Abstract
1. Post-transection changes in intracellular Na+ ([Na+]i) were measured in lizard peripheral axons ionophoretically injected with the Na(+)-sensitive ratiometric dye, sodium-binding benzofuran isophthalate (SBFI). 2. Following axonal transection in physiological saline [Na+]i increased to more than 100 mM in a region that quickly extended hundreds of micrometers from the transection site. This post-transection increase in [Na+]i was similar when the bath contained 5 microM tetrodotoxin, but was absent in Na(+)-free solution. Depolarization of uncut axons in 50 mM K+ produced little or no elevation of [Na+]i until veratridine was added. These results suggest that the post-transection increase in [Na+]i was due mainly to Na+ entry via the cut end, rather than via depolarization-activated Na+ channels. 3. The spatiotemporal profile of the post-transection increase in [Na+]i could be accounted for by movement of Na+ from the cut end with an apparent diffusion coefficient of 1.3 x 10(-5) cm2 s-1. 4. [Na+]i began to decline toward resting levels by 20 +/- 15 min (mean +/- S.D.) post-transection, except in regions of the axon within 160 +/- 85 microns of the transection site, where [Na+]i remained high. The boundary between axonal regions in which [Na+]i did or did not recover probably defines a locus of resealing of the axonal membrane. 5. [Na+]i returned to resting values within about 1 h after resealing, even in axonal regions where the normal transmembrane [Na+] gradient had completely dissipated. The recovery of [Na+]i was faster and reached lower levels than expected by diffusional redistribution of Na+ along the axon. Partial recovery occurred even in an isolated internode, indicating that the internodal axolemma can actively extrude Na+.
Full text
PDF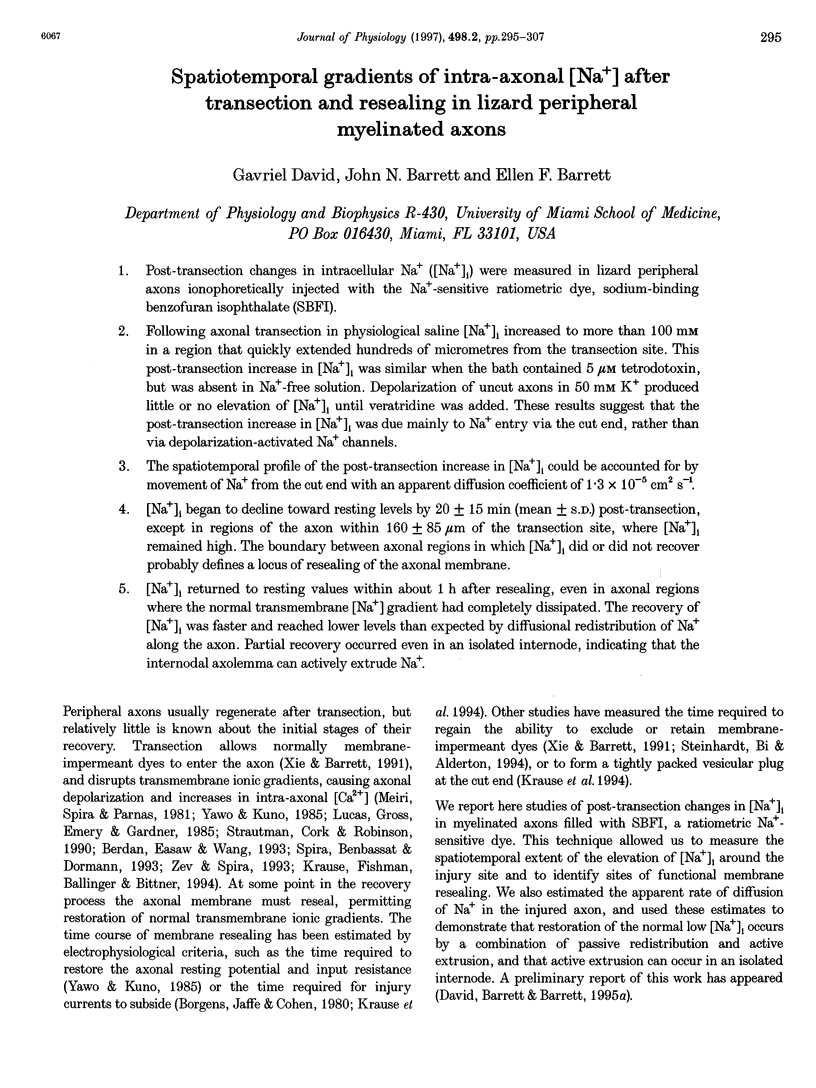
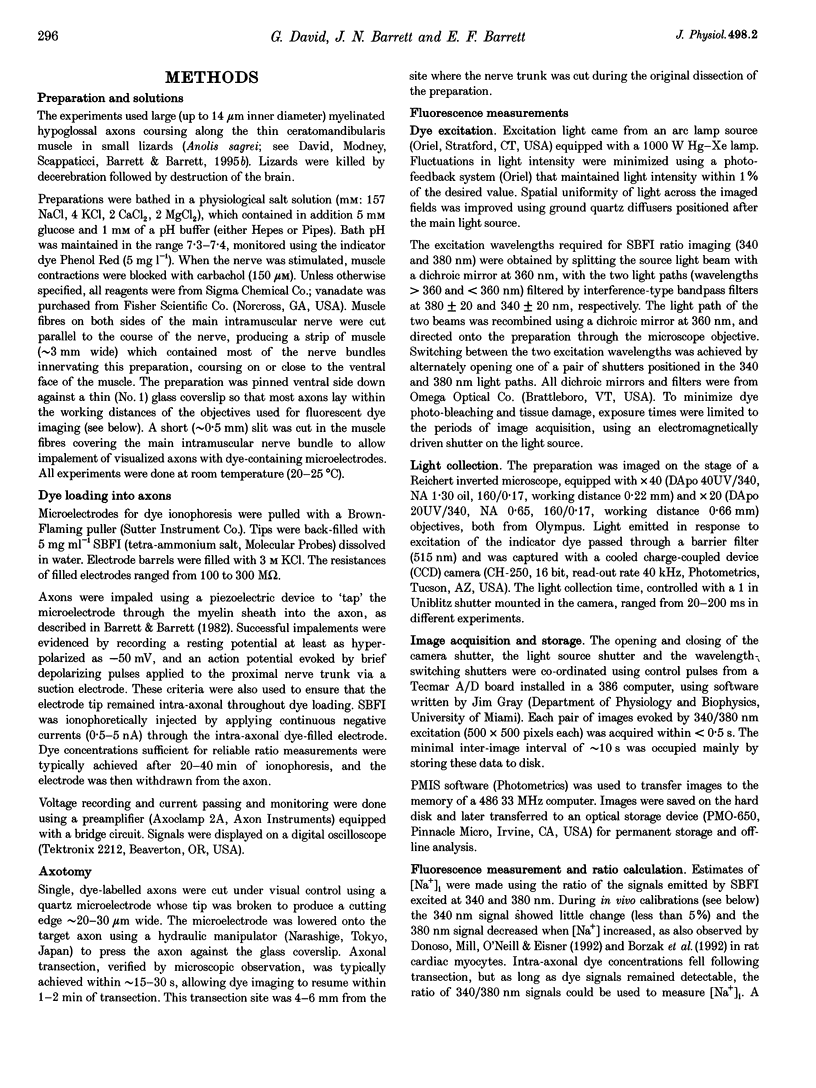
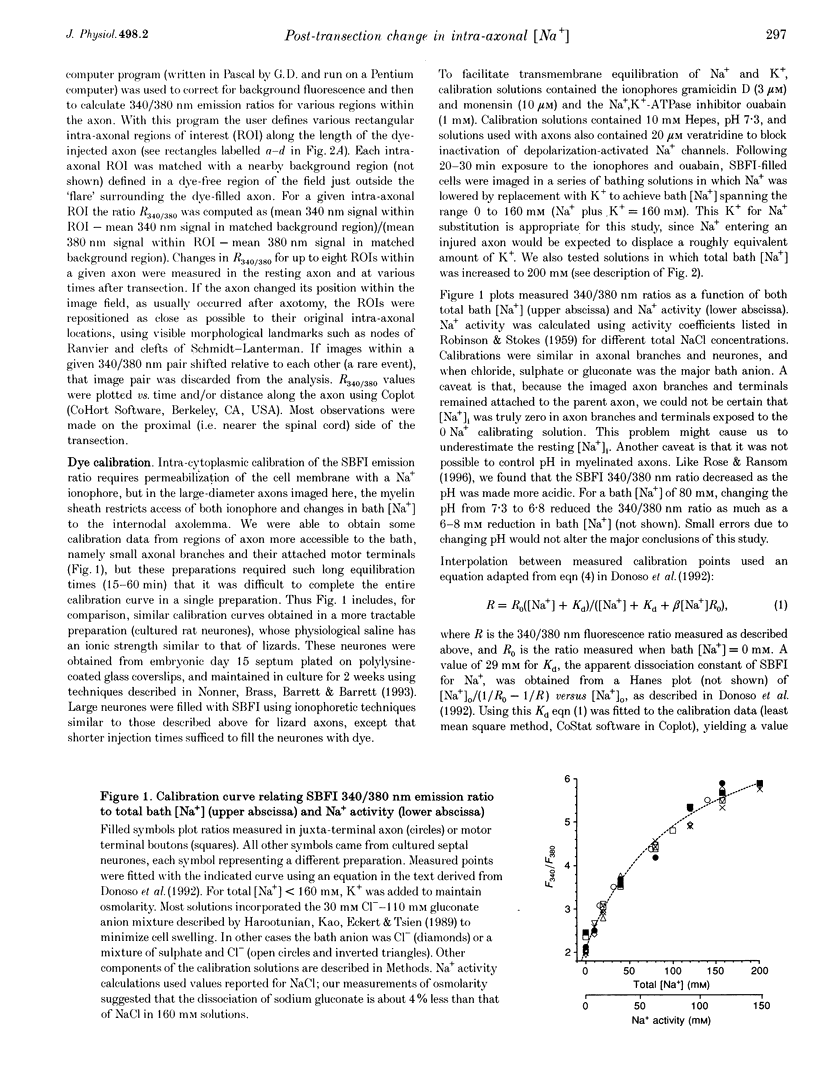
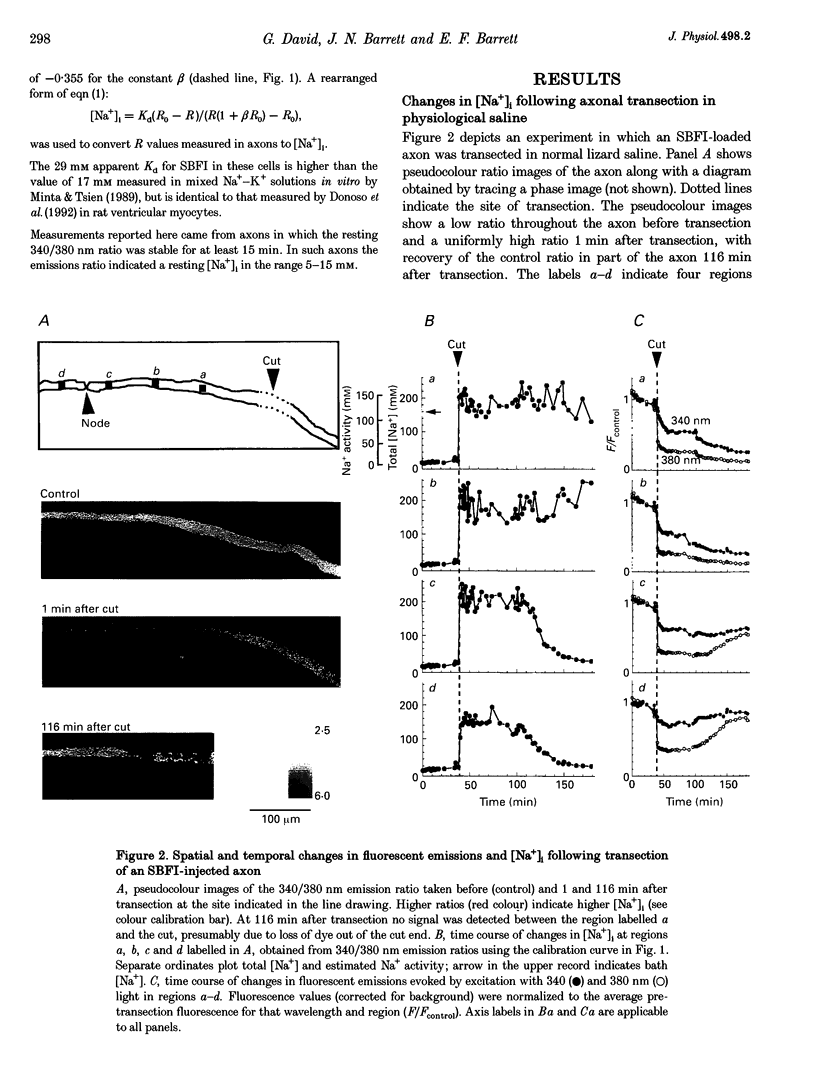
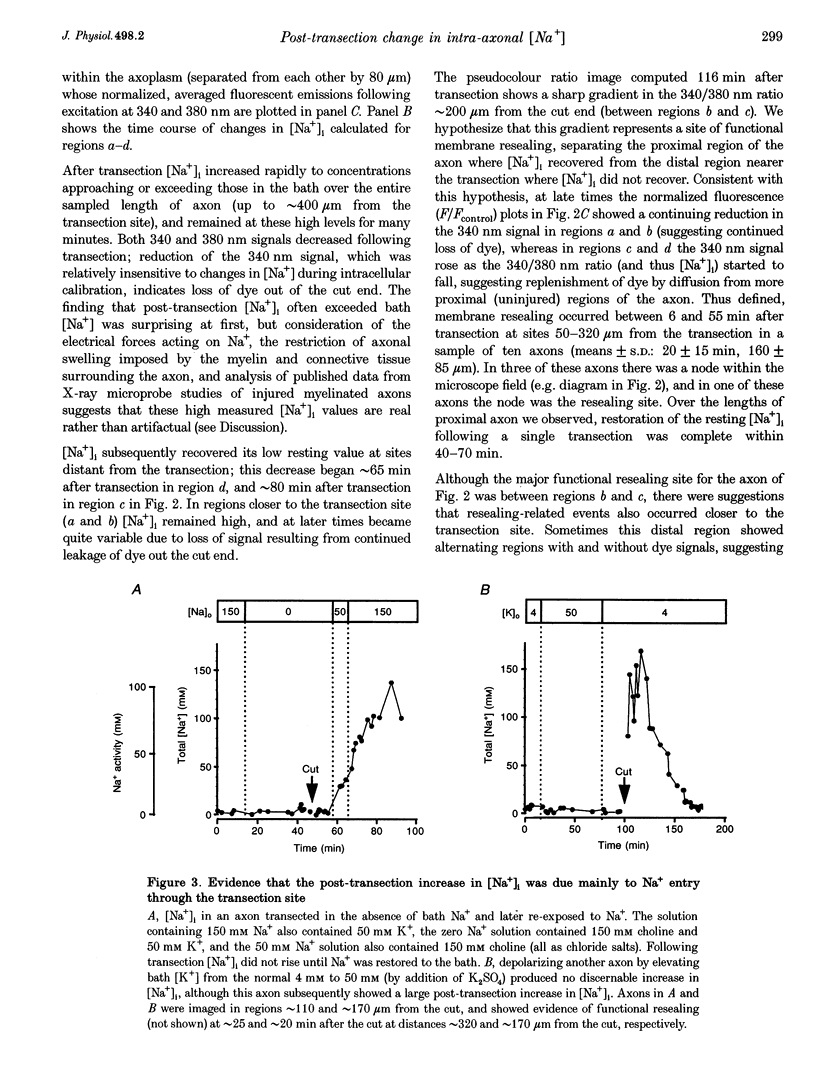
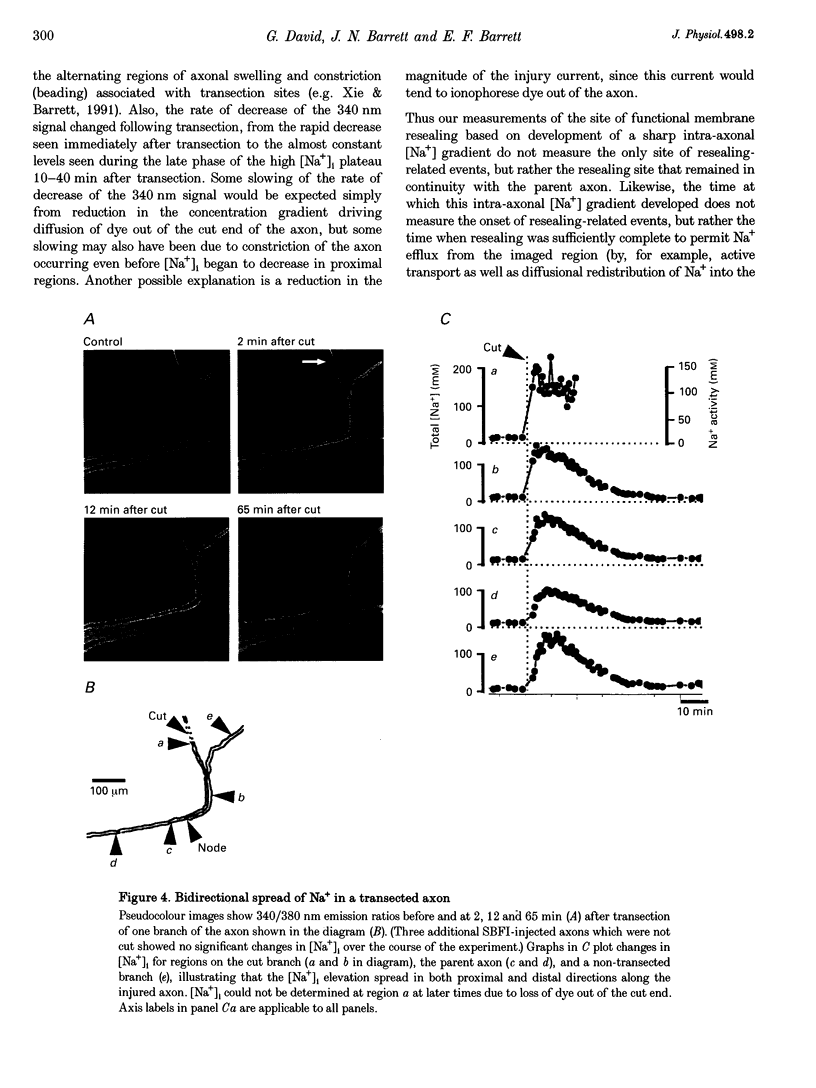
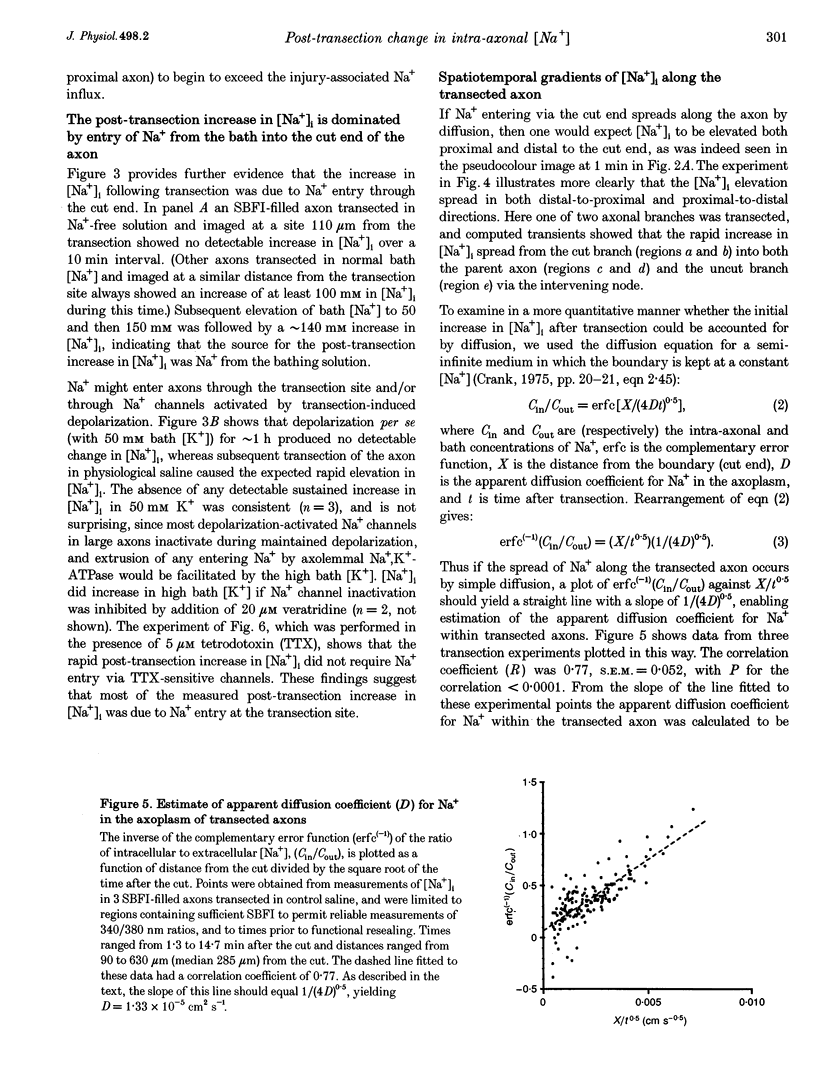
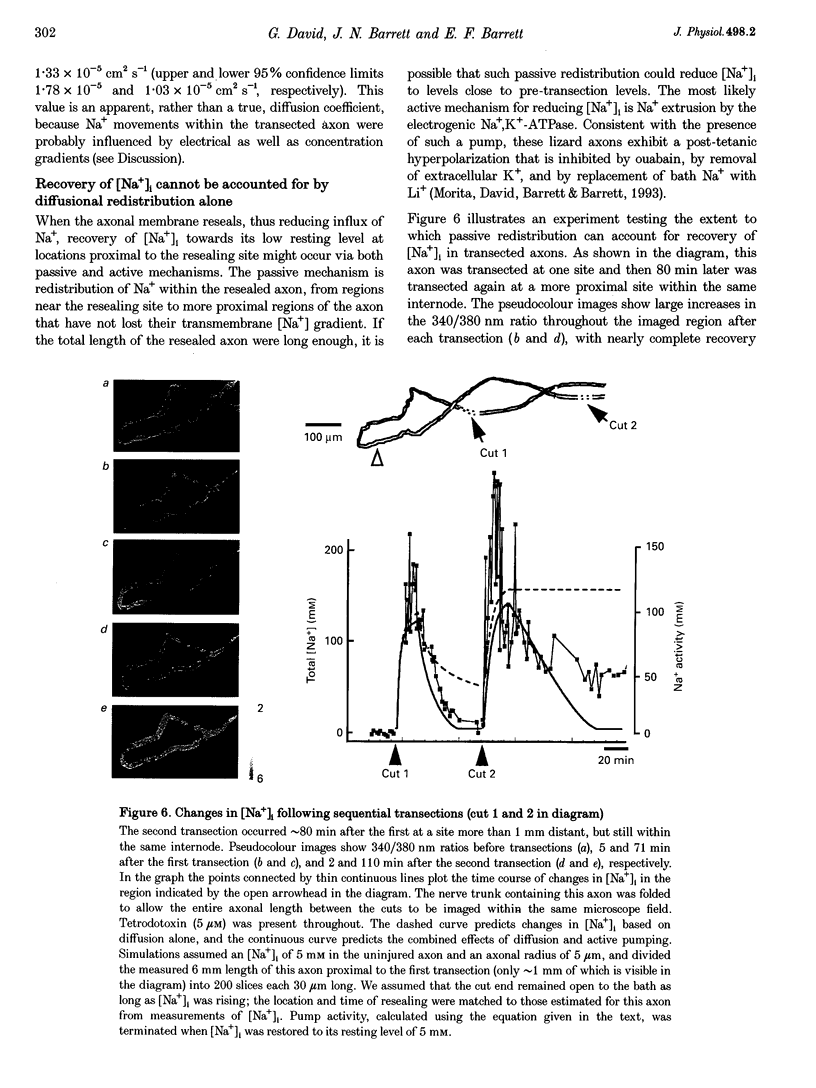
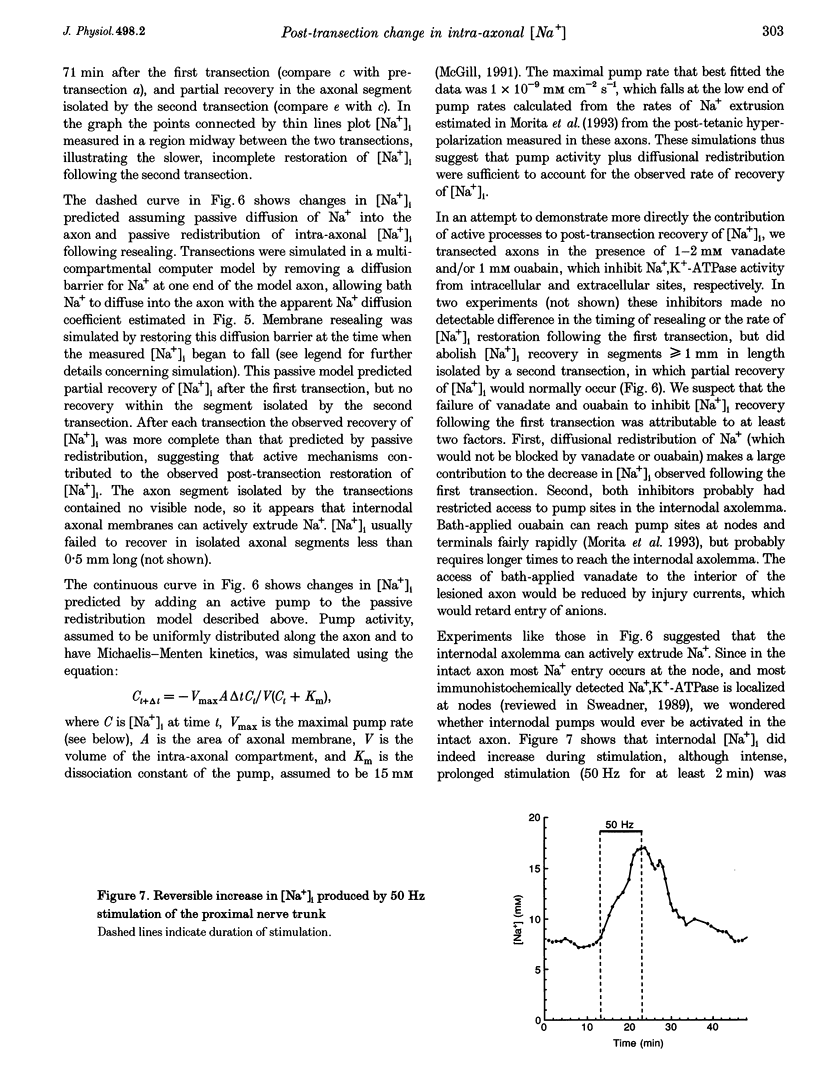
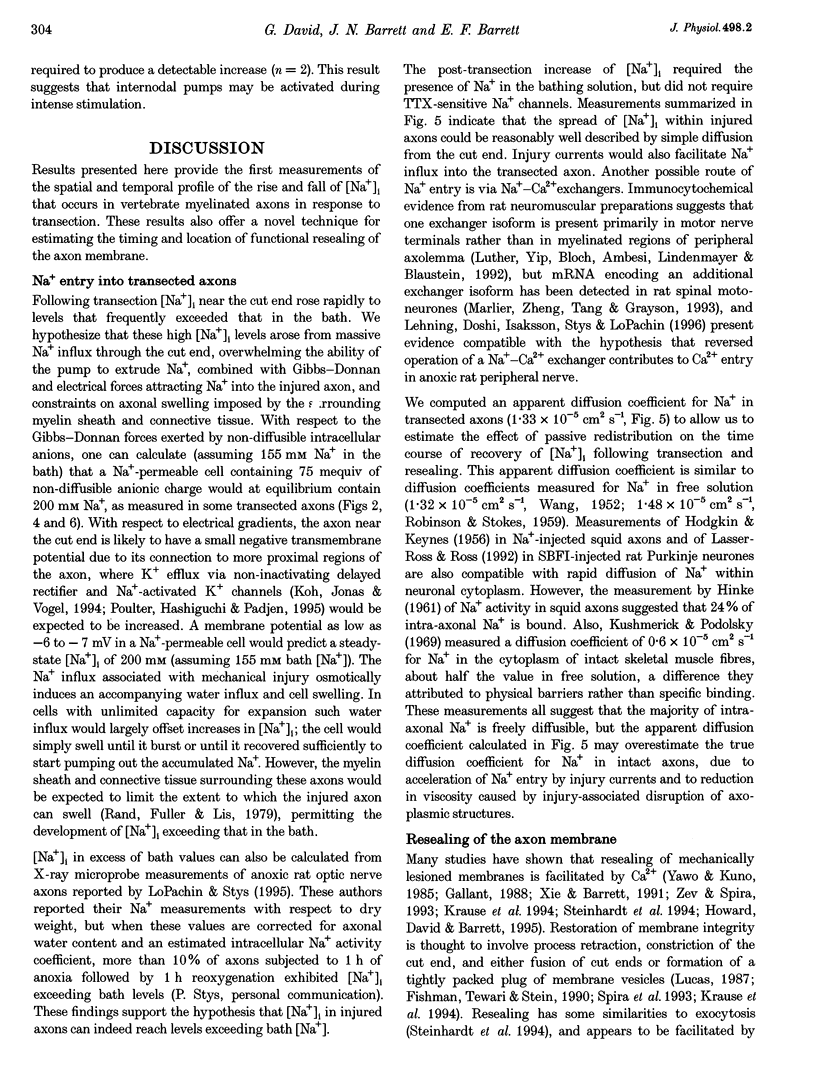
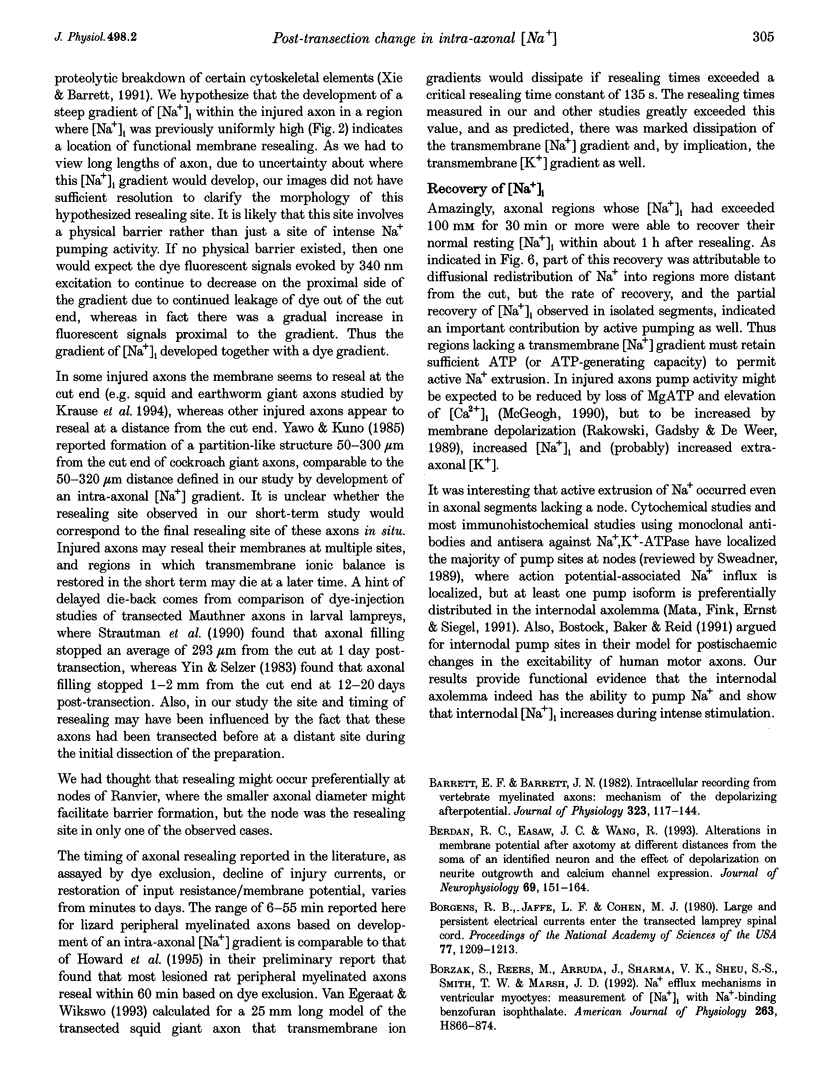
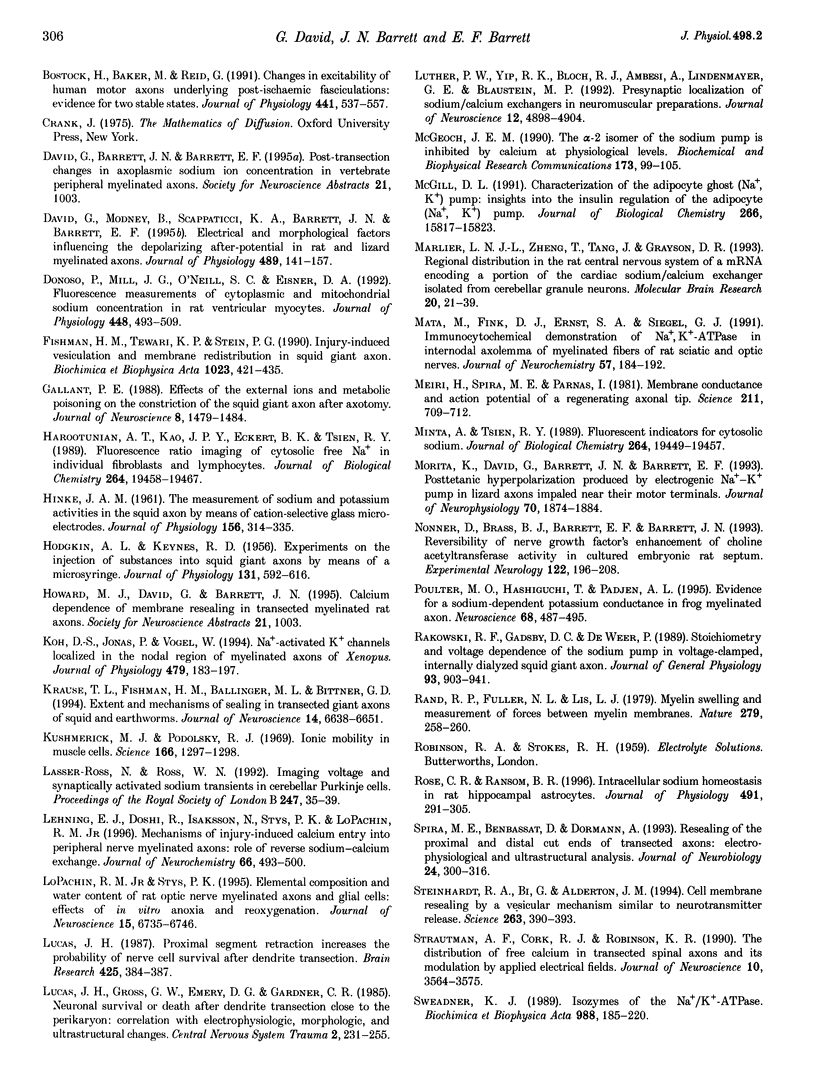
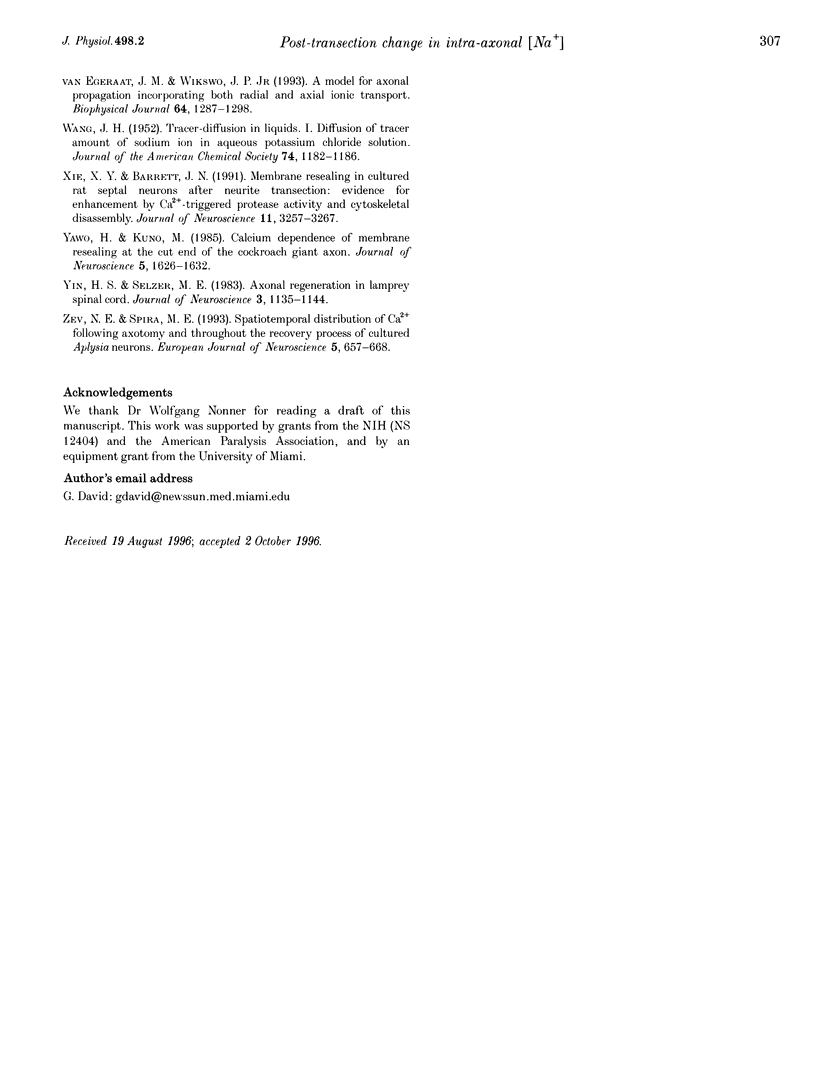
Images in this article
Selected References
These references are in PubMed. This may not be the complete list of references from this article.
- Barrett E. F., Barrett J. N. Intracellular recording from vertebrate myelinated axons: mechanism of the depolarizing afterpotential. J Physiol. 1982 Feb;323:117–144. doi: 10.1113/jphysiol.1982.sp014064. [DOI] [PMC free article] [PubMed] [Google Scholar]
- Berdan R. C., Easaw J. C., Wang R. Alterations in membrane potential after axotomy at different distances from the soma of an identified neuron and the effect of depolarization on neurite outgrowth and calcium channel expression. J Neurophysiol. 1993 Jan;69(1):151–164. doi: 10.1152/jn.1993.69.1.151. [DOI] [PubMed] [Google Scholar]
- Borgens R. B., Jaffe L. F., Cohen M. J. Large and persistent electrical currents enter the transected lamprey spinal cord. Proc Natl Acad Sci U S A. 1980 Feb;77(2):1209–1213. doi: 10.1073/pnas.77.2.1209. [DOI] [PMC free article] [PubMed] [Google Scholar]
- Bostock H., Baker M., Reid G. Changes in excitability of human motor axons underlying post-ischaemic fasciculations: evidence for two stable states. J Physiol. 1991 Sep;441:537–557. doi: 10.1113/jphysiol.1991.sp018766. [DOI] [PMC free article] [PubMed] [Google Scholar]
- David G., Modney B., Scappaticci K. A., Barrett J. N., Barrett E. F. Electrical and morphological factors influencing the depolarizing after-potential in rat and lizard myelinated axons. J Physiol. 1995 Nov 15;489(Pt 1):141–157. doi: 10.1113/jphysiol.1995.sp021037. [DOI] [PMC free article] [PubMed] [Google Scholar]
- Donoso P., Mill J. G., O'Neill S. C., Eisner D. A. Fluorescence measurements of cytoplasmic and mitochondrial sodium concentration in rat ventricular myocytes. J Physiol. 1992 Mar;448:493–509. doi: 10.1113/jphysiol.1992.sp019053. [DOI] [PMC free article] [PubMed] [Google Scholar]
- Fishman H. M., Tewari K. P., Stein P. G. Injury-induced vesiculation and membrane redistribution in squid giant axon. Biochim Biophys Acta. 1990 Apr 30;1023(3):421–435. doi: 10.1016/0005-2736(90)90135-b. [DOI] [PubMed] [Google Scholar]
- Gallant P. E. Effects of the external ions and metabolic poisoning on the constriction of the squid giant axon after axotomy. J Neurosci. 1988 May;8(5):1479–1484. doi: 10.1523/JNEUROSCI.08-05-01479.1988. [DOI] [PMC free article] [PubMed] [Google Scholar]
- HINKE J. A. The measurement of sodium and potassium activities in the squid axon by means of cation-selective glass micro-electrodes. J Physiol. 1961 Apr;156:314–335. doi: 10.1113/jphysiol.1961.sp006678. [DOI] [PMC free article] [PubMed] [Google Scholar]
- HODGKIN A. L., KEYNES R. D. Experiments on the injection of substances into squid giant axons by means of a microsyringe. J Physiol. 1956 Mar 28;131(3):592–616. doi: 10.1113/jphysiol.1956.sp005485. [DOI] [PMC free article] [PubMed] [Google Scholar]
- Harootunian A. T., Kao J. P., Eckert B. K., Tsien R. Y. Fluorescence ratio imaging of cytosolic free Na+ in individual fibroblasts and lymphocytes. J Biol Chem. 1989 Nov 15;264(32):19458–19467. [PubMed] [Google Scholar]
- Koh D. S., Jonas P., Vogel W. Na(+)-activated K+ channels localized in the nodal region of myelinated axons of Xenopus. J Physiol. 1994 Sep 1;479(Pt 2):183–197. doi: 10.1113/jphysiol.1994.sp020287. [DOI] [PMC free article] [PubMed] [Google Scholar]
- Krause T. L., Fishman H. M., Ballinger M. L., Bittner G. D. Extent and mechanism of sealing in transected giant axons of squid and earthworms. J Neurosci. 1994 Nov;14(11 Pt 1):6638–6651. doi: 10.1523/JNEUROSCI.14-11-06638.1994. [DOI] [PMC free article] [PubMed] [Google Scholar]
- Kushmerick M. J., Podolsky R. J. Ionic mobility in muscle cells. Science. 1969 Dec 5;166(3910):1297–1298. doi: 10.1126/science.166.3910.1297. [DOI] [PubMed] [Google Scholar]
- Lasser-Ross N., Ross W. N. Imaging voltage and synaptically activated sodium transients in cerebellar Purkinje cells. Proc Biol Sci. 1992 Jan 22;247(1318):35–39. doi: 10.1098/rspb.1992.0006. [DOI] [PubMed] [Google Scholar]
- Lehning E. J., Doshi R., Isaksson N., Stys P. K., LoPachin R. M., Jr Mechanisms of injury-induced calcium entry into peripheral nerve myelinated axons: role of reverse sodium-calcium exchange. J Neurochem. 1996 Feb;66(2):493–500. doi: 10.1046/j.1471-4159.1996.66020493.x. [DOI] [PubMed] [Google Scholar]
- LoPachin R. M., Jr, Stys P. K. Elemental composition and water content of rat optic nerve myelinated axons and glial cells: effects of in vitro anoxia and reoxygenation. J Neurosci. 1995 Oct;15(10):6735–6746. doi: 10.1523/JNEUROSCI.15-10-06735.1995. [DOI] [PMC free article] [PubMed] [Google Scholar]
- Lucas J. H., Gross G. W., Emery D. G., Gardner C. R. Neuronal survival or death after dendrite transection close to the perikaryon: correlation with electrophysiologic, morphologic, and ultrastructural changes. Cent Nerv Syst Trauma. 1985 Winter;2(4):231–255. doi: 10.1089/cns.1985.2.231. [DOI] [PubMed] [Google Scholar]
- Lucas J. H. Proximal segment retraction increases the probability of nerve cell survival after dendrite transection. Brain Res. 1987 Nov 10;425(2):384–387. doi: 10.1016/0006-8993(87)90526-9. [DOI] [PubMed] [Google Scholar]
- Luther P. W., Yip R. K., Bloch R. J., Ambesi A., Lindenmayer G. E., Blaustein M. P. Presynaptic localization of sodium/calcium exchangers in neuromuscular preparations. J Neurosci. 1992 Dec;12(12):4898–4904. doi: 10.1523/JNEUROSCI.12-12-04898.1992. [DOI] [PMC free article] [PubMed] [Google Scholar]
- Marlier L. N., Zheng T., Tang J., Grayson D. R. Regional distribution in the rat central nervous system of a mRNA encoding a portion of the cardiac sodium/calcium exchanger isolated from cerebellar granule neurons. Brain Res Mol Brain Res. 1993 Oct;20(1-2):21–39. doi: 10.1016/0169-328x(93)90107-z. [DOI] [PubMed] [Google Scholar]
- Mata M., Fink D. J., Ernst S. A., Siegel G. J. Immunocytochemical demonstration of Na+,K(+)-ATPase in internodal axolemma of myelinated fibers of rat sciatic and optic nerves. J Neurochem. 1991 Jul;57(1):184–192. doi: 10.1111/j.1471-4159.1991.tb02114.x. [DOI] [PubMed] [Google Scholar]
- McGeoch J. E. The alpha-2 isomer of the sodium pump is inhibited by calcium at physiological levels. Biochem Biophys Res Commun. 1990 Nov 30;173(1):99–105. doi: 10.1016/s0006-291x(05)81027-7. [DOI] [PubMed] [Google Scholar]
- McGill D. L. Characterization of the adipocyte ghost (Na+,K+) pump. Insights into the insulin regulation of the adipocyte (Na+,K+) pump. J Biol Chem. 1991 Aug 25;266(24):15817–15823. [PubMed] [Google Scholar]
- Meiri H., Spira M. E., Parnas I. Membrane conductance and action potential of a regenerating axonal tip. Science. 1981 Feb 13;211(4483):709–712. doi: 10.1126/science.7455707. [DOI] [PubMed] [Google Scholar]
- Minta A., Tsien R. Y. Fluorescent indicators for cytosolic sodium. J Biol Chem. 1989 Nov 15;264(32):19449–19457. [PubMed] [Google Scholar]
- Morita K., David G., Barrett J. N., Barrett E. F. Posttetanic hyperpolarization produced by electrogenic Na(+)-K+ pump in lizard axons impaled near their motor terminals. J Neurophysiol. 1993 Nov;70(5):1874–1884. doi: 10.1152/jn.1993.70.5.1874. [DOI] [PubMed] [Google Scholar]
- Nonner D., Brass B. J., Barrett E. F., Barrett J. N. Reversibility of nerve growth factor's enhancement of choline acetyltransferase activity in cultured embryonic rat septum. Exp Neurol. 1993 Aug;122(2):196–208. doi: 10.1006/exnr.1993.1120. [DOI] [PubMed] [Google Scholar]
- Poulter M. O., Hashiguchi T., Padjen A. L. Evidence for a sodium-dependent potassium conductance in frog myelinated axon. Neuroscience. 1995 Sep;68(2):487–495. doi: 10.1016/0306-4522(95)00138-9. [DOI] [PubMed] [Google Scholar]
- Rakowski R. F., Gadsby D. C., De Weer P. Stoichiometry and voltage dependence of the sodium pump in voltage-clamped, internally dialyzed squid giant axon. J Gen Physiol. 1989 May;93(5):903–941. doi: 10.1085/jgp.93.5.903. [DOI] [PMC free article] [PubMed] [Google Scholar]
- Rand R. P., Fuller N. L., Lis L. J. Myelin swelling and measurement of forces between myelin membranes. Nature. 1979 May 17;279(5710):258–260. doi: 10.1038/279258a0. [DOI] [PubMed] [Google Scholar]
- Rose C. R., Ransom B. R. Intracellular sodium homeostasis in rat hippocampal astrocytes. J Physiol. 1996 Mar 1;491(Pt 2):291–305. doi: 10.1113/jphysiol.1996.sp021216. [DOI] [PMC free article] [PubMed] [Google Scholar]
- Spira M. E., Benbassat D., Dormann A. Resealing of the proximal and distal cut ends of transected axons: electrophysiological and ultrastructural analysis. J Neurobiol. 1993 Mar;24(3):300–316. doi: 10.1002/neu.480240304. [DOI] [PubMed] [Google Scholar]
- Steinhardt R. A., Bi G., Alderton J. M. Cell membrane resealing by a vesicular mechanism similar to neurotransmitter release. Science. 1994 Jan 21;263(5145):390–393. doi: 10.1126/science.7904084. [DOI] [PubMed] [Google Scholar]
- Strautman A. F., Cork R. J., Robinson K. R. The distribution of free calcium in transected spinal axons and its modulation by applied electrical fields. J Neurosci. 1990 Nov;10(11):3564–3575. doi: 10.1523/JNEUROSCI.10-11-03564.1990. [DOI] [PMC free article] [PubMed] [Google Scholar]
- Sweadner K. J. Isozymes of the Na+/K+-ATPase. Biochim Biophys Acta. 1989 May 9;988(2):185–220. doi: 10.1016/0304-4157(89)90019-1. [DOI] [PubMed] [Google Scholar]
- Xie X. Y., Barrett J. N. Membrane resealing in cultured rat septal neurons after neurite transection: evidence for enhancement by Ca(2+)-triggered protease activity and cytoskeletal disassembly. J Neurosci. 1991 Oct;11(10):3257–3267. doi: 10.1523/JNEUROSCI.11-10-03257.1991. [DOI] [PMC free article] [PubMed] [Google Scholar]
- Yawo H., Kuno M. Calcium dependence of membrane sealing at the cut end of the cockroach giant axon. J Neurosci. 1985 Jun;5(6):1626–1632. doi: 10.1523/JNEUROSCI.05-06-01626.1985. [DOI] [PMC free article] [PubMed] [Google Scholar]
- Yin H. S., Selzer M. E. Axonal regeneration in lamprey spinal cord. J Neurosci. 1983 Jun;3(6):1135–1144. doi: 10.1523/JNEUROSCI.03-06-01135.1983. [DOI] [PMC free article] [PubMed] [Google Scholar]
- Ziv N. E., Spira M. E. Spatiotemporal distribution of Ca2+ following axotomy and throughout the recovery process of cultured Aplysia neurons. Eur J Neurosci. 1993 Jun 1;5(6):657–668. doi: 10.1111/j.1460-9568.1993.tb00531.x. [DOI] [PubMed] [Google Scholar]
- van Egeraat J. M., Wikswo J. P., Jr A model for axonal propagation incorporating both radial and axial ionic transport. Biophys J. 1993 Apr;64(4):1287–1298. doi: 10.1016/S0006-3495(93)81495-4. [DOI] [PMC free article] [PubMed] [Google Scholar]