Abstract
1. Time-resolved cell membrane capacitance (Cm) measurements were used in combination with fura-2 microfluorometry under whole-cell patch clamp recording to investigate the kinetics and Ca2+ sensitivity of exocytotic granule fusion evoked by depolarizing stimuli at single, isolated nerve endings of the rat neurohypophysis. 2. Single step depolarizations or trains of depolarizing pulses evoked voltage-dependent, inward Ca2+ currents (ICa) and induced both Ca(2+)-dependent and Ca(2+)-independent changes in Cm. Three distinct Cm responses were observed and were differentiated by their kinetics and Ca2+ sensitivity: a non-exocytotic transient (delta Cm,t) and an exocytotic Cm 'jump' (delta Cm,J) and a slower, often latent, exocytotic Cm rise (delta Cm,s) that outlasted the depolarizing pulse stimulus. 3. The delta Cm,t was characterized by a rapid, transient component observed in 70% of nerve endings and a voltage-activation relationship that preceded that of the ICa. The amplitude and kinetics of the delta Cm,t were unaffected by ICa block by Cd2+, Ca2+ load reduction, or alterations in intracellular Ca2+ buffering. 4. In contrast to the delta Cm,t, both the delta Cm,J and delta Cm,s were Ca2+ dependent as evidenced by their sensitivity to Cd2+ block of ICa, intraterminal application of 10 mM BAPTA and reduced [Ca2+]o or replacement of Ca2+ as the charge carrier with Ba2+. 5. The delta Cm,J was proportional to depolarization-evoked Ca2+ influx with initial exocytotic rate of approximately 350 granule fusions s-1. The amplitude of the delta Cm,J rose exponentially (tau = 40 ms) and approached an asymptote (15.5 fF) with longer duration depolarizations indicating the fusion from and depletion of an immediately releasable pool (IRP) estimated at nineteen docked and primed secretory granules. 6. The delta Cm,s was induced by the application of repetitive long duration pulses and defined as the exocytosis of secretory granules from a readily releasable granule pool (RRP). The delta Cm,s response occurred only after exceeding a [Ca2+]i threshold value and rose thereafter in proportion to Ca2+ influx with a mean initial secretory rate of 36 granule fusions s-1. The mean latency for delta Cm,s activation was 850 ms following the initiation of the step depolarizations. The delta Cm,s response magnitude, reflecting the size of the RRP, was dependent on the resting [Ca2+]i and the nerve ending size, and was depletable using repetitive depolarizations of long duration. 7. Recruitment into and release from the RRP and IRP were differentially sensitive to changes in intraterminal Ca2+ buffering conditions. For example, introduction of 5 mM EGTA was shown to have no effect on the evoked IRP but significantly reduced the RRP. In comparison, diminishment of the endogenous Ca2+ buffering capacity of nerve endings by treatment with the mitochondrial Ca2+ uniporter blocker Ruthenium Red (10 microM) potentiated the RRP size but had no significant effect on the IRP size. 8. The present study indicates that the Ca(2+)-dependent recruitment of and release from functionally distinct pools of peptide-containing secretory granules in combination with the [Ca2+]i regulatory properties of neurohypophysial nerve endings may explain both the depletion of peptide release under prolonged stimulus and the potentiation of peptide release observed to occur during recurrent phasic action potential activity in this system.
Full text
PDF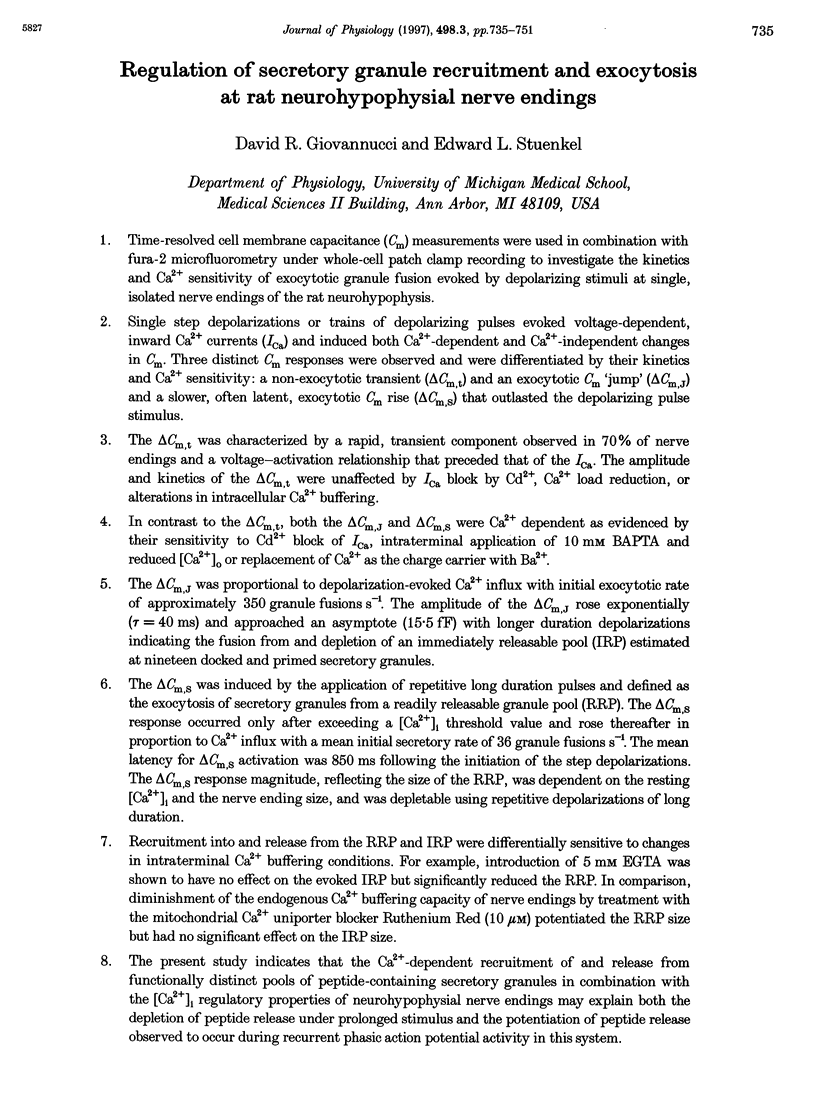
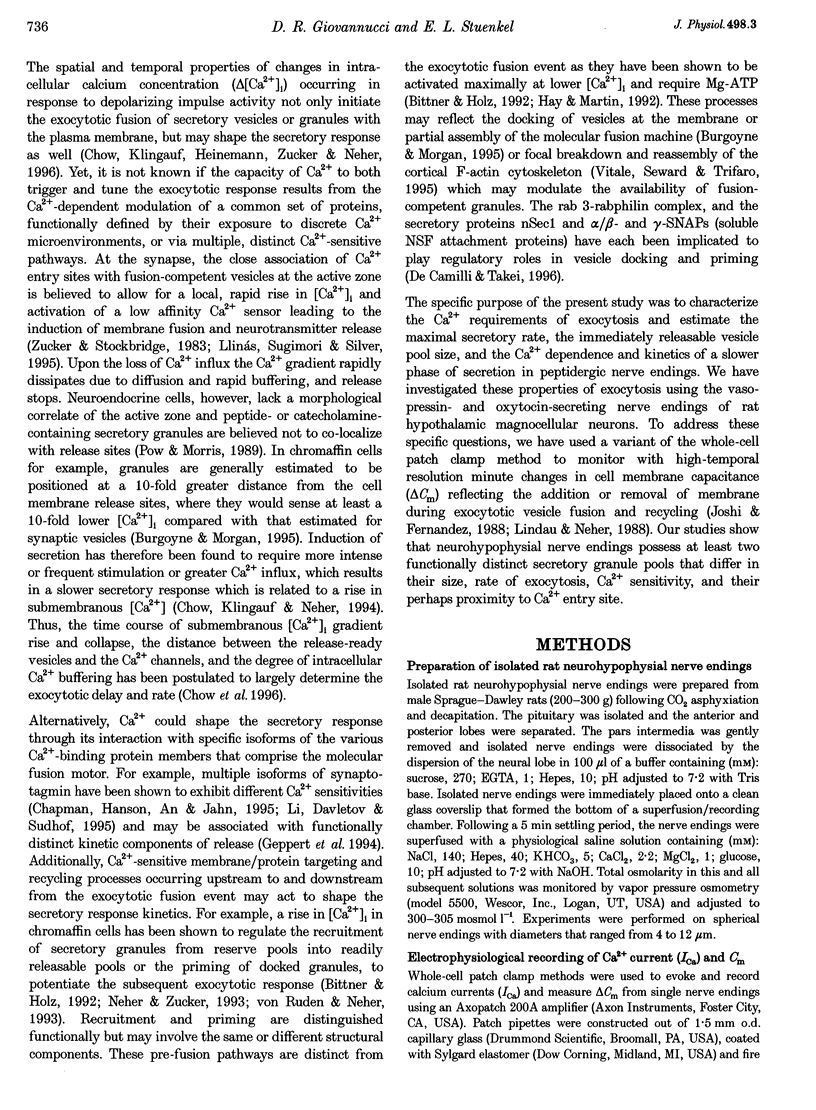
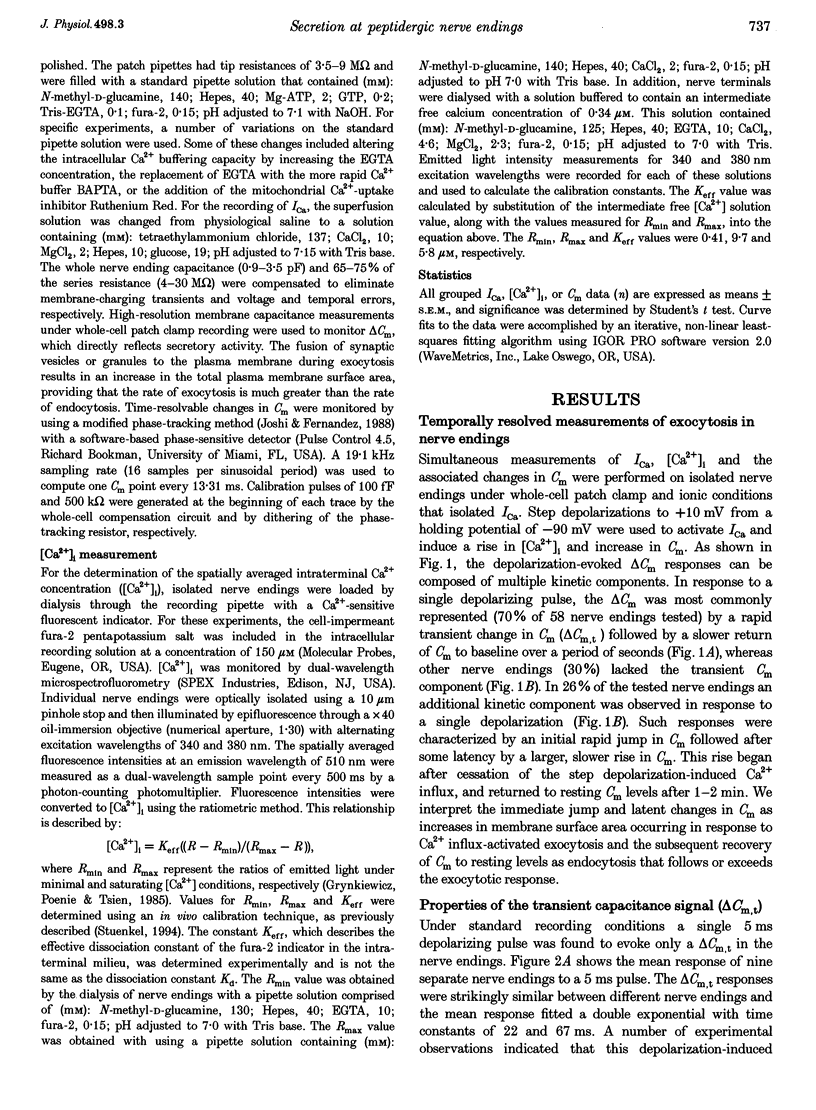
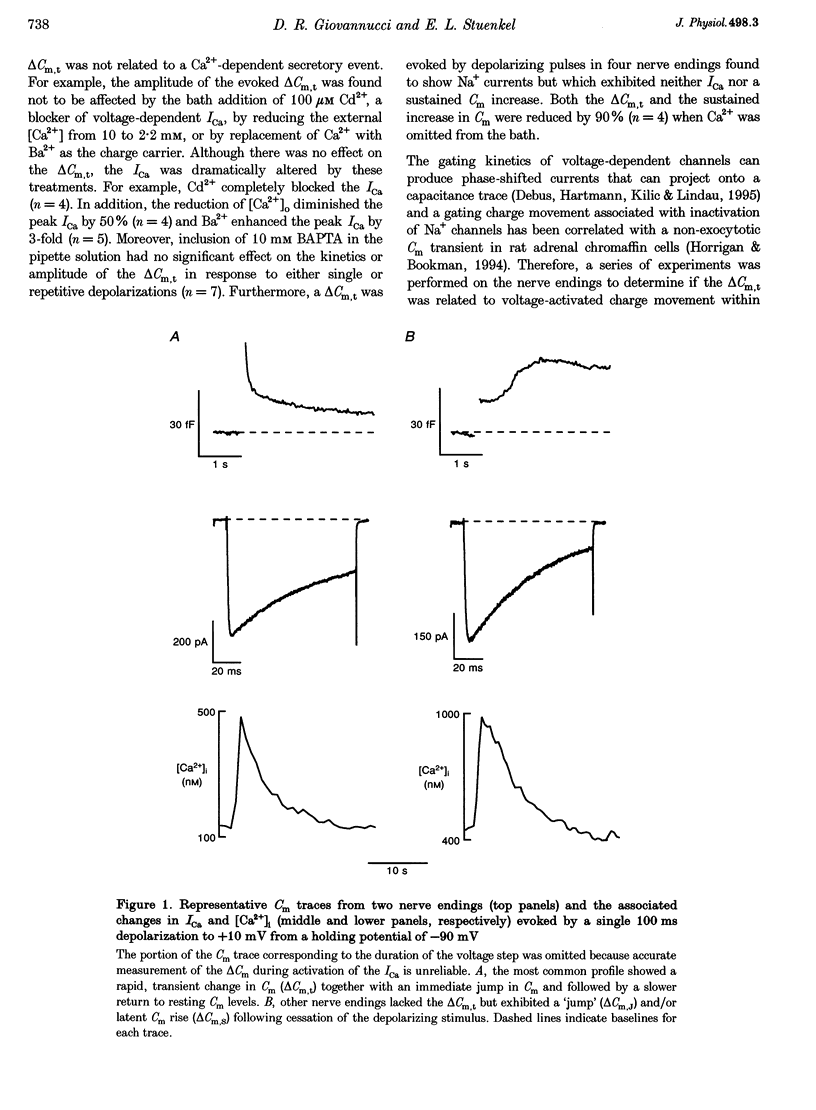
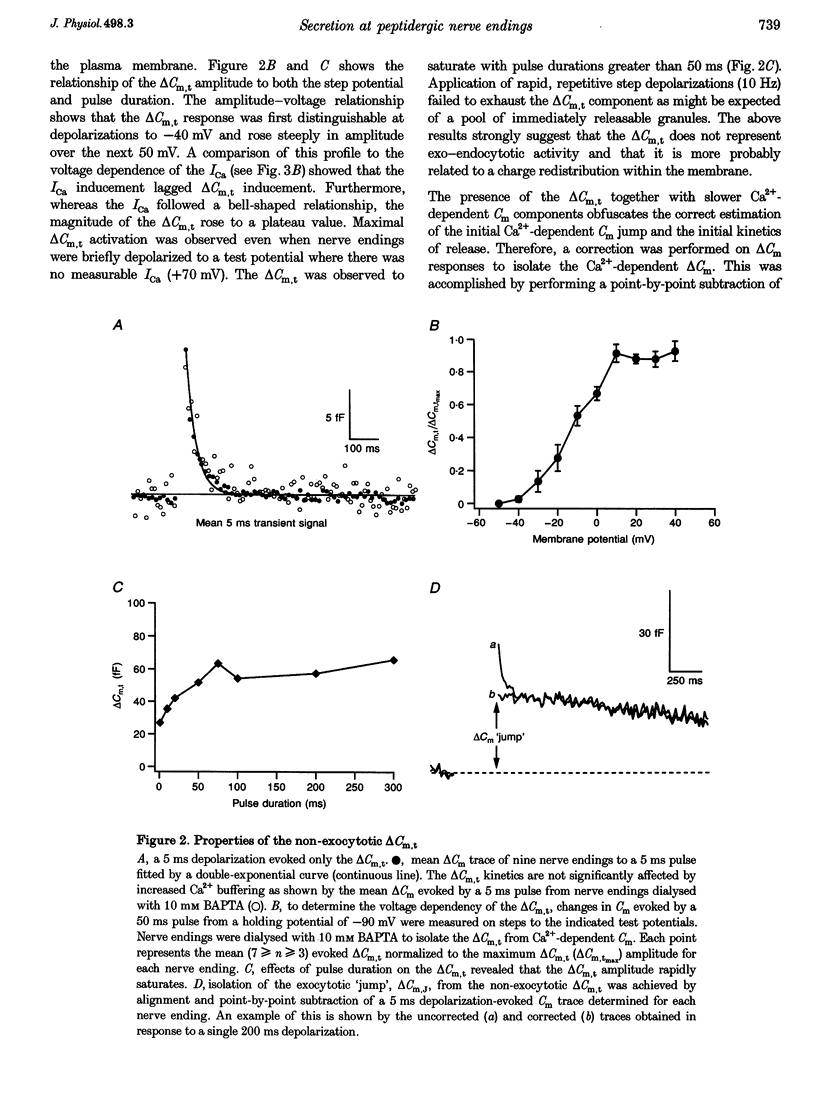
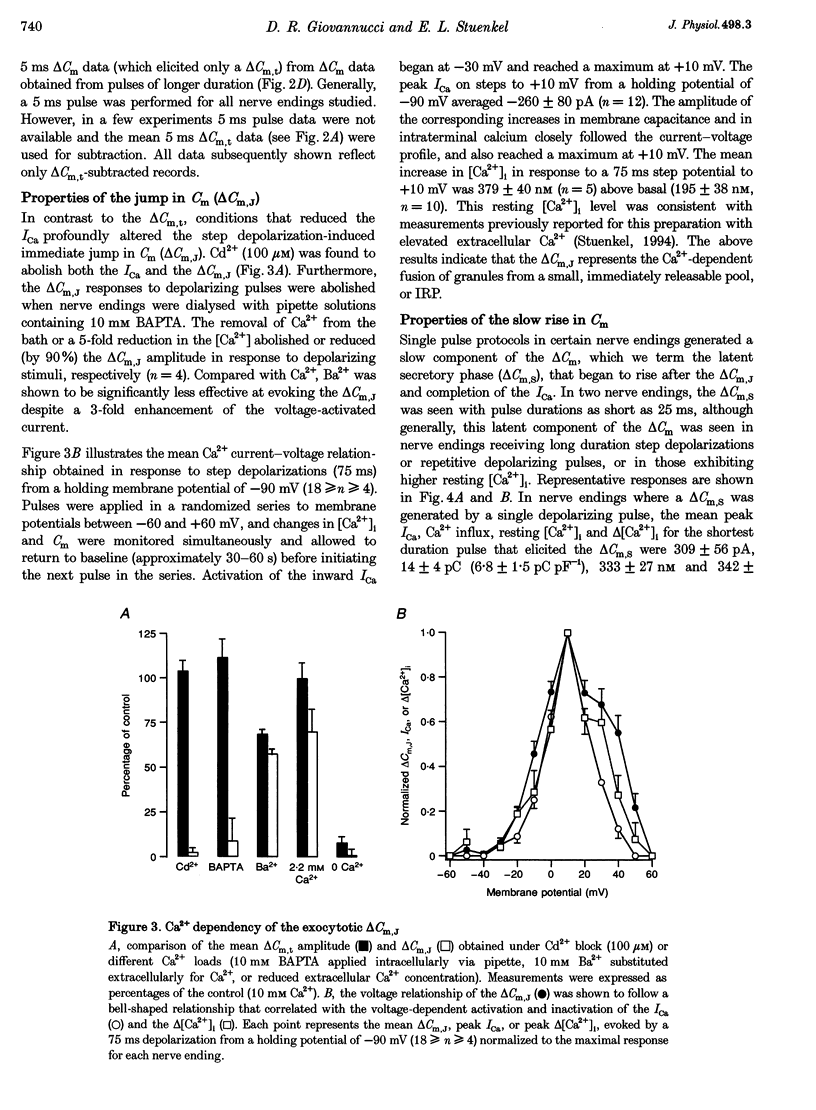
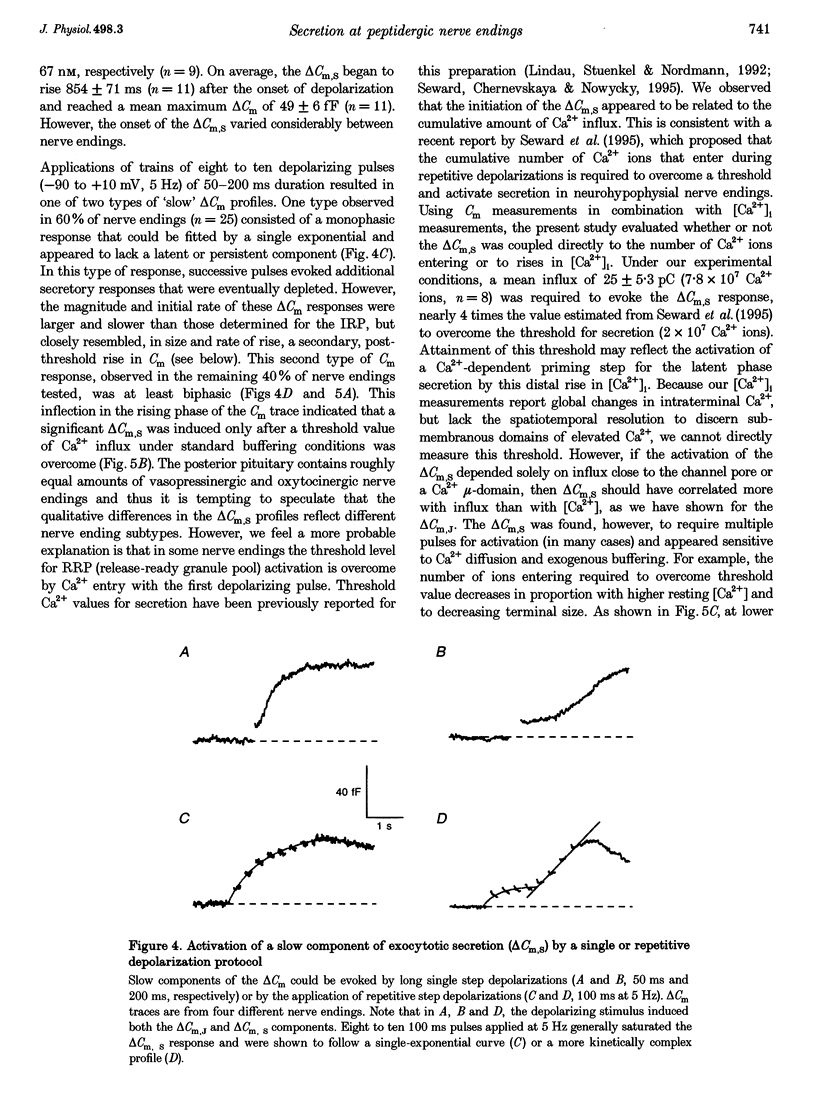
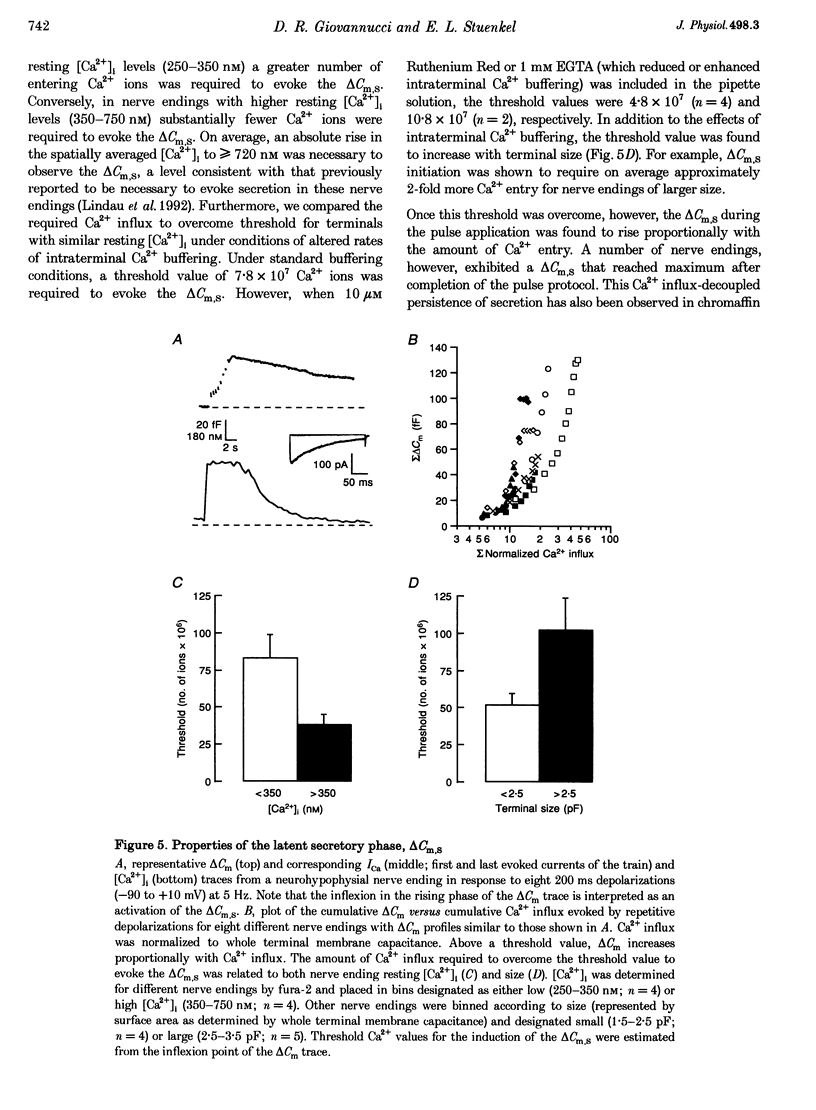
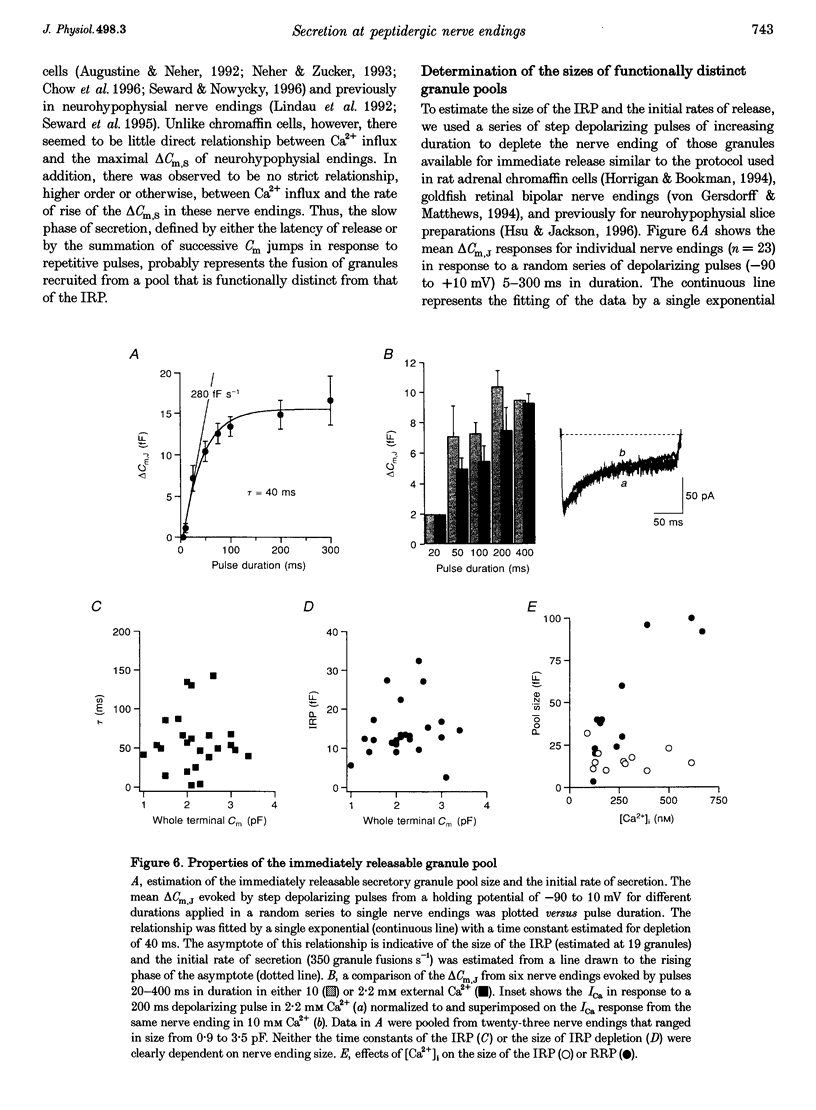
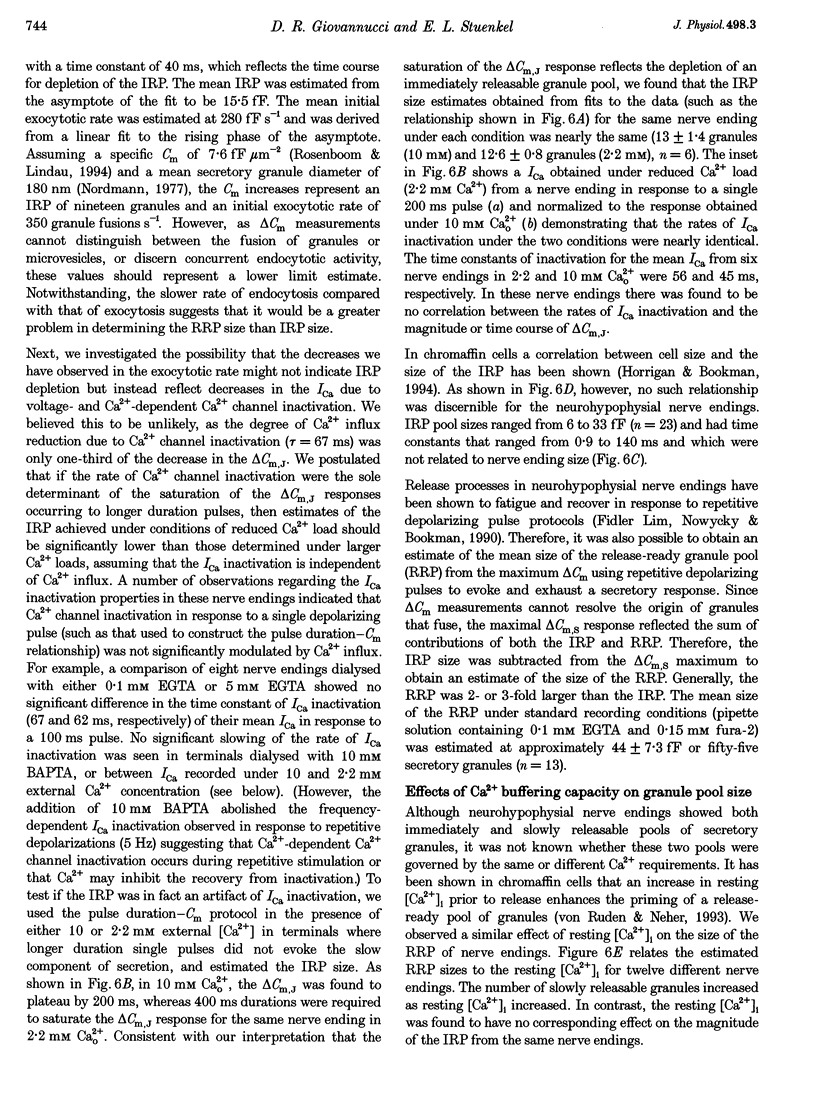
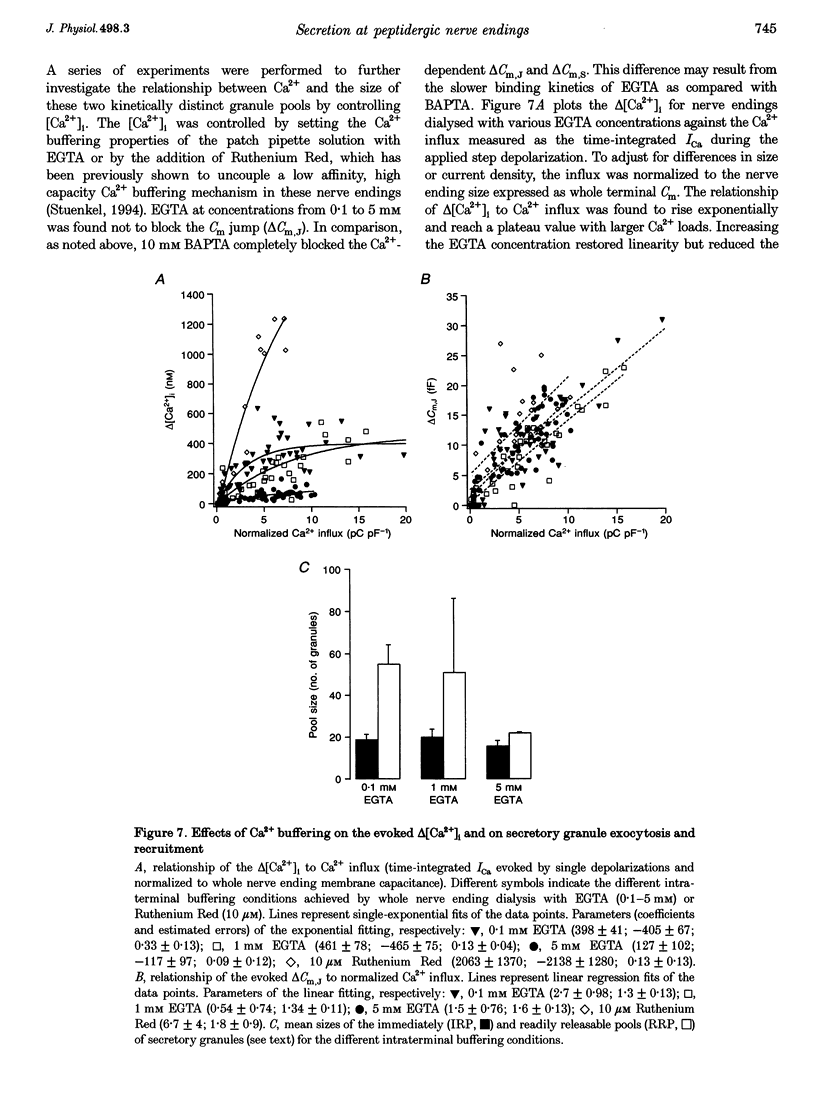
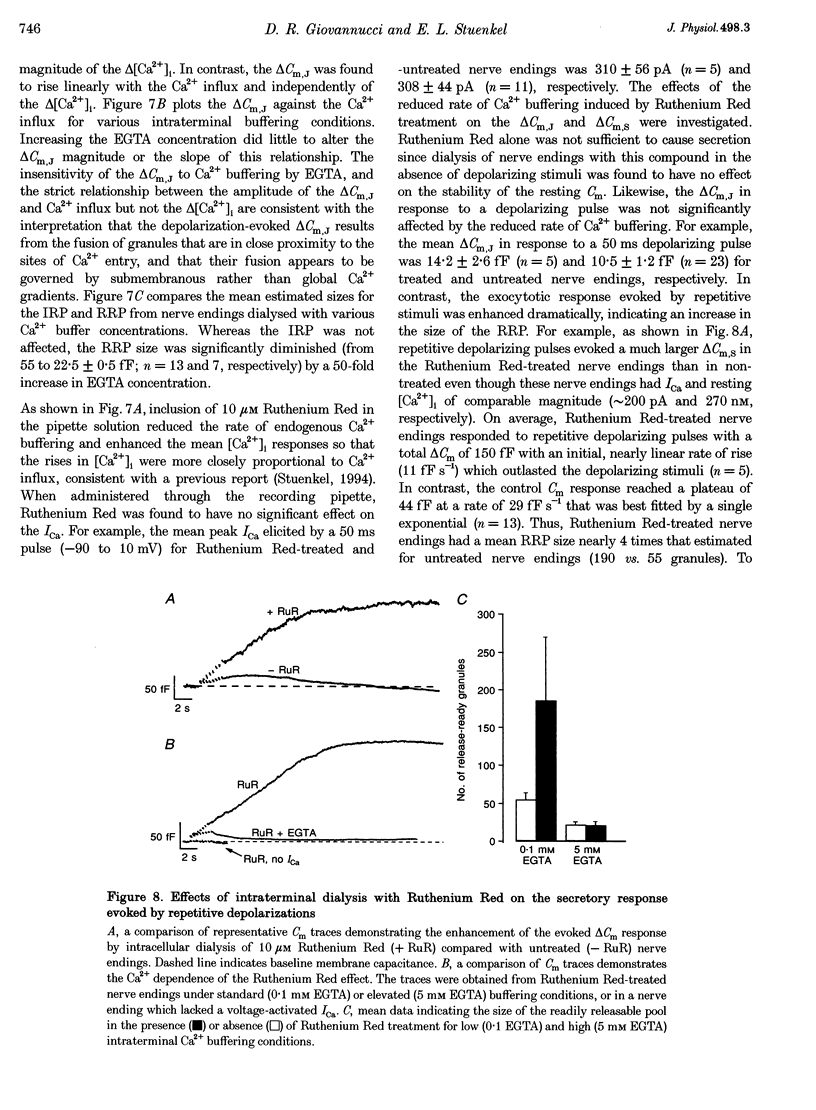
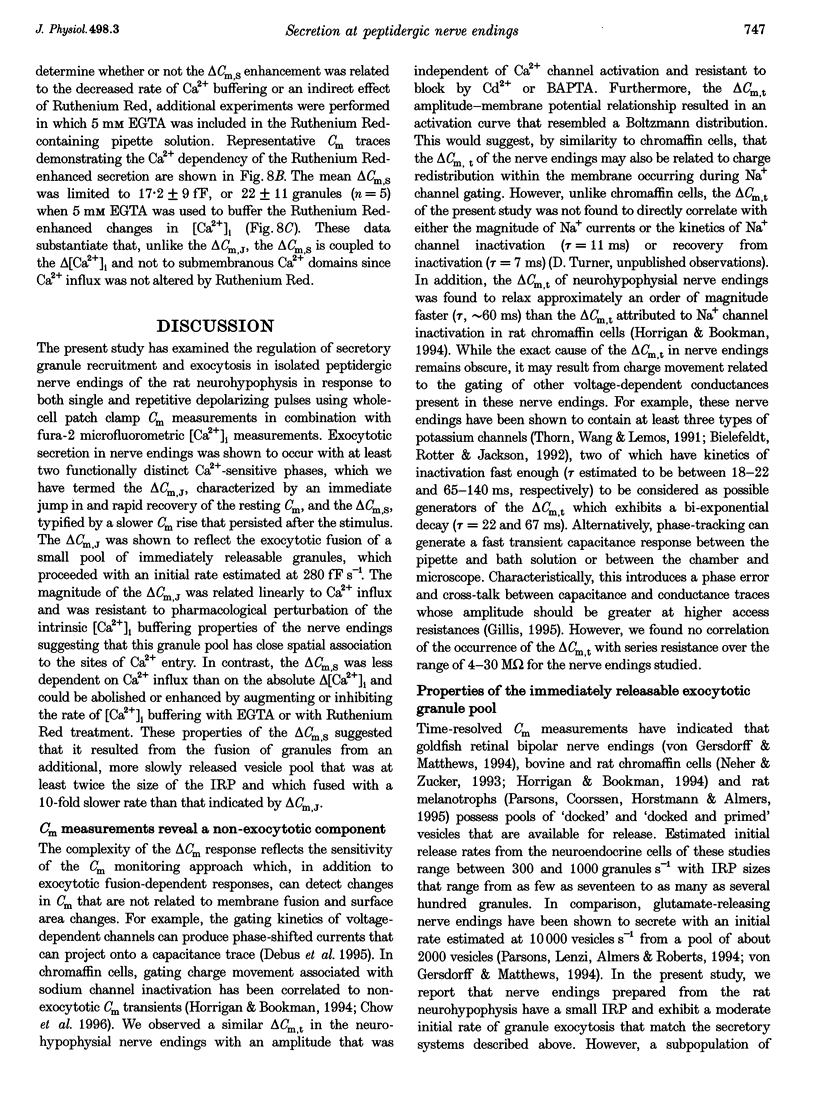
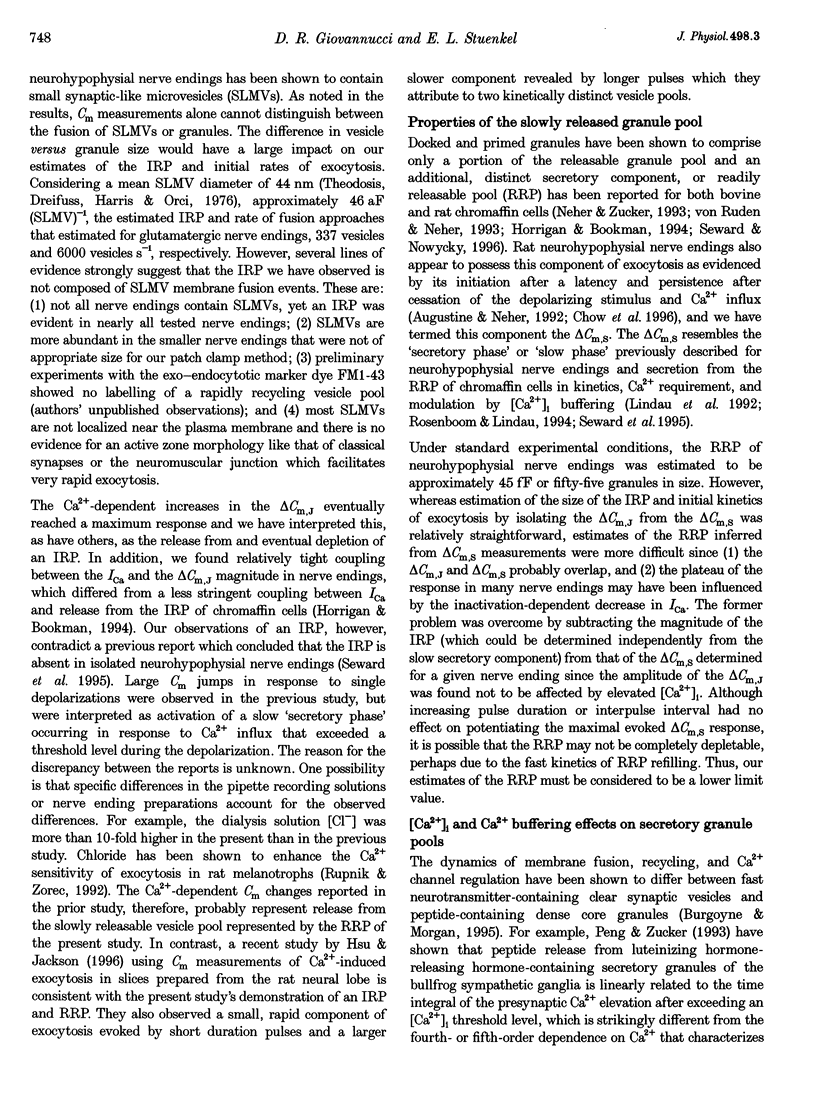
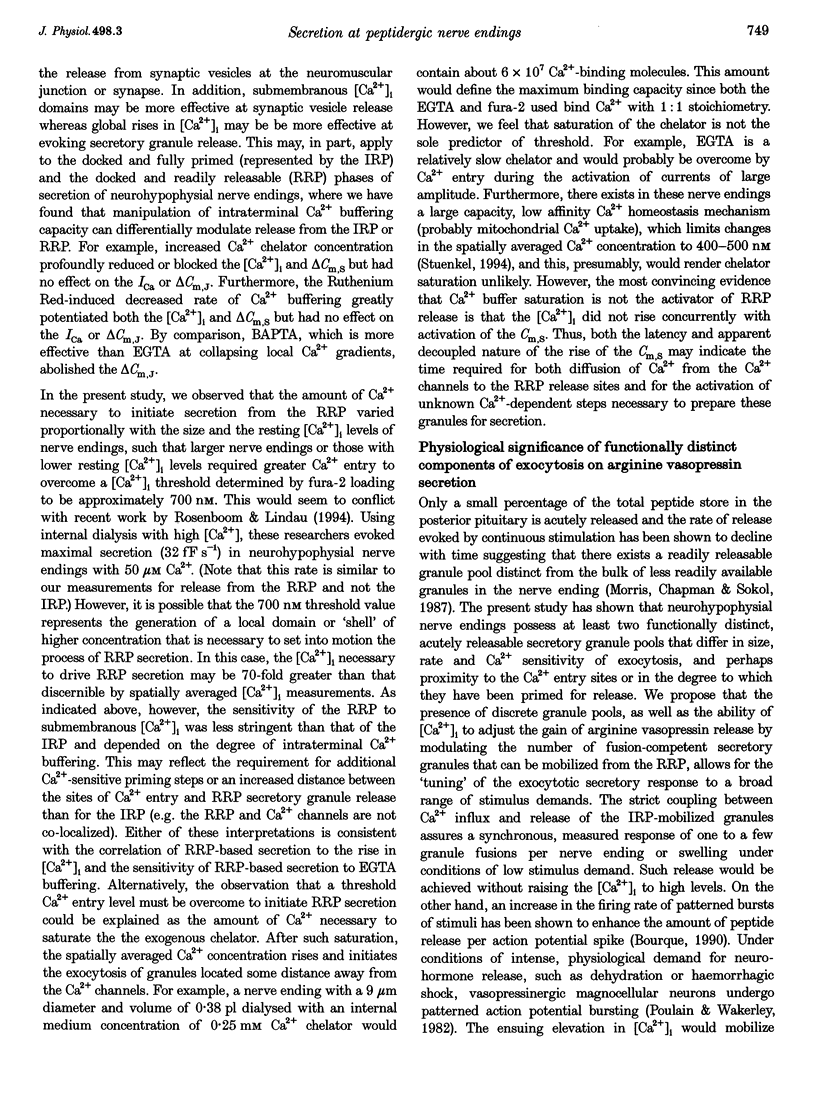
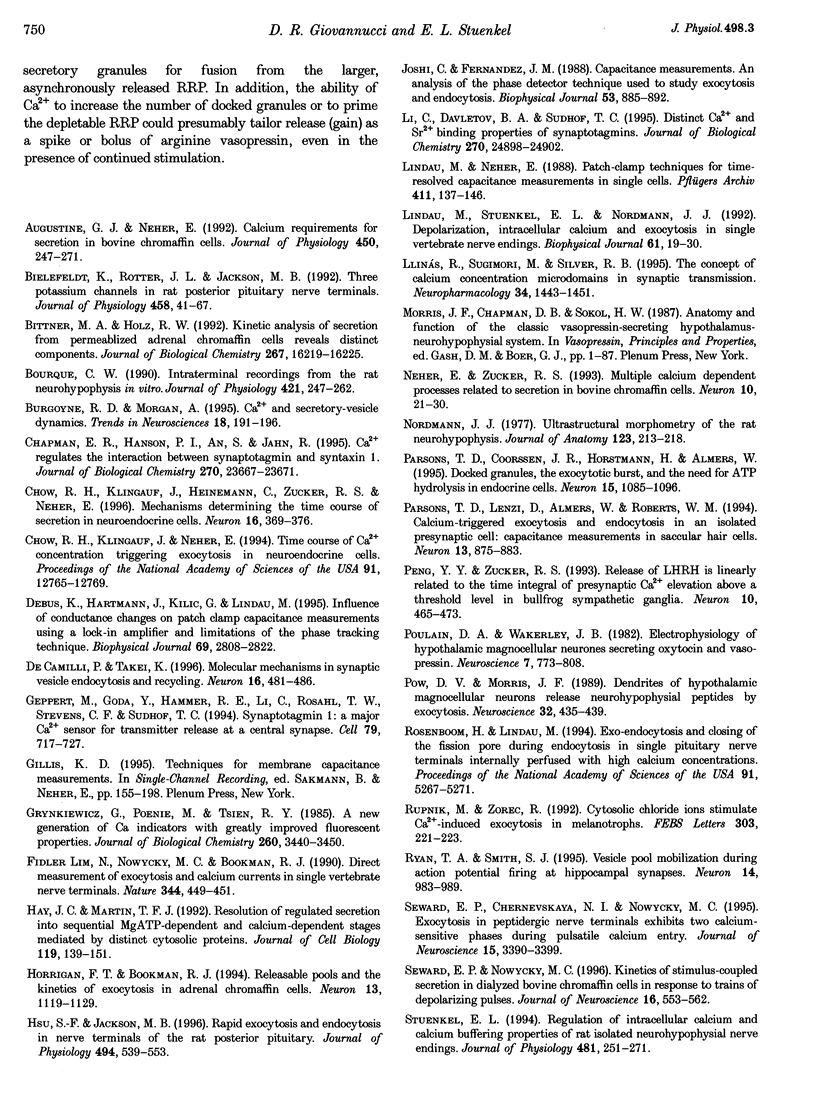
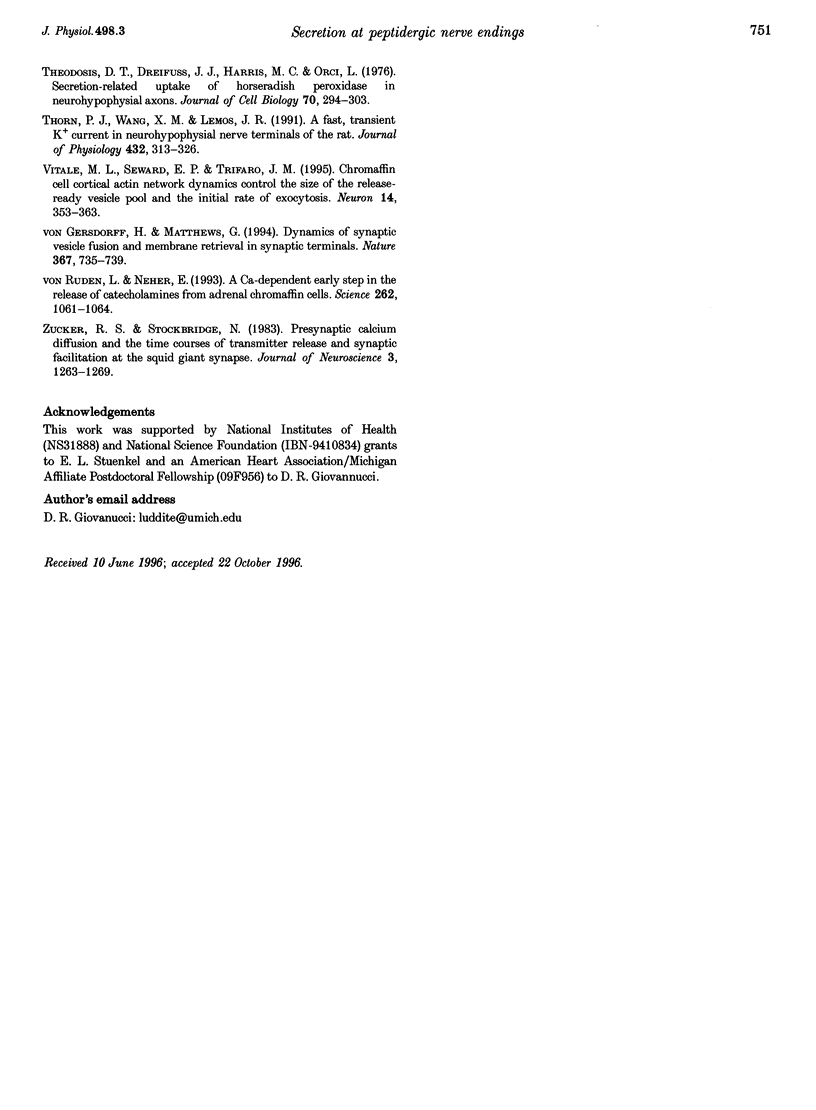
Images in this article
Selected References
These references are in PubMed. This may not be the complete list of references from this article.
- Augustine G. J., Neher E. Calcium requirements for secretion in bovine chromaffin cells. J Physiol. 1992 May;450:247–271. doi: 10.1113/jphysiol.1992.sp019126. [DOI] [PMC free article] [PubMed] [Google Scholar]
- Bielefeldt K., Rotter J. L., Jackson M. B. Three potassium channels in rat posterior pituitary nerve terminals. J Physiol. 1992 Dec;458:41–67. doi: 10.1113/jphysiol.1992.sp019405. [DOI] [PMC free article] [PubMed] [Google Scholar]
- Bittner M. A., Holz R. W. Kinetic analysis of secretion from permeabilized adrenal chromaffin cells reveals distinct components. J Biol Chem. 1992 Aug 15;267(23):16219–16225. [PubMed] [Google Scholar]
- Bourque C. W. Intraterminal recordings from the rat neurohypophysis in vitro. J Physiol. 1990 Feb;421:247–262. doi: 10.1113/jphysiol.1990.sp017943. [DOI] [PMC free article] [PubMed] [Google Scholar]
- Burgoyne R. D., Morgan A. Ca2+ and secretory-vesicle dynamics. Trends Neurosci. 1995 Apr;18(4):191–196. doi: 10.1016/0166-2236(95)93900-i. [DOI] [PubMed] [Google Scholar]
- Chapman E. R., Hanson P. I., An S., Jahn R. Ca2+ regulates the interaction between synaptotagmin and syntaxin 1. J Biol Chem. 1995 Oct 6;270(40):23667–23671. doi: 10.1074/jbc.270.40.23667. [DOI] [PubMed] [Google Scholar]
- Chow R. H., Klingauf J., Heinemann C., Zucker R. S., Neher E. Mechanisms determining the time course of secretion in neuroendocrine cells. Neuron. 1996 Feb;16(2):369–376. doi: 10.1016/s0896-6273(00)80054-9. [DOI] [PubMed] [Google Scholar]
- Chow R. H., Klingauf J., Neher E. Time course of Ca2+ concentration triggering exocytosis in neuroendocrine cells. Proc Natl Acad Sci U S A. 1994 Dec 20;91(26):12765–12769. doi: 10.1073/pnas.91.26.12765. [DOI] [PMC free article] [PubMed] [Google Scholar]
- De Camilli P., Takei K. Molecular mechanisms in synaptic vesicle endocytosis and recycling. Neuron. 1996 Mar;16(3):481–486. doi: 10.1016/s0896-6273(00)80068-9. [DOI] [PubMed] [Google Scholar]
- Debus K., Hartmann J., Kilic G., Lindau M. Influence of conductance changes on patch clamp capacitance measurements using a lock-in amplifier and limitations of the phase tracking technique. Biophys J. 1995 Dec;69(6):2808–2822. doi: 10.1016/S0006-3495(95)80154-2. [DOI] [PMC free article] [PubMed] [Google Scholar]
- Geppert M., Goda Y., Hammer R. E., Li C., Rosahl T. W., Stevens C. F., Südhof T. C. Synaptotagmin I: a major Ca2+ sensor for transmitter release at a central synapse. Cell. 1994 Nov 18;79(4):717–727. doi: 10.1016/0092-8674(94)90556-8. [DOI] [PubMed] [Google Scholar]
- Grynkiewicz G., Poenie M., Tsien R. Y. A new generation of Ca2+ indicators with greatly improved fluorescence properties. J Biol Chem. 1985 Mar 25;260(6):3440–3450. [PubMed] [Google Scholar]
- Hay J. C., Martin T. F. Resolution of regulated secretion into sequential MgATP-dependent and calcium-dependent stages mediated by distinct cytosolic proteins. J Cell Biol. 1992 Oct;119(1):139–151. doi: 10.1083/jcb.119.1.139. [DOI] [PMC free article] [PubMed] [Google Scholar]
- Horrigan F. T., Bookman R. J. Releasable pools and the kinetics of exocytosis in adrenal chromaffin cells. Neuron. 1994 Nov;13(5):1119–1129. doi: 10.1016/0896-6273(94)90050-7. [DOI] [PubMed] [Google Scholar]
- Hsu S. F., Jackson M. B. Rapid exocytosis and endocytosis in nerve terminals of the rat posterior pituitary. J Physiol. 1996 Jul 15;494(Pt 2):539–553. doi: 10.1113/jphysiol.1996.sp021512. [DOI] [PMC free article] [PubMed] [Google Scholar]
- Joshi C., Fernandez J. M. Capacitance measurements. An analysis of the phase detector technique used to study exocytosis and endocytosis. Biophys J. 1988 Jun;53(6):885–892. doi: 10.1016/S0006-3495(88)83169-2. [DOI] [PMC free article] [PubMed] [Google Scholar]
- Li C., Davletov B. A., Südhof T. C. Distinct Ca2+ and Sr2+ binding properties of synaptotagmins. Definition of candidate Ca2+ sensors for the fast and slow components of neurotransmitter release. J Biol Chem. 1995 Oct 20;270(42):24898–24902. doi: 10.1074/jbc.270.42.24898. [DOI] [PubMed] [Google Scholar]
- Lim N. F., Nowycky M. C., Bookman R. J. Direct measurement of exocytosis and calcium currents in single vertebrate nerve terminals. Nature. 1990 Mar 29;344(6265):449–451. doi: 10.1038/344449a0. [DOI] [PubMed] [Google Scholar]
- Lindau M., Neher E. Patch-clamp techniques for time-resolved capacitance measurements in single cells. Pflugers Arch. 1988 Feb;411(2):137–146. doi: 10.1007/BF00582306. [DOI] [PubMed] [Google Scholar]
- Lindau M., Stuenkel E. L., Nordmann J. J. Depolarization, intracellular calcium and exocytosis in single vertebrate nerve endings. Biophys J. 1992 Jan;61(1):19–30. doi: 10.1016/S0006-3495(92)81812-X. [DOI] [PMC free article] [PubMed] [Google Scholar]
- Llinás R., Sugimori M., Silver R. B. The concept of calcium concentration microdomains in synaptic transmission. Neuropharmacology. 1995 Nov;34(11):1443–1451. doi: 10.1016/0028-3908(95)00150-5. [DOI] [PubMed] [Google Scholar]
- Neher E., Zucker R. S. Multiple calcium-dependent processes related to secretion in bovine chromaffin cells. Neuron. 1993 Jan;10(1):21–30. doi: 10.1016/0896-6273(93)90238-m. [DOI] [PubMed] [Google Scholar]
- Nordmann J. J. Ultrastructural morphometry of the rat neurohypophysis. J Anat. 1977 Feb;123(Pt 1):213–218. [PMC free article] [PubMed] [Google Scholar]
- Parsons T. D., Coorssen J. R., Horstmann H., Almers W. Docked granules, the exocytic burst, and the need for ATP hydrolysis in endocrine cells. Neuron. 1995 Nov;15(5):1085–1096. doi: 10.1016/0896-6273(95)90097-7. [DOI] [PubMed] [Google Scholar]
- Parsons T. D., Lenzi D., Almers W., Roberts W. M. Calcium-triggered exocytosis and endocytosis in an isolated presynaptic cell: capacitance measurements in saccular hair cells. Neuron. 1994 Oct;13(4):875–883. doi: 10.1016/0896-6273(94)90253-4. [DOI] [PubMed] [Google Scholar]
- Peng Y. Y., Zucker R. S. Release of LHRH is linearly related to the time integral of presynaptic Ca2+ elevation above a threshold level in bullfrog sympathetic ganglia. Neuron. 1993 Mar;10(3):465–473. doi: 10.1016/0896-6273(93)90334-n. [DOI] [PubMed] [Google Scholar]
- Poulain D. A., Wakerley J. B. Electrophysiology of hypothalamic magnocellular neurones secreting oxytocin and vasopressin. Neuroscience. 1982 Apr;7(4):773–808. doi: 10.1016/0306-4522(82)90044-6. [DOI] [PubMed] [Google Scholar]
- Pow D. V., Morris J. F. Dendrites of hypothalamic magnocellular neurons release neurohypophysial peptides by exocytosis. Neuroscience. 1989;32(2):435–439. doi: 10.1016/0306-4522(89)90091-2. [DOI] [PubMed] [Google Scholar]
- Rosenboom H., Lindau M. Exo-endocytosis and closing of the fission pore during endocytosis in single pituitary nerve terminals internally perfused with high calcium concentrations. Proc Natl Acad Sci U S A. 1994 Jun 7;91(12):5267–5271. doi: 10.1073/pnas.91.12.5267. [DOI] [PMC free article] [PubMed] [Google Scholar]
- Rupnik M., Zorec R. Cytosolic chloride ions stimulate Ca(2+)-induced exocytosis in melanotrophs. FEBS Lett. 1992 Jun 1;303(2-3):221–223. doi: 10.1016/0014-5793(92)80524-k. [DOI] [PubMed] [Google Scholar]
- Ryan T. A., Smith S. J. Vesicle pool mobilization during action potential firing at hippocampal synapses. Neuron. 1995 May;14(5):983–989. doi: 10.1016/0896-6273(95)90336-4. [DOI] [PubMed] [Google Scholar]
- Seward E. P., Chernevskaya N. I., Nowycky M. C. Exocytosis in peptidergic nerve terminals exhibits two calcium-sensitive phases during pulsatile calcium entry. J Neurosci. 1995 May;15(5 Pt 1):3390–3399. doi: 10.1523/JNEUROSCI.15-05-03390.1995. [DOI] [PMC free article] [PubMed] [Google Scholar]
- Seward E. P., Nowycky M. C. Kinetics of stimulus-coupled secretion in dialyzed bovine chromaffin cells in response to trains of depolarizing pulses. J Neurosci. 1996 Jan 15;16(2):553–562. doi: 10.1523/JNEUROSCI.16-02-00553.1996. [DOI] [PMC free article] [PubMed] [Google Scholar]
- Stuenkel E. L. Regulation of intracellular calcium and calcium buffering properties of rat isolated neurohypophysial nerve endings. J Physiol. 1994 Dec 1;481(Pt 2):251–271. doi: 10.1113/jphysiol.1994.sp020436. [DOI] [PMC free article] [PubMed] [Google Scholar]
- Theodosis D. T., Dreifuss J., Harris M. C., Orci L. Secretion-related uptake of horseradish peroxidase in neurohypophysial axons. J Cell Biol. 1976 Aug;70(2 Pt 1):294–303. doi: 10.1083/jcb.70.2.294. [DOI] [PMC free article] [PubMed] [Google Scholar]
- Thorn P. J., Wang X. M., Lemos J. R. A fast, transient K+ current in neurohypophysial nerve terminals of the rat. J Physiol. 1991 Jan;432:313–326. doi: 10.1113/jphysiol.1991.sp018386. [DOI] [PMC free article] [PubMed] [Google Scholar]
- Vitale M. L., Seward E. P., Trifaró J. M. Chromaffin cell cortical actin network dynamics control the size of the release-ready vesicle pool and the initial rate of exocytosis. Neuron. 1995 Feb;14(2):353–363. doi: 10.1016/0896-6273(95)90291-0. [DOI] [PubMed] [Google Scholar]
- Zucker R. S., Stockbridge N. Presynaptic calcium diffusion and the time courses of transmitter release and synaptic facilitation at the squid giant synapse. J Neurosci. 1983 Jun;3(6):1263–1269. doi: 10.1523/JNEUROSCI.03-06-01263.1983. [DOI] [PMC free article] [PubMed] [Google Scholar]
- von Gersdorff H., Matthews G. Dynamics of synaptic vesicle fusion and membrane retrieval in synaptic terminals. Nature. 1994 Feb 24;367(6465):735–739. doi: 10.1038/367735a0. [DOI] [PubMed] [Google Scholar]
- von Rüden L., Neher E. A Ca-dependent early step in the release of catecholamines from adrenal chromaffin cells. Science. 1993 Nov 12;262(5136):1061–1065. doi: 10.1126/science.8235626. [DOI] [PubMed] [Google Scholar]