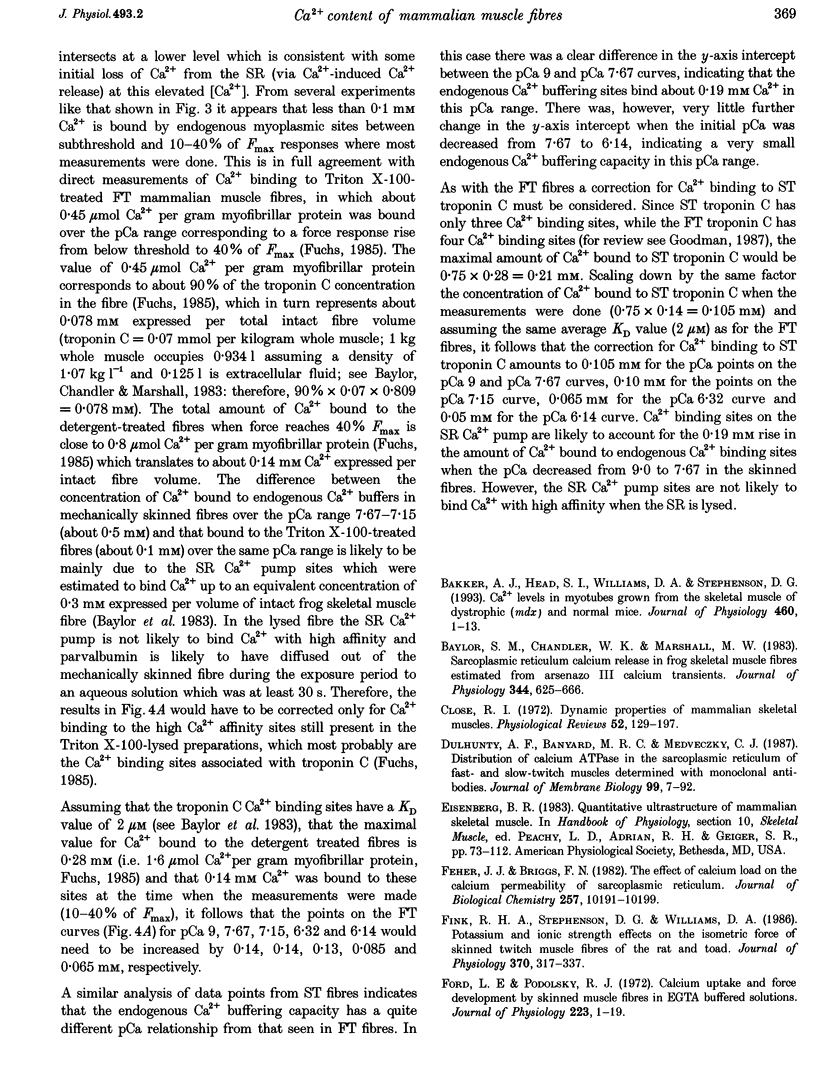
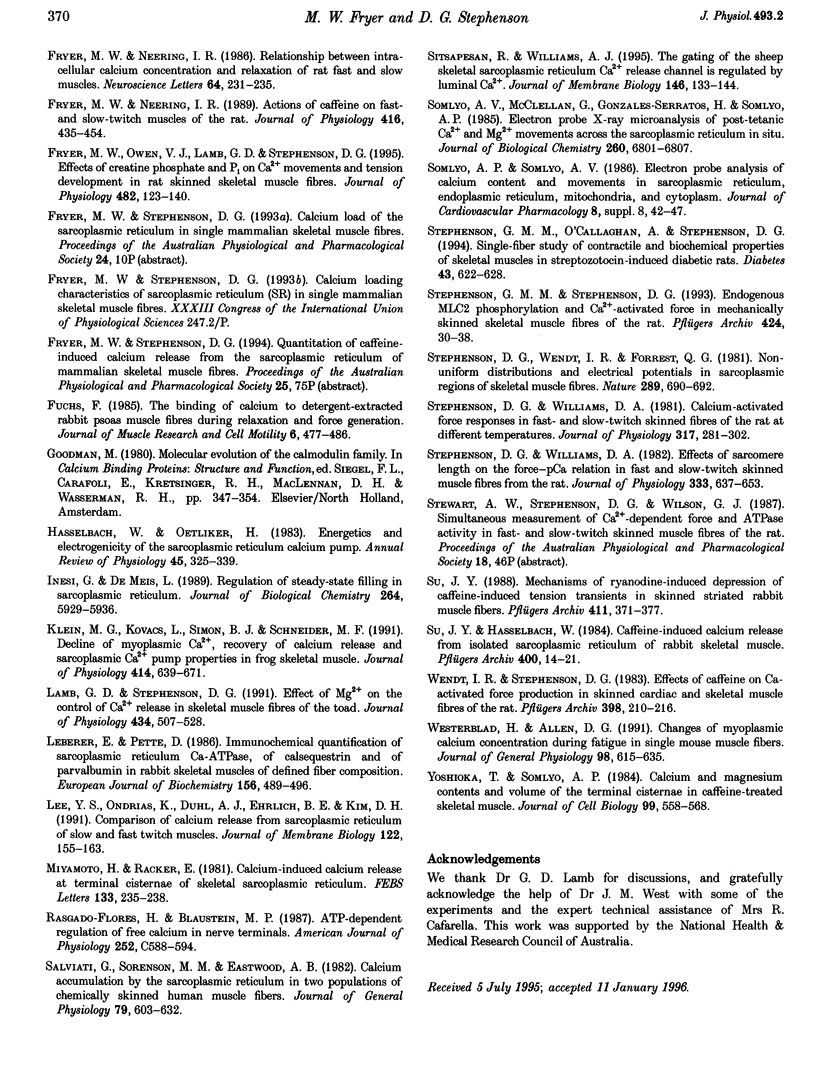
Images in this article
Selected References
These references are in PubMed. This may not be the complete list of references from this article.
- Bakker A. J., Head S. I., Williams D. A., Stephenson D. G. Ca2+ levels in myotubes grown from the skeletal muscle of dystrophic (mdx) and normal mice. J Physiol. 1993 Jan;460:1–13. doi: 10.1113/jphysiol.1993.sp019455. [DOI] [PMC free article] [PubMed] [Google Scholar]
- Baylor S. M., Chandler W. K., Marshall M. W. Sarcoplasmic reticulum calcium release in frog skeletal muscle fibres estimated from Arsenazo III calcium transients. J Physiol. 1983 Nov;344:625–666. doi: 10.1113/jphysiol.1983.sp014959. [DOI] [PMC free article] [PubMed] [Google Scholar]
- Close R. I. Dynamic properties of mammalian skeletal muscles. Physiol Rev. 1972 Jan;52(1):129–197. doi: 10.1152/physrev.1972.52.1.129. [DOI] [PubMed] [Google Scholar]
- Dulhunty A. F., Banyard M. R., Medveczky C. J. Distribution of calcium ATPase in the sarcoplasmic reticulum of fast- and slow-twitch muscles determined with monoclonal antibodies. J Membr Biol. 1987;99(2):79–92. doi: 10.1007/BF01871228. [DOI] [PubMed] [Google Scholar]
- Feher J. J., Briggs F. N. The effect of calcium load on the calcium permeability of sarcoplasmic reticulum. J Biol Chem. 1982 Sep 10;257(17):10191–10199. [PubMed] [Google Scholar]
- Fink R. H., Stephenson D. G., Williams D. A. Potassium and ionic strength effects on the isometric force of skinned twitch muscle fibres of the rat and toad. J Physiol. 1986 Jan;370:317–337. doi: 10.1113/jphysiol.1986.sp015937. [DOI] [PMC free article] [PubMed] [Google Scholar]
- Ford L. E., Podolsky R. J. Calcium uptake and force development by skinned muscle fibres in EGTA buffered solutions. J Physiol. 1972 May;223(1):1–19. doi: 10.1113/jphysiol.1972.sp009830. [DOI] [PMC free article] [PubMed] [Google Scholar]
- Fryer M. W., Neering I. R. Actions of caffeine on fast- and slow-twitch muscles of the rat. J Physiol. 1989 Sep;416:435–454. doi: 10.1113/jphysiol.1989.sp017770. [DOI] [PMC free article] [PubMed] [Google Scholar]
- Fryer M. W., Neering I. R. Relationship between intracellular calcium concentration and relaxation of rat fast and slow muscles. Neurosci Lett. 1986 Feb 28;64(2):231–235. doi: 10.1016/0304-3940(86)90106-0. [DOI] [PubMed] [Google Scholar]
- Fryer M. W., Owen V. J., Lamb G. D., Stephenson D. G. Effects of creatine phosphate and P(i) on Ca2+ movements and tension development in rat skinned skeletal muscle fibres. J Physiol. 1995 Jan 1;482(Pt 1):123–140. doi: 10.1113/jphysiol.1995.sp020504. [DOI] [PMC free article] [PubMed] [Google Scholar]
- Fuchs F. The binding of calcium to detergent-extracted rabbit psoas muscle fibres during relaxation and force generation. J Muscle Res Cell Motil. 1985 Aug;6(4):477–486. doi: 10.1007/BF00712584. [DOI] [PubMed] [Google Scholar]
- Hasselbach W., Oetliker H. Energetics and electrogenicity of the sarcoplasmic reticulum calcium pump. Annu Rev Physiol. 1983;45:325–339. doi: 10.1146/annurev.ph.45.030183.001545. [DOI] [PubMed] [Google Scholar]
- Inesi G., de Meis L. Regulation of steady state filling in sarcoplasmic reticulum. Roles of back-inhibition, leakage, and slippage of the calcium pump. J Biol Chem. 1989 Apr 5;264(10):5929–5936. [PubMed] [Google Scholar]
- Klein M. G., Kovacs L., Simon B. J., Schneider M. F. Decline of myoplasmic Ca2+, recovery of calcium release and sarcoplasmic Ca2+ pump properties in frog skeletal muscle. J Physiol. 1991 Sep;441:639–671. doi: 10.1113/jphysiol.1991.sp018771. [DOI] [PMC free article] [PubMed] [Google Scholar]
- Lamb G. D., Stephenson D. G. Effect of Mg2+ on the control of Ca2+ release in skeletal muscle fibres of the toad. J Physiol. 1991 Mar;434:507–528. doi: 10.1113/jphysiol.1991.sp018483. [DOI] [PMC free article] [PubMed] [Google Scholar]
- Leberer E., Pette D. Immunochemical quantification of sarcoplasmic reticulum Ca-ATPase, of calsequestrin and of parvalbumin in rabbit skeletal muscles of defined fiber composition. Eur J Biochem. 1986 May 2;156(3):489–496. doi: 10.1111/j.1432-1033.1986.tb09607.x. [DOI] [PubMed] [Google Scholar]
- Lee Y. S., Ondrias K., Duhl A. J., Ehrlich B. E., Kim D. H. Comparison of calcium release from sarcoplasmic reticulum of slow and fast twitch muscles. J Membr Biol. 1991 Jun;122(2):155–163. doi: 10.1007/BF01872638. [DOI] [PubMed] [Google Scholar]
- Miyamoto H., Racker E. Calcium-induced calcium release at terminal cisternae of skeletal sarcoplasmic reticulum. FEBS Lett. 1981 Oct 26;133(2):235–238. doi: 10.1016/0014-5793(81)80513-3. [DOI] [PubMed] [Google Scholar]
- Rasgado-Flores H., Blaustein M. P. ATP-dependent regulation of cytoplasmic free calcium in nerve terminals. Am J Physiol. 1987 Jun;252(6 Pt 1):C588–C594. doi: 10.1152/ajpcell.1987.252.6.C588. [DOI] [PubMed] [Google Scholar]
- Salviati G., Sorenson M. M., Eastwood A. B. Calcium accumulation by the sarcoplasmic reticulum in two populations of chemically skinned human muscle fibers. Effects of calcium and cyclic AMP. J Gen Physiol. 1982 Apr;79(4):603–632. doi: 10.1085/jgp.79.4.603. [DOI] [PMC free article] [PubMed] [Google Scholar]
- Sitsapesan R., Williams A. J. The gating of the sheep skeletal sarcoplasmic reticulum Ca(2+)-release channel is regulated by luminal Ca2+. J Membr Biol. 1995 Jul;146(2):133–144. doi: 10.1007/BF00238004. [DOI] [PubMed] [Google Scholar]
- Somlyo A. V., McClellan G., Gonzalez-Serratos H., Somlyo A. P. Electron probe X-ray microanalysis of post-tetanic Ca2+ and Mg2+ movements across the sarcoplasmic reticulum in situ. J Biol Chem. 1985 Jun 10;260(11):6801–6807. [PubMed] [Google Scholar]
- Stephenson D. G., Wendt I. R., Forrest Q. G. Non-uniform ion distributions and electrical potentials in sarcoplasmic regions of skeletal muscle fibres. Nature. 1981 Feb 19;289(5799):690–692. doi: 10.1038/289690a0. [DOI] [PubMed] [Google Scholar]
- Stephenson D. G., Williams D. A. Calcium-activated force responses in fast- and slow-twitch skinned muscle fibres of the rat at different temperatures. J Physiol. 1981 Aug;317:281–302. doi: 10.1113/jphysiol.1981.sp013825. [DOI] [PMC free article] [PubMed] [Google Scholar]
- Stephenson D. G., Williams D. A. Effects of sarcomere length on the force-pCa relation in fast- and slow-twitch skinned muscle fibres from the rat. J Physiol. 1982 Dec;333:637–653. doi: 10.1113/jphysiol.1982.sp014473. [DOI] [PMC free article] [PubMed] [Google Scholar]
- Stephenson G. M., Stephenson D. G. Endogenous MLC2 phosphorylation and Ca(2+)-activated force in mechanically skinned skeletal muscle fibres of the rat. Pflugers Arch. 1993 Jun;424(1):30–38. doi: 10.1007/BF00375099. [DOI] [PubMed] [Google Scholar]
- Su J. Y., Hasselbach W. Caffeine-induced calcium release from isolated sarcoplasmic reticulum of rabbit skeletal muscle. Pflugers Arch. 1984 Jan;400(1):14–21. doi: 10.1007/BF00670530. [DOI] [PubMed] [Google Scholar]
- Su J. Y. Mechanisms of ryanodine-induced depression of caffeine-induced tension transients in skinned striated rabbit muscle fibers. Pflugers Arch. 1988 Apr;411(4):371–377. doi: 10.1007/BF00587715. [DOI] [PubMed] [Google Scholar]
- Wendt I. R., Stephenson D. G. Effects of caffeine on Ca-activated force production in skinned cardiac and skeletal muscle fibres of the rat. Pflugers Arch. 1983 Aug;398(3):210–216. doi: 10.1007/BF00657153. [DOI] [PubMed] [Google Scholar]
- Westerblad H., Allen D. G. Changes of myoplasmic calcium concentration during fatigue in single mouse muscle fibers. J Gen Physiol. 1991 Sep;98(3):615–635. doi: 10.1085/jgp.98.3.615. [DOI] [PMC free article] [PubMed] [Google Scholar]
- Yoshioka T., Somlyo A. P. Calcium and magnesium contents and volume of the terminal cisternae in caffeine-treated skeletal muscle. J Cell Biol. 1984 Aug;99(2):558–568. doi: 10.1083/jcb.99.2.558. [DOI] [PMC free article] [PubMed] [Google Scholar]