Abstract
1. The time course of cross-bridge detachment-attachment following a step stretch was determined in single frog muscle fibres (at 4 degrees (1 and 2.1 microns sarcomere length) by imposing, under sarcomere length control by a striation follower, test step releases of various amplitudes (2-13 nm per half-sarcomere) at successive times (4-55 ms) after a conditioning stretch of approximately 4 nm per half-sarcomere. 2. The comparison with the control tension transients, elicited by releases not preceded by the conditioning stretch, shows that, early after the conditioning stretch, the quick tension recovery following small releases is depressed and the quick tension recovery following large releases is potentiated. Both effects are expected as a consequence of the strain produced in the cross-bridges by the conditioning stretch. 3. These effects disappear and the tension transient is reprimed, indicating substitution of freshly attached cross-bridges for strained cross-bridges, with a time constant of approximately 10 ms. 4. A novel multiple-exponential equation, based on the hypothesis of complete substitution of freshly attached cross-bridges for the cross-bridges that underwent the stretch, has been used to fit the whole tension transient following step stretches of different sizes (2-6 nm per half-sarcomere). For a stretch of 4 nm, the time constant of the exponential process responsible for cross-bridge detachment (tau d, 9.3 ms) almost coincides with the time constant of repriming as measured by the double-step experiments. The time constant of the exponential process representing the cumulative effects of attachment and force generation (tau 3) is 13.6 ms. 5. For stretches of different sizes the amount of quick tension recovery attributable to the reversal of the working stroke elicited by the stretches is estimated by subtracting, from the original tension transient, the contribution to tension recovery due to detachment-attachment of cross-bridges as estimated by the multiple-exponential analysis. Following this calculation, the structural change in the myosin heads responsible for the reversal of the working stroke can be 2 nm at maximum, suggesting that the elastic component in the cross-bridges is at least twice as rigid as previously thought.
Full text
PDF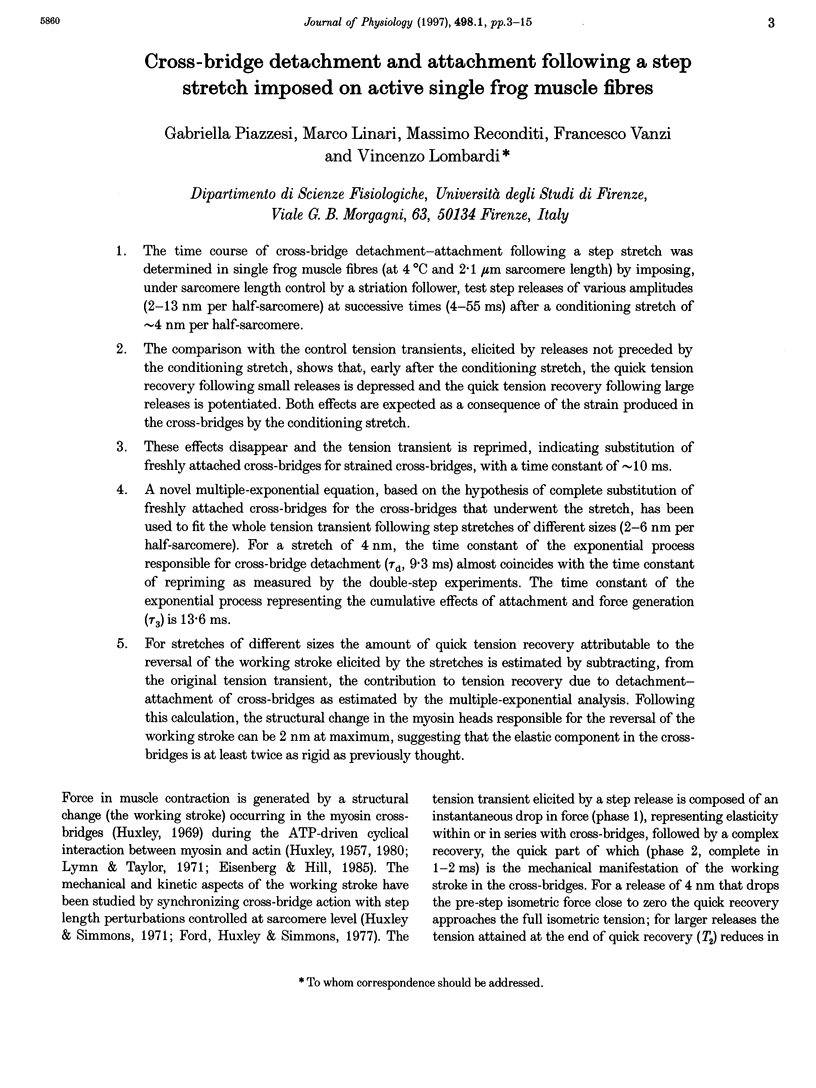
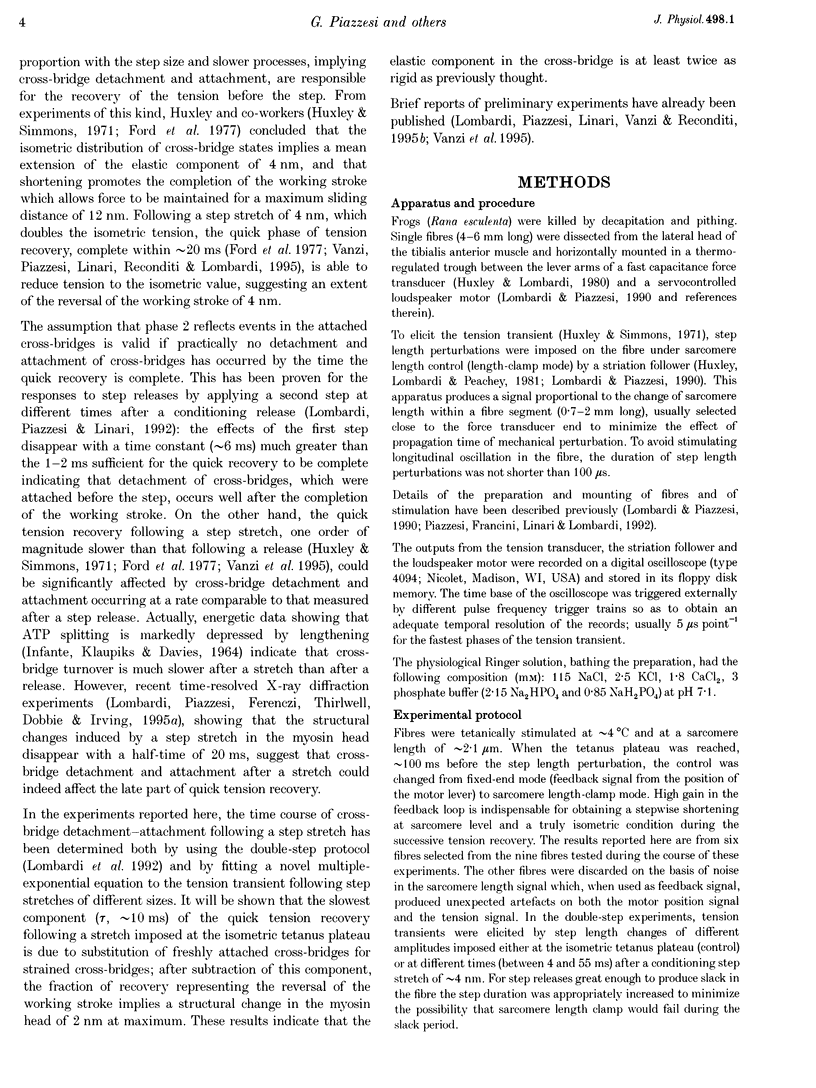
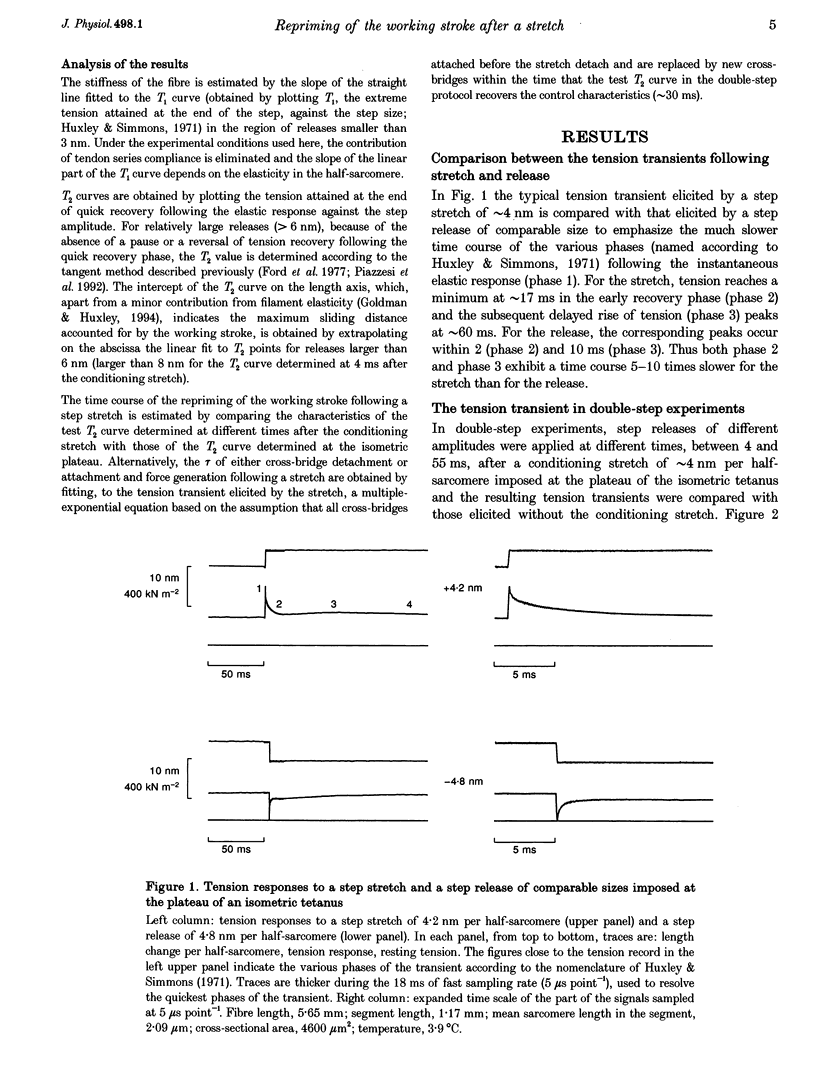
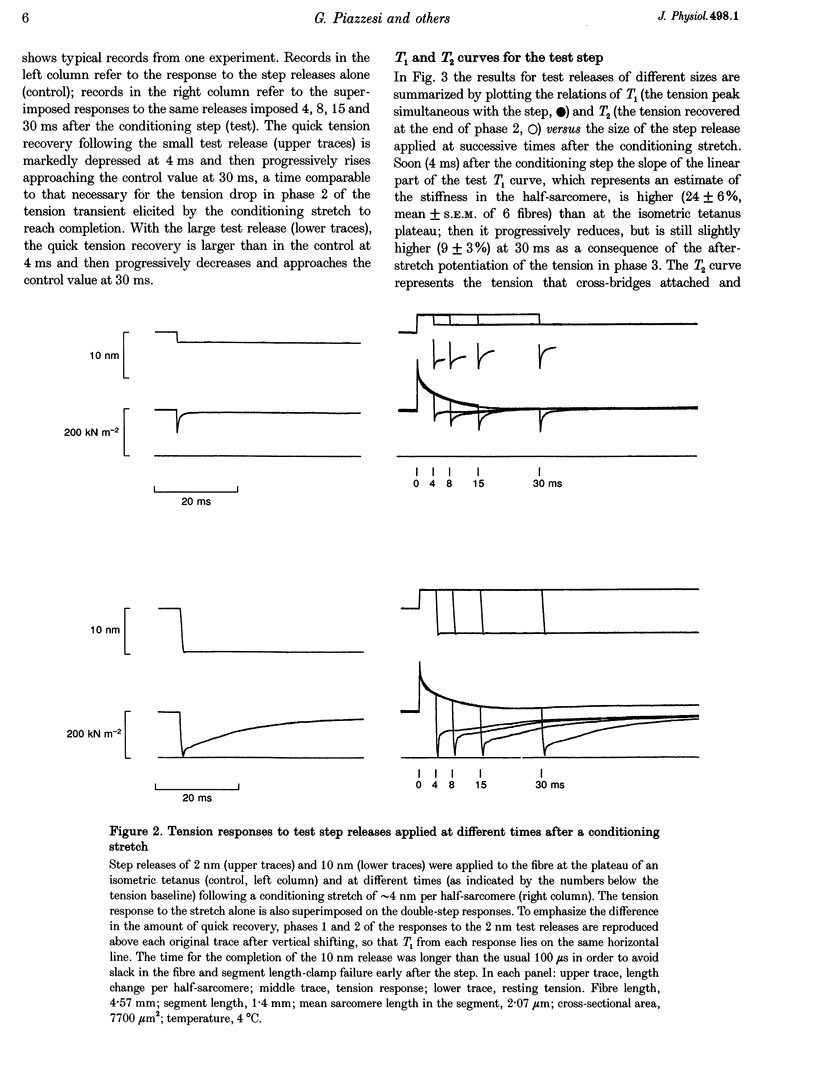
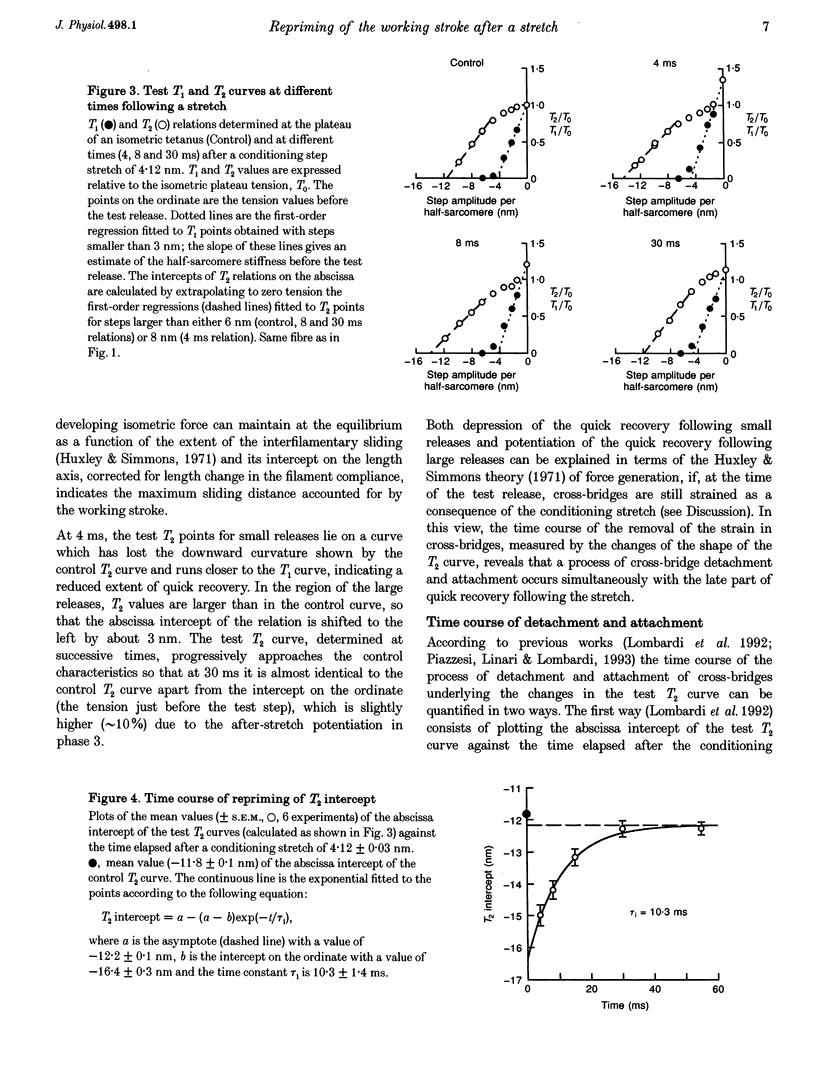
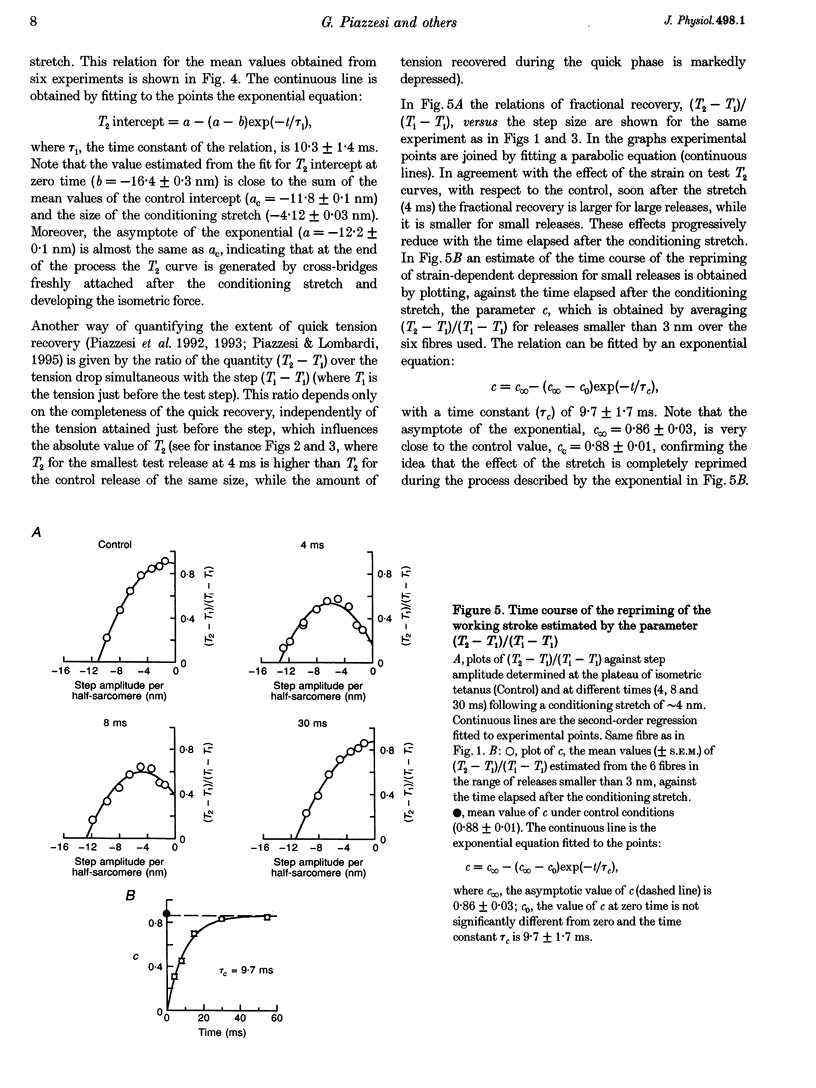
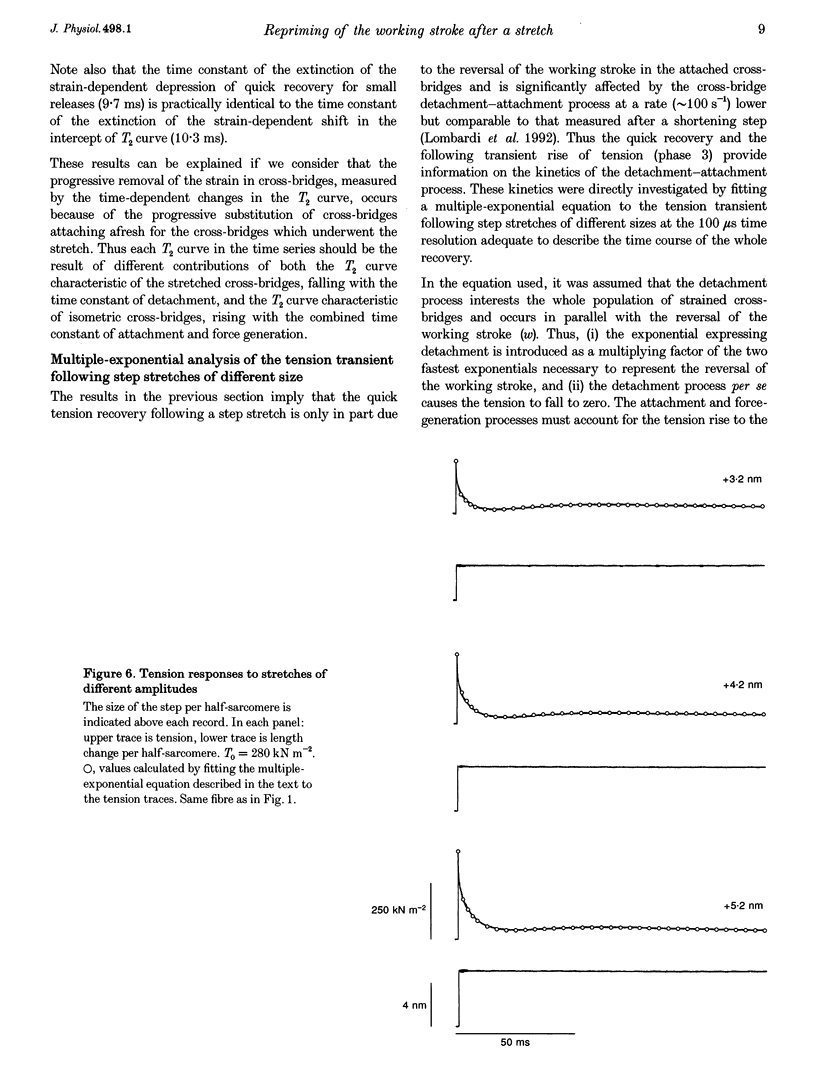
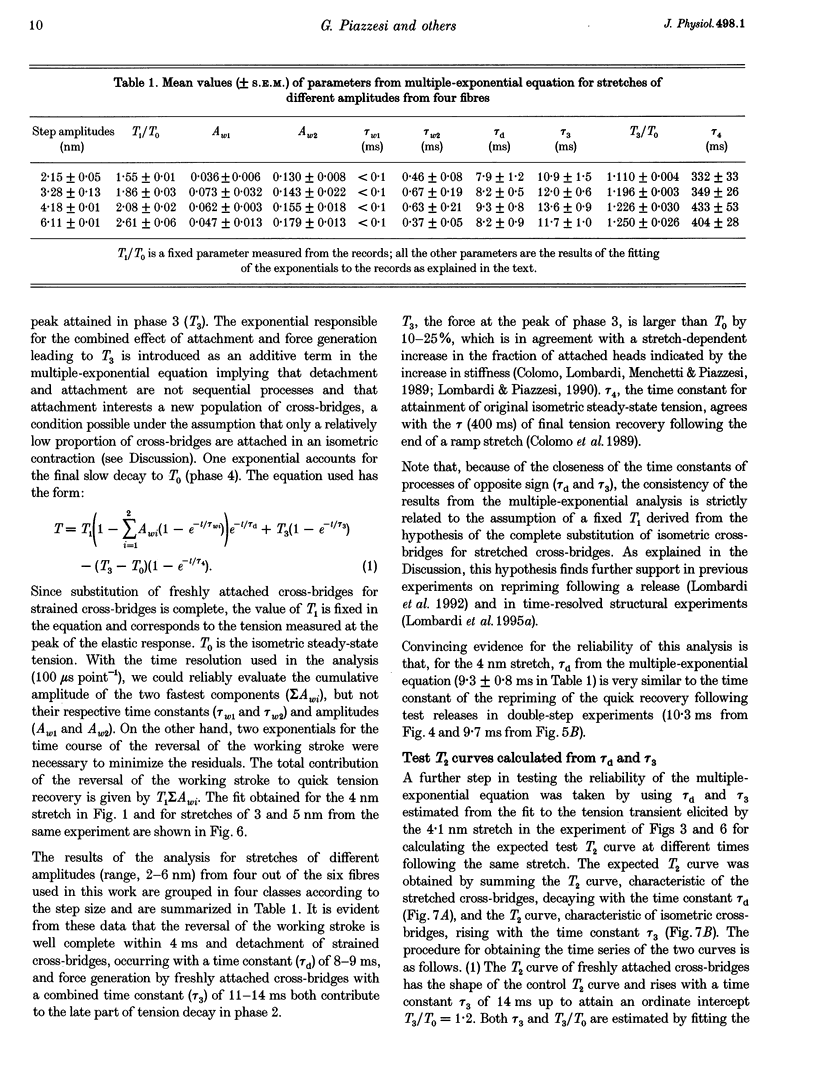
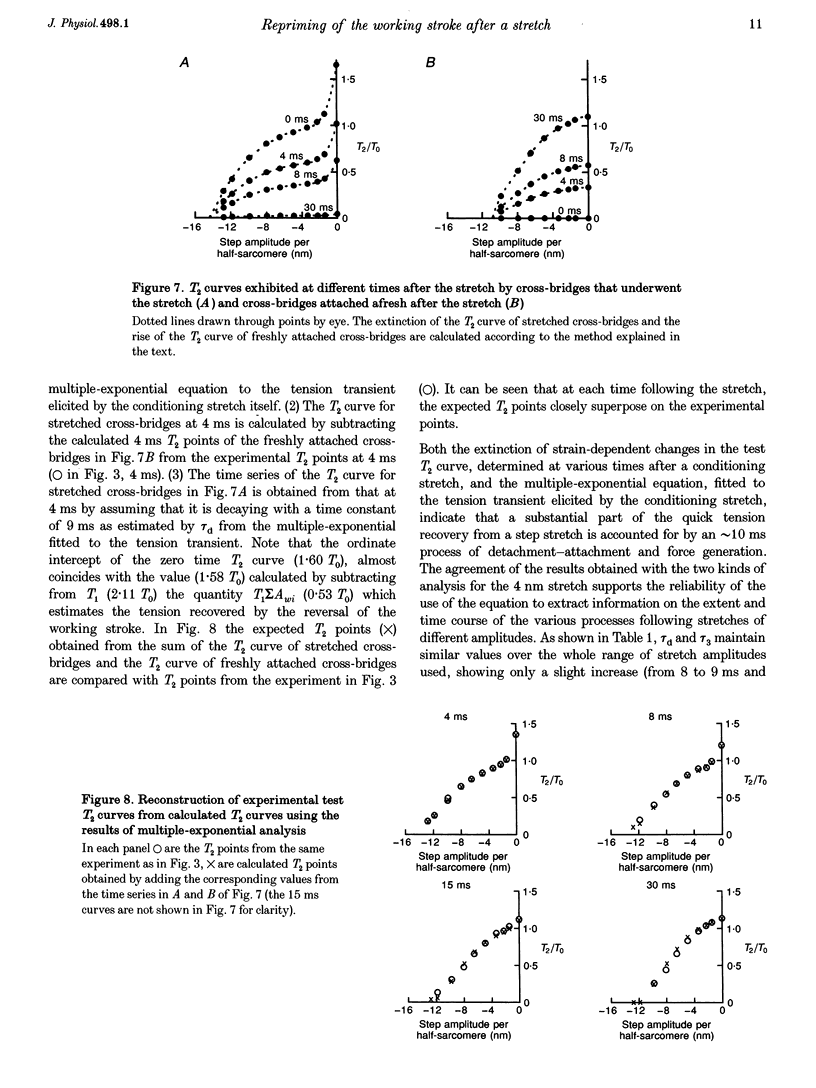
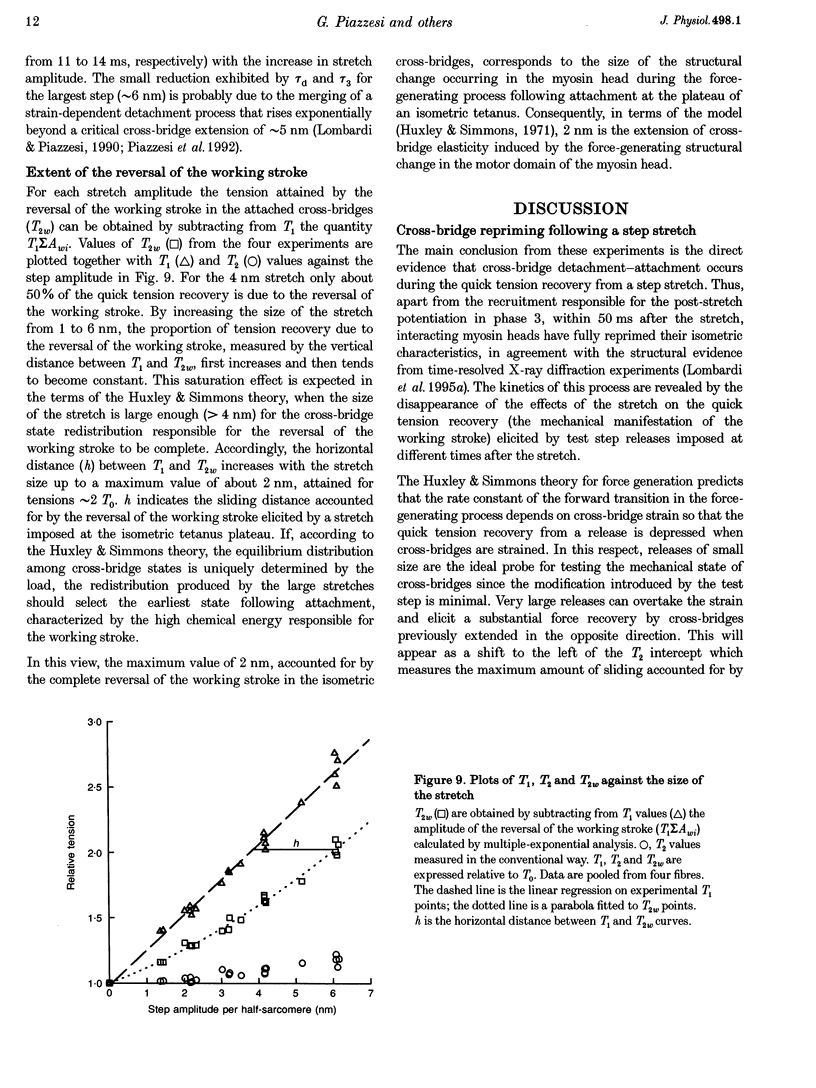
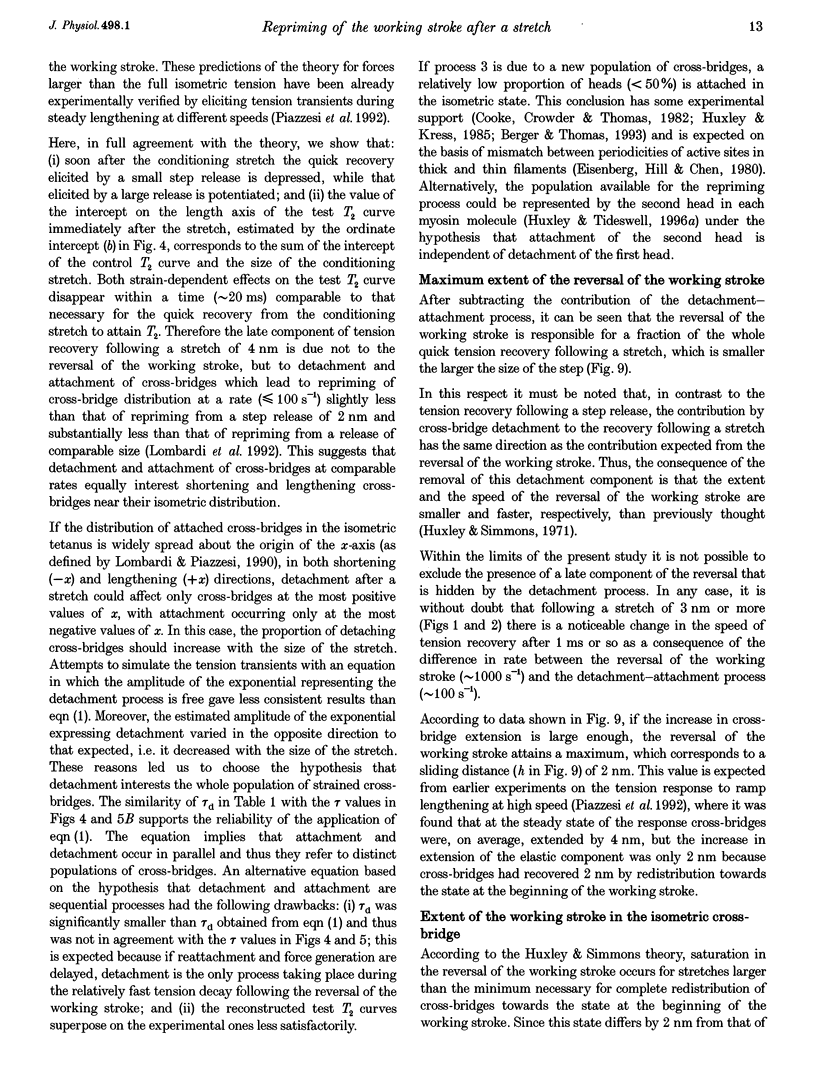
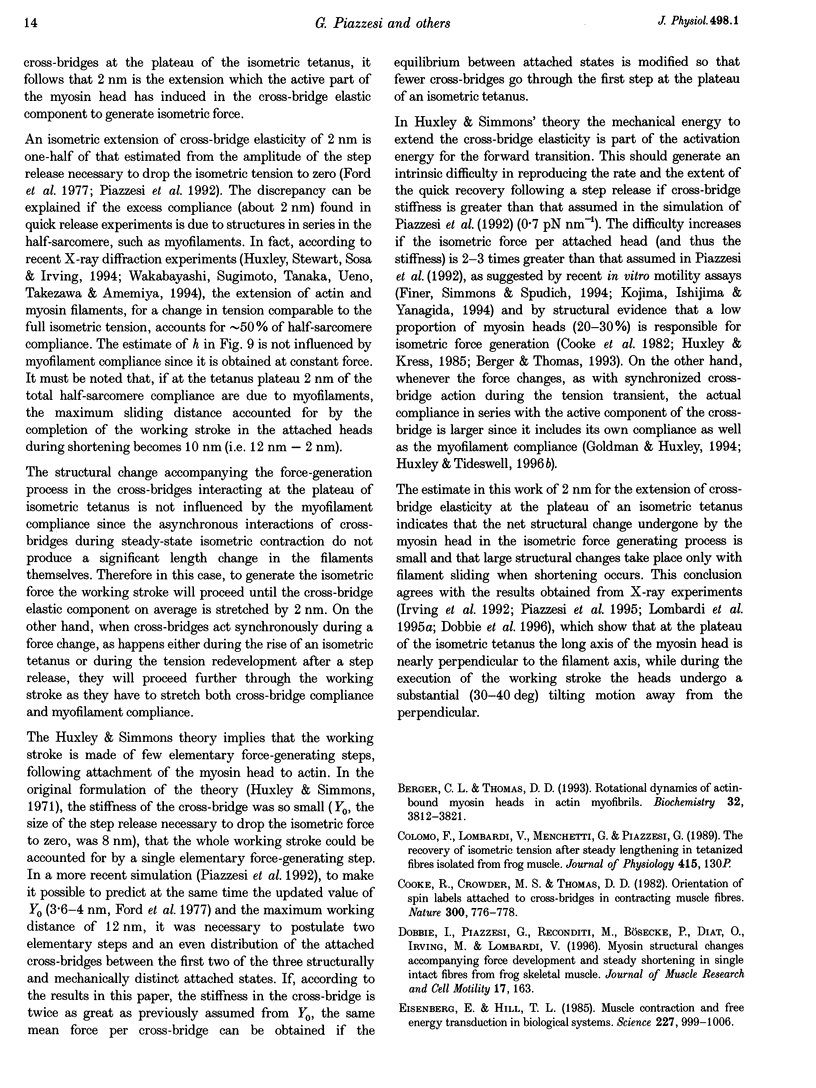
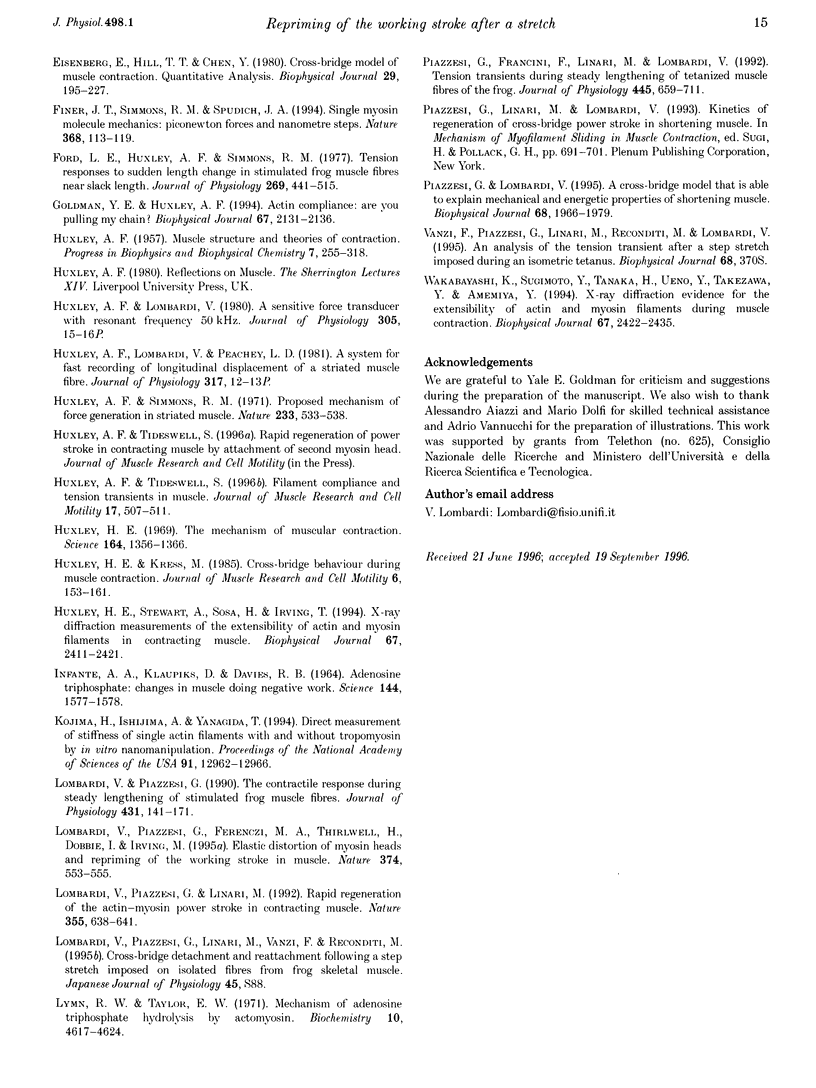
Selected References
These references are in PubMed. This may not be the complete list of references from this article.
- Berger C. L., Thomas D. D. Rotational dynamics of actin-bound myosin heads in active myofibrils. Biochemistry. 1993 Apr 13;32(14):3812–3821. doi: 10.1021/bi00065a038. [DOI] [PubMed] [Google Scholar]
- Cooke R., Crowder M. S., Thomas D. D. Orientation of spin labels attached to cross-bridges in contracting muscle fibres. Nature. 1982 Dec 23;300(5894):776–778. doi: 10.1038/300776a0. [DOI] [PubMed] [Google Scholar]
- Eisenberg E., Hill T. L., Chen Y. Cross-bridge model of muscle contraction. Quantitative analysis. Biophys J. 1980 Feb;29(2):195–227. doi: 10.1016/S0006-3495(80)85126-5. [DOI] [PMC free article] [PubMed] [Google Scholar]
- Eisenberg E., Hill T. L. Muscle contraction and free energy transduction in biological systems. Science. 1985 Mar 1;227(4690):999–1006. doi: 10.1126/science.3156404. [DOI] [PubMed] [Google Scholar]
- Finer J. T., Simmons R. M., Spudich J. A. Single myosin molecule mechanics: piconewton forces and nanometre steps. Nature. 1994 Mar 10;368(6467):113–119. doi: 10.1038/368113a0. [DOI] [PubMed] [Google Scholar]
- Ford L. E., Huxley A. F., Simmons R. M. Tension responses to sudden length change in stimulated frog muscle fibres near slack length. J Physiol. 1977 Jul;269(2):441–515. doi: 10.1113/jphysiol.1977.sp011911. [DOI] [PMC free article] [PubMed] [Google Scholar]
- Goldman Y. E., Huxley A. F. Actin compliance: are you pulling my chain? Biophys J. 1994 Dec;67(6):2131–2133. doi: 10.1016/S0006-3495(94)80700-3. [DOI] [PMC free article] [PubMed] [Google Scholar]
- HUXLEY A. F. Muscle structure and theories of contraction. Prog Biophys Biophys Chem. 1957;7:255–318. [PubMed] [Google Scholar]
- Huxley A. F., Simmons R. M. Proposed mechanism of force generation in striated muscle. Nature. 1971 Oct 22;233(5321):533–538. doi: 10.1038/233533a0. [DOI] [PubMed] [Google Scholar]
- Huxley A. F., Tideswell S. Filament compliance and tension transients in muscle. J Muscle Res Cell Motil. 1996 Aug;17(4):507–511. doi: 10.1007/BF00123366. [DOI] [PubMed] [Google Scholar]
- Huxley H. E., Kress M. Crossbridge behaviour during muscle contraction. J Muscle Res Cell Motil. 1985 Apr;6(2):153–161. doi: 10.1007/BF00713057. [DOI] [PubMed] [Google Scholar]
- Huxley H. E., Stewart A., Sosa H., Irving T. X-ray diffraction measurements of the extensibility of actin and myosin filaments in contracting muscle. Biophys J. 1994 Dec;67(6):2411–2421. doi: 10.1016/S0006-3495(94)80728-3. [DOI] [PMC free article] [PubMed] [Google Scholar]
- INFANTE A. A., KLAUPIKS D., DAVIES R. E. ADENOSINE TRIPHOSPHATE: CHANGES IN MUSCLES DOING NEGATIVE WORK. Science. 1964 Jun 26;144(3626):1577–1578. doi: 10.1126/science.144.3626.1577. [DOI] [PubMed] [Google Scholar]
- Kojima H., Ishijima A., Yanagida T. Direct measurement of stiffness of single actin filaments with and without tropomyosin by in vitro nanomanipulation. Proc Natl Acad Sci U S A. 1994 Dec 20;91(26):12962–12966. doi: 10.1073/pnas.91.26.12962. [DOI] [PMC free article] [PubMed] [Google Scholar]
- Lombardi V., Piazzesi G., Ferenczi M. A., Thirlwell H., Dobbie I., Irving M. Elastic distortion of myosin heads and repriming of the working stroke in muscle. Nature. 1995 Apr 6;374(6522):553–555. doi: 10.1038/374553a0. [DOI] [PubMed] [Google Scholar]
- Lombardi V., Piazzesi G., Linari M. Rapid regeneration of the actin-myosin power stroke in contracting muscle. Nature. 1992 Feb 13;355(6361):638–641. doi: 10.1038/355638a0. [DOI] [PubMed] [Google Scholar]
- Lombardi V., Piazzesi G. The contractile response during steady lengthening of stimulated frog muscle fibres. J Physiol. 1990 Dec;431:141–171. doi: 10.1113/jphysiol.1990.sp018324. [DOI] [PMC free article] [PubMed] [Google Scholar]
- Piazzesi G., Francini F., Linari M., Lombardi V. Tension transients during steady lengthening of tetanized muscle fibres of the frog. J Physiol. 1992 Jan;445:659–711. doi: 10.1113/jphysiol.1992.sp018945. [DOI] [PMC free article] [PubMed] [Google Scholar]
- Piazzesi G., Linari M., Lombardi V. Kinetics of regeneration of cross-bridge power stroke in shortening muscle. Adv Exp Med Biol. 1993;332:691–701. doi: 10.1007/978-1-4615-2872-2_61. [DOI] [PubMed] [Google Scholar]
- Piazzesi G., Lombardi V. A cross-bridge model that is able to explain mechanical and energetic properties of shortening muscle. Biophys J. 1995 May;68(5):1966–1979. doi: 10.1016/S0006-3495(95)80374-7. [DOI] [PMC free article] [PubMed] [Google Scholar]
- Wakabayashi K., Sugimoto Y., Tanaka H., Ueno Y., Takezawa Y., Amemiya Y. X-ray diffraction evidence for the extensibility of actin and myosin filaments during muscle contraction. Biophys J. 1994 Dec;67(6):2422–2435. doi: 10.1016/S0006-3495(94)80729-5. [DOI] [PMC free article] [PubMed] [Google Scholar]