Abstract
1. Mechanical properties of the surface membrane of skeletal muscle were determined on sarcolemmal vesicles (mean diameter, 71 microns) shed by rabbit psoas muscle swelling in 140 mM KC1 containing collagenase. 2. Vesicles were stressed by partial aspiration into parallel bore pipettes. The isotropic membrane tension so created caused an increase in membrane area which expresses itself in an elongation of the vesicle projection into the pipette. 3. For individual vesicles, a linear relationship between membrane tension and membrane area increase was found up to the point when the vesicle burst, i.e. sarcolemmal vesicles behaved as perfectly elastic structures. 4. The maximum tension sarcolemmal vesicles could sustain before bursting was 12.4 +/- 0.2 mN m-1 (median +/- 95% confidence interval), and the corresponding fractional increase in membrane area was 0.026 +/- 0.005 (median +/- 95% confidence interval). The elastic modulus of area expansion was 490 +/- 88 mN m-1 (mean +/- S.D.). 5. In conformity with cited comparable work on red blood cells and artificial lipid vesicles, the strength and area elasticity of the skeletal muscle membrane are considered properties of the fluid lipid matrix of the membrane and of the degree to which the bilayer is perturbed by lipid-protein interaction.
Full text
PDF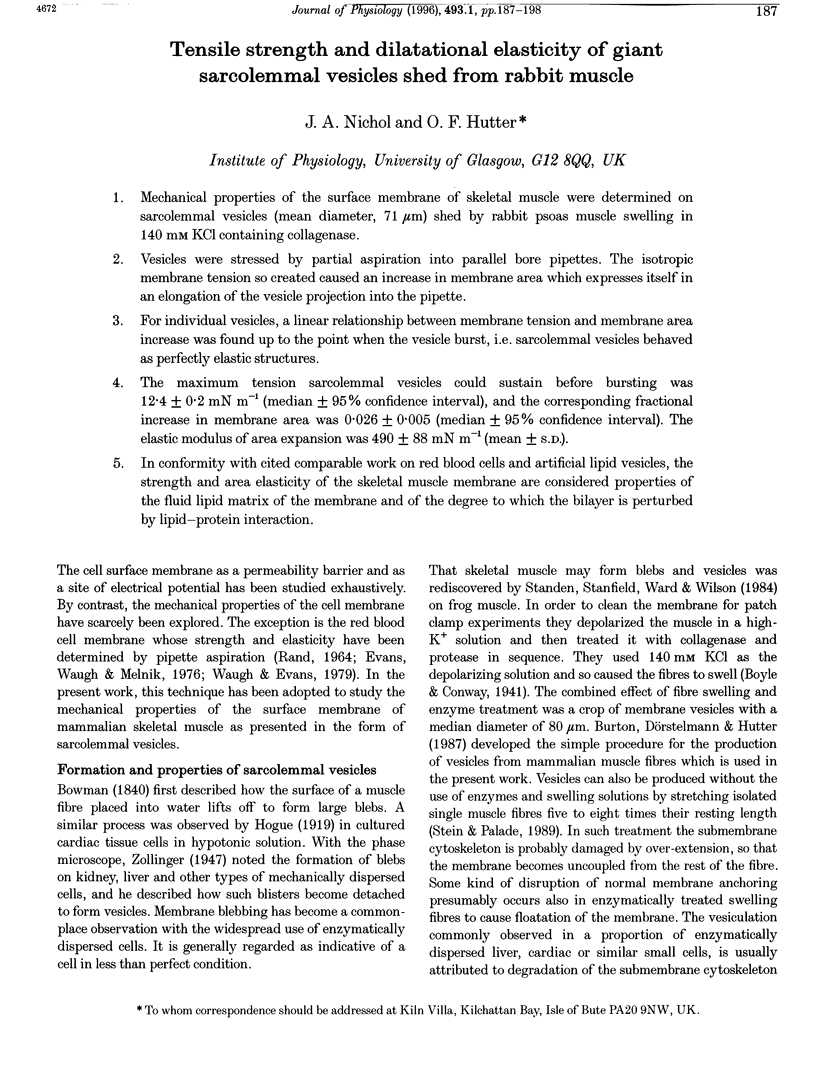
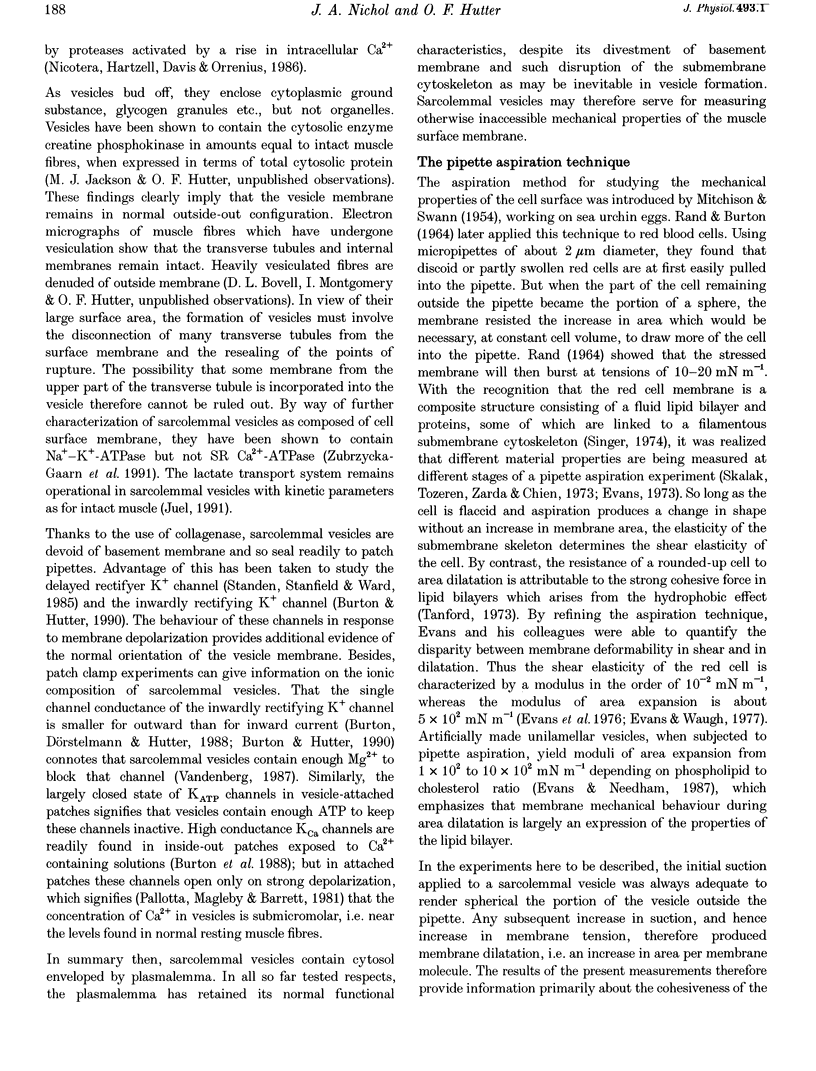
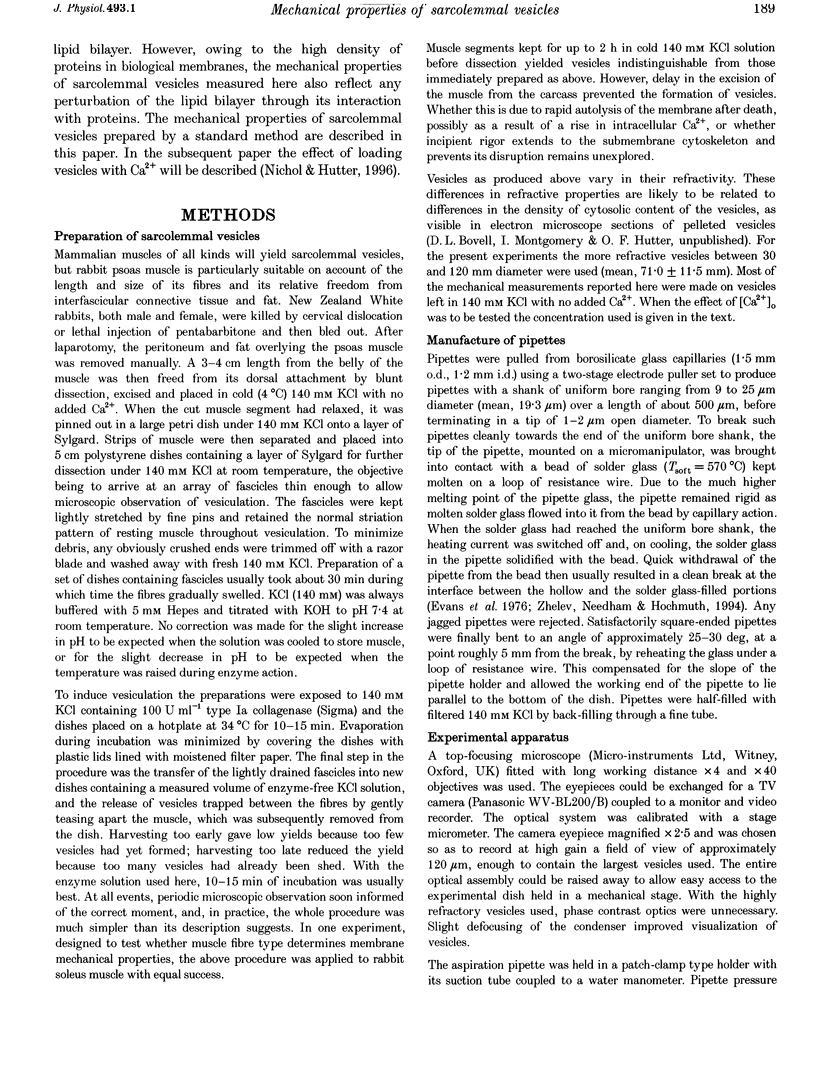
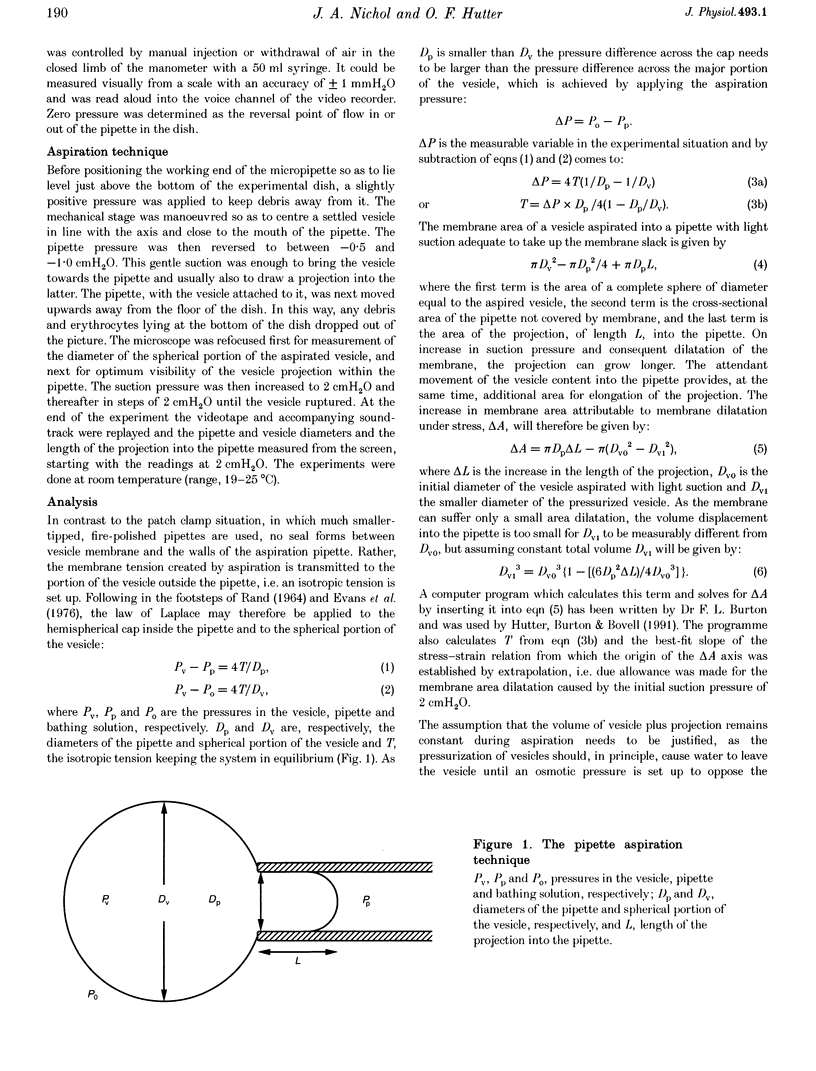
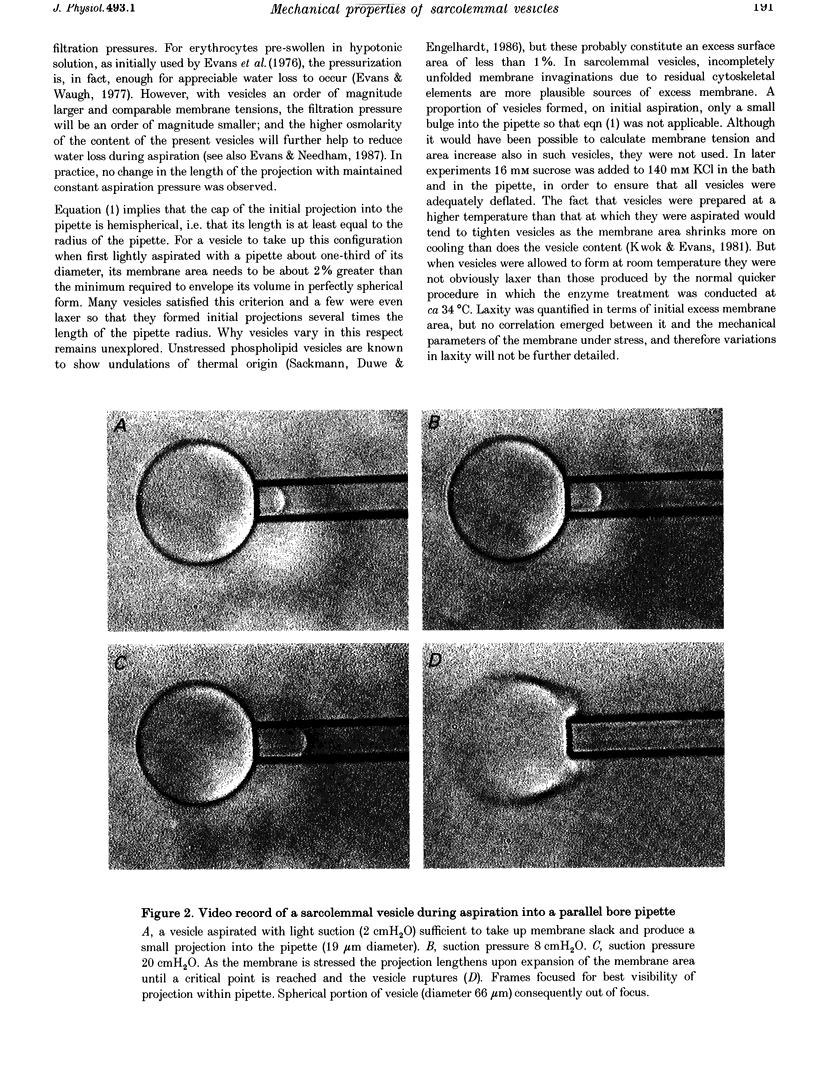
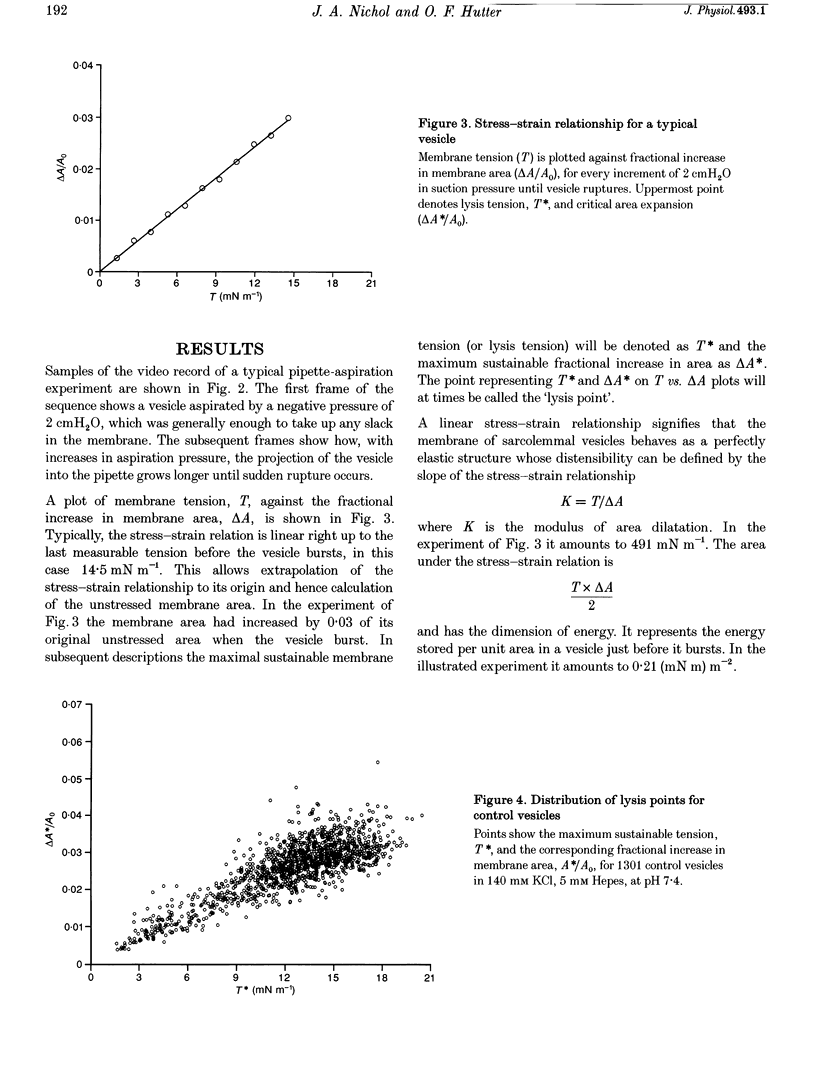
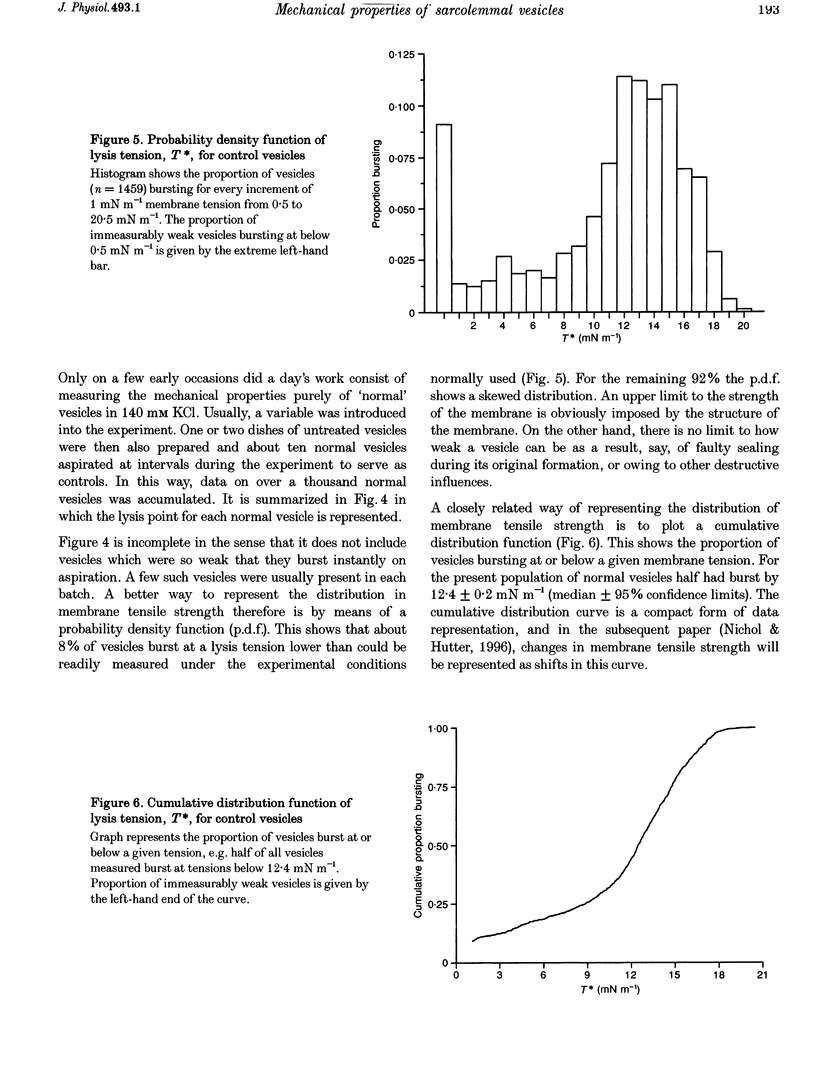
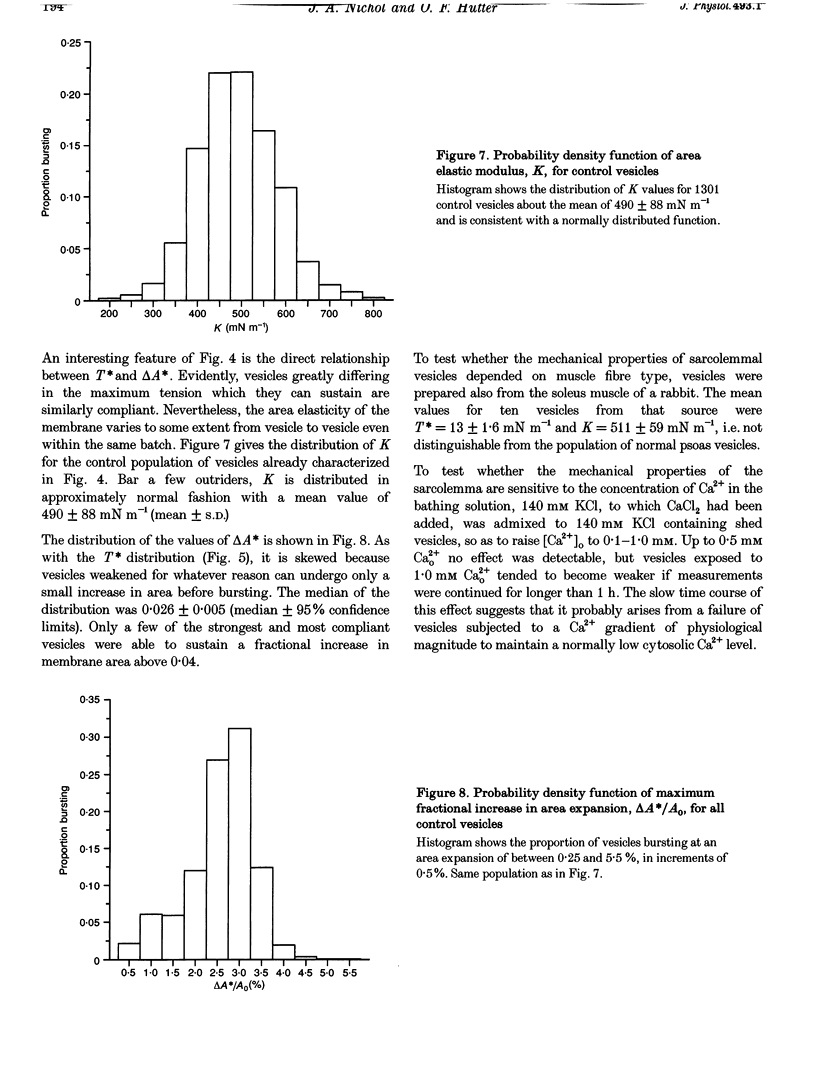
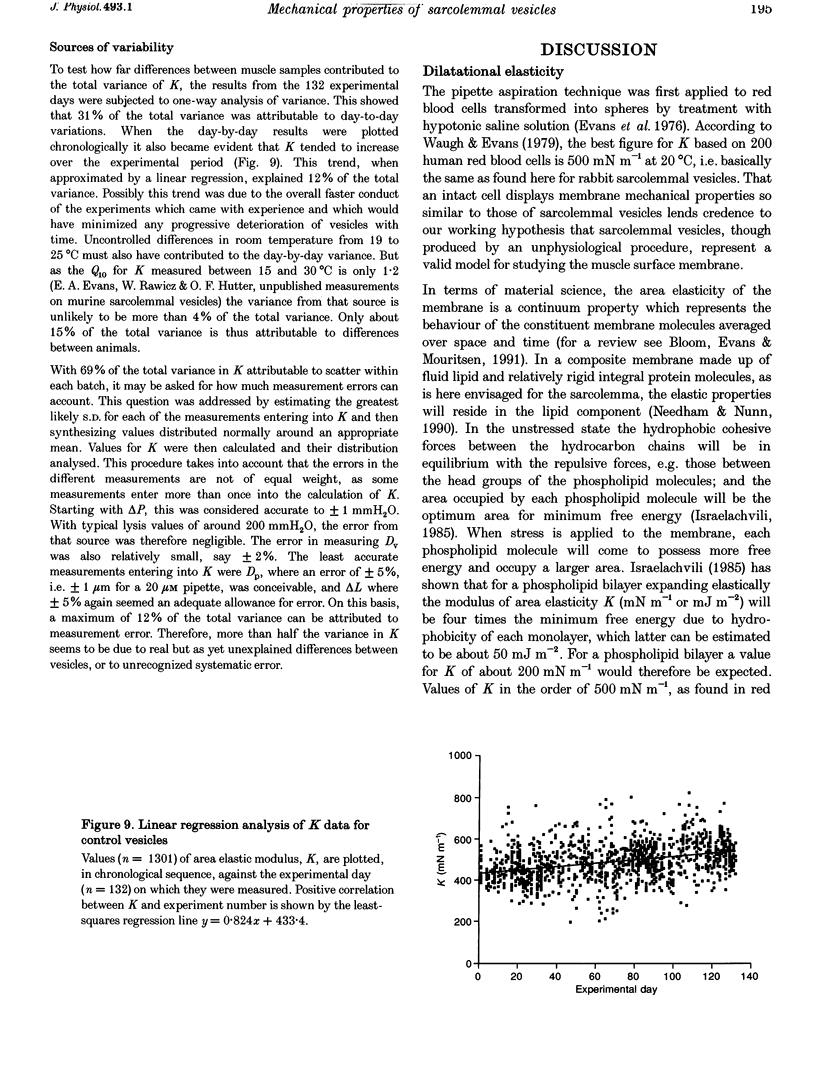
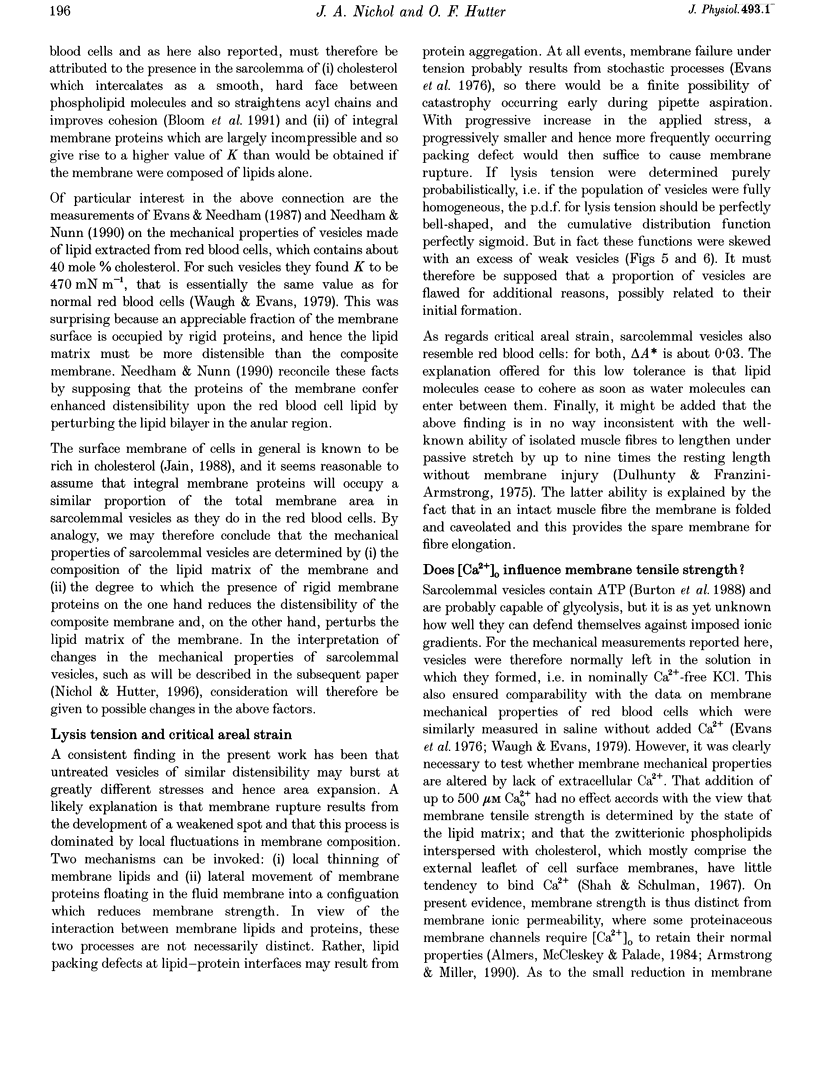
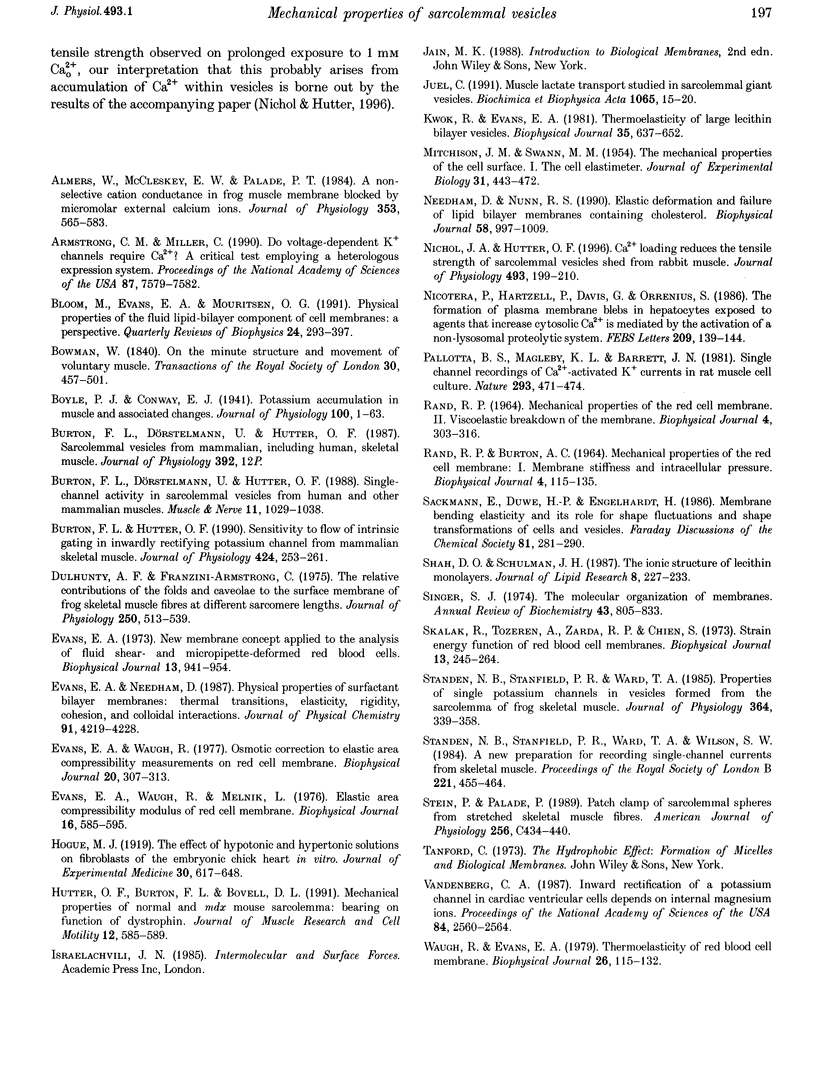
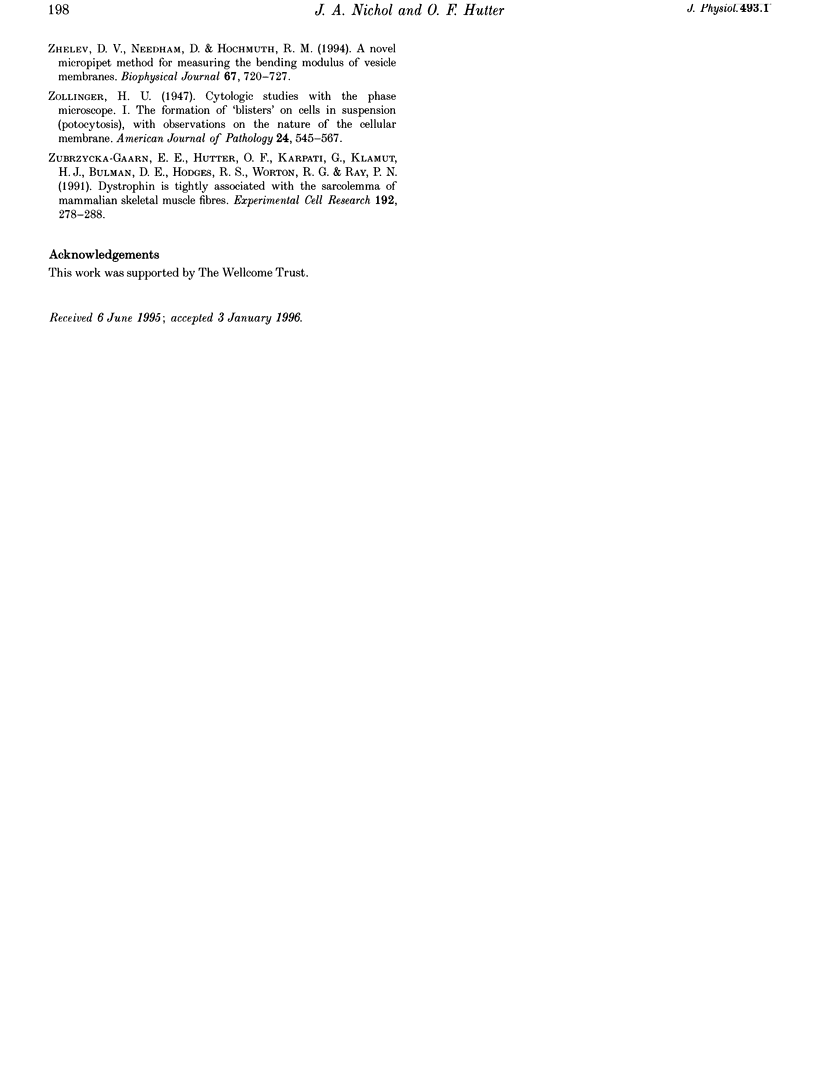
Images in this article
Selected References
These references are in PubMed. This may not be the complete list of references from this article.
- Almers W., McCleskey E. W., Palade P. T. A non-selective cation conductance in frog muscle membrane blocked by micromolar external calcium ions. J Physiol. 1984 Aug;353:565–583. doi: 10.1113/jphysiol.1984.sp015351. [DOI] [PMC free article] [PubMed] [Google Scholar]
- Armstrong C. M., Miller C. Do voltage-dependent K+ channels require Ca2+? A critical test employing a heterologous expression system. Proc Natl Acad Sci U S A. 1990 Oct;87(19):7579–7582. doi: 10.1073/pnas.87.19.7579. [DOI] [PMC free article] [PubMed] [Google Scholar]
- Bloom M., Evans E., Mouritsen O. G. Physical properties of the fluid lipid-bilayer component of cell membranes: a perspective. Q Rev Biophys. 1991 Aug;24(3):293–397. doi: 10.1017/s0033583500003735. [DOI] [PubMed] [Google Scholar]
- Boyle P. J., Conway E. J. Potassium accumulation in muscle and associated changes. J Physiol. 1941 Aug 11;100(1):1–63. doi: 10.1113/jphysiol.1941.sp003922. [DOI] [PMC free article] [PubMed] [Google Scholar]
- Burton F. L., Hutter O. F. Sensitivity to flow of intrinsic gating in inwardly rectifying potassium channel from mammalian skeletal muscle. J Physiol. 1990 May;424:253–261. doi: 10.1113/jphysiol.1990.sp018065. [DOI] [PMC free article] [PubMed] [Google Scholar]
- Burton F., Dörstelmann U., Hutter O. F. Single-channel activity in sarcolemmal vesicles from human and other mammalian muscles. Muscle Nerve. 1988 Oct;11(10):1029–1038. doi: 10.1002/mus.880111004. [DOI] [PubMed] [Google Scholar]
- Dulhunty A. F., Franzini-Armstrong C. The relative contributions of the folds and caveolae to the surface membrane of frog skeletal muscle fibres at different sarcomere lengths. J Physiol. 1975 Sep;250(3):513–539. doi: 10.1113/jphysiol.1975.sp011068. [DOI] [PMC free article] [PubMed] [Google Scholar]
- Evans E. A. New membrane concept applied to the analysis of fluid shear- and micropipette-deformed red blood cells. Biophys J. 1973 Sep;13(9):941–954. doi: 10.1016/S0006-3495(73)86036-9. [DOI] [PMC free article] [PubMed] [Google Scholar]
- Evans E. A., Waugh R., Melnik L. Elastic area compressibility modulus of red cell membrane. Biophys J. 1976 Jun;16(6):585–595. doi: 10.1016/S0006-3495(76)85713-X. [DOI] [PMC free article] [PubMed] [Google Scholar]
- Evans E. A., Waugh R. Osmotic correction to elastic area compressibility measurements on red cell membrane. Biophys J. 1977 Dec;20(3):307–313. doi: 10.1016/S0006-3495(77)85551-3. [DOI] [PMC free article] [PubMed] [Google Scholar]
- Hutter O. F., Burton F. L., Bovell D. L. Mechanical properties of normal and mdx mouse sarcolemma: bearing on function of dystrophin. J Muscle Res Cell Motil. 1991 Dec;12(6):585–589. doi: 10.1007/BF01738447. [DOI] [PubMed] [Google Scholar]
- Juel C. Muscle lactate transport studied in sarcolemmal giant vesicles. Biochim Biophys Acta. 1991 May 31;1065(1):15–20. doi: 10.1016/0005-2736(91)90004-r. [DOI] [PubMed] [Google Scholar]
- Kwok R., Evans E. Thermoelasticity of large lecithin bilayer vesicles. Biophys J. 1981 Sep;35(3):637–652. doi: 10.1016/S0006-3495(81)84817-5. [DOI] [PMC free article] [PubMed] [Google Scholar]
- Needham D., Nunn R. S. Elastic deformation and failure of lipid bilayer membranes containing cholesterol. Biophys J. 1990 Oct;58(4):997–1009. doi: 10.1016/S0006-3495(90)82444-9. [DOI] [PMC free article] [PubMed] [Google Scholar]
- Nichol J. A., Hutter O. F. Ca2+ loading reduces the tensile strength of sarcolemmal vesicles shed from rabbit muscle. J Physiol. 1996 May 15;493(Pt 1):199–209. doi: 10.1113/jphysiol.1996.sp021375. [DOI] [PMC free article] [PubMed] [Google Scholar]
- Nicotera P., Hartzell P., Davis G., Orrenius S. The formation of plasma membrane blebs in hepatocytes exposed to agents that increase cytosolic Ca2+ is mediated by the activation of a non-lysosomal proteolytic system. FEBS Lett. 1986 Dec 1;209(1):139–144. doi: 10.1016/0014-5793(86)81099-7. [DOI] [PubMed] [Google Scholar]
- Pallotta B. S., Magleby K. L., Barrett J. N. Single channel recordings of Ca2+-activated K+ currents in rat muscle cell culture. Nature. 1981 Oct 8;293(5832):471–474. doi: 10.1038/293471a0. [DOI] [PubMed] [Google Scholar]
- RAND R. P., BURTON A. C. MECHANICAL PROPERTIES OF THE RED CELL MEMBRANE. I. MEMBRANE STIFFNESS AND INTRACELLULAR PRESSURE. Biophys J. 1964 Mar;4:115–135. doi: 10.1016/s0006-3495(64)86773-4. [DOI] [PMC free article] [PubMed] [Google Scholar]
- RAND R. P. MECHANICAL PROPERTIES OF THE RED CELL MEMBRANE. II. VISCOELASTIC BREAKDOWN OF THE MEMBRANE. Biophys J. 1964 Jul;4:303–316. doi: 10.1016/s0006-3495(64)86784-9. [DOI] [PMC free article] [PubMed] [Google Scholar]
- Sackmann E., Duwe H. P., Engelhardt H. Membrane bending elasticity and its role for shape fluctuations and shape transformations of cells and vesicles. Faraday Discuss Chem Soc. 1986;(81):281–290. doi: 10.1039/dc9868100281. [DOI] [PubMed] [Google Scholar]
- Shah D. O., Schulman J. H. The ionic structure of lecithin monolayers. J Lipid Res. 1967 May;8(3):227–233. [PubMed] [Google Scholar]
- Singer S. J. The molecular organization of membranes. Annu Rev Biochem. 1974;43(0):805–833. doi: 10.1146/annurev.bi.43.070174.004105. [DOI] [PubMed] [Google Scholar]
- Skalak R., Tozeren A., Zarda R. P., Chien S. Strain energy function of red blood cell membranes. Biophys J. 1973 Mar;13(3):245–264. doi: 10.1016/S0006-3495(73)85983-1. [DOI] [PMC free article] [PubMed] [Google Scholar]
- Standen N. B., Stanfield P. R., Ward T. A. Properties of single potassium channels in vesicles formed from the sarcolemma of frog skeletal muscle. J Physiol. 1985 Jul;364:339–358. doi: 10.1113/jphysiol.1985.sp015749. [DOI] [PMC free article] [PubMed] [Google Scholar]
- Standen N. B., Stanfield P. R., Ward T. A., Wilson S. W. A new preparation for recording single-channel currents from skeletal muscle. Proc R Soc Lond B Biol Sci. 1984 Jun 22;221(1225):455–464. doi: 10.1098/rspb.1984.0044. [DOI] [PubMed] [Google Scholar]
- Stein P., Palade P. Patch clamp of sarcolemmal spheres from stretched skeletal muscle fibers. Am J Physiol. 1989 Feb;256(2 Pt 1):C434–C440. doi: 10.1152/ajpcell.1989.256.2.C434. [DOI] [PubMed] [Google Scholar]
- Vandenberg C. A. Inward rectification of a potassium channel in cardiac ventricular cells depends on internal magnesium ions. Proc Natl Acad Sci U S A. 1987 Apr;84(8):2560–2564. doi: 10.1073/pnas.84.8.2560. [DOI] [PMC free article] [PubMed] [Google Scholar]
- Waugh R., Evans E. A. Thermoelasticity of red blood cell membrane. Biophys J. 1979 Apr;26(1):115–131. doi: 10.1016/S0006-3495(79)85239-X. [DOI] [PMC free article] [PubMed] [Google Scholar]
- ZOLLINGER H. U. Cytologic studies with the phase Microscope; the formation of blisters on cells in suspension, photocytosis, with observations on the nature of the cellular membrane. Am J Pathol. 1948 May;24(3):545–567. [PMC free article] [PubMed] [Google Scholar]
- Zhelev D. V., Needham D., Hochmuth R. M. A novel micropipet method for measuring the bending modulus of vesicle membranes. Biophys J. 1994 Aug;67(2):720–727. doi: 10.1016/S0006-3495(94)80530-2. [DOI] [PMC free article] [PubMed] [Google Scholar]
- Zubrzycka-Gaarn E. E., Hutter O. F., Karpati G., Klamut H. J., Bulman D. E., Hodges R. S., Worton R. G., Ray P. N. Dystrophin is tightly associated with the sarcolemma of mammalian skeletal muscle fibers. Exp Cell Res. 1991 Jan;192(1):278–288. doi: 10.1016/0014-4827(91)90187-y. [DOI] [PubMed] [Google Scholar]