Abstract
1. Shaker B K+ channels, expressed in the insect cell line Sf9, were studied in zero K+, Na+ or N-methyl-D-glucamine (NMG)-containing solutions. In the absence of K+ ions on both sides of the membrane, the K+ conductance collapsed with the delivery of short depolarizing pulses that activated the channels. The collapse of the conductance was fully prevented when the channels were kept closed at a holding potential of -80 mV. 2. The fall in K+ conductance had the notable characteristic of being strikingly stable. At -80 mV or more negative holding potentials, the conductance never recovered (cells observed for up to 1 h). 3. The extent of collapse of the K+ conductance depended on the number of depolarizing activating pulses applied in zero K+ solutions. For moderate to low frequencies of pulsing (1 to 0.002 Hz), the extent of the collapse did not depend on the frequency. 4. K+, Rb+, Cs+ and NH4+ added to the external Na+ solution impeded the fall in K+ conductance. 5. TEA added to the external, zero K+, Na(+)-containing solution also precluded the fall of the conductance. The protection by TEA paralleled its block of the outward K+ currents recorded with standard recording solutions. 6. The fall in K+ conductance was prevented by depolarized holding potentials. 7. The K+ conductance that was thought to be irreversibly lost at -80 mV or more negative holding potentials was fully recovered, however, after a prolonged (tens of seconds to minutes) change in the holding potential to depolarized values (above -50 mV). Full recovery could be obtained at any time after the former halt of the conductance.
Full text
PDF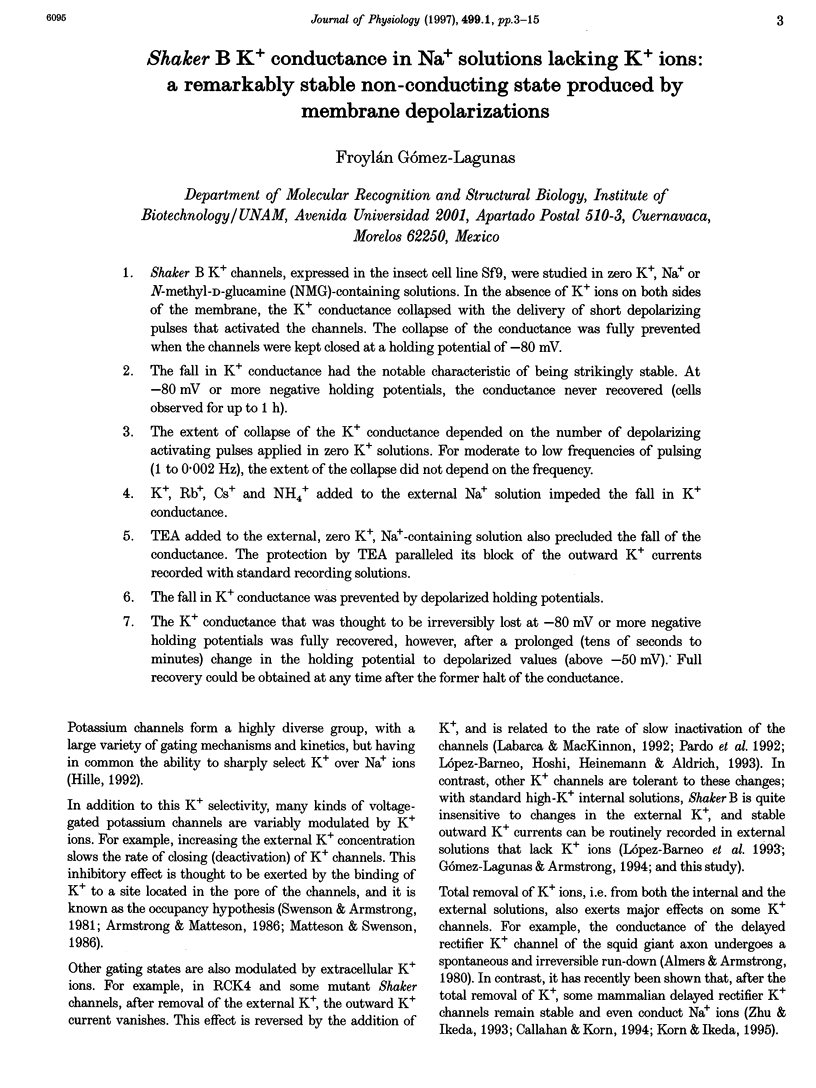
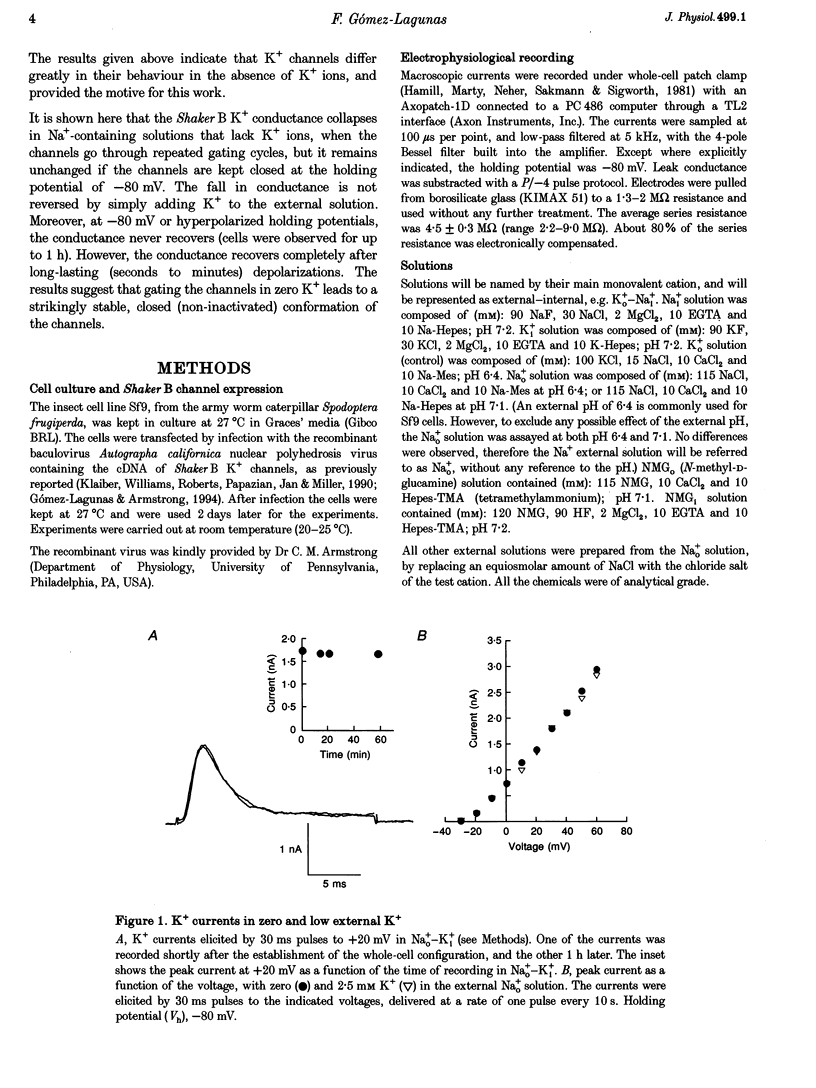
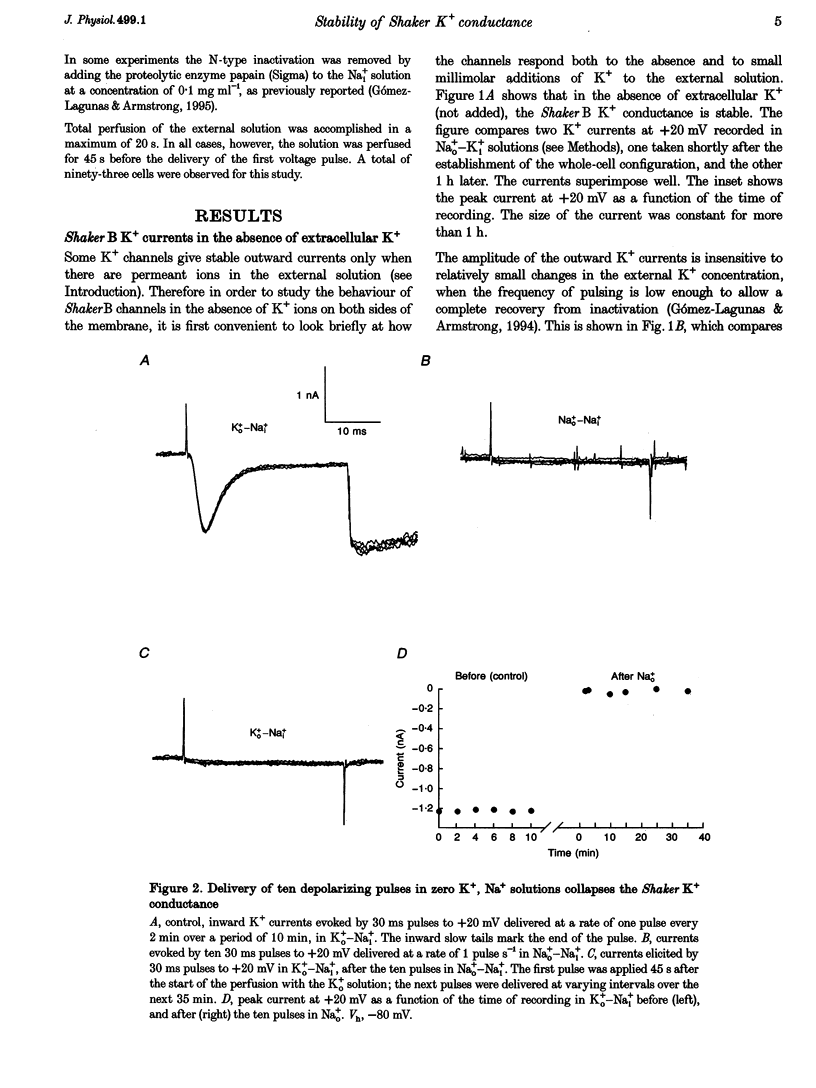
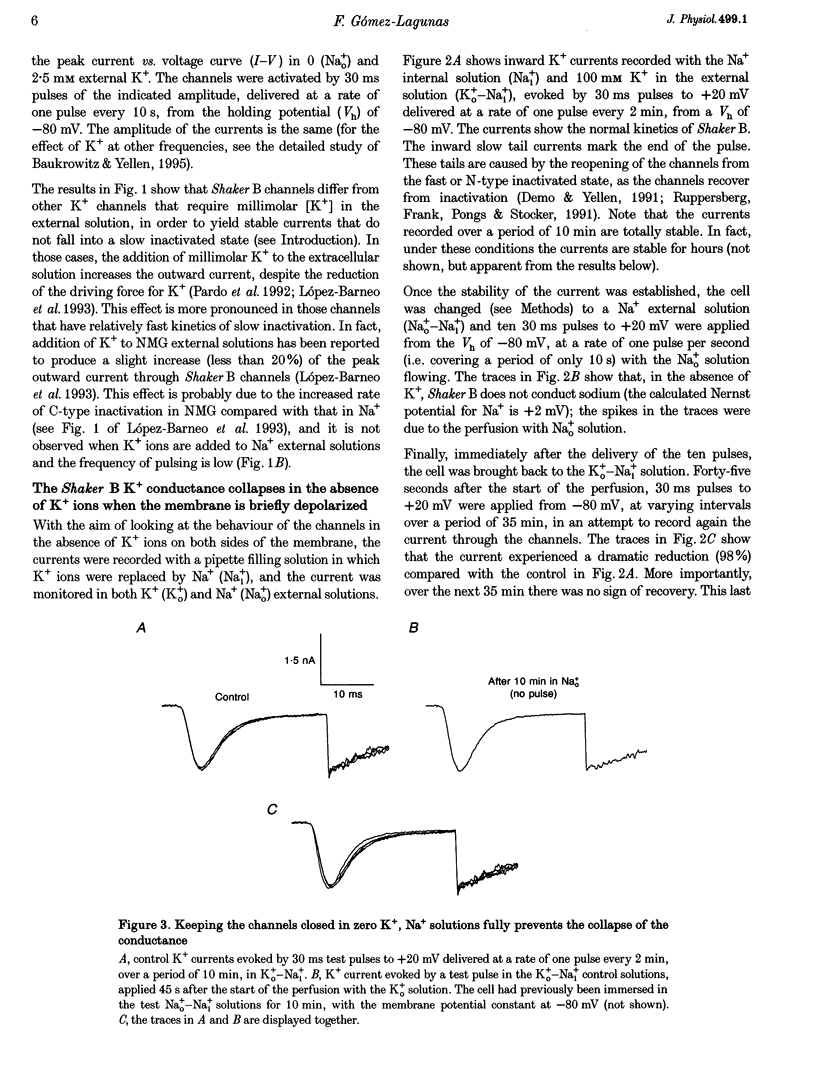
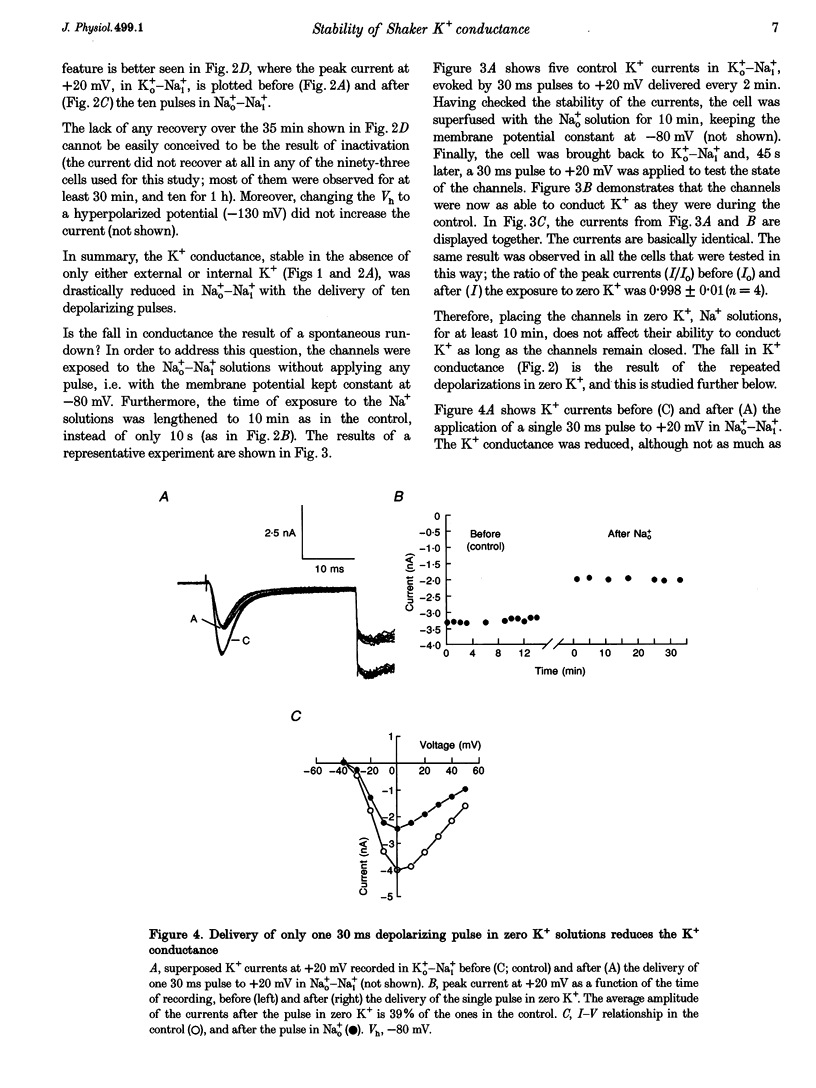
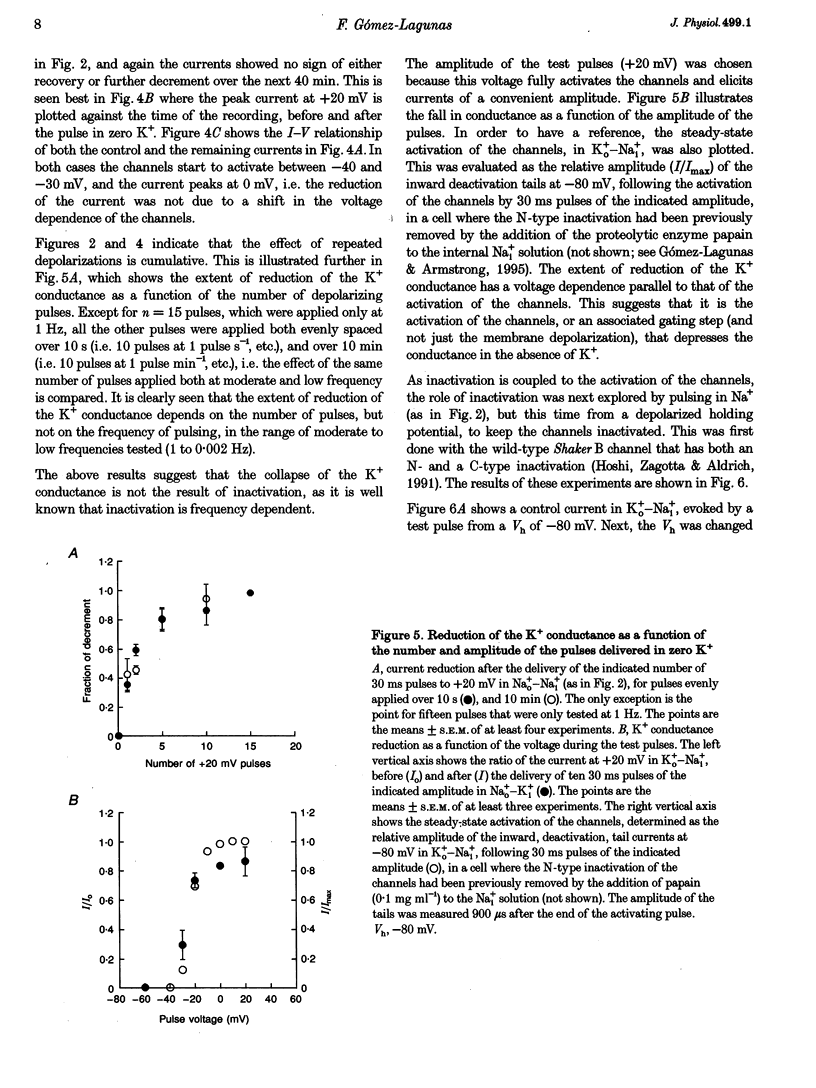
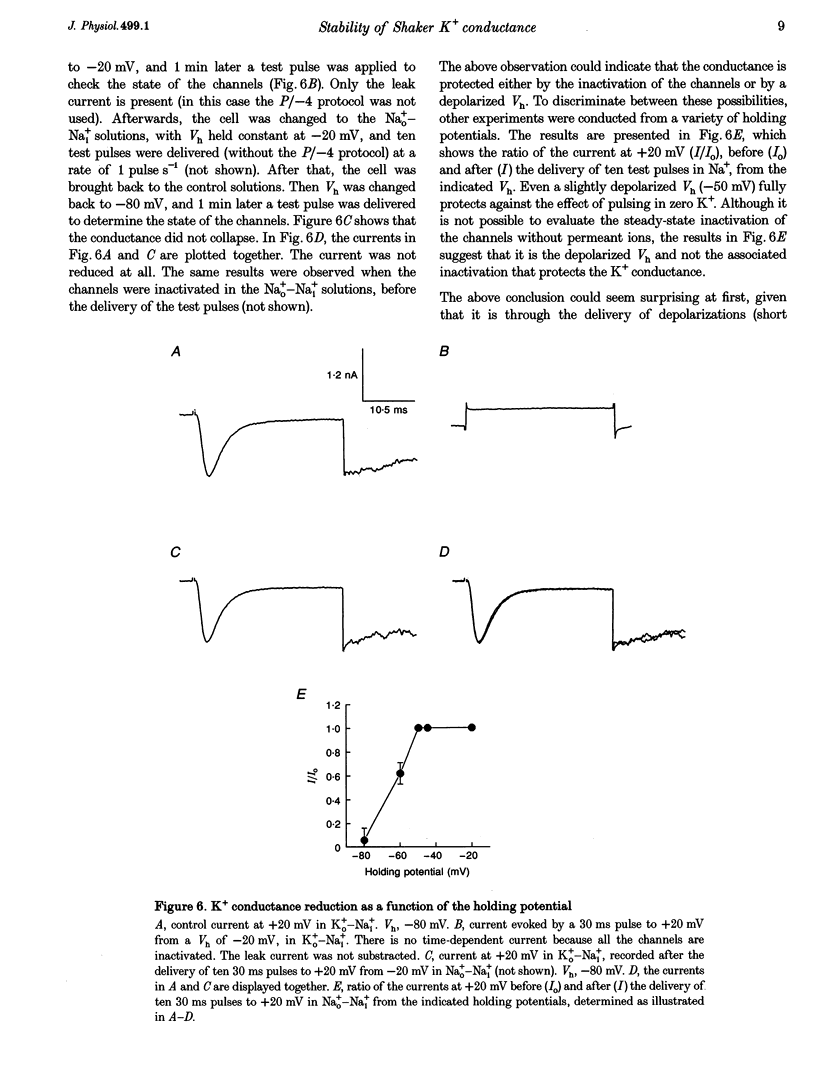
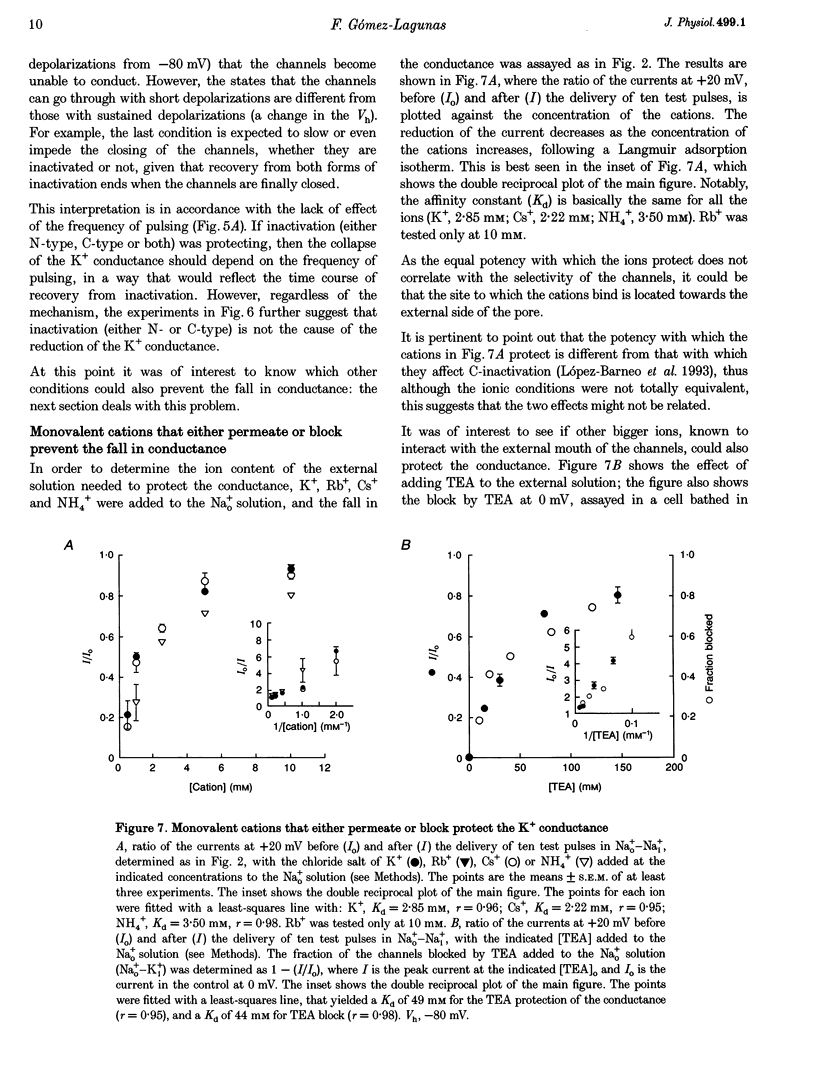
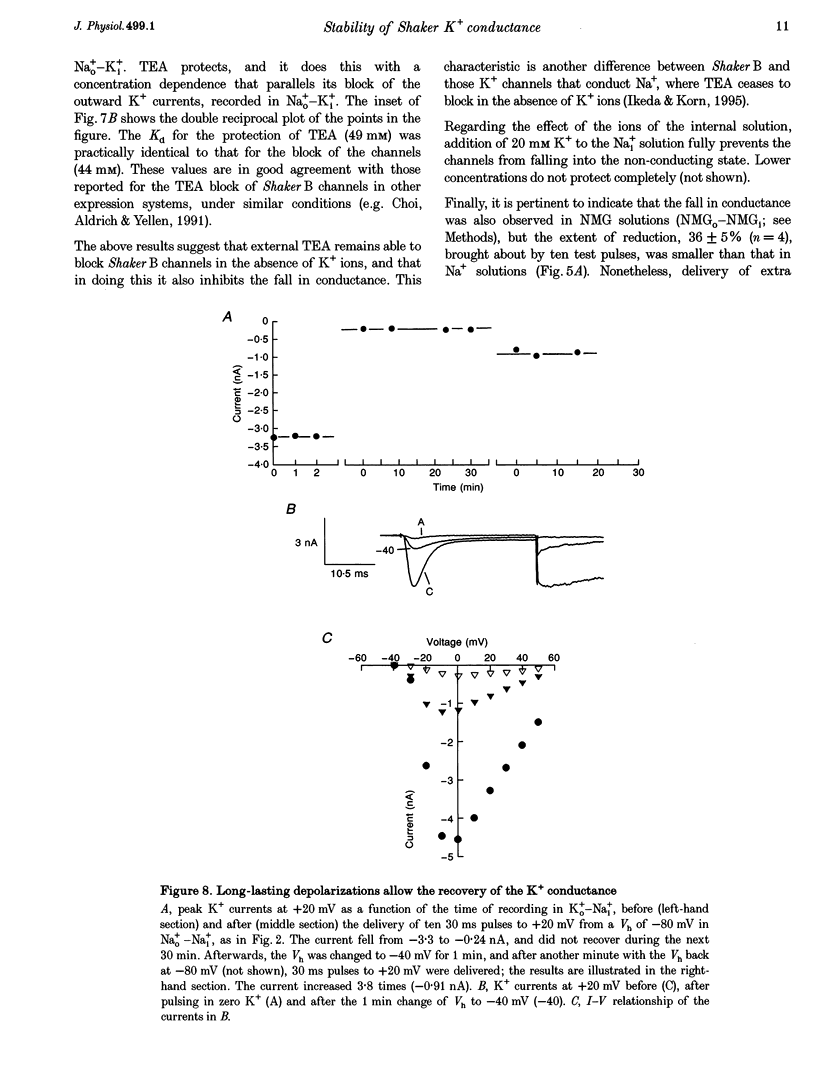
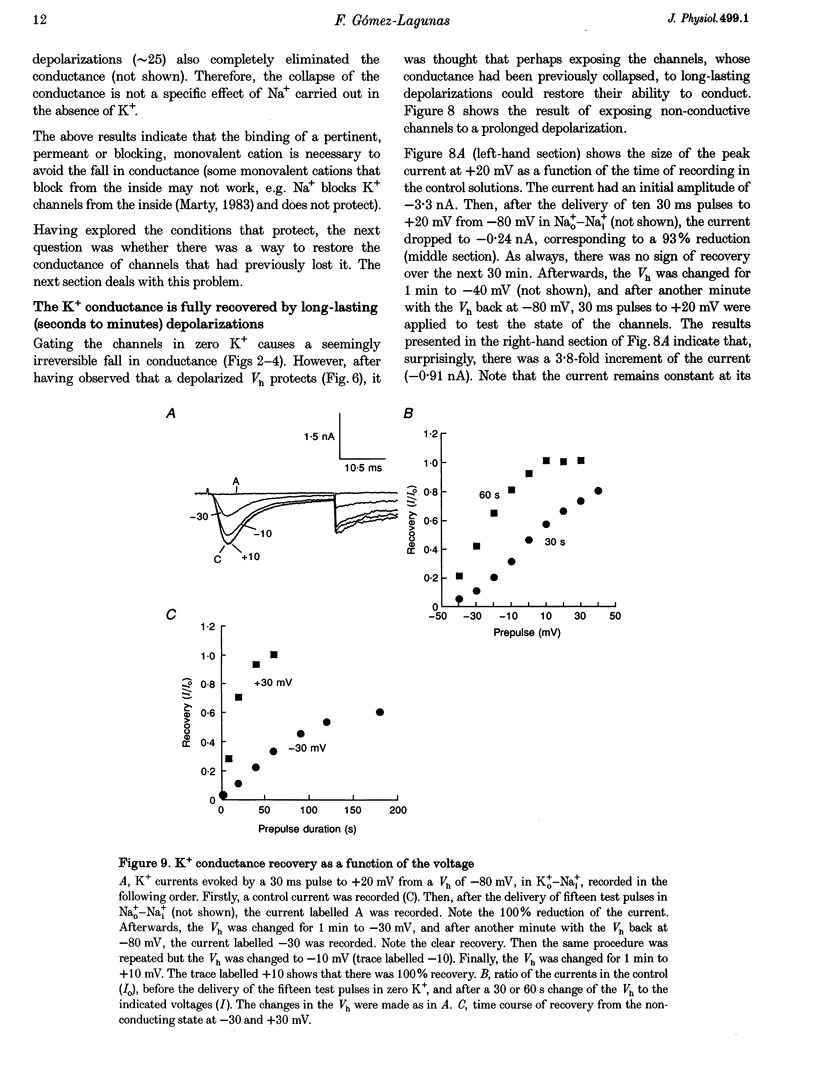
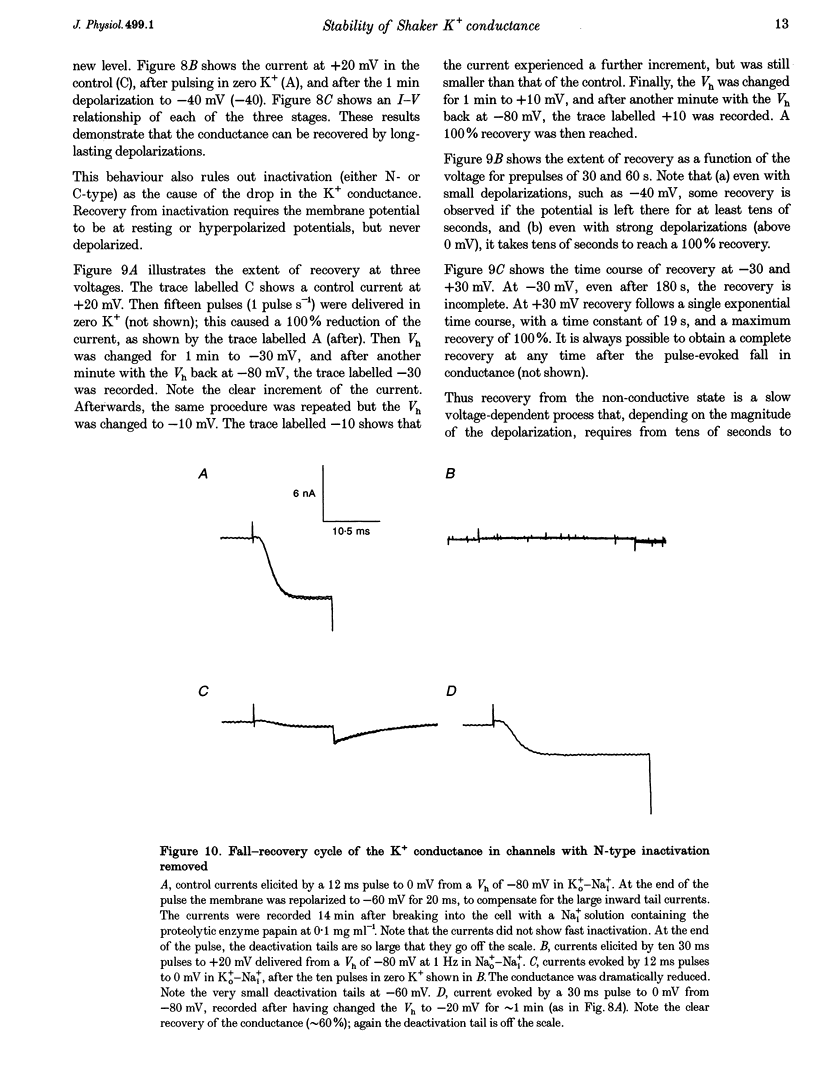
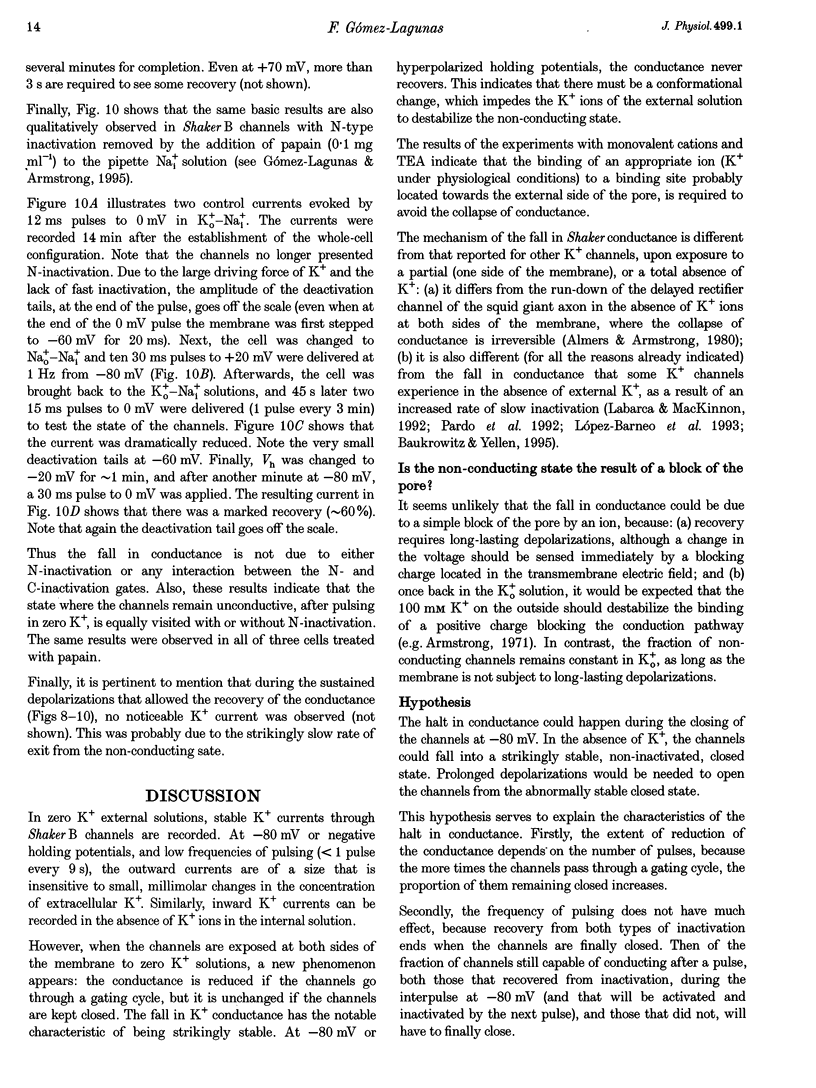
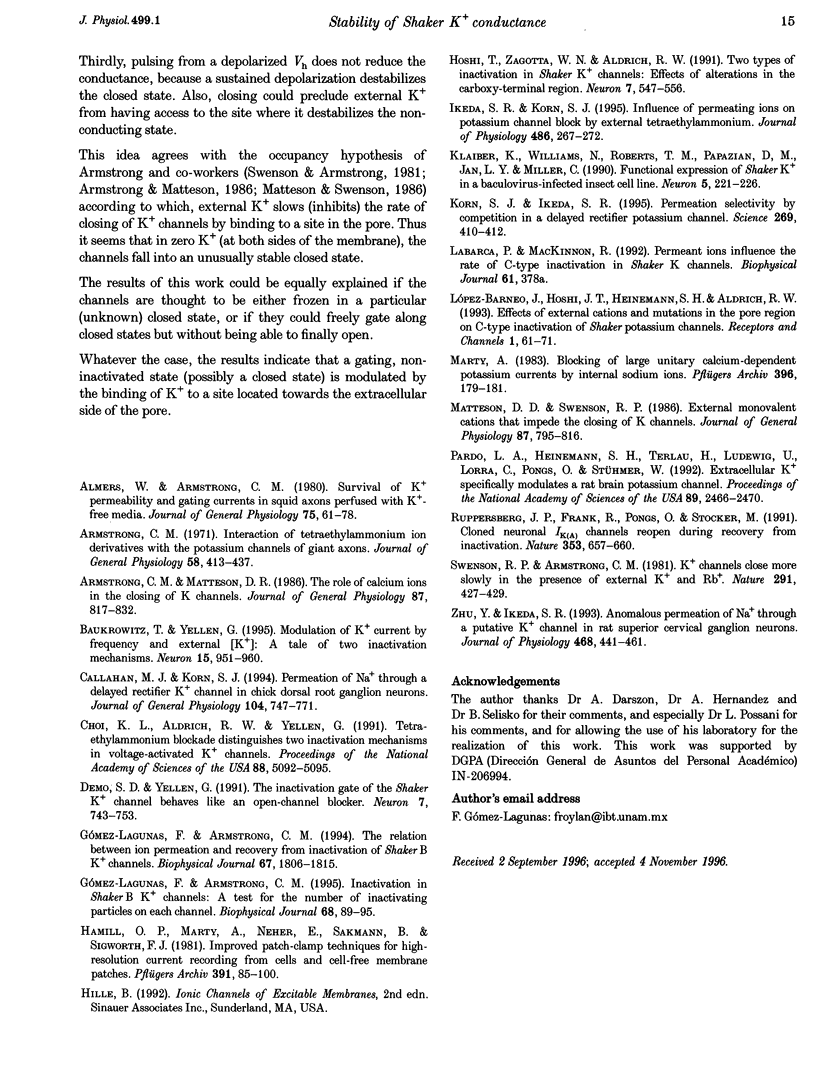
Selected References
These references are in PubMed. This may not be the complete list of references from this article.
- Armstrong C. M. Interaction of tetraethylammonium ion derivatives with the potassium channels of giant axons. J Gen Physiol. 1971 Oct;58(4):413–437. doi: 10.1085/jgp.58.4.413. [DOI] [PMC free article] [PubMed] [Google Scholar]
- Armstrong C. M., Matteson D. R. The role of calcium ions in the closing of K channels. J Gen Physiol. 1986 May;87(5):817–832. doi: 10.1085/jgp.87.5.817. [DOI] [PMC free article] [PubMed] [Google Scholar]
- Baukrowitz T., Yellen G. Modulation of K+ current by frequency and external [K+]: a tale of two inactivation mechanisms. Neuron. 1995 Oct;15(4):951–960. doi: 10.1016/0896-6273(95)90185-x. [DOI] [PubMed] [Google Scholar]
- Callahan M. J., Korn S. J. Permeation of Na+ through a delayed rectifier K+ channel in chick dorsal root ganglion neurons. J Gen Physiol. 1994 Oct;104(4):747–771. doi: 10.1085/jgp.104.4.747. [DOI] [PMC free article] [PubMed] [Google Scholar]
- Choi K. L., Aldrich R. W., Yellen G. Tetraethylammonium blockade distinguishes two inactivation mechanisms in voltage-activated K+ channels. Proc Natl Acad Sci U S A. 1991 Jun 15;88(12):5092–5095. doi: 10.1073/pnas.88.12.5092. [DOI] [PMC free article] [PubMed] [Google Scholar]
- Demo S. D., Yellen G. The inactivation gate of the Shaker K+ channel behaves like an open-channel blocker. Neuron. 1991 Nov;7(5):743–753. doi: 10.1016/0896-6273(91)90277-7. [DOI] [PubMed] [Google Scholar]
- Gomez-Lagunas F., Armstrong C. M. Inactivation in ShakerB K+ channels: a test for the number of inactivating particles on each channel. Biophys J. 1995 Jan;68(1):89–95. doi: 10.1016/S0006-3495(95)80162-1. [DOI] [PMC free article] [PubMed] [Google Scholar]
- Gómez-Lagunas F., Armstrong C. M. The relation between ion permeation and recovery from inactivation of ShakerB K+ channels. Biophys J. 1994 Nov;67(5):1806–1815. doi: 10.1016/S0006-3495(94)80662-9. [DOI] [PMC free article] [PubMed] [Google Scholar]
- Hamill O. P., Marty A., Neher E., Sakmann B., Sigworth F. J. Improved patch-clamp techniques for high-resolution current recording from cells and cell-free membrane patches. Pflugers Arch. 1981 Aug;391(2):85–100. doi: 10.1007/BF00656997. [DOI] [PubMed] [Google Scholar]
- Hoshi T., Zagotta W. N., Aldrich R. W. Two types of inactivation in Shaker K+ channels: effects of alterations in the carboxy-terminal region. Neuron. 1991 Oct;7(4):547–556. doi: 10.1016/0896-6273(91)90367-9. [DOI] [PubMed] [Google Scholar]
- Ikeda S. R., Korn S. J. Influence of permeating ions on potassium channel block by external tetraethylammonium. J Physiol. 1995 Jul 15;486(Pt 2):267–272. doi: 10.1113/jphysiol.1995.sp020809. [DOI] [PMC free article] [PubMed] [Google Scholar]
- Klaiber K., Williams N., Roberts T. M., Papazian D. M., Jan L. Y., Miller C. Functional expression of Shaker K+ channels in a baculovirus-infected insect cell line. Neuron. 1990 Aug;5(2):221–226. doi: 10.1016/0896-6273(90)90311-3. [DOI] [PubMed] [Google Scholar]
- Korn S. J., Ikeda S. R. Permeation selectivity by competition in a delayed rectifier potassium channel. Science. 1995 Jul 21;269(5222):410–412. doi: 10.1126/science.7618108. [DOI] [PubMed] [Google Scholar]
- López-Barneo J., Hoshi T., Heinemann S. H., Aldrich R. W. Effects of external cations and mutations in the pore region on C-type inactivation of Shaker potassium channels. Receptors Channels. 1993;1(1):61–71. [PubMed] [Google Scholar]
- Marty A. Blocking of large unitary calcium-dependent potassium currents by internal sodium ions. Pflugers Arch. 1983 Feb;396(2):179–181. doi: 10.1007/BF00615524. [DOI] [PubMed] [Google Scholar]
- Matteson D. R., Swenson R. P., Jr External monovalent cations that impede the closing of K channels. J Gen Physiol. 1986 May;87(5):795–816. doi: 10.1085/jgp.87.5.795. [DOI] [PMC free article] [PubMed] [Google Scholar]
- Pardo L. A., Heinemann S. H., Terlau H., Ludewig U., Lorra C., Pongs O., Stühmer W. Extracellular K+ specifically modulates a rat brain K+ channel. Proc Natl Acad Sci U S A. 1992 Mar 15;89(6):2466–2470. doi: 10.1073/pnas.89.6.2466. [DOI] [PMC free article] [PubMed] [Google Scholar]
- Ruppersberg J. P., Frank R., Pongs O., Stocker M. Cloned neuronal IK(A) channels reopen during recovery from inactivation. Nature. 1991 Oct 17;353(6345):657–660. doi: 10.1038/353657a0. [DOI] [PubMed] [Google Scholar]
- Swenson R. P., Jr, Armstrong C. M. K+ channels close more slowly in the presence of external K+ and Rb+. Nature. 1981 Jun 4;291(5814):427–429. doi: 10.1038/291427a0. [DOI] [PubMed] [Google Scholar]
- Zhu Y., Ikeda S. R. Anomalous permeation of Na+ through a putative K+ channel in rat superior cervical ganglion neurones. J Physiol. 1993 Aug;468:441–461. doi: 10.1113/jphysiol.1993.sp019781. [DOI] [PMC free article] [PubMed] [Google Scholar]