Abstract
1. Endothelial control of the rate of constrictions and the underlying pacemaker potentials has been studied in vitro in guinea-pig mesenteric lymphatic vessels. 2. ACh stimulated 60% of intraluminally perfused vessels to slow or abolish lymphatic constrictions. This action was inhibited by atropine and was likely to be due to the release of endothelium-derived nitric oxide (EDNO) as the effect was absent after endothelial lysis, mimicked by sodium nitroprusside (SNP), blocked by N omega-nitro L-arginine (NOLA) and partially inhibited by Methylene Blue (MB). 3. The remaining 40% of perfused vessels did not mechanically respond to ACh or SNP. In four of seven such vessels this appeared to be due to excessive perfusion-associated release of EDNO, as incubation with NOLA restored the response to SNP. 4. Application of NOLA or MB in perfused vessels significantly increased constriction frequency, further indicating perfusion-associated release of EDNO. 5. ACh induced a marked increase in endothelial [Ca2+]i of both mechanically responding and non-responding vessels. This ACh-induced increase could be repetitively induced when Ca2+ was present in the perfusate, but rapidly ran down when a Ca(2+)-free EGTA perfusate was used. 6. Intracellular recordings from the smooth muscle of non-perfused vessel segments demonstrated an ACh-induced hyperpolarization and decrease in membrane resistance, changes which were prevented by atropine, NOLA, MB and endothelial lysis and mimicked by SNP. 7. ACh directly reduced the size of the underlying pacemaker potentials termed spontaneous transient depolarizations (STDs). 8. NOLA and MB enhanced STDs in non-perfused vessel segments indicating an endogenous release of EDNO. 9. It is concluded that the lymphatic endothelium produces and releases EDNO endogenously, during perfusion or after stimulation with ACh, to decrease the efficacy of STDs to generate action potentials and resultant constrictions.
Full text
PDF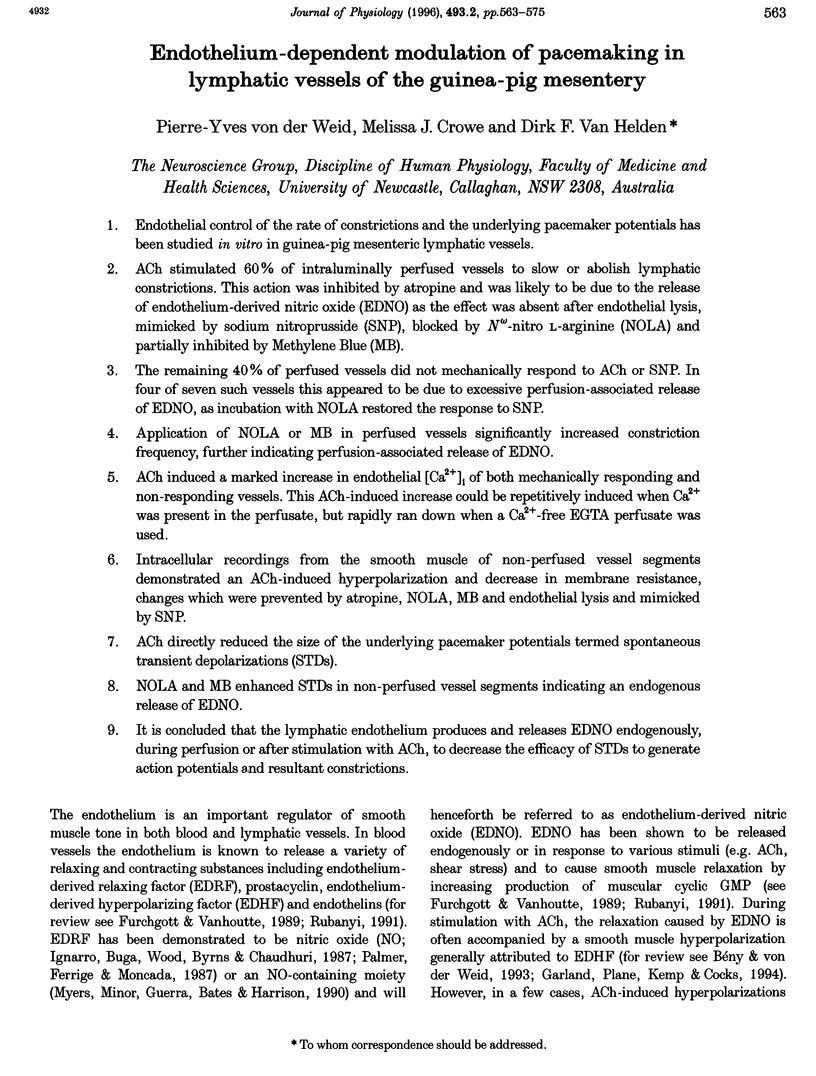
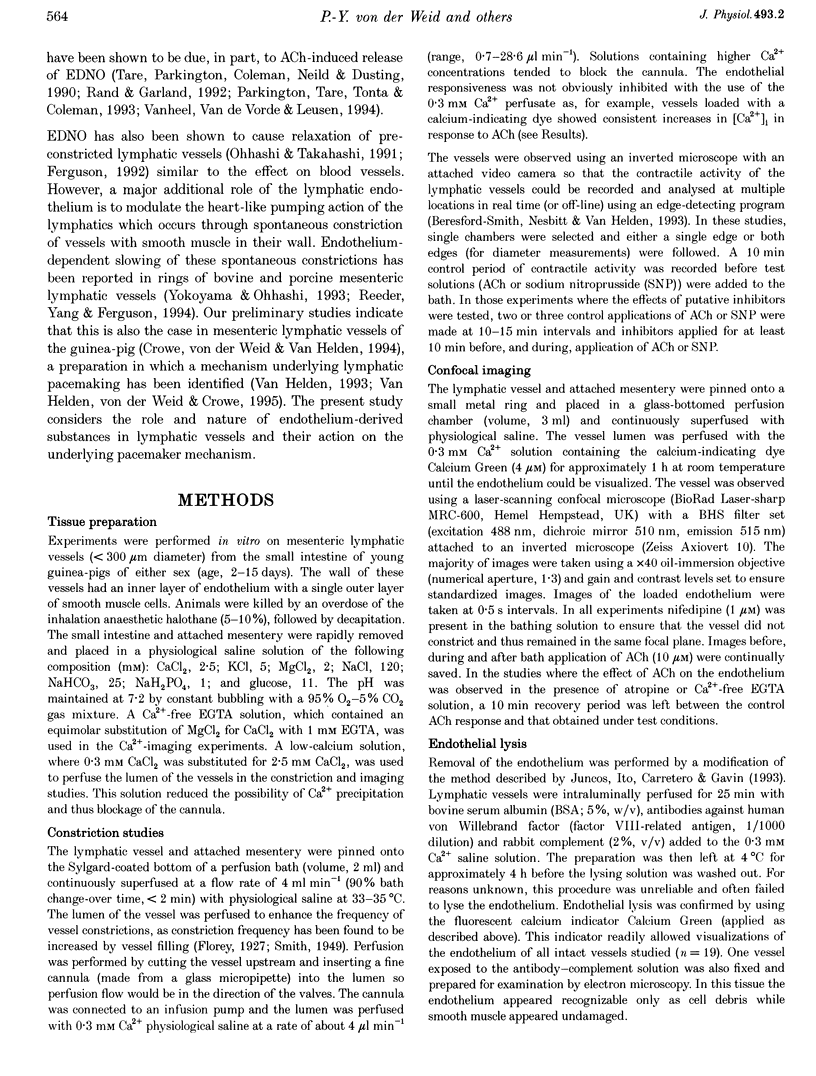
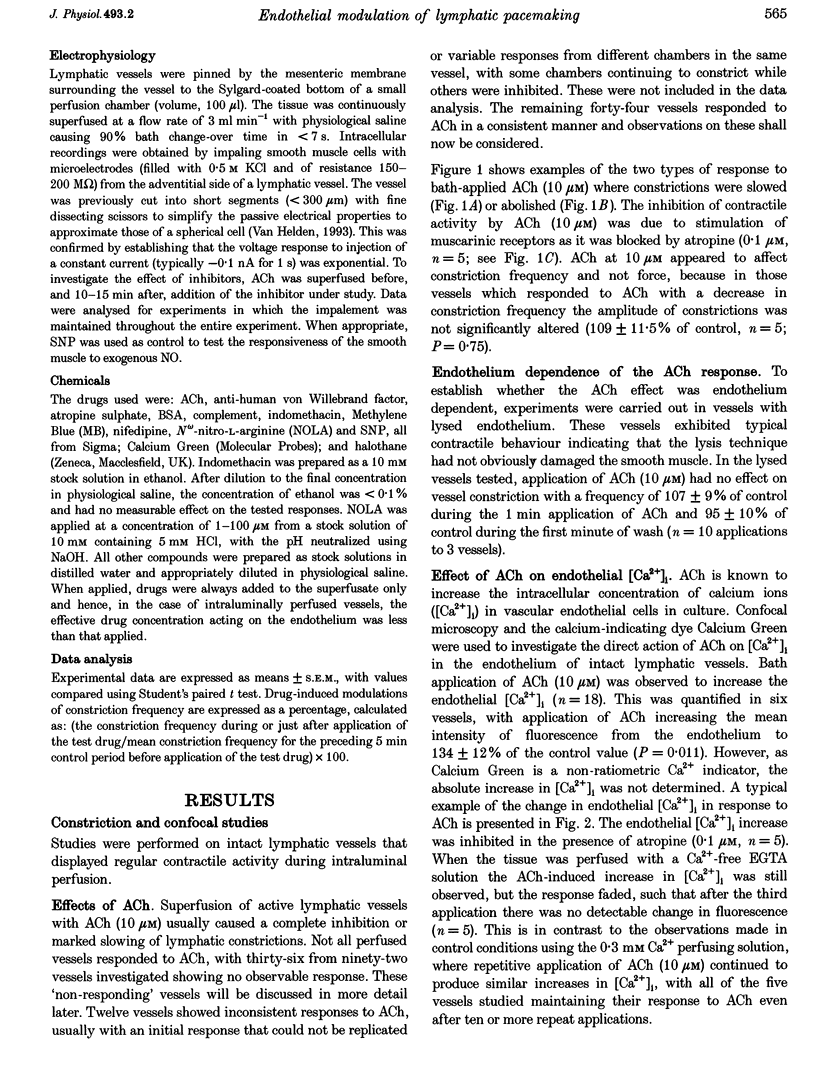
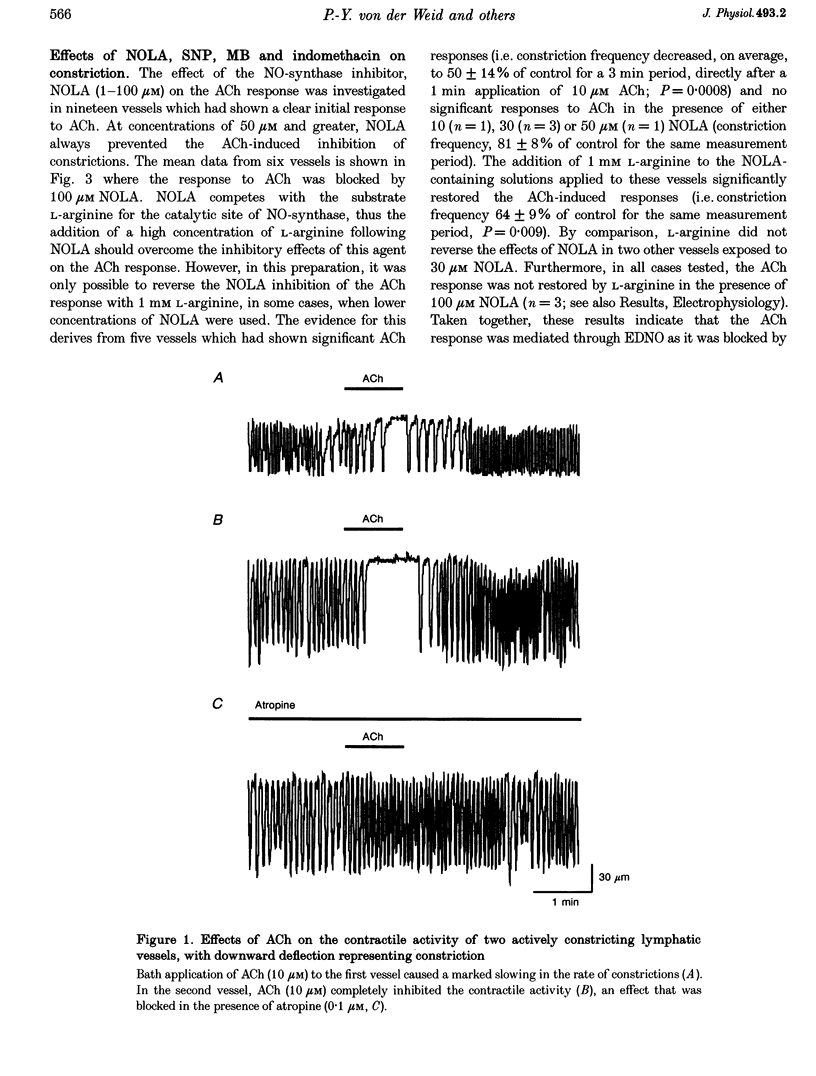
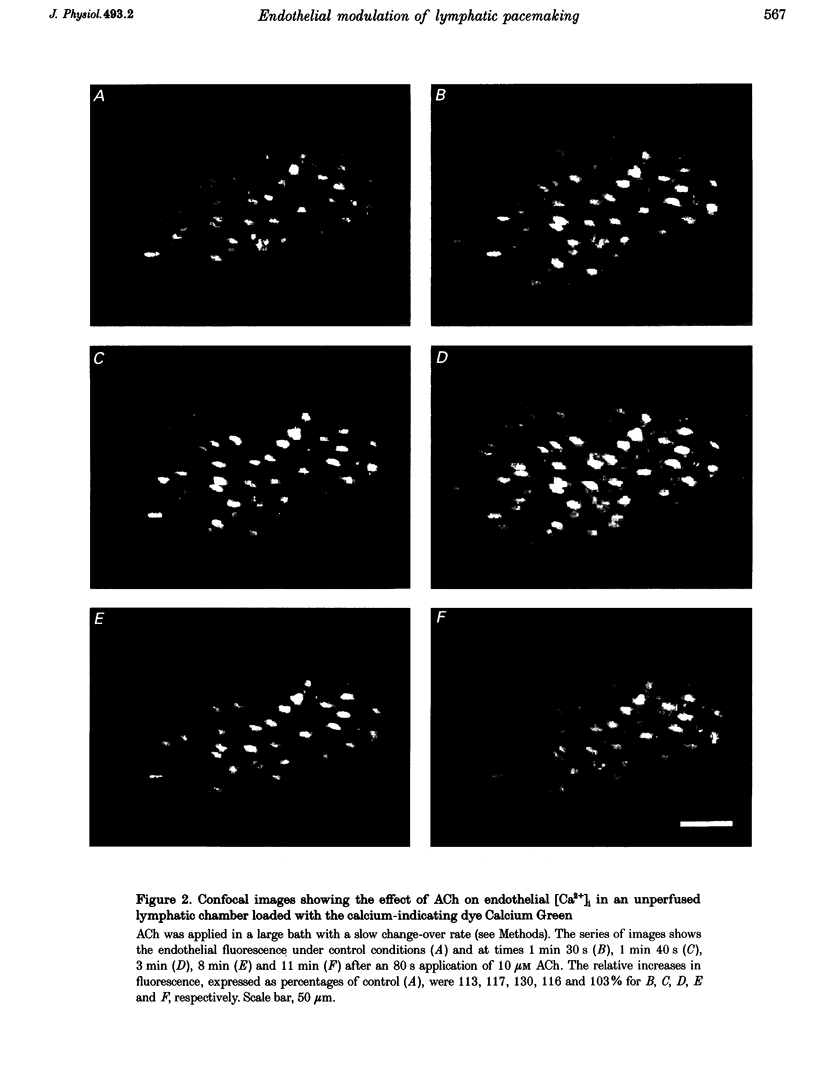
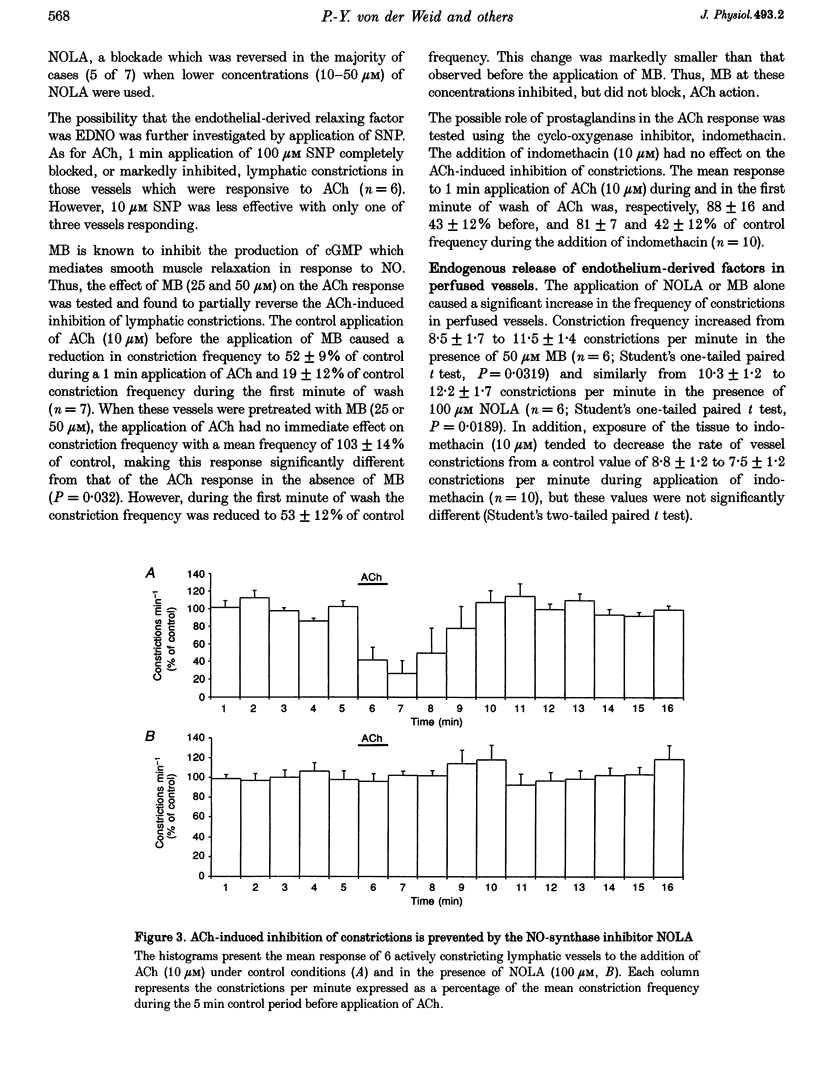
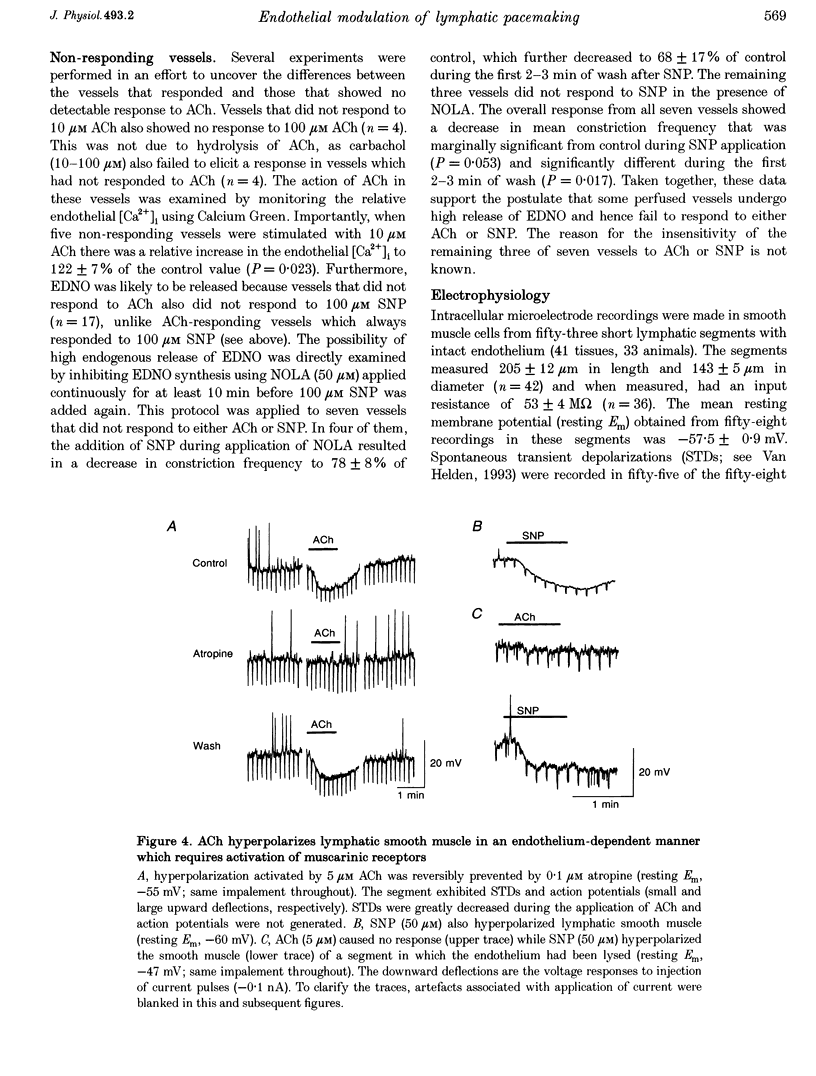
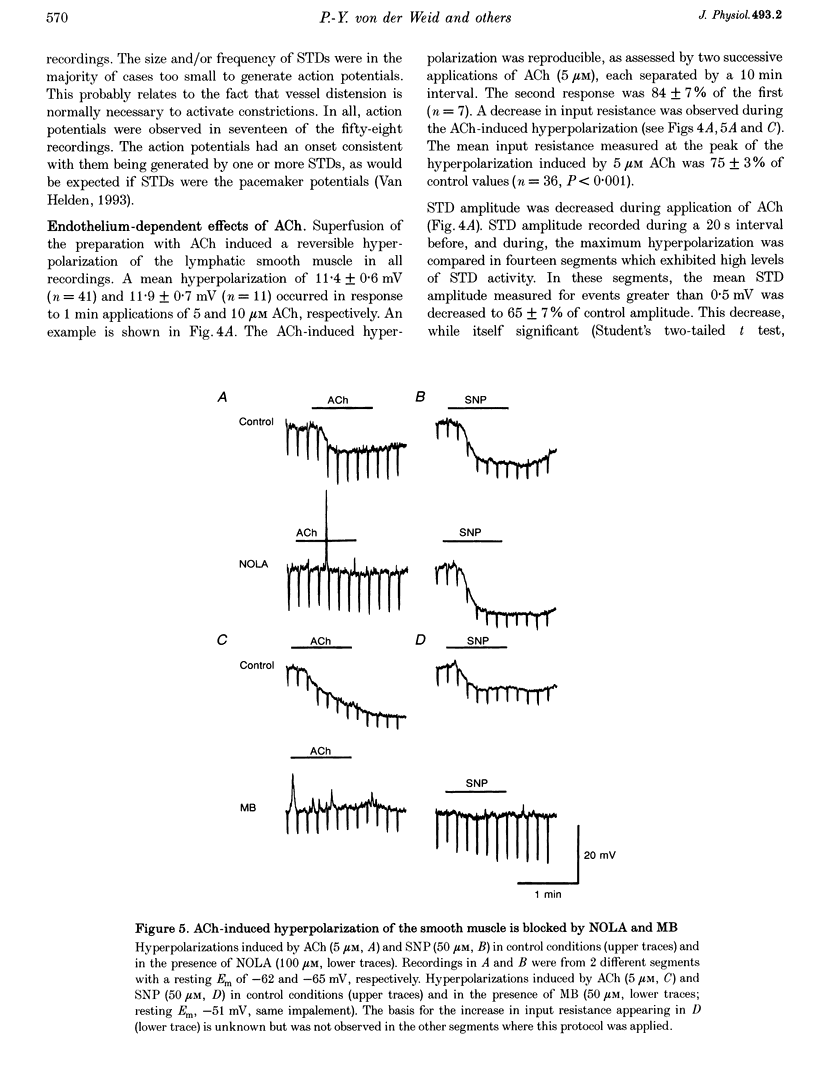
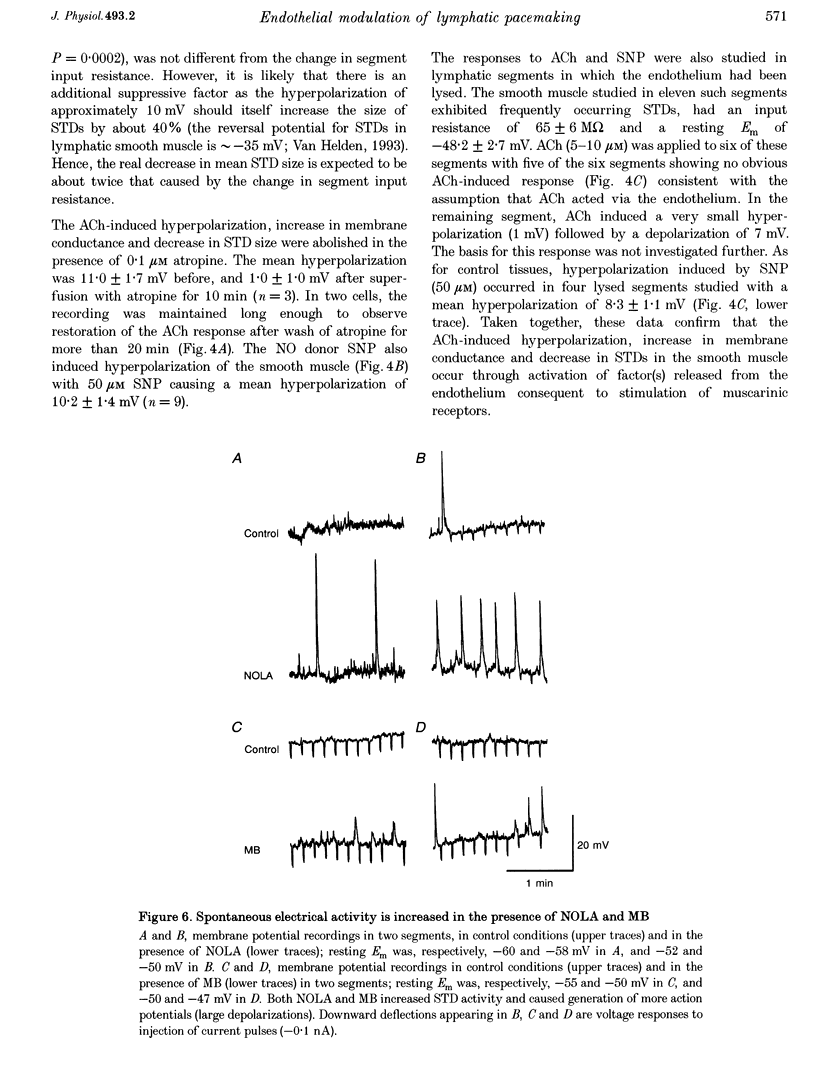
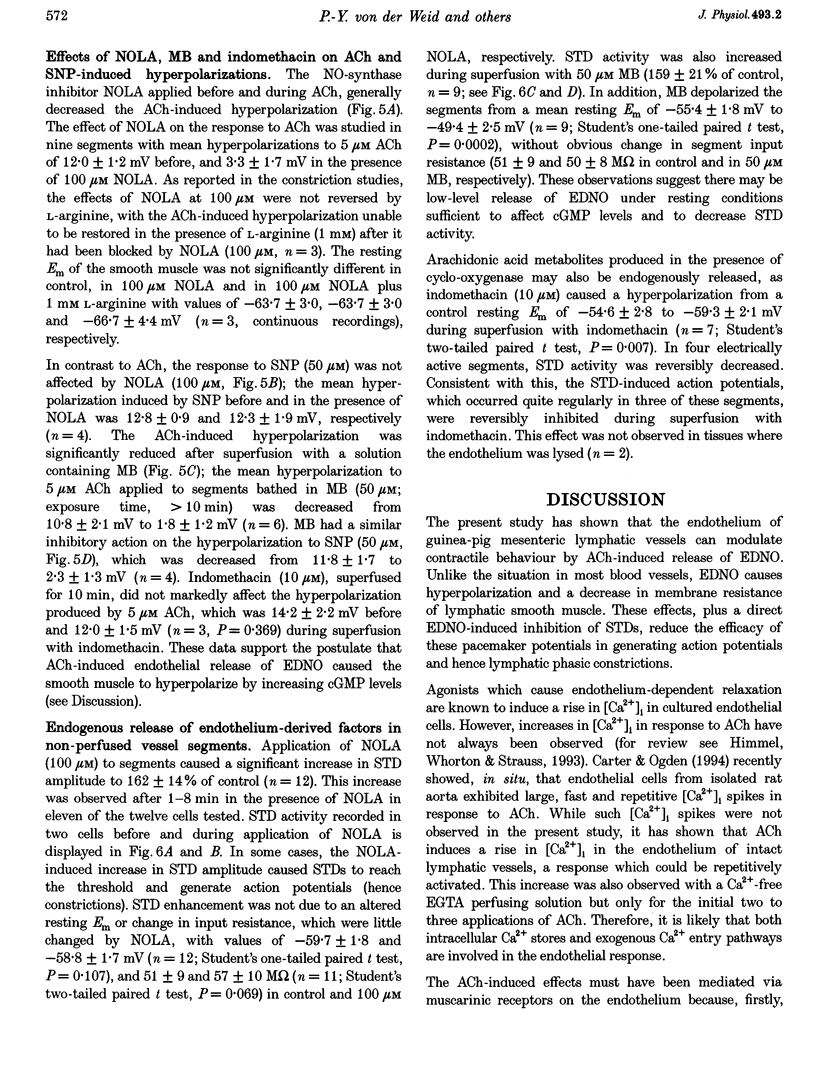
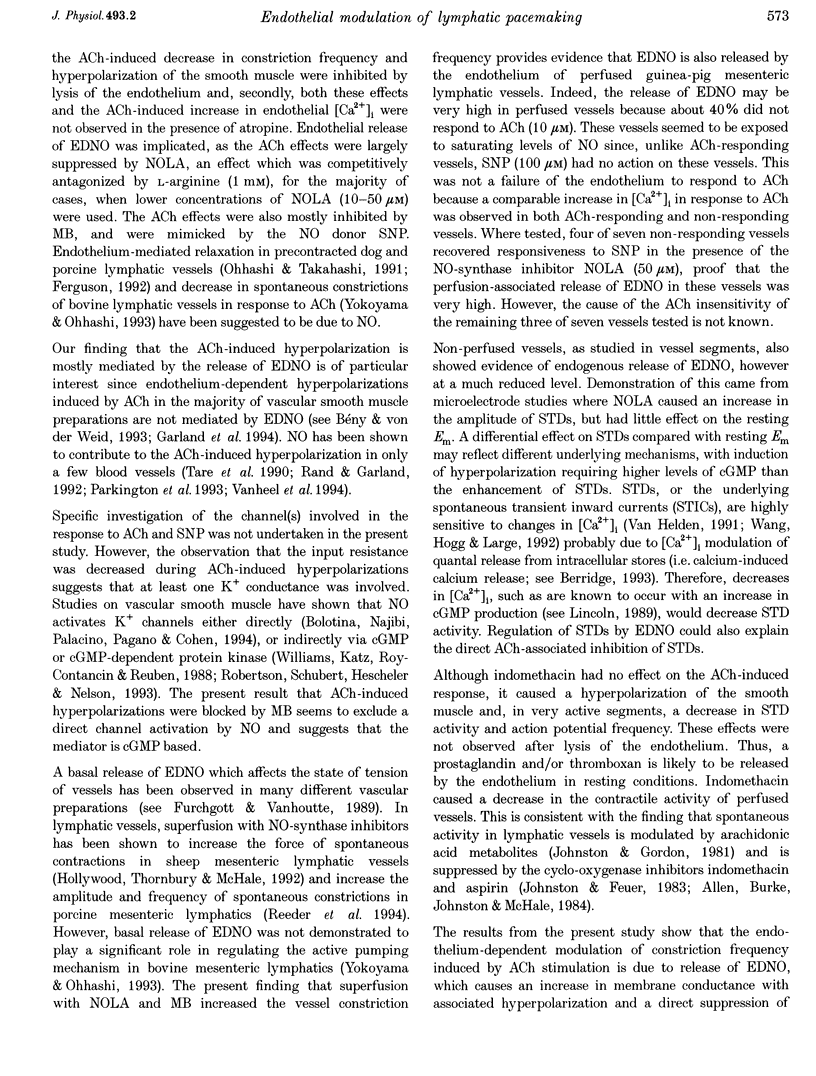
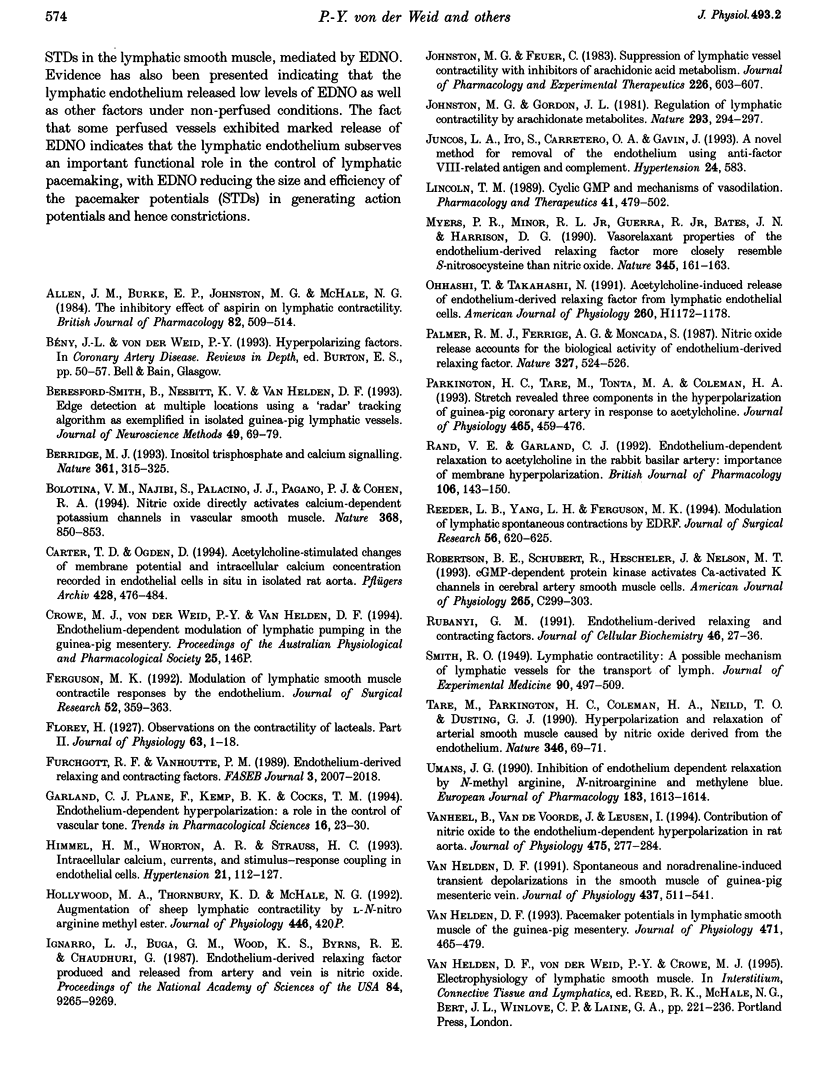
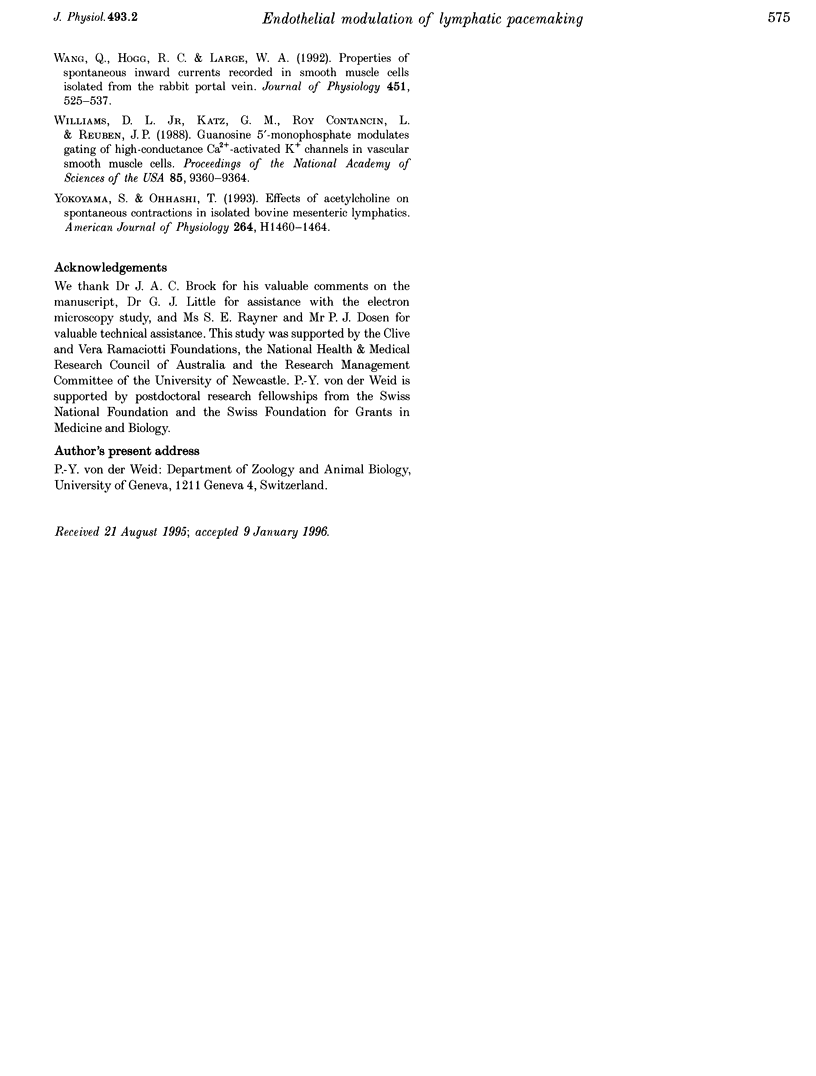
Images in this article
Selected References
These references are in PubMed. This may not be the complete list of references from this article.
- Allen J. M., Burke E. P., Johnston M. G., McHale N. G. The inhibitory effect of aspirin on lymphatic contractility. Br J Pharmacol. 1984 Jun;82(2):509–514. doi: 10.1111/j.1476-5381.1984.tb10787.x. [DOI] [PMC free article] [PubMed] [Google Scholar]
- Beresford-Smith B., Nesbitt K. V., Van Helden D. F. Edge detection at multiple locations using a 'radar' tracking algorithm as exemplified in isolated guinea-pig lymphatic vessels. J Neurosci Methods. 1993 Aug;49(1-2):69–79. doi: 10.1016/0165-0270(93)90110-d. [DOI] [PubMed] [Google Scholar]
- Berridge M. J. Inositol trisphosphate and calcium signalling. Nature. 1993 Jan 28;361(6410):315–325. doi: 10.1038/361315a0. [DOI] [PubMed] [Google Scholar]
- Bolotina V. M., Najibi S., Palacino J. J., Pagano P. J., Cohen R. A. Nitric oxide directly activates calcium-dependent potassium channels in vascular smooth muscle. Nature. 1994 Apr 28;368(6474):850–853. doi: 10.1038/368850a0. [DOI] [PubMed] [Google Scholar]
- Carter T. D., Ogden D. Acetylcholine-stimulated changes of membrane potential and intracellular Ca2+ concentration recorded in endothelial cells in situ in the isolated rat aorta. Pflugers Arch. 1994 Oct;428(5-6):476–484. doi: 10.1007/BF00374568. [DOI] [PubMed] [Google Scholar]
- Ferguson M. K. Modulation of lymphatic smooth muscle contractile responses by the endothelium. J Surg Res. 1992 Apr;52(4):359–363. doi: 10.1016/0022-4804(92)90116-h. [DOI] [PubMed] [Google Scholar]
- Florey H. Observations on the contractility of lacteals: Part II. J Physiol. 1927 Jun 7;63(1):1–18. doi: 10.1113/jphysiol.1927.sp002375. [DOI] [PMC free article] [PubMed] [Google Scholar]
- Furchgott R. F., Vanhoutte P. M. Endothelium-derived relaxing and contracting factors. FASEB J. 1989 Jul;3(9):2007–2018. [PubMed] [Google Scholar]
- Garland C. J., Plane F., Kemp B. K., Cocks T. M. Endothelium-dependent hyperpolarization: a role in the control of vascular tone. Trends Pharmacol Sci. 1995 Jan;16(1):23–30. doi: 10.1016/s0165-6147(00)88969-5. [DOI] [PubMed] [Google Scholar]
- Himmel H. M., Whorton A. R., Strauss H. C. Intracellular calcium, currents, and stimulus-response coupling in endothelial cells. Hypertension. 1993 Jan;21(1):112–127. doi: 10.1161/01.hyp.21.1.112. [DOI] [PubMed] [Google Scholar]
- Ignarro L. J., Buga G. M., Wood K. S., Byrns R. E., Chaudhuri G. Endothelium-derived relaxing factor produced and released from artery and vein is nitric oxide. Proc Natl Acad Sci U S A. 1987 Dec;84(24):9265–9269. doi: 10.1073/pnas.84.24.9265. [DOI] [PMC free article] [PubMed] [Google Scholar]
- Johnston M. G., Feuer C. Suppression of lymphatic vessel contractility with inhibitors of arachidonic acid metabolism. J Pharmacol Exp Ther. 1983 Aug;226(2):603–607. [PubMed] [Google Scholar]
- Johnston M. G., Gordon J. L. Regulation of lymphatic contractility by arachidonate metabolites. Nature. 1981 Sep 24;293(5830):294–297. doi: 10.1038/293294a0. [DOI] [PubMed] [Google Scholar]
- Lincoln T. M. Cyclic GMP and mechanisms of vasodilation. Pharmacol Ther. 1989;41(3):479–502. doi: 10.1016/0163-7258(89)90127-7. [DOI] [PubMed] [Google Scholar]
- Myers P. R., Minor R. L., Jr, Guerra R., Jr, Bates J. N., Harrison D. G. Vasorelaxant properties of the endothelium-derived relaxing factor more closely resemble S-nitrosocysteine than nitric oxide. Nature. 1990 May 10;345(6271):161–163. doi: 10.1038/345161a0. [DOI] [PubMed] [Google Scholar]
- Ohhashi T., Takahashi N. Acetylcholine-induced release of endothelium-derived relaxing factor from lymphatic endothelial cells. Am J Physiol. 1991 Apr;260(4 Pt 2):H1172–H1178. doi: 10.1152/ajpheart.1991.260.4.H1172. [DOI] [PubMed] [Google Scholar]
- Palmer R. M., Ferrige A. G., Moncada S. Nitric oxide release accounts for the biological activity of endothelium-derived relaxing factor. Nature. 1987 Jun 11;327(6122):524–526. doi: 10.1038/327524a0. [DOI] [PubMed] [Google Scholar]
- Parkington H. C., Tare M., Tonta M. A., Coleman H. A. Stretch revealed three components in the hyperpolarization of guinea-pig coronary artery in response to acetylcholine. J Physiol. 1993 Jun;465:459–476. doi: 10.1113/jphysiol.1993.sp019687. [DOI] [PMC free article] [PubMed] [Google Scholar]
- Rand V. E., Garland C. J. Endothelium-dependent relaxation to acetylcholine in the rabbit basilar artery: importance of membrane hyperpolarization. Br J Pharmacol. 1992 May;106(1):143–150. doi: 10.1111/j.1476-5381.1992.tb14307.x. [DOI] [PMC free article] [PubMed] [Google Scholar]
- Reeder L. B., Yang L. H., Ferguson M. K. Modulation of lymphatic spontaneous contractions by EDRF. J Surg Res. 1994 Jun;56(6):620–625. doi: 10.1006/jsre.1994.1098. [DOI] [PubMed] [Google Scholar]
- Robertson B. E., Schubert R., Hescheler J., Nelson M. T. cGMP-dependent protein kinase activates Ca-activated K channels in cerebral artery smooth muscle cells. Am J Physiol. 1993 Jul;265(1 Pt 1):C299–C303. doi: 10.1152/ajpcell.1993.265.1.C299. [DOI] [PubMed] [Google Scholar]
- Rubanyi G. M. Endothelium-derived relaxing and contracting factors. J Cell Biochem. 1991 May;46(1):27–36. doi: 10.1002/jcb.240460106. [DOI] [PubMed] [Google Scholar]
- Tare M., Parkington H. C., Coleman H. A., Neild T. O., Dusting G. J. Hyperpolarization and relaxation of arterial smooth muscle caused by nitric oxide derived from the endothelium. Nature. 1990 Jul 5;346(6279):69–71. doi: 10.1038/346069a0. [DOI] [PubMed] [Google Scholar]
- Van Helden D. F. Pacemaker potentials in lymphatic smooth muscle of the guinea-pig mesentery. J Physiol. 1993 Nov;471:465–479. doi: 10.1113/jphysiol.1993.sp019910. [DOI] [PMC free article] [PubMed] [Google Scholar]
- Van Helden D. F. Spontaneous and noradrenaline-induced transient depolarizations in the smooth muscle of guinea-pig mesenteric vein. J Physiol. 1991 Jun;437:511–541. doi: 10.1113/jphysiol.1991.sp018609. [DOI] [PMC free article] [PubMed] [Google Scholar]
- Vanheel B., Van de Voorde J., Leusen I. Contribution of nitric oxide to the endothelium-dependent hyperpolarization in rat aorta. J Physiol. 1994 Mar 1;475(2):277–284. doi: 10.1113/jphysiol.1994.sp020068. [DOI] [PMC free article] [PubMed] [Google Scholar]
- Wang Q., Hogg R. C., Large W. A. Properties of spontaneous inward currents recorded in smooth muscle cells isolated from the rabbit portal vein. J Physiol. 1992;451:525–537. doi: 10.1113/jphysiol.1992.sp019177. [DOI] [PMC free article] [PubMed] [Google Scholar]
- Williams D. L., Jr, Katz G. M., Roy-Contancin L., Reuben J. P. Guanosine 5'-monophosphate modulates gating of high-conductance Ca2+-activated K+ channels in vascular smooth muscle cells. Proc Natl Acad Sci U S A. 1988 Dec;85(23):9360–9364. doi: 10.1073/pnas.85.23.9360. [DOI] [PMC free article] [PubMed] [Google Scholar]
- Yokoyama S., Ohhashi T. Effects of acetylcholine on spontaneous contractions in isolated bovine mesenteric lymphatics. Am J Physiol. 1993 May;264(5 Pt 2):H1460–H1464. doi: 10.1152/ajpheart.1993.264.5.H1460. [DOI] [PubMed] [Google Scholar]