Abstract
1. In cultures of rat forebrain neurones, mitochondria buffer glutamate-induced, NMDA receptor-mediated Ca2+ influx. Here, we have used the fluorescent calcium indicator, indo-1 AM to record [Ca2+]i from single cells. We varied either the glutamate concentration or the duration of exposure to investigate the cellular mechanisms recruited to buffer [Ca2+]i within different stimulation protocols. 2. For a 15 s stimulus, the recovery time doubled as the glutamate concentration was raised from 3 to 300 microM. Changing the duration of exposure from 15 s to 5 min increased the recovery time tenfold even when the glutamate concentration was held at 3 microM. 3. We used a selective inhibitor of the mitochondrial Na(+)-Ca2+ exchange, CGP-37157. When applied immediately after a 15 s, 100 microM glutamate challenge, CGP-37157 consistently caused a rapid fall in [Ca2+]i followed by a slow rise after the drug was washed out. A similar pattern was seen with the 5 min, 3 microM glutamate stimulus. The effects of CGP-37157 are consistent with the release of substantial mitochondrial Ca2+ stores during recovery from an intense glutamate stimulus. 4. These studies suggest that mitochondria become progressively more important for buffering glutamate-induced Ca2+ loads as the stimulus intensity increases. The recovery of [Ca2+]i to baseline following glutamate removal is critically regulated by the release of Ca2+ from mitochondrial stores via mitochondrial Na(+)-Ca2+ exchange. The data highlight a previously under-appreciated role for [Na+]i in the regulation of [Ca2+]i in central neurones.
Full text
PDF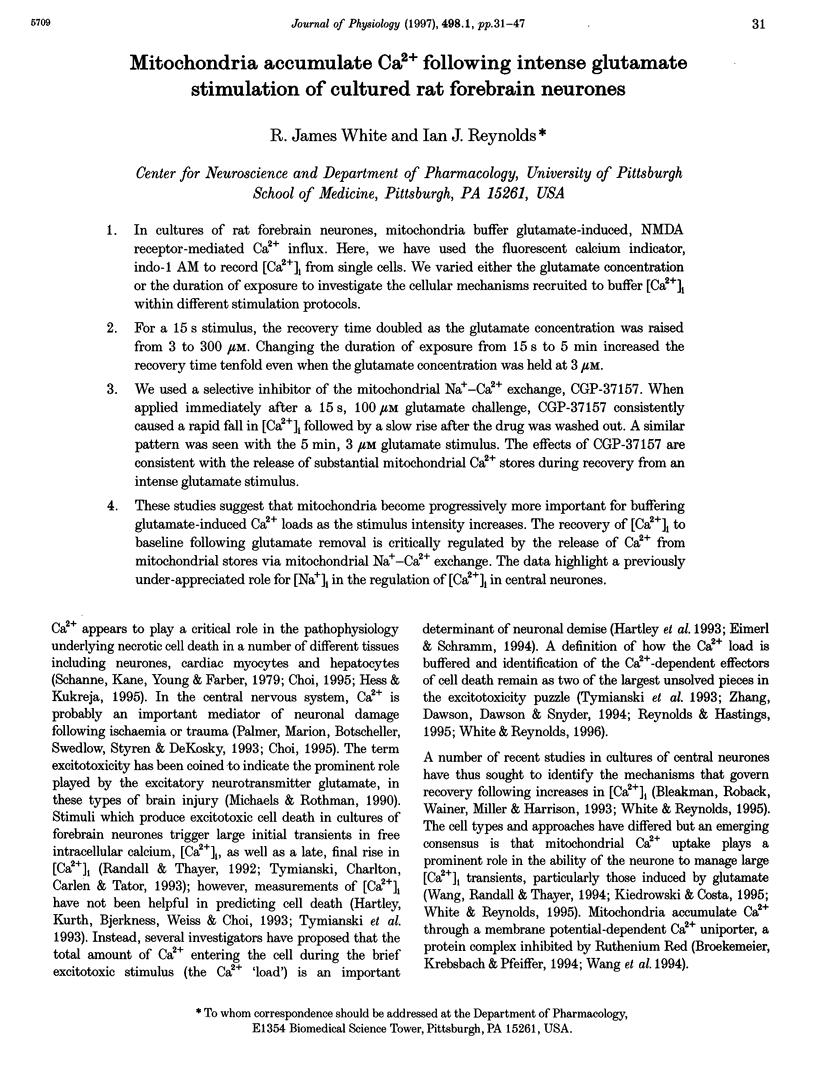
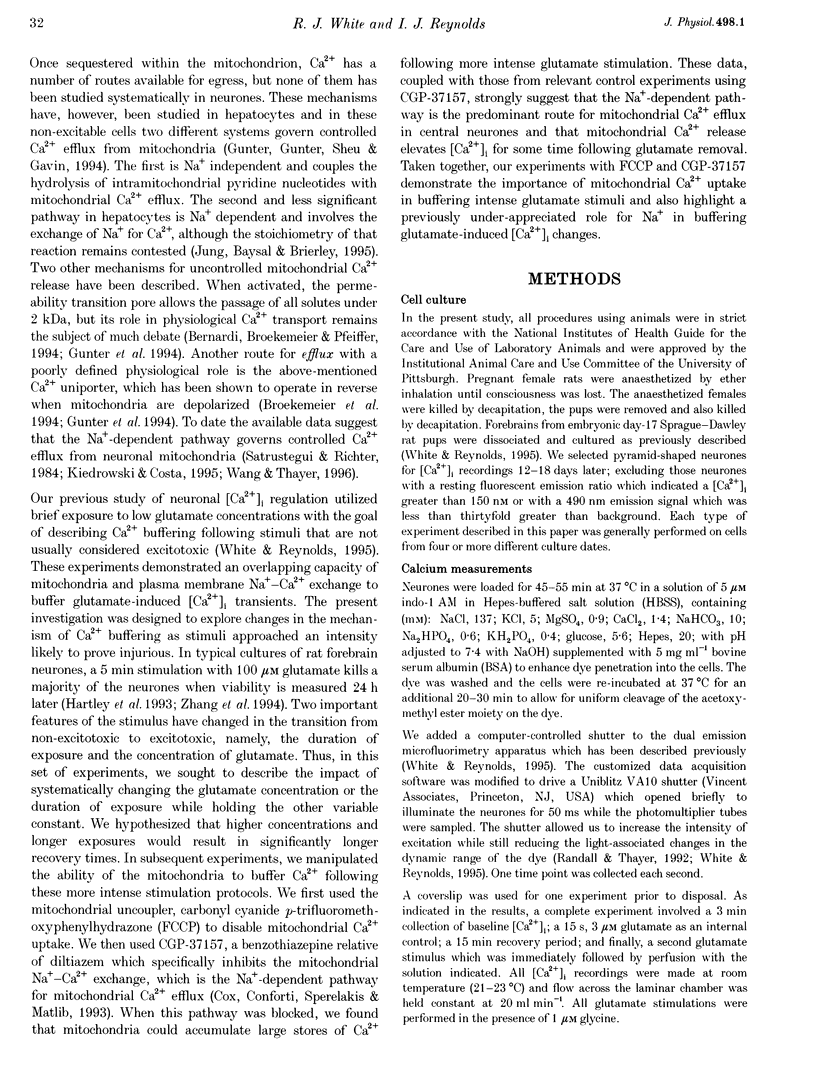
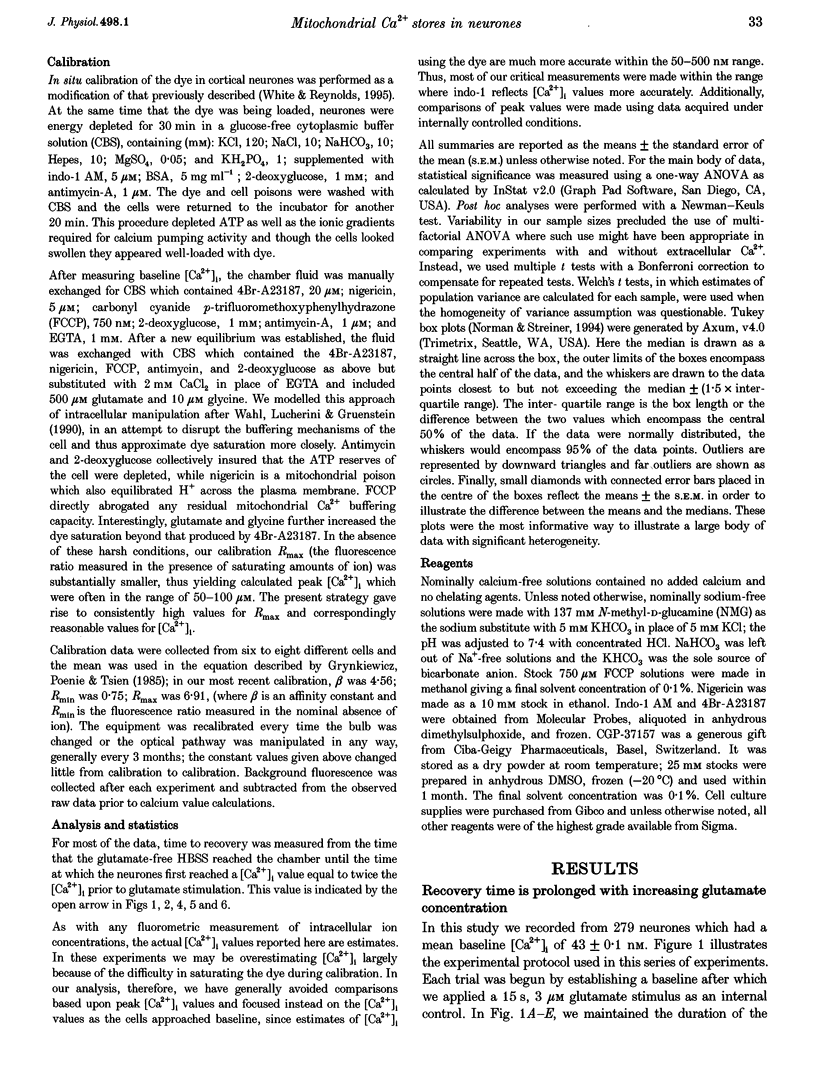
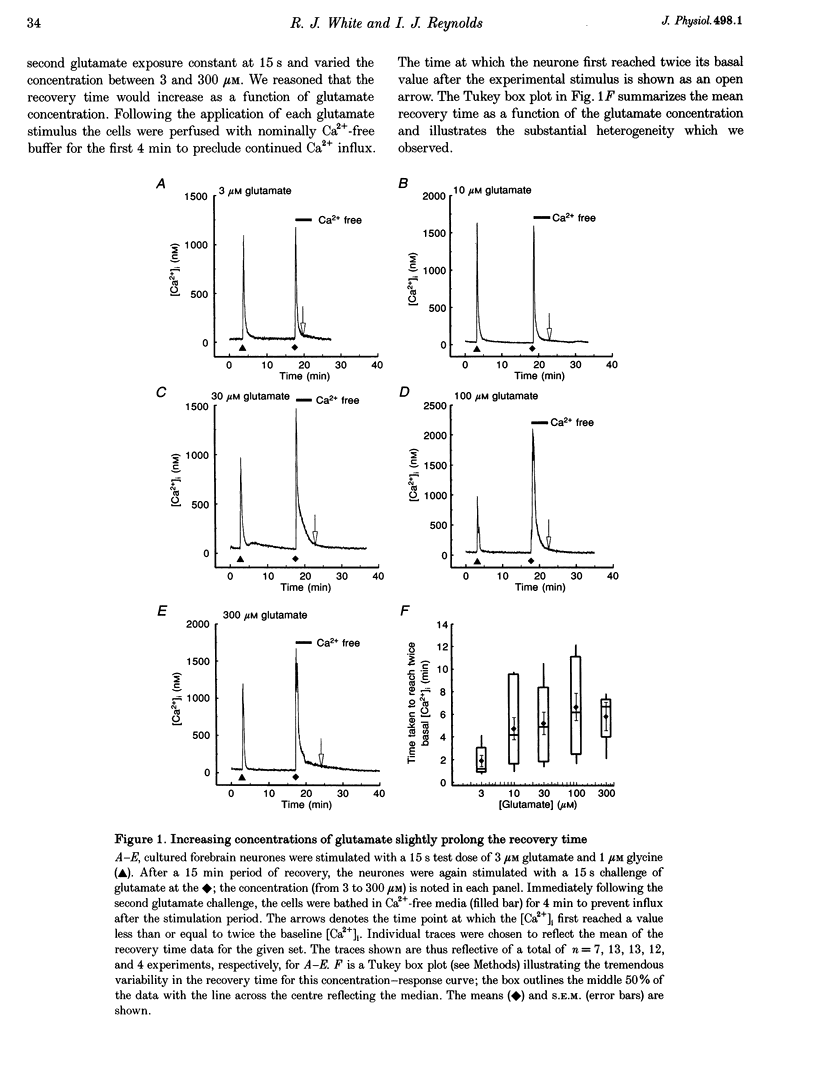
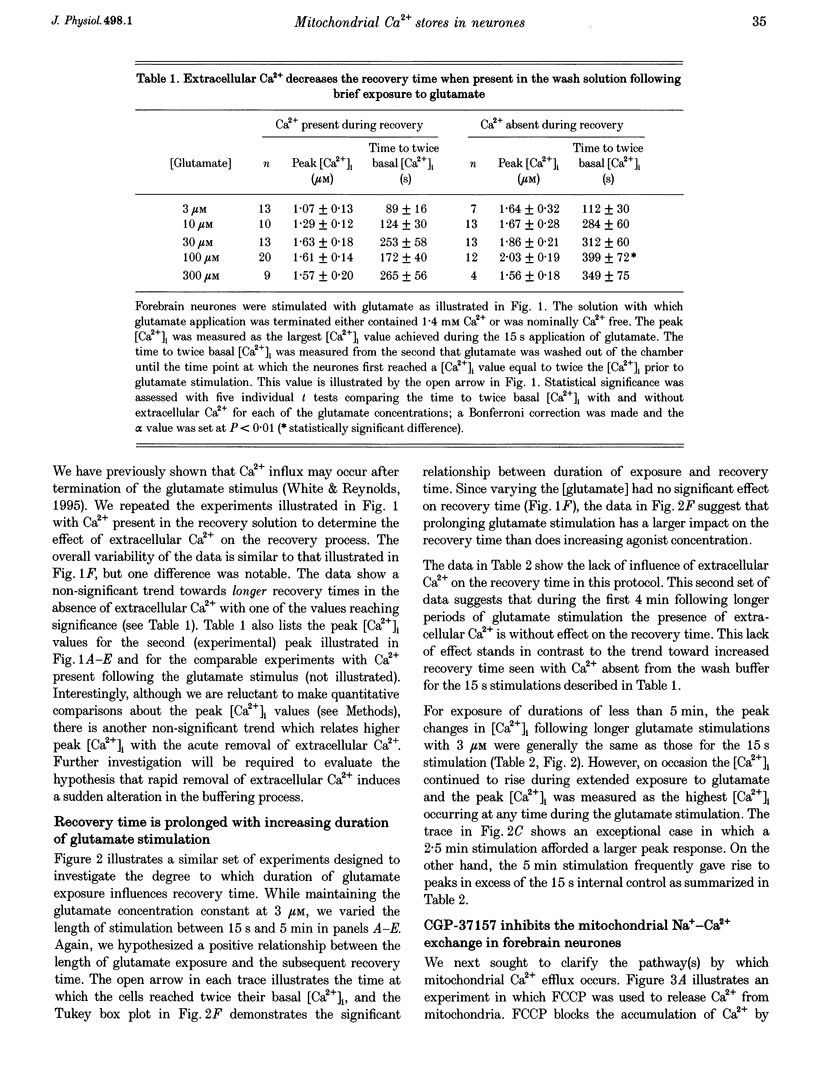
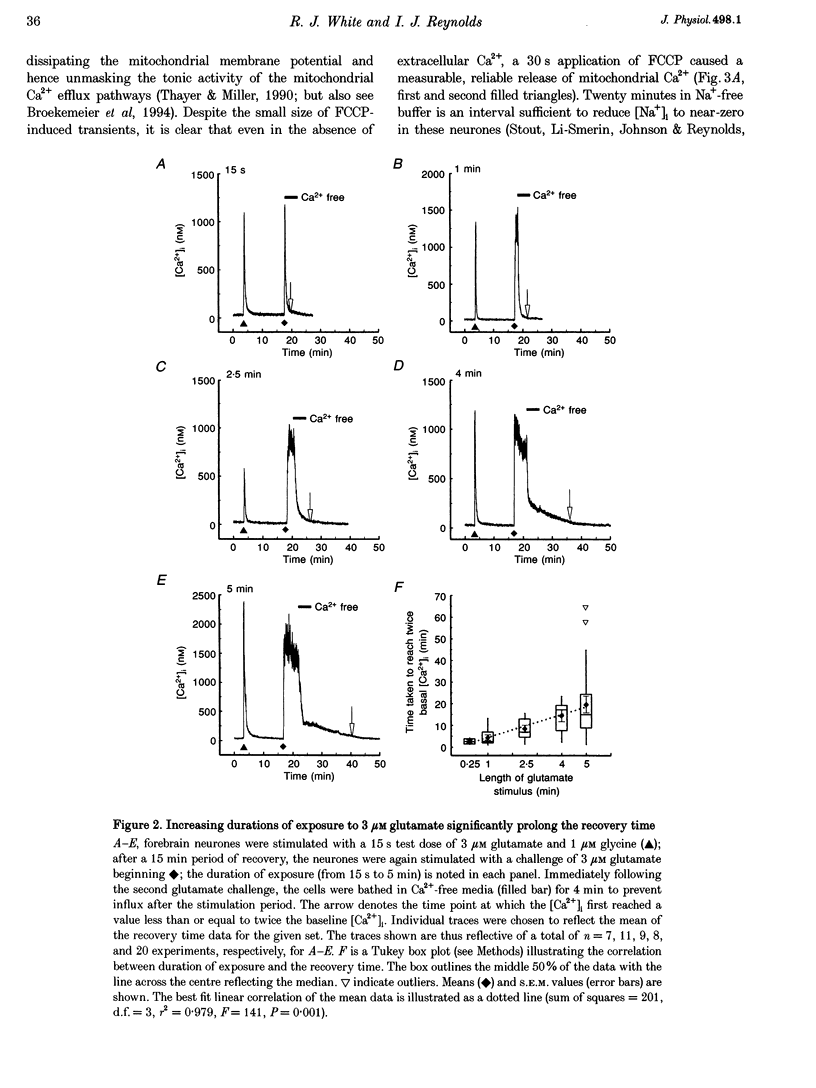
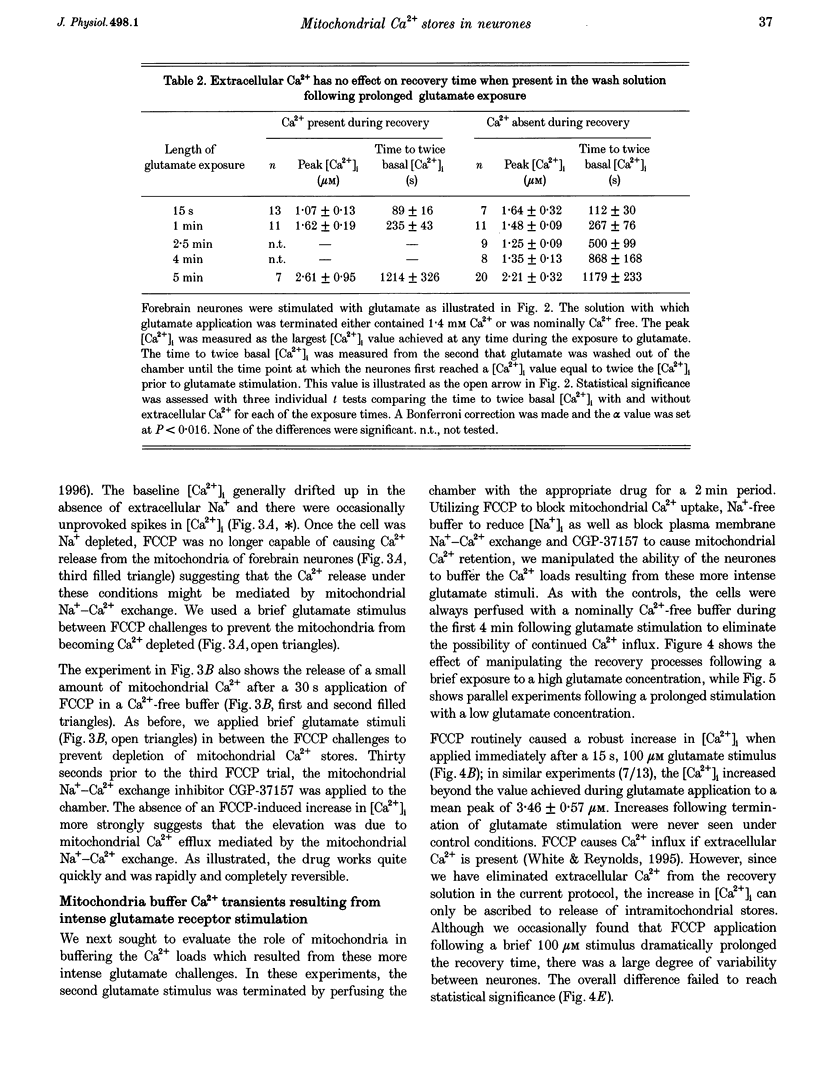
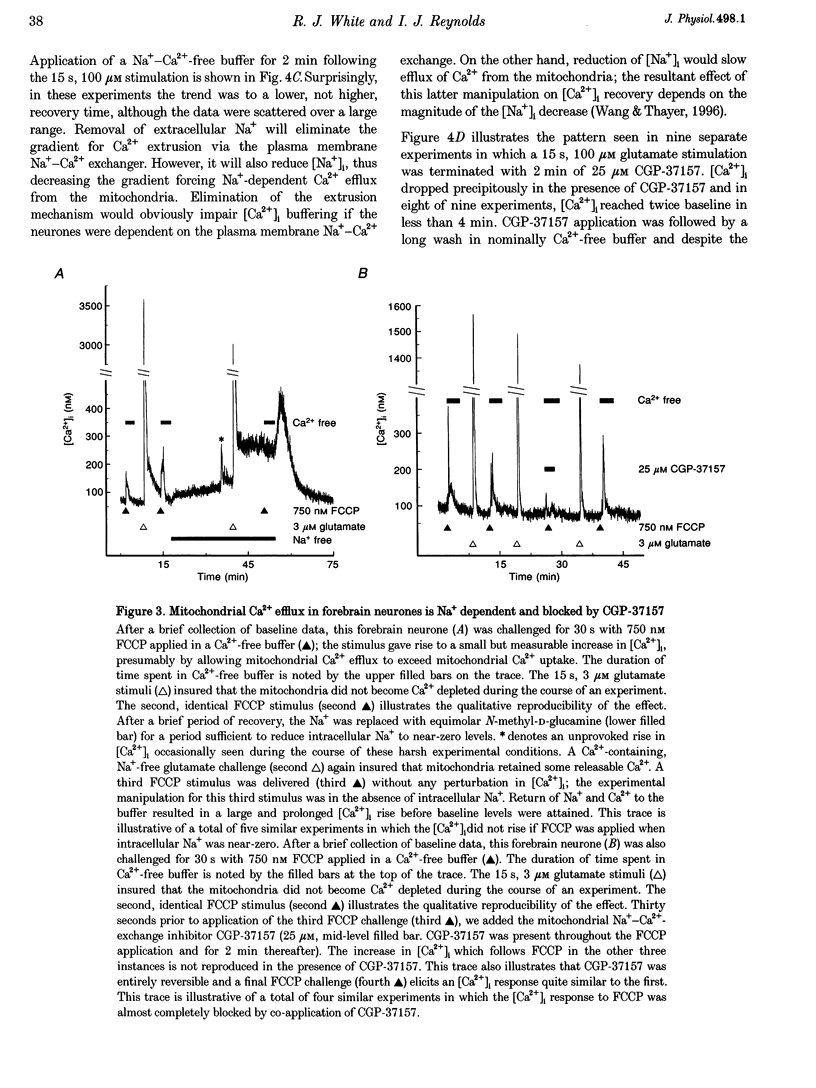
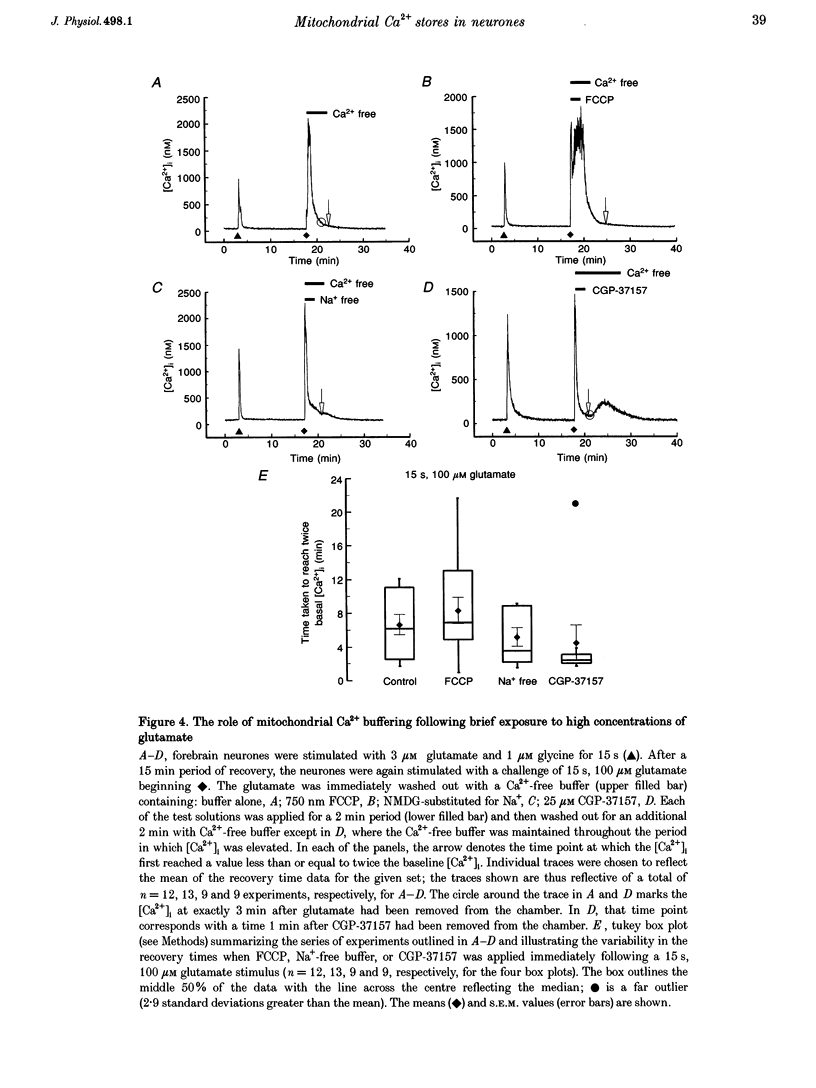
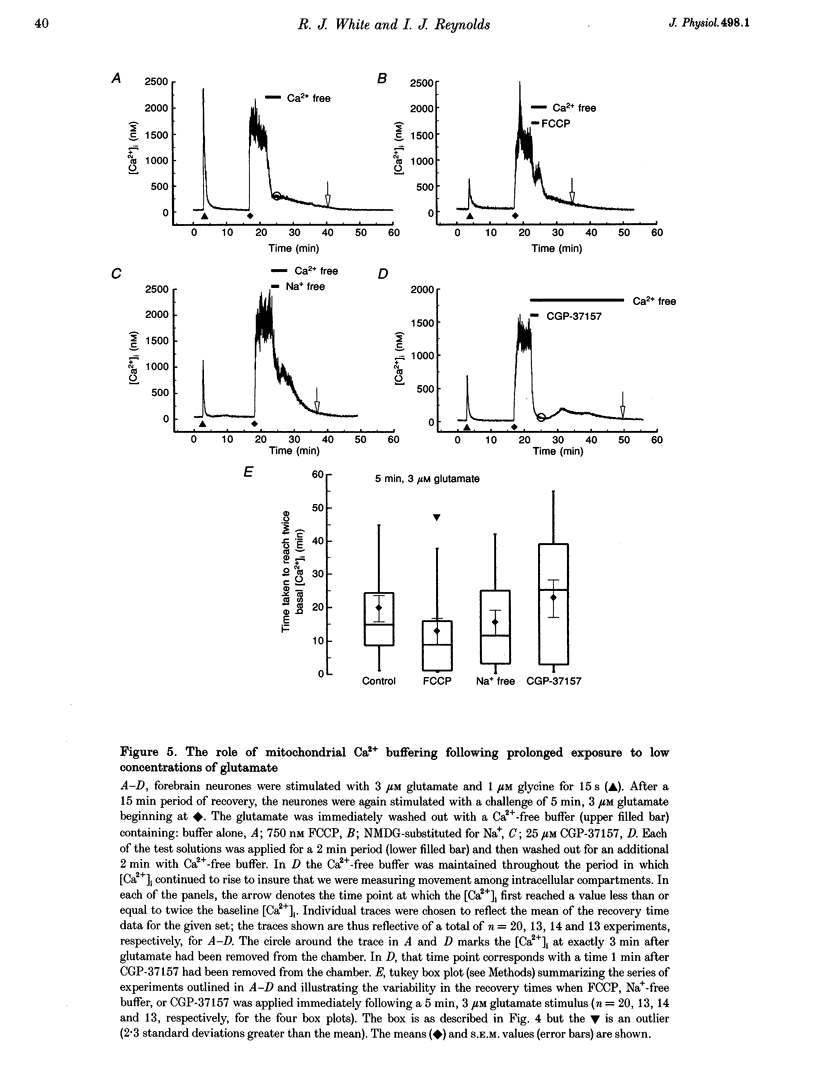
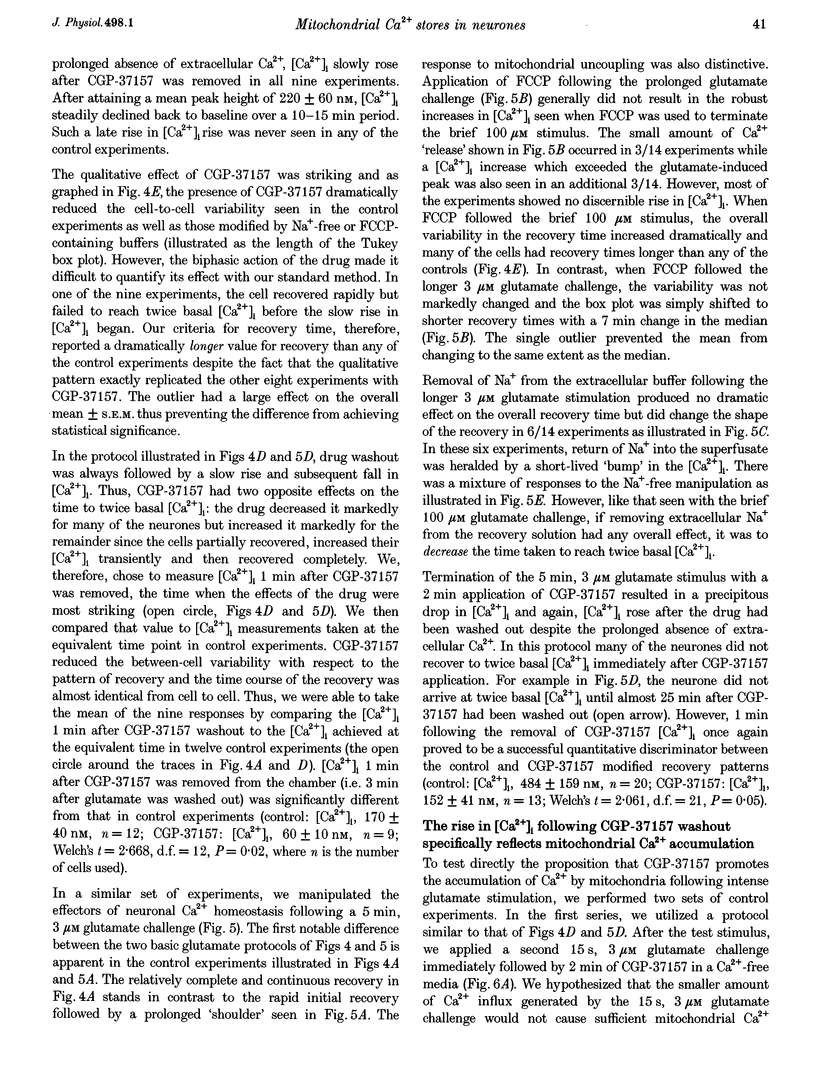
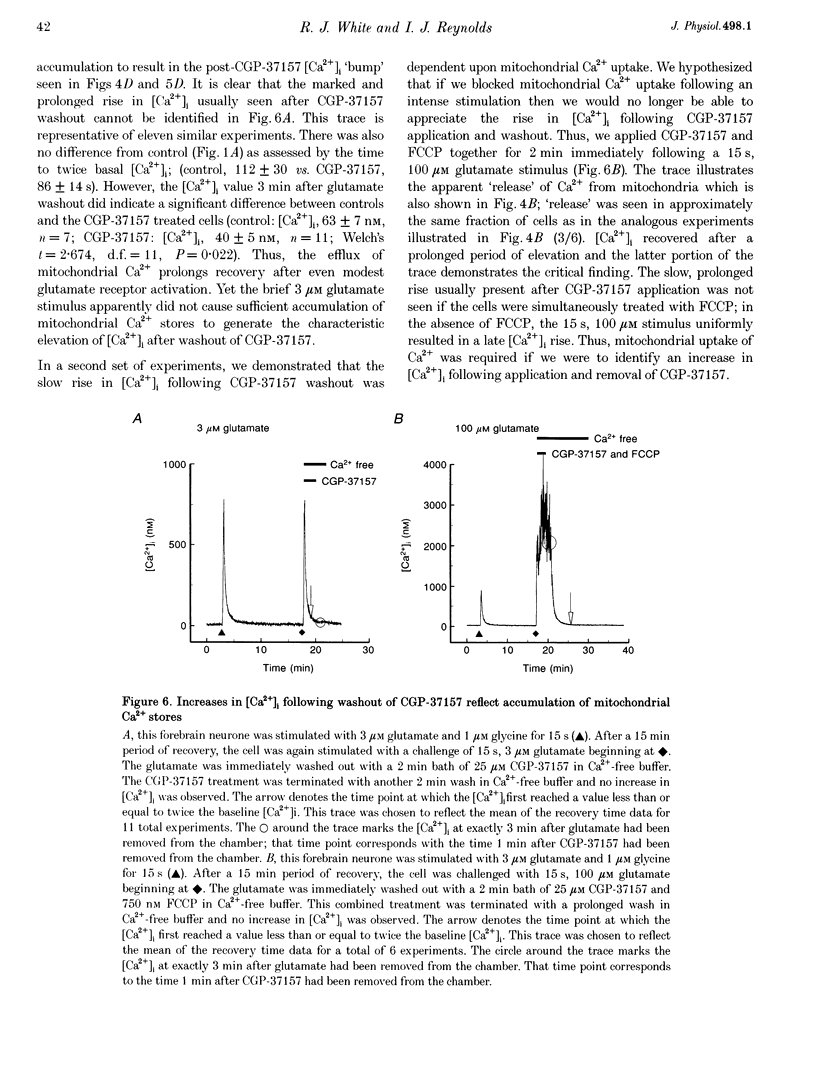
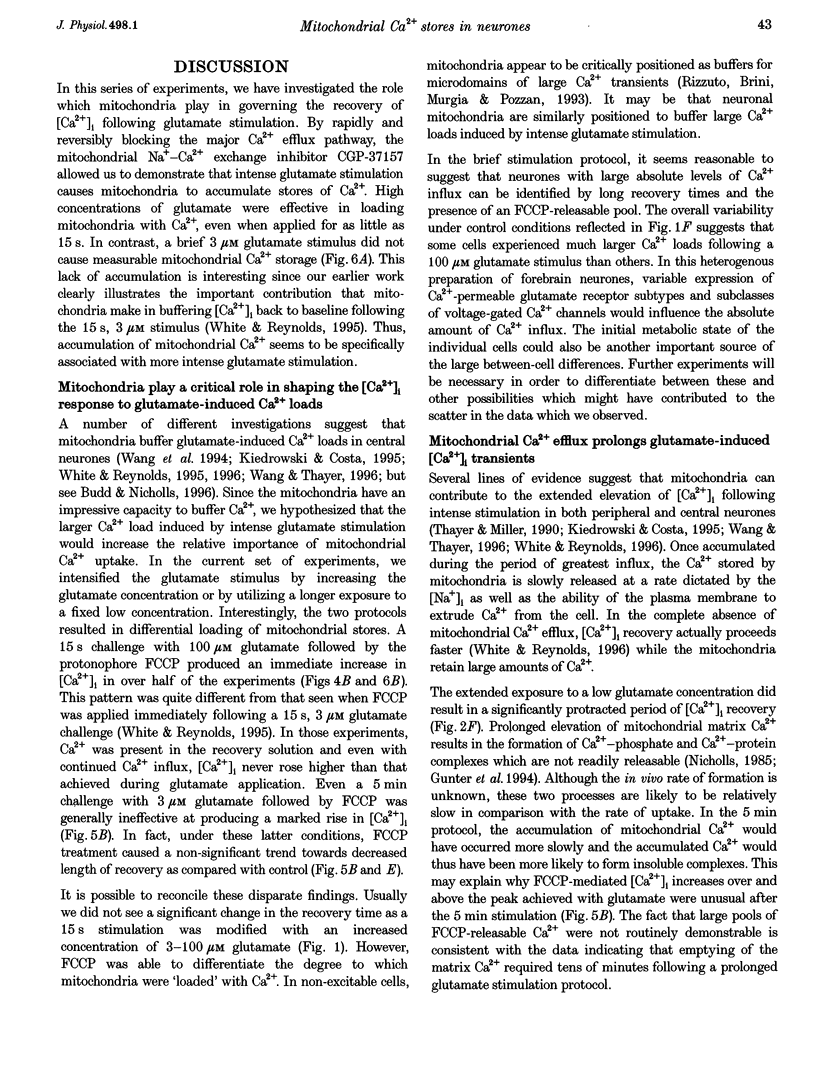
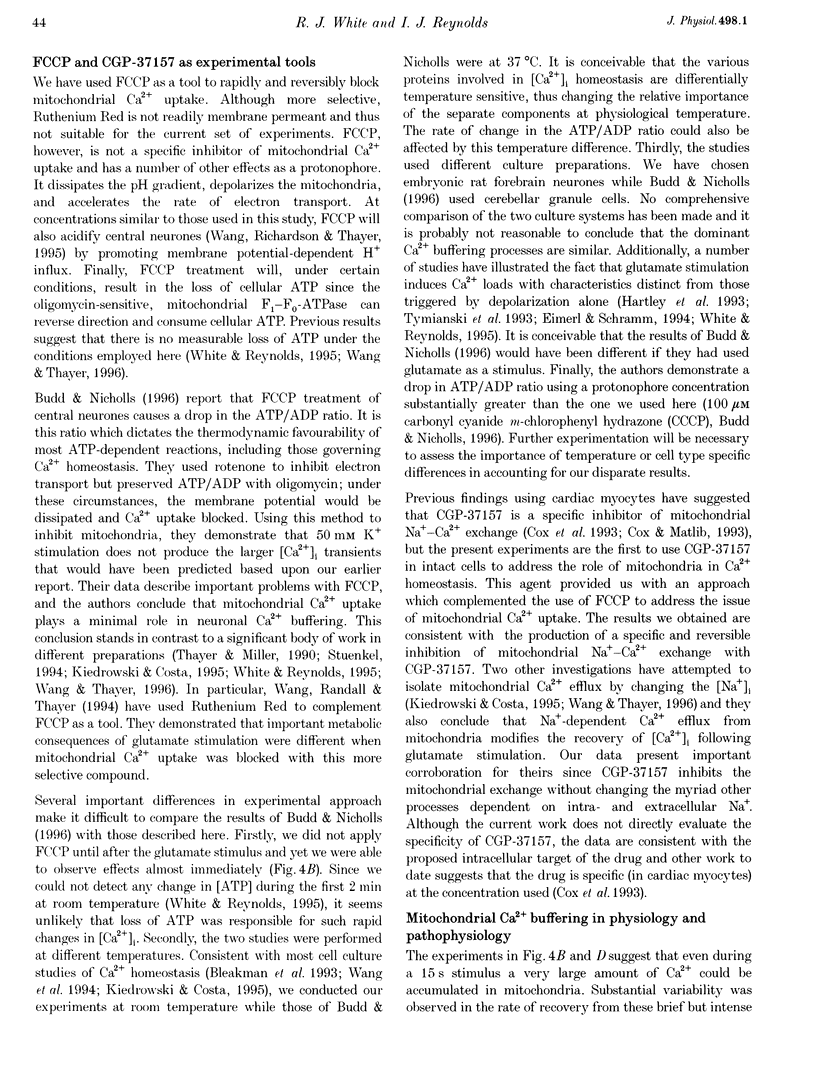
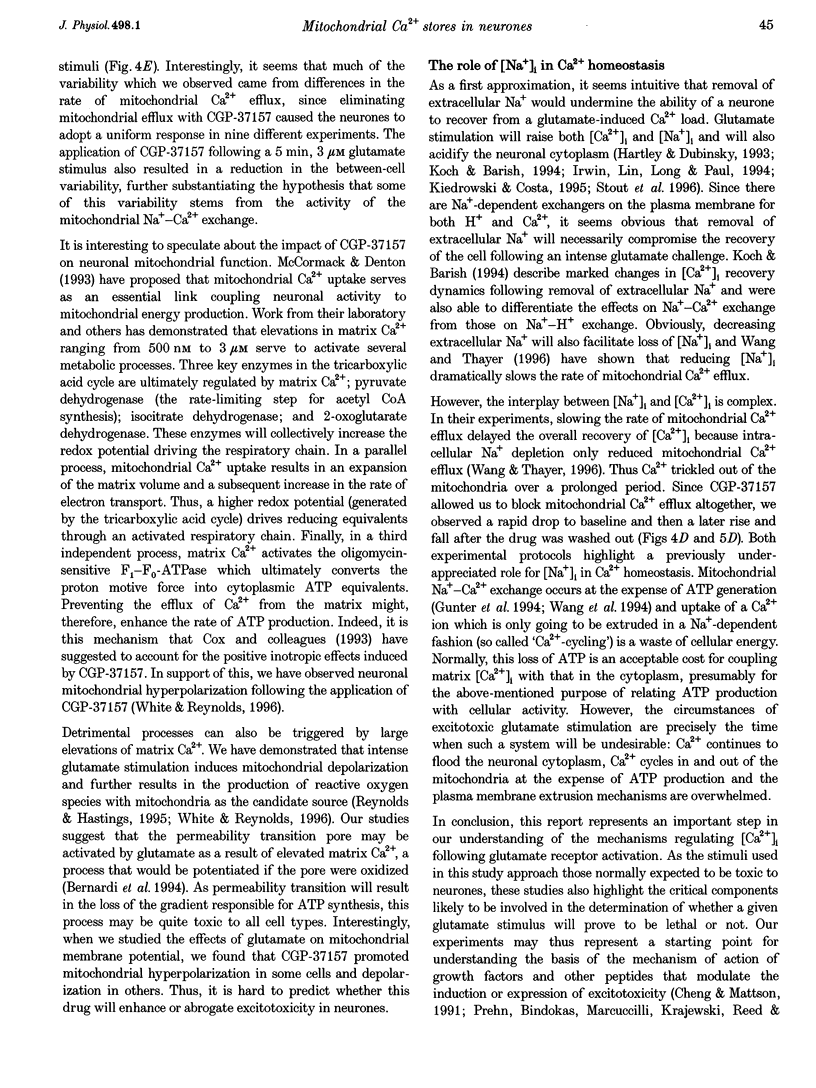
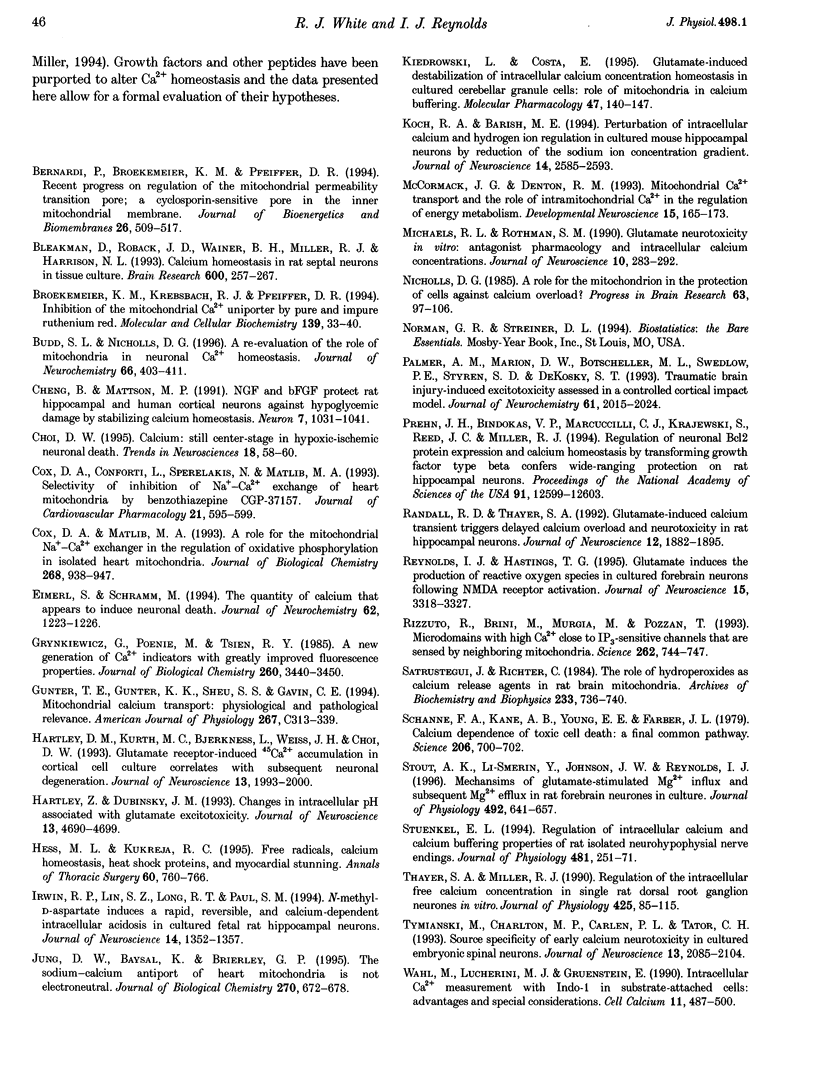
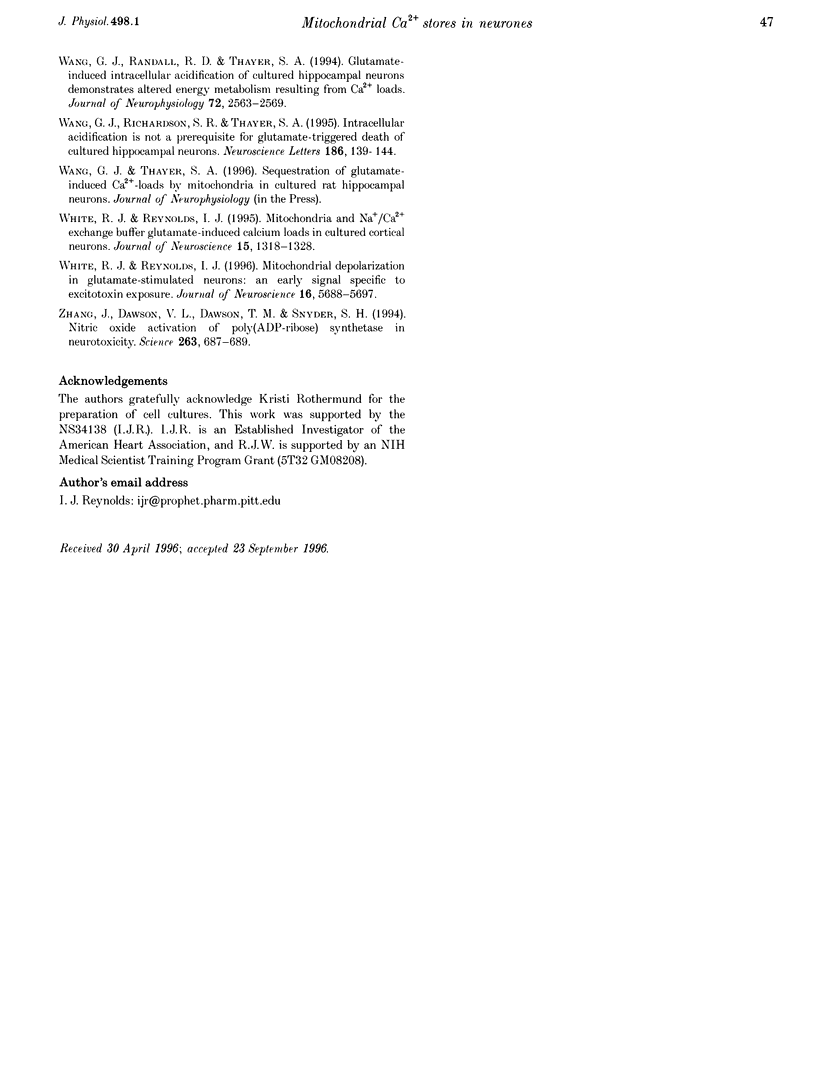
Selected References
These references are in PubMed. This may not be the complete list of references from this article.
- Bernardi P., Broekemeier K. M., Pfeiffer D. R. Recent progress on regulation of the mitochondrial permeability transition pore; a cyclosporin-sensitive pore in the inner mitochondrial membrane. J Bioenerg Biomembr. 1994 Oct;26(5):509–517. doi: 10.1007/BF00762735. [DOI] [PubMed] [Google Scholar]
- Bleakman D., Roback J. D., Wainer B. H., Miller R. J., Harrison N. L. Calcium homeostasis in rat septal neurons in tissue culture. Brain Res. 1993 Jan 15;600(2):257–267. doi: 10.1016/0006-8993(93)91381-2. [DOI] [PubMed] [Google Scholar]
- Broekemeier K. M., Krebsbach R. J., Pfeiffer D. R. Inhibition of the mitochondrial Ca2+ uniporter by pure and impure ruthenium red. Mol Cell Biochem. 1994 Oct 12;139(1):33–40. doi: 10.1007/BF00944201. [DOI] [PubMed] [Google Scholar]
- Budd S. L., Nicholls D. G. A reevaluation of the role of mitochondria in neuronal Ca2+ homeostasis. J Neurochem. 1996 Jan;66(1):403–411. doi: 10.1046/j.1471-4159.1996.66010403.x. [DOI] [PubMed] [Google Scholar]
- Cheng B., Mattson M. P. NGF and bFGF protect rat hippocampal and human cortical neurons against hypoglycemic damage by stabilizing calcium homeostasis. Neuron. 1991 Dec;7(6):1031–1041. doi: 10.1016/0896-6273(91)90347-3. [DOI] [PubMed] [Google Scholar]
- Choi D. W. Calcium: still center-stage in hypoxic-ischemic neuronal death. Trends Neurosci. 1995 Feb;18(2):58–60. [PubMed] [Google Scholar]
- Cox D. A., Conforti L., Sperelakis N., Matlib M. A. Selectivity of inhibition of Na(+)-Ca2+ exchange of heart mitochondria by benzothiazepine CGP-37157. J Cardiovasc Pharmacol. 1993 Apr;21(4):595–599. doi: 10.1097/00005344-199304000-00013. [DOI] [PubMed] [Google Scholar]
- Cox D. A., Matlib M. A. A role for the mitochondrial Na(+)-Ca2+ exchanger in the regulation of oxidative phosphorylation in isolated heart mitochondria. J Biol Chem. 1993 Jan 15;268(2):938–947. [PubMed] [Google Scholar]
- Eimerl S., Schramm M. The quantity of calcium that appears to induce neuronal death. J Neurochem. 1994 Mar;62(3):1223–1226. doi: 10.1046/j.1471-4159.1994.62031223.x. [DOI] [PubMed] [Google Scholar]
- Grynkiewicz G., Poenie M., Tsien R. Y. A new generation of Ca2+ indicators with greatly improved fluorescence properties. J Biol Chem. 1985 Mar 25;260(6):3440–3450. [PubMed] [Google Scholar]
- Gunter T. E., Gunter K. K., Sheu S. S., Gavin C. E. Mitochondrial calcium transport: physiological and pathological relevance. Am J Physiol. 1994 Aug;267(2 Pt 1):C313–C339. doi: 10.1152/ajpcell.1994.267.2.C313. [DOI] [PubMed] [Google Scholar]
- Hartley D. M., Kurth M. C., Bjerkness L., Weiss J. H., Choi D. W. Glutamate receptor-induced 45Ca2+ accumulation in cortical cell culture correlates with subsequent neuronal degeneration. J Neurosci. 1993 May;13(5):1993–2000. doi: 10.1523/JNEUROSCI.13-05-01993.1993. [DOI] [PMC free article] [PubMed] [Google Scholar]
- Hartley Z., Dubinsky J. M. Changes in intracellular pH associated with glutamate excitotoxicity. J Neurosci. 1993 Nov;13(11):4690–4699. doi: 10.1523/JNEUROSCI.13-11-04690.1993. [DOI] [PMC free article] [PubMed] [Google Scholar]
- Hess M. L., Kukreja R. C. Free radicals, calcium homeostasis, heat shock proteins, and myocardial stunning. Ann Thorac Surg. 1995 Sep;60(3):760–766. doi: 10.1016/0003-4975(95)00574-5. [DOI] [PubMed] [Google Scholar]
- Irwin R. P., Lin S. Z., Long R. T., Paul S. M. N-methyl-D-aspartate induces a rapid, reversible, and calcium-dependent intracellular acidosis in cultured fetal rat hippocampal neurons. J Neurosci. 1994 Mar;14(3 Pt 1):1352–1357. doi: 10.1523/JNEUROSCI.14-03-01352.1994. [DOI] [PMC free article] [PubMed] [Google Scholar]
- Jung D. W., Baysal K., Brierley G. P. The sodium-calcium antiport of heart mitochondria is not electroneutral. J Biol Chem. 1995 Jan 13;270(2):672–678. doi: 10.1074/jbc.270.2.672. [DOI] [PubMed] [Google Scholar]
- Kiedrowski L., Costa E. Glutamate-induced destabilization of intracellular calcium concentration homeostasis in cultured cerebellar granule cells: role of mitochondria in calcium buffering. Mol Pharmacol. 1995 Jan;47(1):140–147. [PubMed] [Google Scholar]
- Koch R. A., Barish M. E. Perturbation of intracellular calcium and hydrogen ion regulation in cultured mouse hippocampal neurons by reduction of the sodium ion concentration gradient. J Neurosci. 1994 May;14(5 Pt 1):2585–2593. doi: 10.1523/JNEUROSCI.14-05-02585.1994. [DOI] [PMC free article] [PubMed] [Google Scholar]
- McCormack J. G., Denton R. M. Mitochondrial Ca2+ transport and the role of intramitochondrial Ca2+ in the regulation of energy metabolism. Dev Neurosci. 1993;15(3-5):165–173. doi: 10.1159/000111332. [DOI] [PubMed] [Google Scholar]
- Michaels R. L., Rothman S. M. Glutamate neurotoxicity in vitro: antagonist pharmacology and intracellular calcium concentrations. J Neurosci. 1990 Jan;10(1):283–292. doi: 10.1523/JNEUROSCI.10-01-00283.1990. [DOI] [PMC free article] [PubMed] [Google Scholar]
- Nicholls D. G. A role for the mitochondrion in the protection of cells against calcium overload? Prog Brain Res. 1985;63:97–106. doi: 10.1016/S0079-6123(08)61978-0. [DOI] [PubMed] [Google Scholar]
- Palmer A. M., Marion D. W., Botscheller M. L., Swedlow P. E., Styren S. D., DeKosky S. T. Traumatic brain injury-induced excitotoxicity assessed in a controlled cortical impact model. J Neurochem. 1993 Dec;61(6):2015–2024. doi: 10.1111/j.1471-4159.1993.tb07437.x. [DOI] [PubMed] [Google Scholar]
- Randall R. D., Thayer S. A. Glutamate-induced calcium transient triggers delayed calcium overload and neurotoxicity in rat hippocampal neurons. J Neurosci. 1992 May;12(5):1882–1895. doi: 10.1523/JNEUROSCI.12-05-01882.1992. [DOI] [PMC free article] [PubMed] [Google Scholar]
- Reynolds I. J., Hastings T. G. Glutamate induces the production of reactive oxygen species in cultured forebrain neurons following NMDA receptor activation. J Neurosci. 1995 May;15(5 Pt 1):3318–3327. doi: 10.1523/JNEUROSCI.15-05-03318.1995. [DOI] [PMC free article] [PubMed] [Google Scholar]
- Rizzuto R., Brini M., Murgia M., Pozzan T. Microdomains with high Ca2+ close to IP3-sensitive channels that are sensed by neighboring mitochondria. Science. 1993 Oct 29;262(5134):744–747. doi: 10.1126/science.8235595. [DOI] [PubMed] [Google Scholar]
- Satrustegui J., Richter C. The role of hydroperoxides as calcium release agents in rat brain mitochondria. Arch Biochem Biophys. 1984 Sep;233(2):736–740. doi: 10.1016/0003-9861(84)90501-0. [DOI] [PubMed] [Google Scholar]
- Schanne F. A., Kane A. B., Young E. E., Farber J. L. Calcium dependence of toxic cell death: a final common pathway. Science. 1979 Nov 9;206(4419):700–702. doi: 10.1126/science.386513. [DOI] [PubMed] [Google Scholar]
- Stout A. K., Li-Smerin Y., Johnson J. W., Reynolds I. J. Mechanisms of glutamate-stimulated Mg2+ influx and subsequent Mg2+ efflux in rat forebrain neurones in culture. J Physiol. 1996 May 1;492(Pt 3):641–657. doi: 10.1113/jphysiol.1996.sp021334. [DOI] [PMC free article] [PubMed] [Google Scholar]
- Stuenkel E. L. Regulation of intracellular calcium and calcium buffering properties of rat isolated neurohypophysial nerve endings. J Physiol. 1994 Dec 1;481(Pt 2):251–271. doi: 10.1113/jphysiol.1994.sp020436. [DOI] [PMC free article] [PubMed] [Google Scholar]
- Thayer S. A., Miller R. J. Regulation of the intracellular free calcium concentration in single rat dorsal root ganglion neurones in vitro. J Physiol. 1990 Jun;425:85–115. doi: 10.1113/jphysiol.1990.sp018094. [DOI] [PMC free article] [PubMed] [Google Scholar]
- Tymianski M., Charlton M. P., Carlen P. L., Tator C. H. Source specificity of early calcium neurotoxicity in cultured embryonic spinal neurons. J Neurosci. 1993 May;13(5):2085–2104. doi: 10.1523/JNEUROSCI.13-05-02085.1993. [DOI] [PMC free article] [PubMed] [Google Scholar]
- Wahl M., Lucherini M. J., Gruenstein E. Intracellular Ca2+ measurement with Indo-1 in substrate-attached cells: advantages and special considerations. Cell Calcium. 1990 Aug;11(7):487–500. doi: 10.1016/0143-4160(90)90081-5. [DOI] [PubMed] [Google Scholar]
- Wang G. J., Randall R. D., Thayer S. A. Glutamate-induced intracellular acidification of cultured hippocampal neurons demonstrates altered energy metabolism resulting from Ca2+ loads. J Neurophysiol. 1994 Dec;72(6):2563–2569. doi: 10.1152/jn.1994.72.6.2563. [DOI] [PubMed] [Google Scholar]
- Wang G. J., Richardson S. R., Thayer S. A. Intracellular acidification is not a prerequisite for glutamate-triggered death of cultured hippocampal neurons. Neurosci Lett. 1995 Feb 17;186(2-3):139–144. doi: 10.1016/0304-3940(95)11305-g. [DOI] [PubMed] [Google Scholar]
- White R. J., Reynolds I. J. Mitochondria and Na+/Ca2+ exchange buffer glutamate-induced calcium loads in cultured cortical neurons. J Neurosci. 1995 Feb;15(2):1318–1328. doi: 10.1523/JNEUROSCI.15-02-01318.1995. [DOI] [PMC free article] [PubMed] [Google Scholar]
- White R. J., Reynolds I. J. Mitochondrial depolarization in glutamate-stimulated neurons: an early signal specific to excitotoxin exposure. J Neurosci. 1996 Sep 15;16(18):5688–5697. doi: 10.1523/JNEUROSCI.16-18-05688.1996. [DOI] [PMC free article] [PubMed] [Google Scholar]
- Zhang J., Dawson V. L., Dawson T. M., Snyder S. H. Nitric oxide activation of poly(ADP-ribose) synthetase in neurotoxicity. Science. 1994 Feb 4;263(5147):687–689. doi: 10.1126/science.8080500. [DOI] [PubMed] [Google Scholar]