Abstract
1. The mechanism of the sustained acetylcholine-induced endothelium-dependent hyperpolarization (EDH) in intact rat small mesenteric arteries prestimulated with noradrenaline (10(-6) M) was investigated by means of the single microelectrode voltage-clamp method. 2. The vascular smooth muscle cells (VSMCs) in this preparation are poorly or even not coupled for the reasons that: (1) the mean input resistance Rlnp of the clamped vascular smooth muscle increases from 120 M omega under control conditions to 440 M omega after application of K+ channel blocking drugs, (2) the voltage relaxation after injection of hyperpolarizing currents has a monoexponential time course and is linearly dependent on Rlnp, and (3) voltage steps induced by current-clamp steps are not transferred to locations in the vascular musculature 120 microns apart from the current injecting microelectrode. 3. Sustained (> 5 min) application of ACh (10(-5) M) hyperpolarized the VSMCs by induction of a hyperpolarizing current. This effect was completely blocked by the inhibitor of the nitric oxide (NO) synthase L-NAME (10(-3) M) but not by the inhibitor of the soluble guanylate cyclase (sGCl) Methylene Blue (MB, 10(-4) M). 4. Application of the NO donor sodium nitroprusside (SNP, 10(-6) M) for more than 5 min mimicked the induction of the endothelium-dependent hyperpolarizing current in vessels with destroyed endothelium. The reversal potential of this current is dependent on the extracellular K+ concentration. The effect of SNP could also not be blocked by MB. 5. The blockers of ATP-dependent and Ca(2+)-dependent K+ channels, glibenclamide (Glb, 10(-5) M) and charybdotoxin (CTX, 5 x 10(-8) M), respectively, blocked a hyperpolarizing current in the VSMCs similar to the ACh- or SNP-induced current. 6. The isolated application of either Glb or CTX did not block the activation of the hyperpolarizing current by SNP. Only the combined administration of Glb and CTX blocked the SNP-induced current completely. 7. Our results suggest that in rat small mesenteric artery, ACh hyperpolarizes the VSMCs tonically by activating both ATP- and Ca(2+)-dependent K+ currents, only via release of NO from the endothelium without need for activation of the sGCl.
Full text
PDF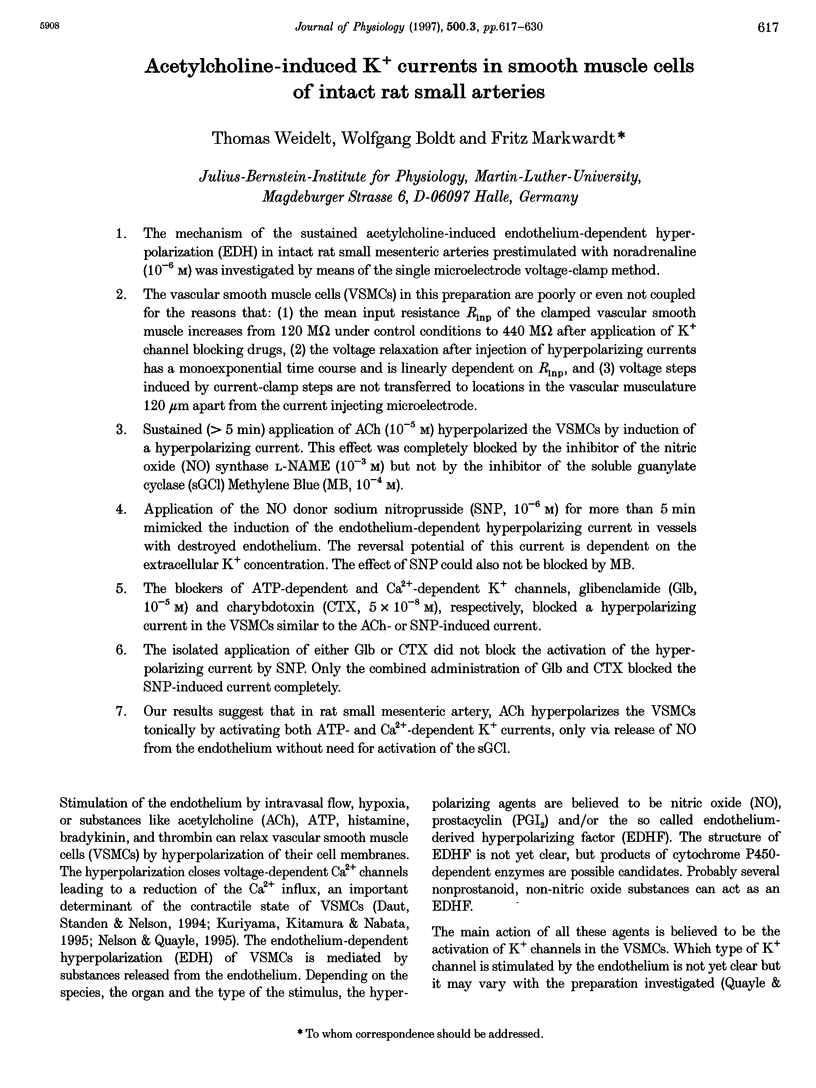
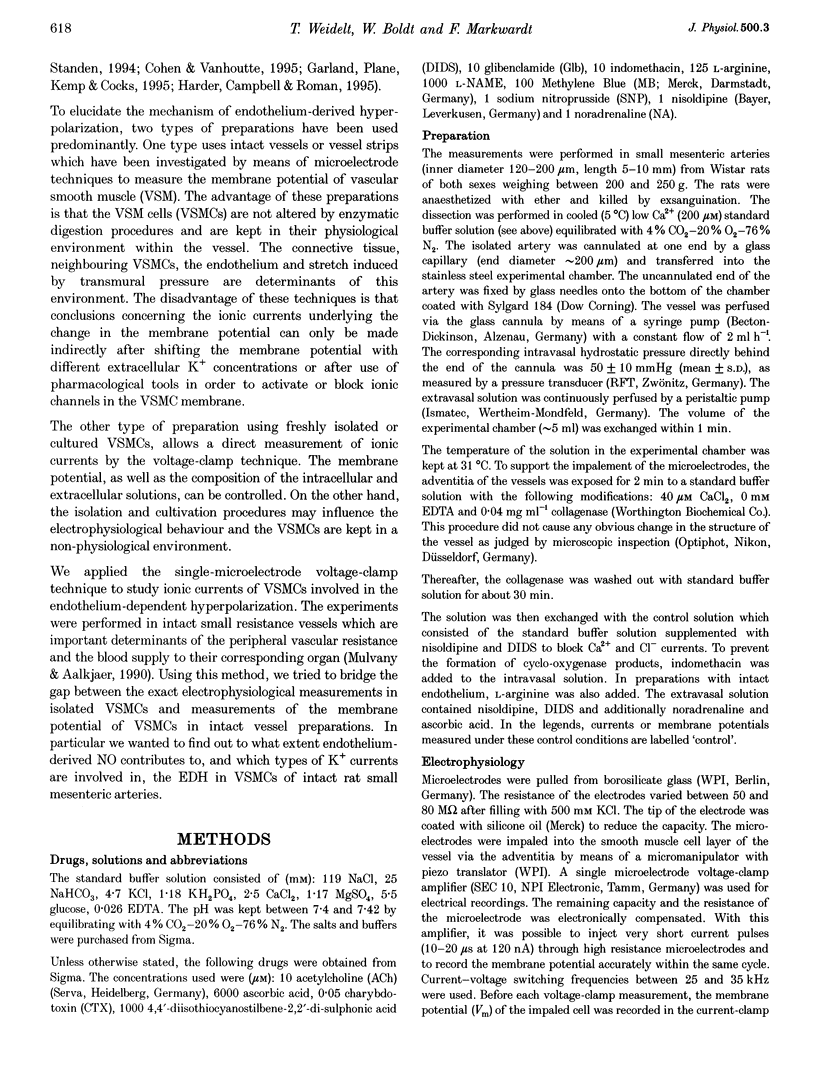
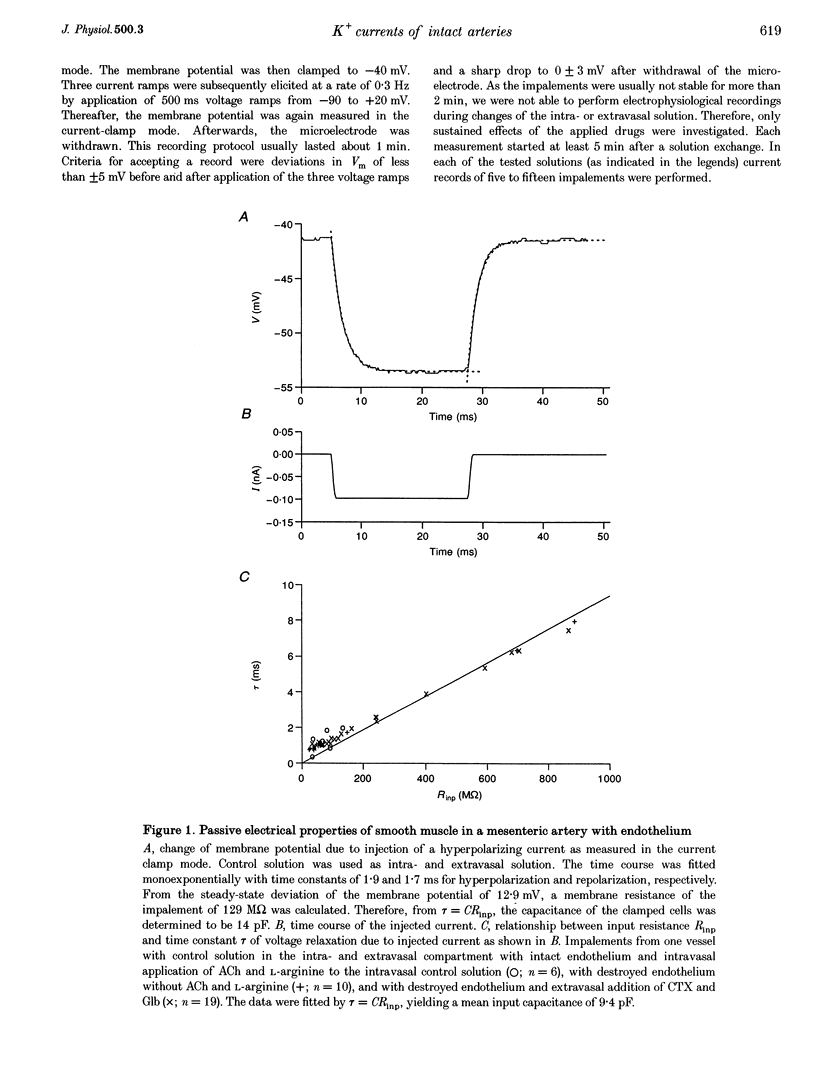
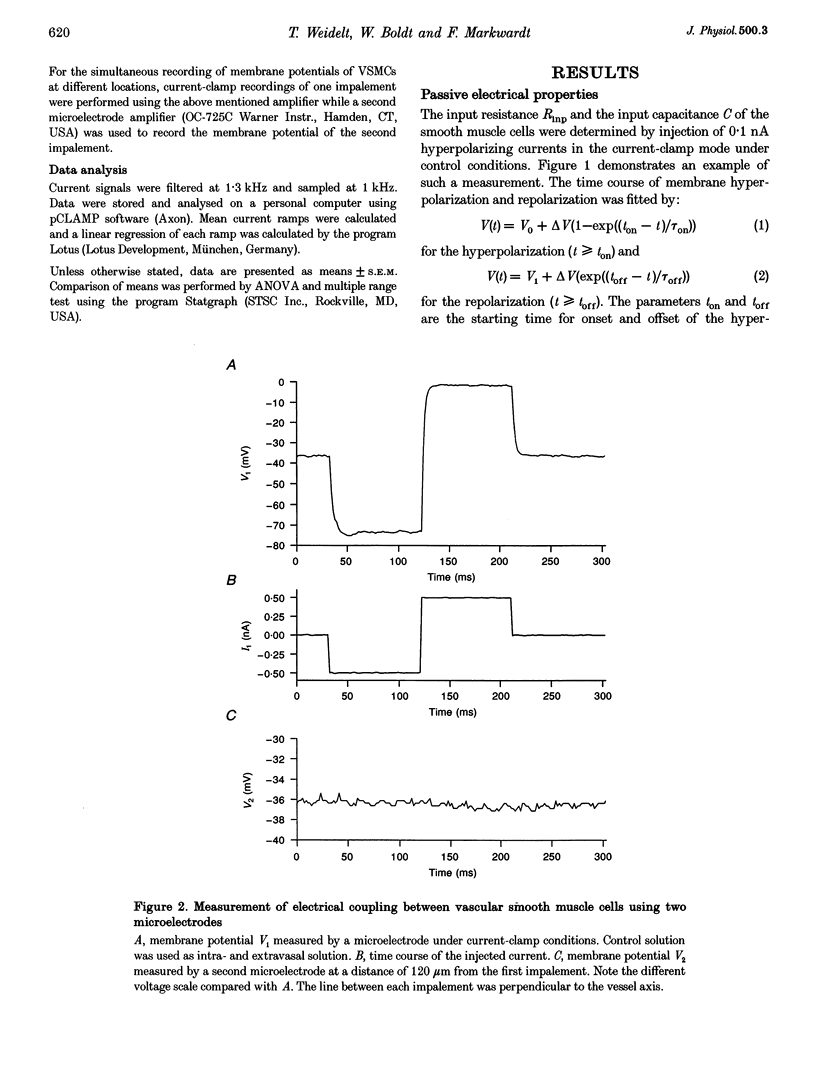
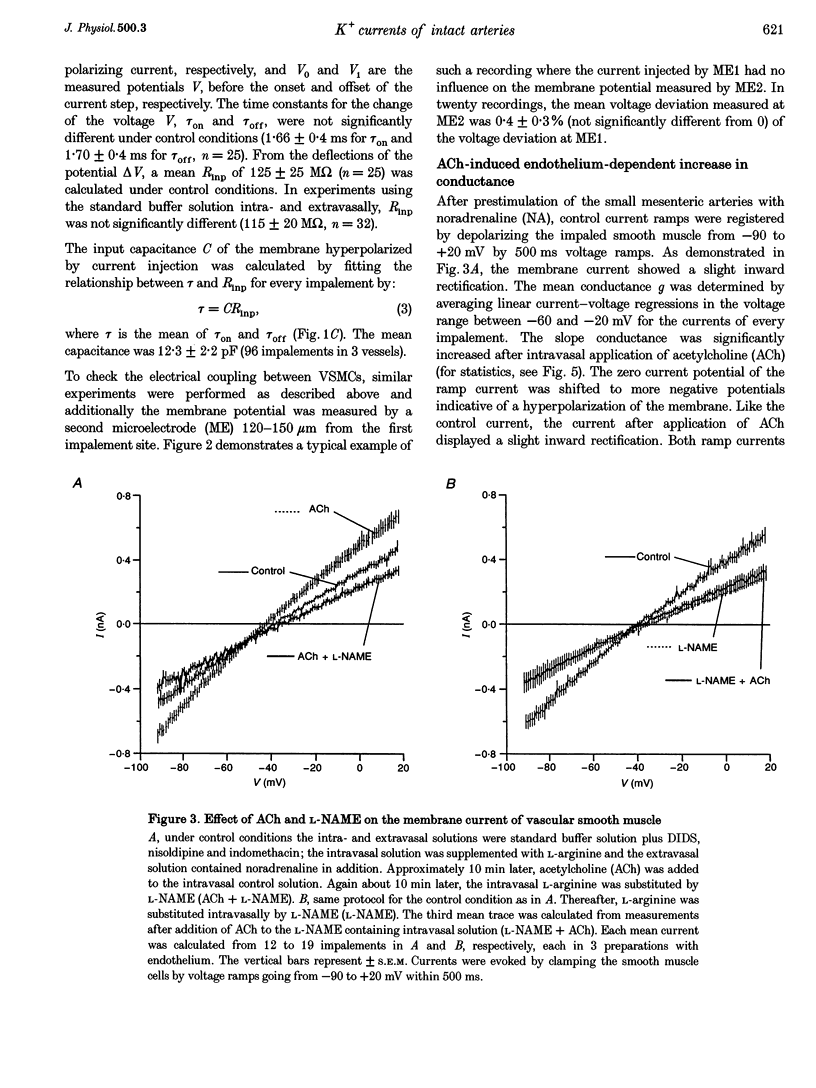
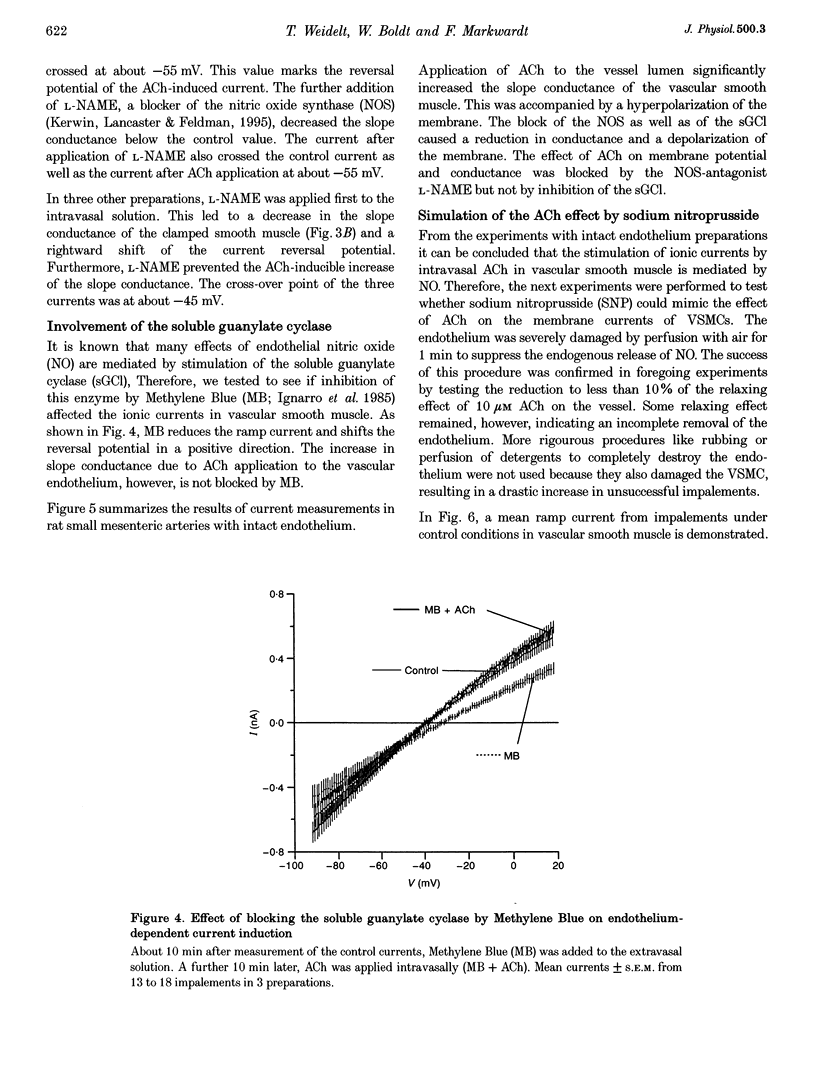
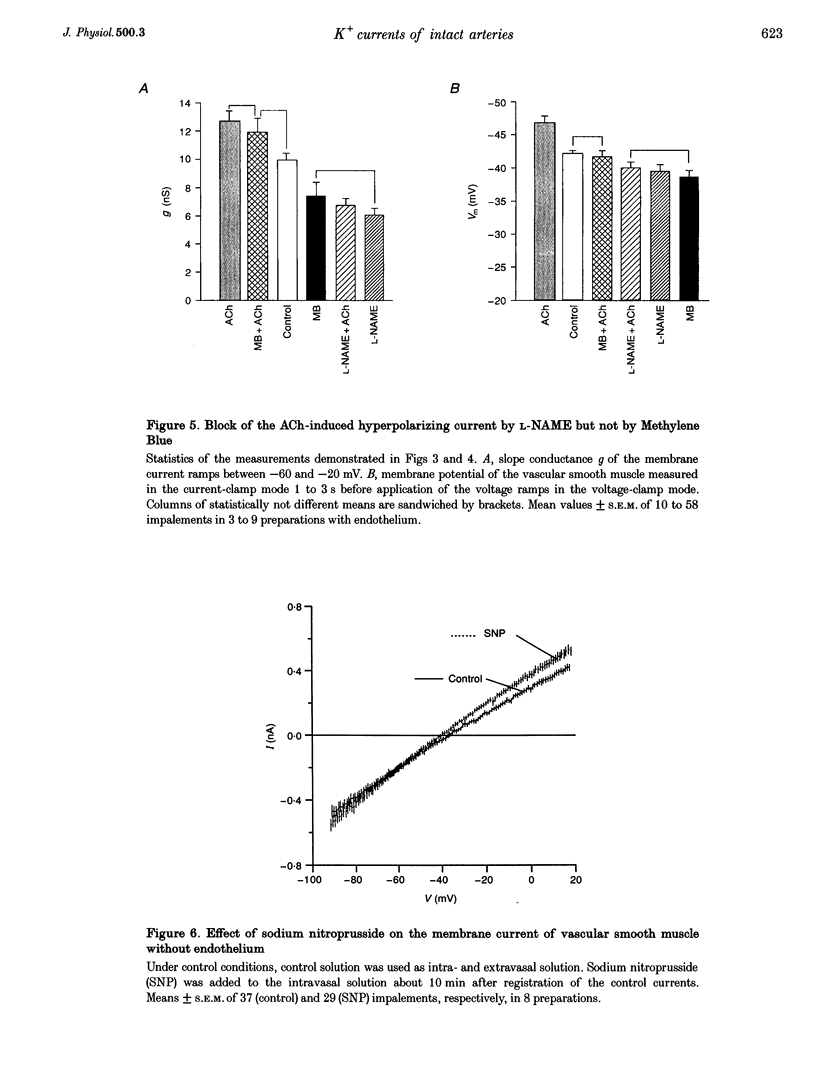
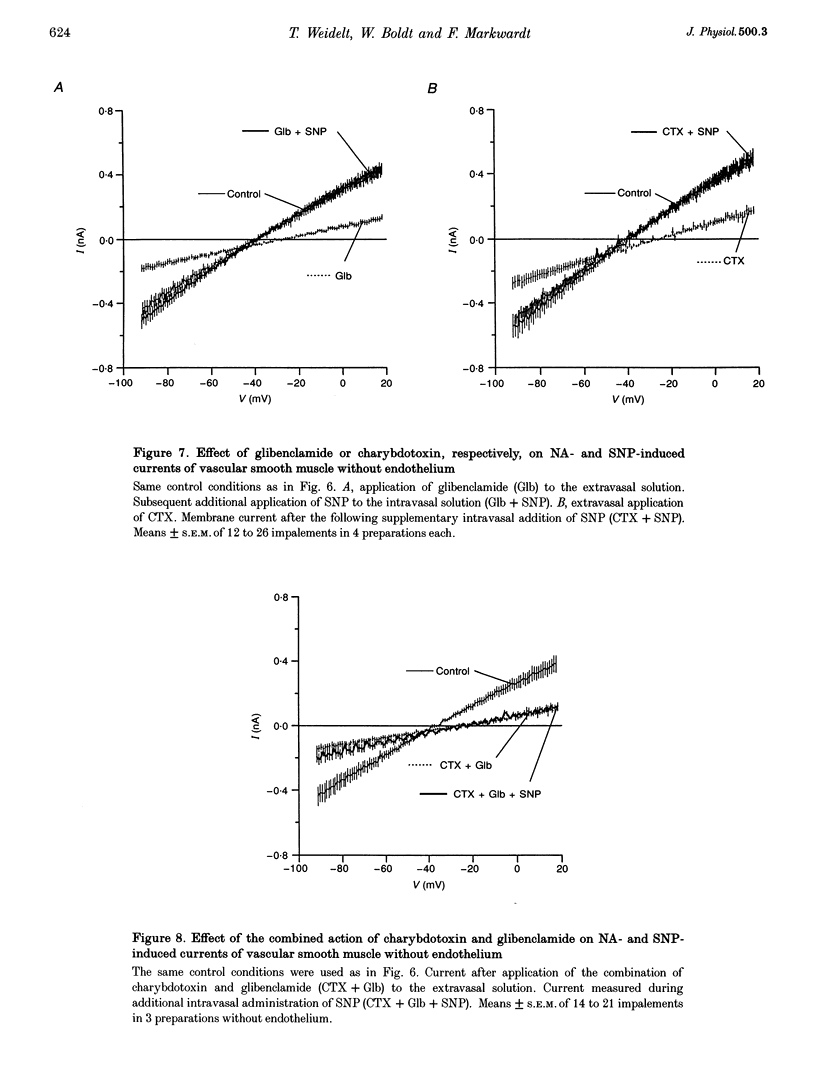
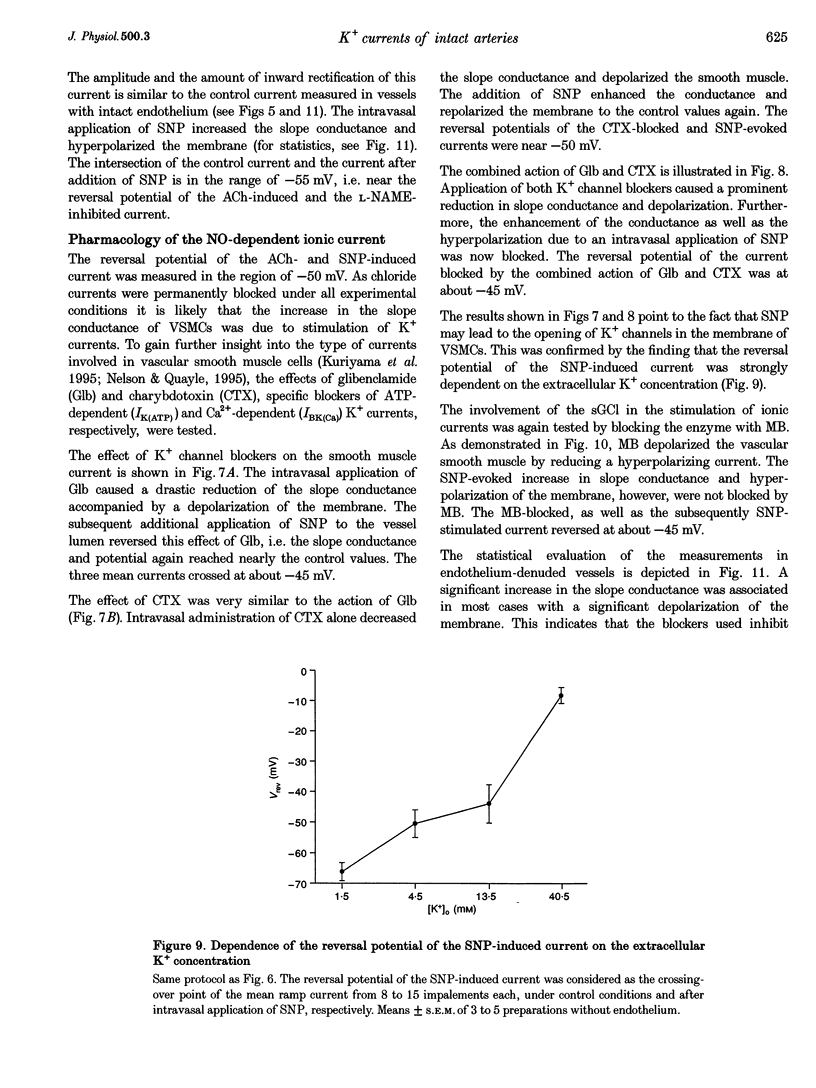
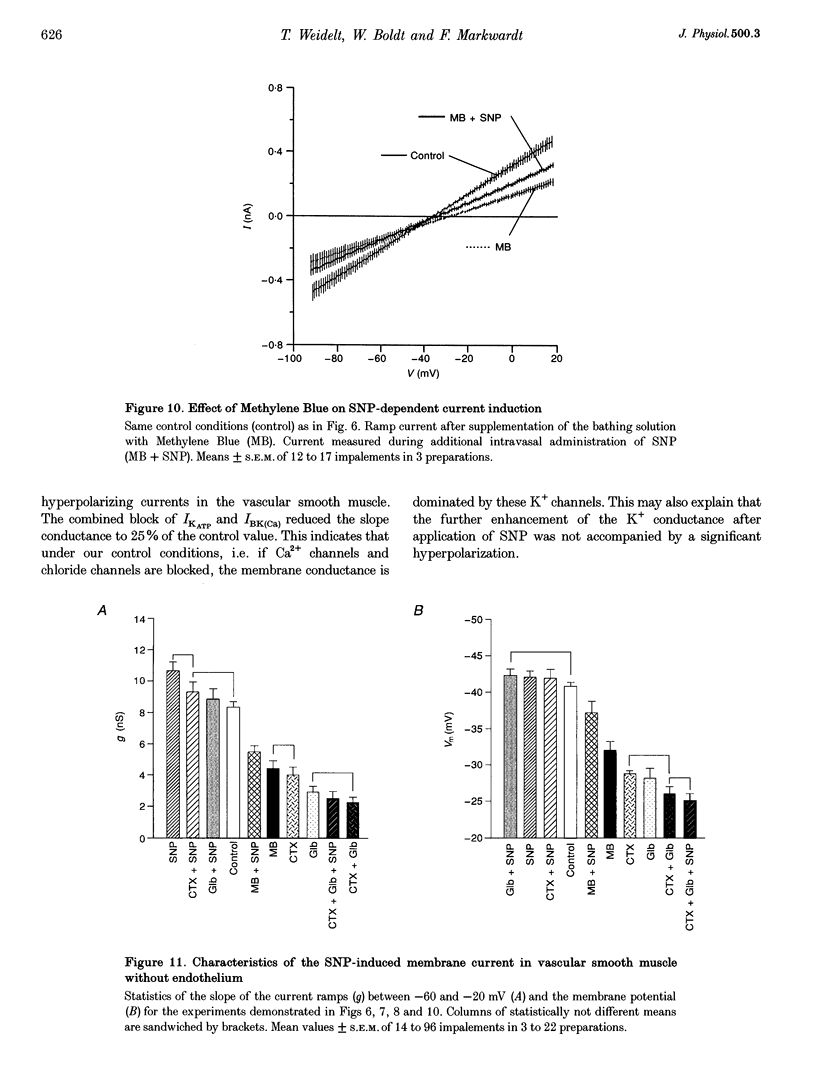
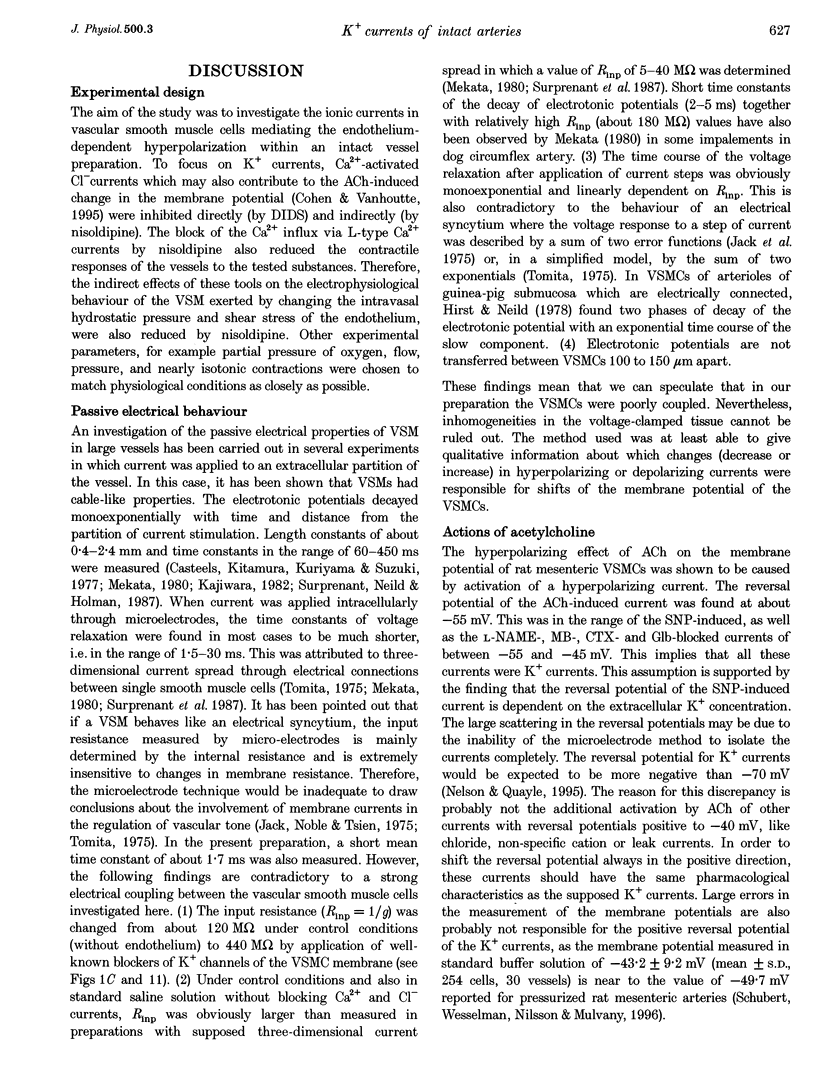
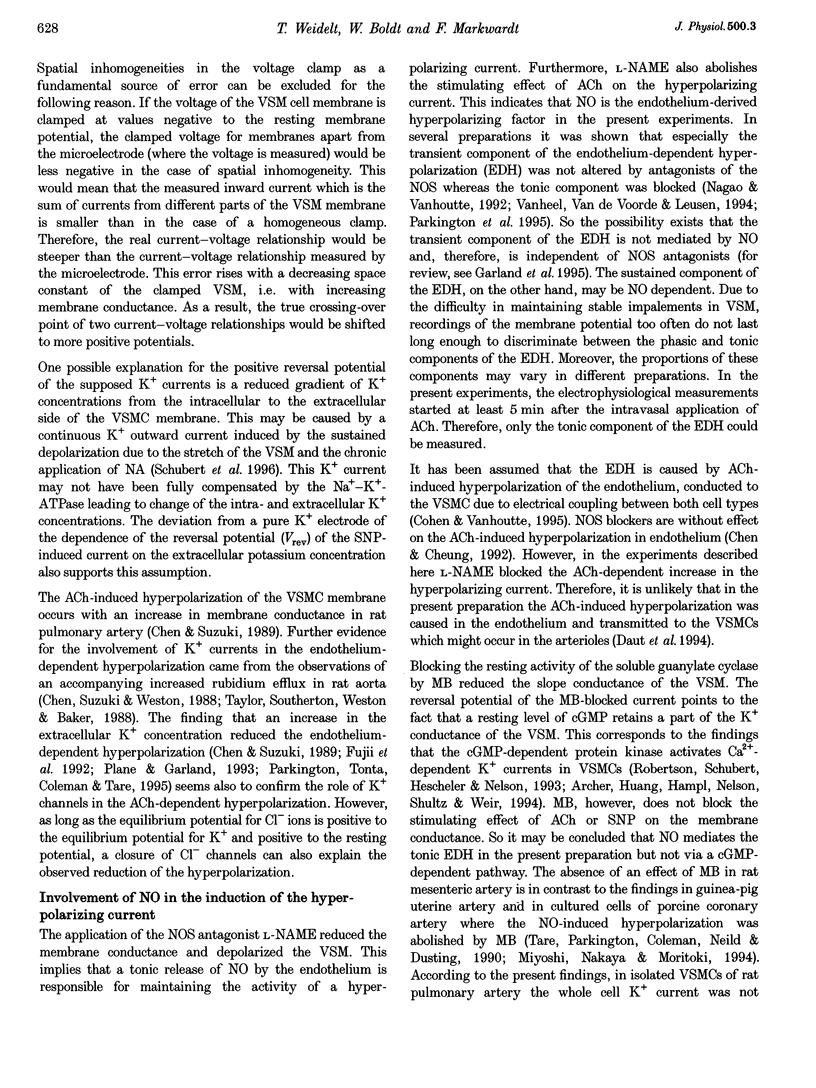
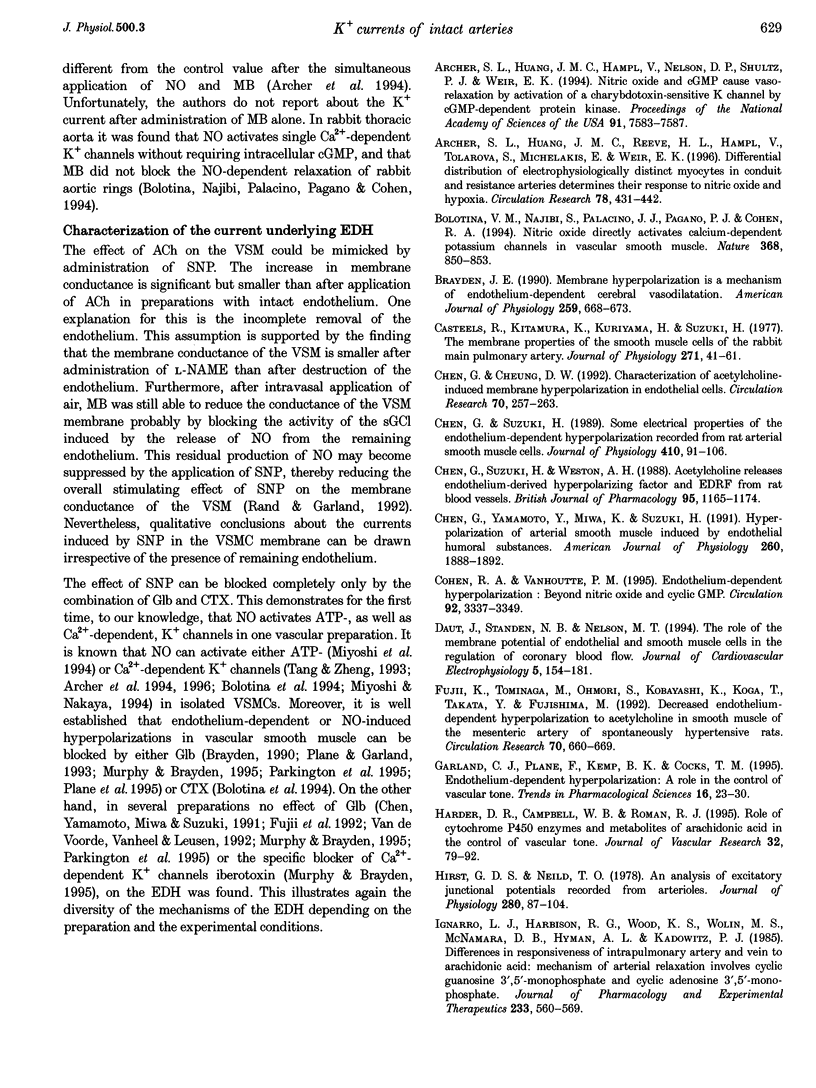
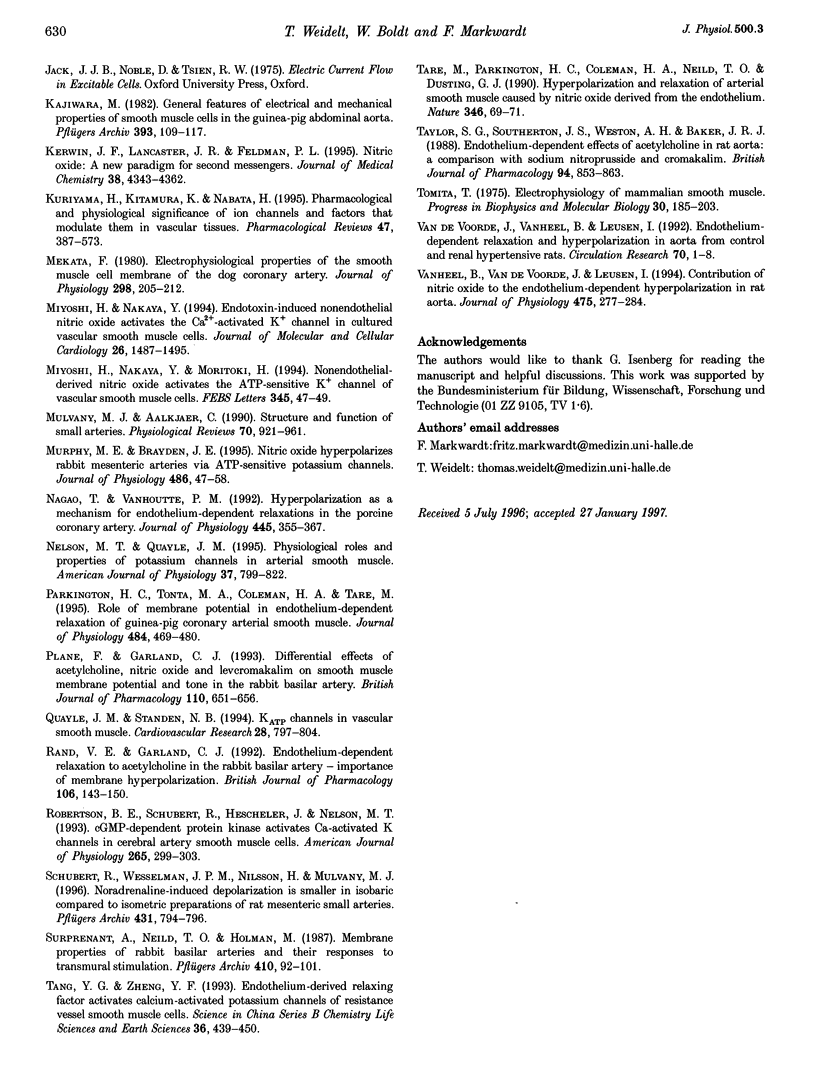
Images in this article
Selected References
These references are in PubMed. This may not be the complete list of references from this article.
- Archer S. L., Huang J. M., Hampl V., Nelson D. P., Shultz P. J., Weir E. K. Nitric oxide and cGMP cause vasorelaxation by activation of a charybdotoxin-sensitive K channel by cGMP-dependent protein kinase. Proc Natl Acad Sci U S A. 1994 Aug 2;91(16):7583–7587. doi: 10.1073/pnas.91.16.7583. [DOI] [PMC free article] [PubMed] [Google Scholar]
- Archer S. L., Huang J. M., Reeve H. L., Hampl V., Tolarová S., Michelakis E., Weir E. K. Differential distribution of electrophysiologically distinct myocytes in conduit and resistance arteries determines their response to nitric oxide and hypoxia. Circ Res. 1996 Mar;78(3):431–442. doi: 10.1161/01.res.78.3.431. [DOI] [PubMed] [Google Scholar]
- Bolotina V. M., Najibi S., Palacino J. J., Pagano P. J., Cohen R. A. Nitric oxide directly activates calcium-dependent potassium channels in vascular smooth muscle. Nature. 1994 Apr 28;368(6474):850–853. doi: 10.1038/368850a0. [DOI] [PubMed] [Google Scholar]
- Casteels R., Kitamura K., Kuriyama H., Suzuki H. The membrane properties of the smooth muscle cells of the rabbit main pulmonary artery. J Physiol. 1977 Sep;271(1):41–61. doi: 10.1113/jphysiol.1977.sp011989. [DOI] [PMC free article] [PubMed] [Google Scholar]
- Chen G. F., Cheung D. W. Characterization of acetylcholine-induced membrane hyperpolarization in endothelial cells. Circ Res. 1992 Feb;70(2):257–263. doi: 10.1161/01.res.70.2.257. [DOI] [PubMed] [Google Scholar]
- Chen G., Suzuki H. Some electrical properties of the endothelium-dependent hyperpolarization recorded from rat arterial smooth muscle cells. J Physiol. 1989 Mar;410:91–106. doi: 10.1113/jphysiol.1989.sp017522. [DOI] [PMC free article] [PubMed] [Google Scholar]
- Chen G., Suzuki H., Weston A. H. Acetylcholine releases endothelium-derived hyperpolarizing factor and EDRF from rat blood vessels. Br J Pharmacol. 1988 Dec;95(4):1165–1174. doi: 10.1111/j.1476-5381.1988.tb11752.x. [DOI] [PMC free article] [PubMed] [Google Scholar]
- Cohen R. A., Vanhoutte P. M. Endothelium-dependent hyperpolarization. Beyond nitric oxide and cyclic GMP. Circulation. 1995 Dec 1;92(11):3337–3349. doi: 10.1161/01.cir.92.11.3337. [DOI] [PubMed] [Google Scholar]
- Daut J., Standen N. B., Nelson M. T. The role of the membrane potential of endothelial and smooth muscle cells in the regulation of coronary blood flow. J Cardiovasc Electrophysiol. 1994 Feb;5(2):154–181. doi: 10.1111/j.1540-8167.1994.tb01156.x. [DOI] [PubMed] [Google Scholar]
- Fujii K., Tominaga M., Ohmori S., Kobayashi K., Koga T., Takata Y., Fujishima M. Decreased endothelium-dependent hyperpolarization to acetylcholine in smooth muscle of the mesenteric artery of spontaneously hypertensive rats. Circ Res. 1992 Apr;70(4):660–669. doi: 10.1161/01.res.70.4.660. [DOI] [PubMed] [Google Scholar]
- Garland C. J., Plane F., Kemp B. K., Cocks T. M. Endothelium-dependent hyperpolarization: a role in the control of vascular tone. Trends Pharmacol Sci. 1995 Jan;16(1):23–30. doi: 10.1016/s0165-6147(00)88969-5. [DOI] [PubMed] [Google Scholar]
- Harder D. R., Campbell W. B., Roman R. J. Role of cytochrome P-450 enzymes and metabolites of arachidonic acid in the control of vascular tone. J Vasc Res. 1995 Mar-Apr;32(2):79–92. doi: 10.1159/000159080. [DOI] [PubMed] [Google Scholar]
- Hirst G. D., Neild T. O. An analysis of excitatory junctional potentials recorded from arterioles. J Physiol. 1978 Jul;280:87–104. doi: 10.1113/jphysiol.1978.sp012374. [DOI] [PMC free article] [PubMed] [Google Scholar]
- Ignarro L. J., Harbison R. G., Wood K. S., Wolin M. S., McNamara D. B., Hyman A. L., Kadowitz P. J. Differences in responsiveness of intrapulmonary artery and vein to arachidonic acid: mechanism of arterial relaxation involves cyclic guanosine 3':5'-monophosphate and cyclic adenosine 3':5'-monophosphate. J Pharmacol Exp Ther. 1985 Jun;233(3):560–569. [PubMed] [Google Scholar]
- Kajiwara M. General features of electrical and mechanical properties of smooth muscle cells in the guinea-pig abdominal aorta. Pflugers Arch. 1982 Mar;393(1):109–117. doi: 10.1007/BF00582402. [DOI] [PubMed] [Google Scholar]
- Kerwin J. F., Jr, Lancaster J. R., Jr, Feldman P. L. Nitric oxide: a new paradigm for second messengers. J Med Chem. 1995 Oct 27;38(22):4343–4362. doi: 10.1021/jm00022a001. [DOI] [PubMed] [Google Scholar]
- Kuriyama H., Kitamura K., Nabata H. Pharmacological and physiological significance of ion channels and factors that modulate them in vascular tissues. Pharmacol Rev. 1995 Sep;47(3):387–573. [PubMed] [Google Scholar]
- Mekata F. Electrophysiological properties of the smooth muscle cell membrane of the dog coronary artery. J Physiol. 1980 Jan;298:205–212. doi: 10.1113/jphysiol.1980.sp013076. [DOI] [PMC free article] [PubMed] [Google Scholar]
- Miyoshi H., Nakaya Y. Endotoxin-induced nonendothelial nitric oxide activates the Ca(2+)-activated K+ channel in cultured vascular smooth muscle cells. J Mol Cell Cardiol. 1994 Nov;26(11):1487–1495. doi: 10.1006/jmcc.1994.1167. [DOI] [PubMed] [Google Scholar]
- Miyoshi H., Nakaya Y., Moritoki H. Nonendothelial-derived nitric oxide activates the ATP-sensitive K+ channel of vascular smooth muscle cells. FEBS Lett. 1994 May 23;345(1):47–49. doi: 10.1016/0014-5793(94)00417-x. [DOI] [PubMed] [Google Scholar]
- Mulvany M. J., Aalkjaer C. Structure and function of small arteries. Physiol Rev. 1990 Oct;70(4):921–961. doi: 10.1152/physrev.1990.70.4.921. [DOI] [PubMed] [Google Scholar]
- Murphy M. E., Brayden J. E. Nitric oxide hyperpolarizes rabbit mesenteric arteries via ATP-sensitive potassium channels. J Physiol. 1995 Jul 1;486(Pt 1):47–58. doi: 10.1113/jphysiol.1995.sp020789. [DOI] [PMC free article] [PubMed] [Google Scholar]
- Nagao T., Vanhoutte P. M. Hyperpolarization as a mechanism for endothelium-dependent relaxations in the porcine coronary artery. J Physiol. 1992 Jan;445:355–367. doi: 10.1113/jphysiol.1992.sp018928. [DOI] [PMC free article] [PubMed] [Google Scholar]
- Parkington H. C., Tonta M. A., Coleman H. A., Tare M. Role of membrane potential in endothelium-dependent relaxation of guinea-pig coronary arterial smooth muscle. J Physiol. 1995 Apr 15;484(Pt 2):469–480. doi: 10.1113/jphysiol.1995.sp020679. [DOI] [PMC free article] [PubMed] [Google Scholar]
- Plane F., Garland C. J. Differential effects of acetylcholine, nitric oxide and levcromakalim on smooth muscle membrane potential and tone in the rabbit basilar artery. Br J Pharmacol. 1993 Oct;110(2):651–656. doi: 10.1111/j.1476-5381.1993.tb13861.x. [DOI] [PMC free article] [PubMed] [Google Scholar]
- Quayle J. M., Standen N. B. KATP channels in vascular smooth muscle. Cardiovasc Res. 1994 Jun;28(6):797–804. doi: 10.1093/cvr/28.6.797. [DOI] [PubMed] [Google Scholar]
- Rand V. E., Garland C. J. Endothelium-dependent relaxation to acetylcholine in the rabbit basilar artery: importance of membrane hyperpolarization. Br J Pharmacol. 1992 May;106(1):143–150. doi: 10.1111/j.1476-5381.1992.tb14307.x. [DOI] [PMC free article] [PubMed] [Google Scholar]
- Schubert R., Wesselman J. P., Nilsson H., Mulvany M. J. Noradrenaline-induced depolarization is smaller in isobaric compared to isometric preparations of rat mesenteric small arteries. Pflugers Arch. 1996 Mar;431(5):794–796. doi: 10.1007/BF02253846. [DOI] [PubMed] [Google Scholar]
- Surprenant A., Neild T. O., Holman M. E. Membrane properties of rabbit basilar arteries and their responses to transmural stimulation. Pflugers Arch. 1987 Sep;410(1-2):92–101. doi: 10.1007/BF00581901. [DOI] [PubMed] [Google Scholar]
- Tang Y. G., Zheng Y. F. Endothelium-derived relaxing factor activates calcium-activated potassium channels of resistance vessel smooth muscle cells. Sci China B. 1993 Apr;36(4):439–450. [PubMed] [Google Scholar]
- Tare M., Parkington H. C., Coleman H. A., Neild T. O., Dusting G. J. Hyperpolarization and relaxation of arterial smooth muscle caused by nitric oxide derived from the endothelium. Nature. 1990 Jul 5;346(6279):69–71. doi: 10.1038/346069a0. [DOI] [PubMed] [Google Scholar]
- Taylor S. G., Southerton J. S., Weston A. H., Baker J. R. Endothelium-dependent effects of acetylcholine in rat aorta: a comparison with sodium nitroprusside and cromakalim. Br J Pharmacol. 1988 Jul;94(3):853–863. doi: 10.1111/j.1476-5381.1988.tb11597.x. [DOI] [PMC free article] [PubMed] [Google Scholar]
- Tomita T. Electrophysiology of mammalian smooth muscle. Prog Biophys Mol Biol. 1975;30(2-3):185–203. doi: 10.1016/0079-6107(76)90009-2. [DOI] [PubMed] [Google Scholar]
- Van de Voorde J., Vanheel B., Leusen I. Endothelium-dependent relaxation and hyperpolarization in aorta from control and renal hypertensive rats. Circ Res. 1992 Jan;70(1):1–8. doi: 10.1161/01.res.70.1.1. [DOI] [PubMed] [Google Scholar]
- Vanheel B., Van de Voorde J., Leusen I. Contribution of nitric oxide to the endothelium-dependent hyperpolarization in rat aorta. J Physiol. 1994 Mar 1;475(2):277–284. doi: 10.1113/jphysiol.1994.sp020068. [DOI] [PMC free article] [PubMed] [Google Scholar]