Abstract
1. The effects of synapses established by smooth dendritic neurones on pyramidal and spiny stellate cells were studied in areas 17 and 18 of the cat visual cortex in vitro. Paired intracellular recordings with biocytin-filled electrodes and subsequent light and electron microscopic analysis were used to determine the sites of synaptic interaction. 2. All smooth dendritic cells established type II synapses previously shown to be made by terminals containing GABA, therefore the studied cells are probably GABAergic. Three classes of presynaptic cell could be defined, based on their efferent synaptic target preference determined from random samples of unlabelled postsynaptic cells. (a) Basket cells (n = 6) innervated mainly somata (49.9 +/- 13.8%) and dendritic shafts (45.2 +/- 10.7%) and, to a lesser extent, dendritic spines (4.9 +/- 4.6%). (b) Dendrite-targeting cells (n = 5) established synapses predominantly on dendritic shafts (84.3 +/- 9.4%) and less frequently on dendritic spines (11.2 +/- 6.7%) or somata (4.5 +/- 4.7%). (c) Double bouquet cells (n = 4) preferred dendritic spines (69.2 +/- 4.2%) to dendritic shafts (30.8 +/- 4.2%) as postsynaptic targets and avoided somata. 3. Interneurones formed 5240 +/- 1600 (range, 2830-9690) synaptic junctions in the slices. Based on the density of synapses made by single interneurones and the volume density of GABAergic synapses, it was calculated that an average interneurone provides 0.66 +/- 0.20% of the GABAergic synapses in its axonal field. 4. The location of synaptic junctions on individual, identified postsynaptic cells reflected the overall postsynaptic target distribution of the same GABAergic neurone. The number of synaptic junctions between pairs of neurones could not be predicted from light microscopic examination. The number of electron microscopically verified synaptic sites was generally smaller for the dendritic domain and larger for the somatic domain than expected from light microscopy. All presynaptic cells established multiple synaptic junctions on their postsynaptic target cells. A basket cell innervated a pyramidal cell via fifteen release sites; the numbers of synapses formed by three dendrite-targeting cells on pyramidal cells were seventeen and eight respectively, and three on a spiny stellate cell; the interaction between a double bouquet cell and a postsynaptic pyramidal cell was mediated by ten synaptic junctions. 5. All three types of interneurone (n = 6; 2 for each type of cell) elicited short-latency IPSPs with fast rise time (10-90%; 2.59 +/- 1.02 ms) and short duration (at half-amplitude, 15.82 +/- 5.24 ms), similar to those mediated by GABAA receptors. 6. Average amplitudes of unitary IPSPs (n = 6) were 845 +/- 796 microV (range, 134-2265 microV). Variability of IPSP amplitude was moderate, the average ratio of IPSP and baseline noise variance was 1.54 +/- 0.96. High frequency activation of single presynaptic dendrite-targeting cells led to an initial summation followed by use-dependent depression of the averaged postsynaptic response. Double bouquet cell-evoked IPSPs, recorded in the soma, had a smaller amplitude than those evoked by the other two cell types. In all connections, transmission failures were rare or absent, particularly when mediated by a high number of release sites. 7. The results demonstrate that different types of neocortical GABAergic neurones innervate distinct domains on the surface of their postsynaptic target cells. Nevertheless, all three types of cell studied here elicit fast IPSPs and provide GABAergic input through multiple synaptic release sites with few, if any, failures of transmission.
Full text
PDF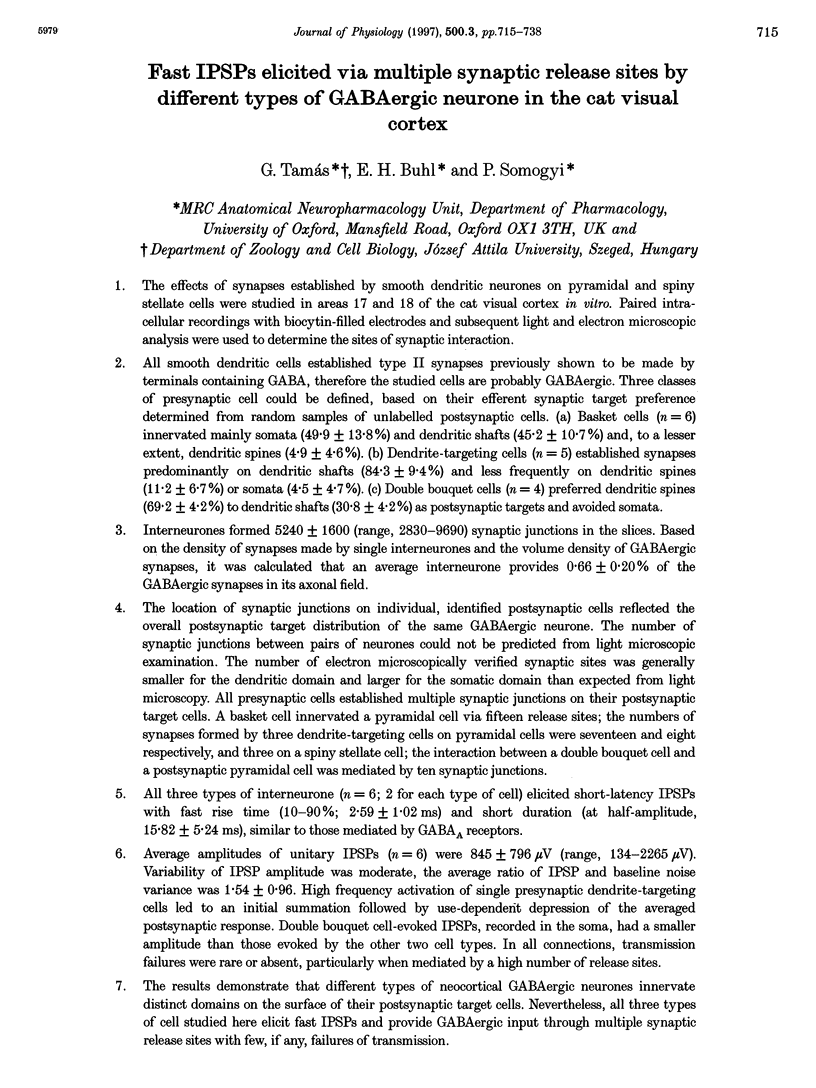
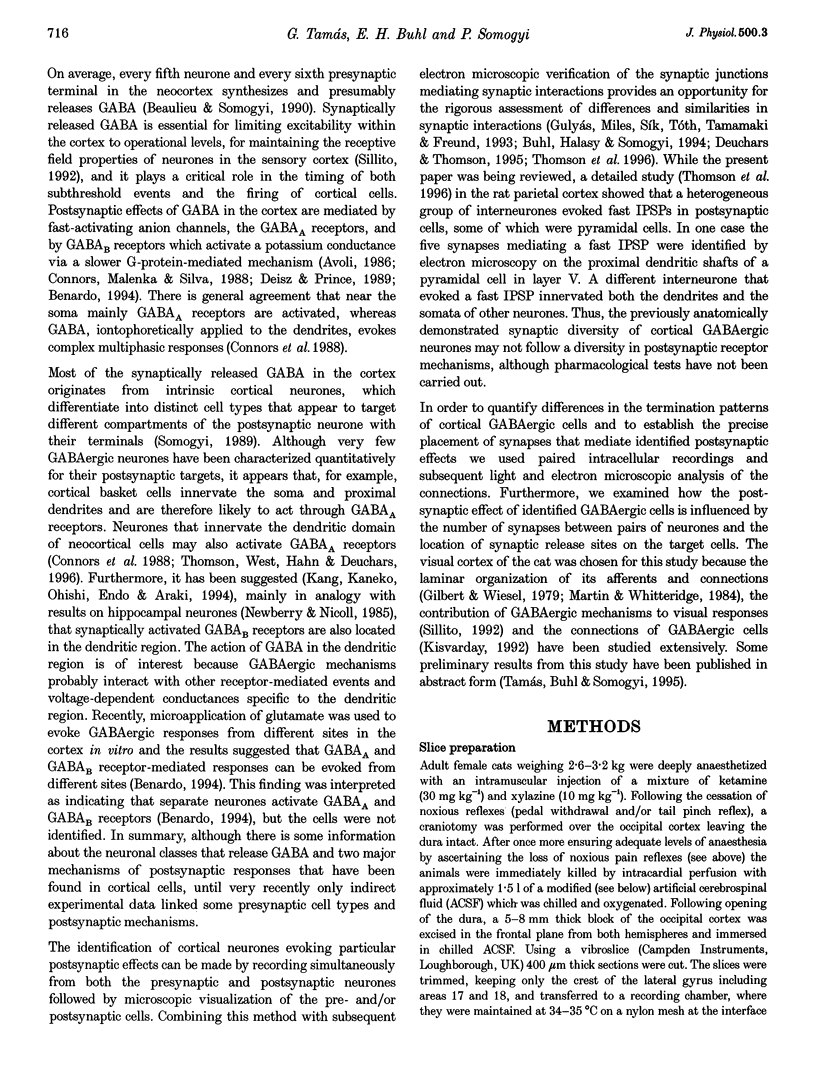
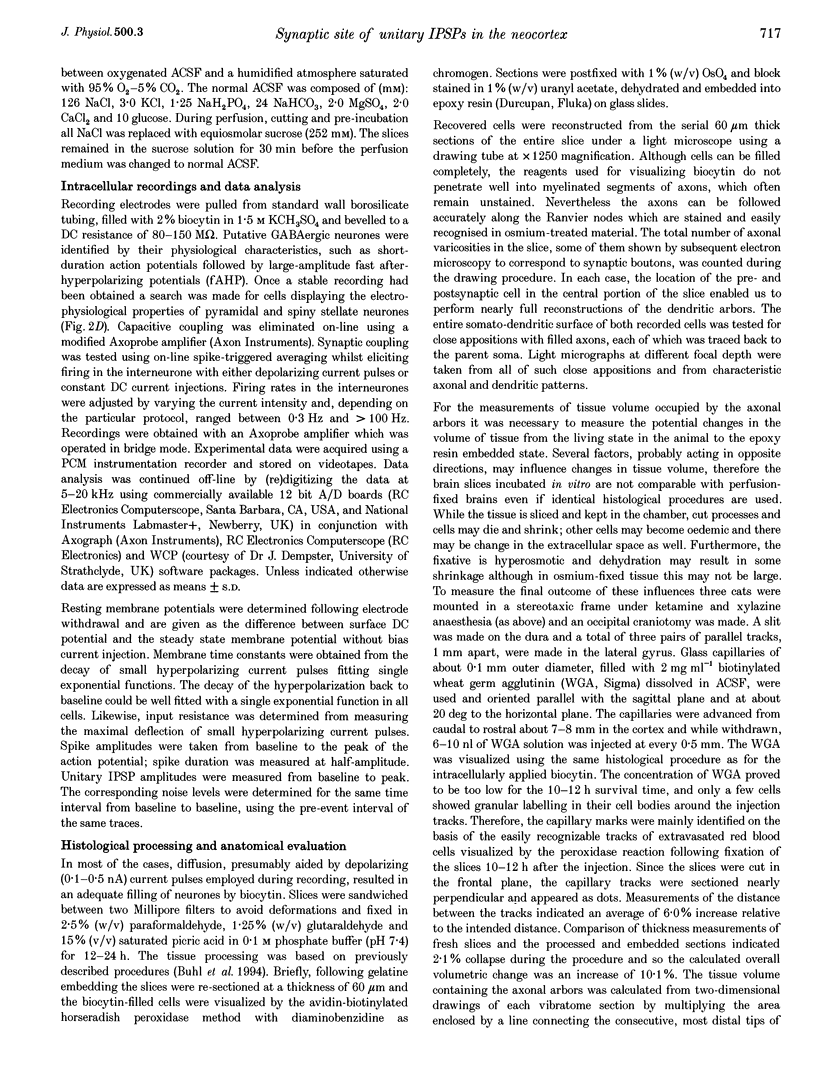
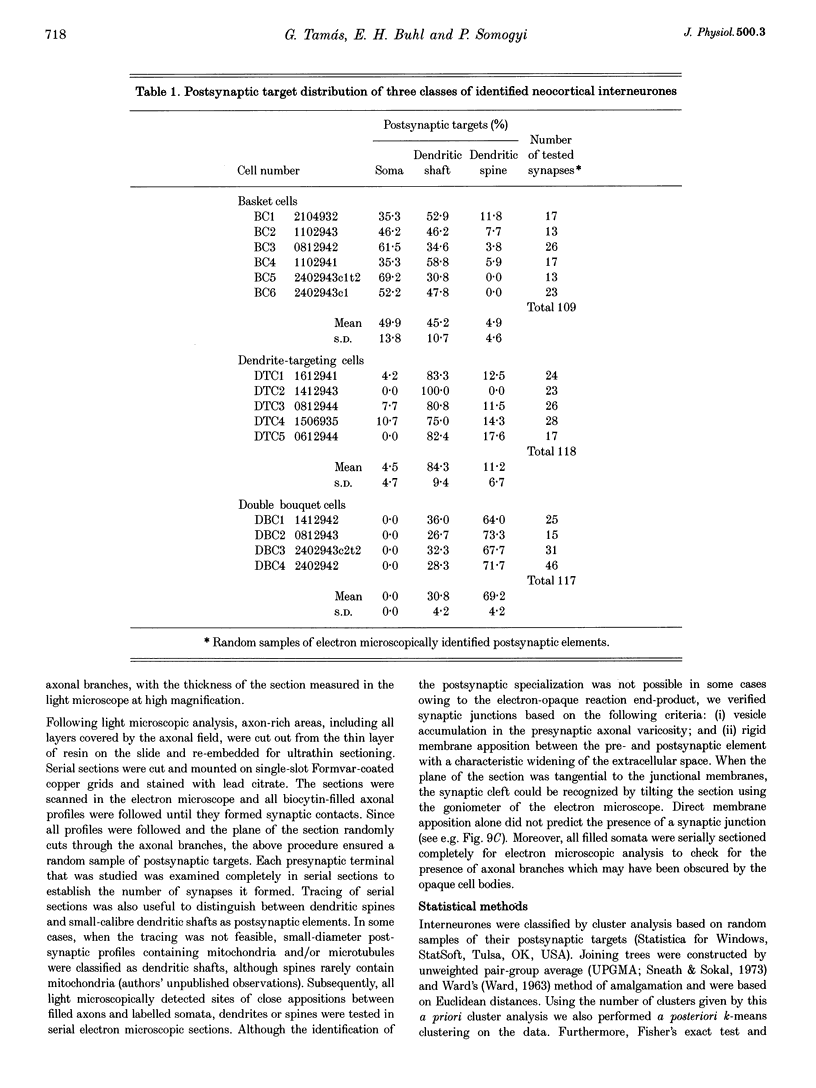
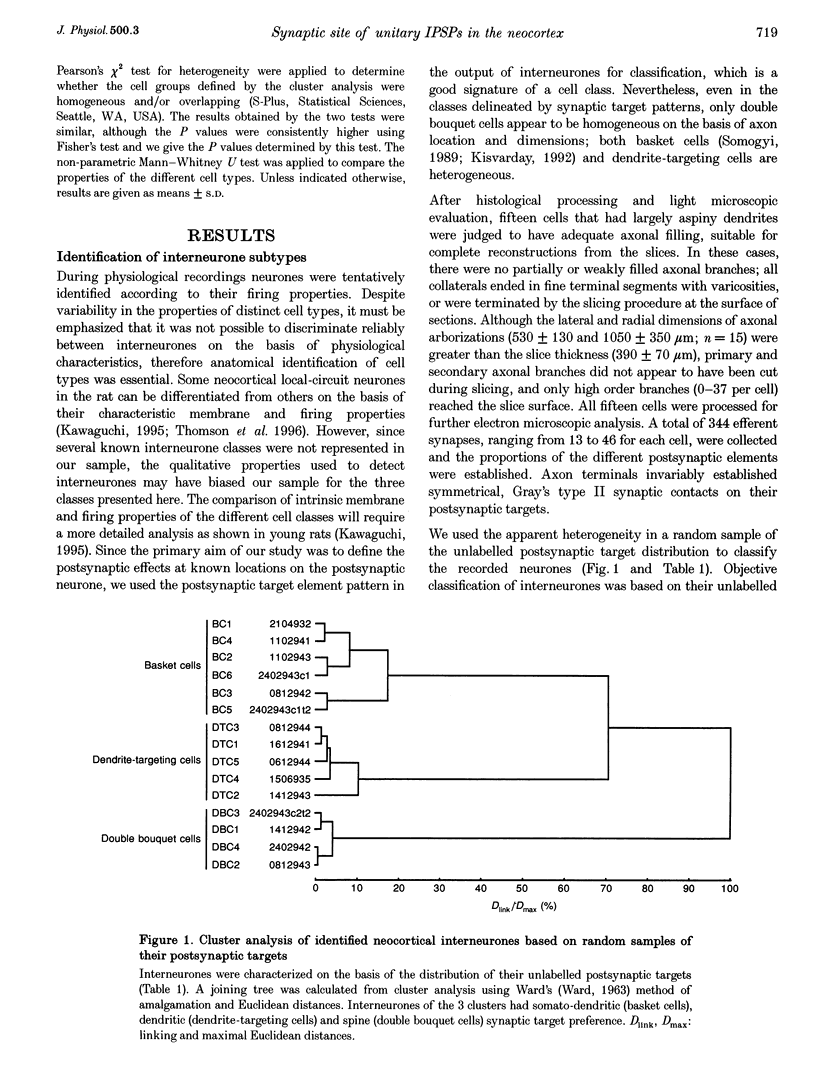
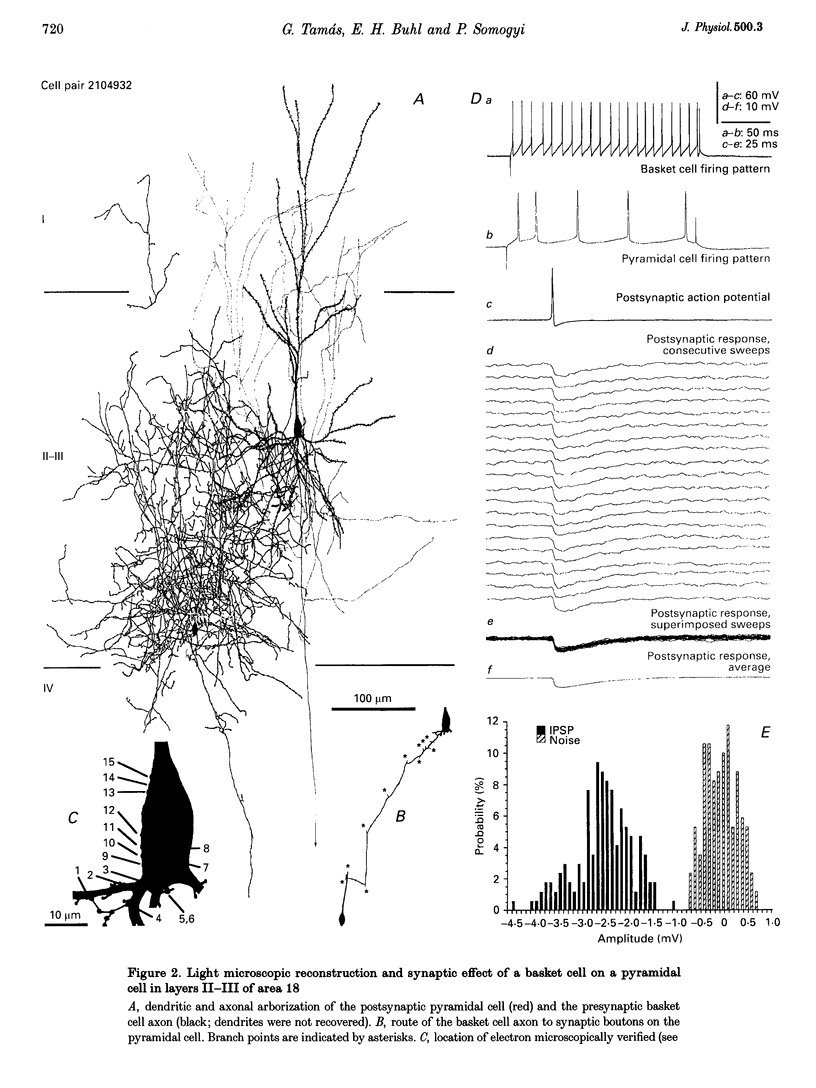
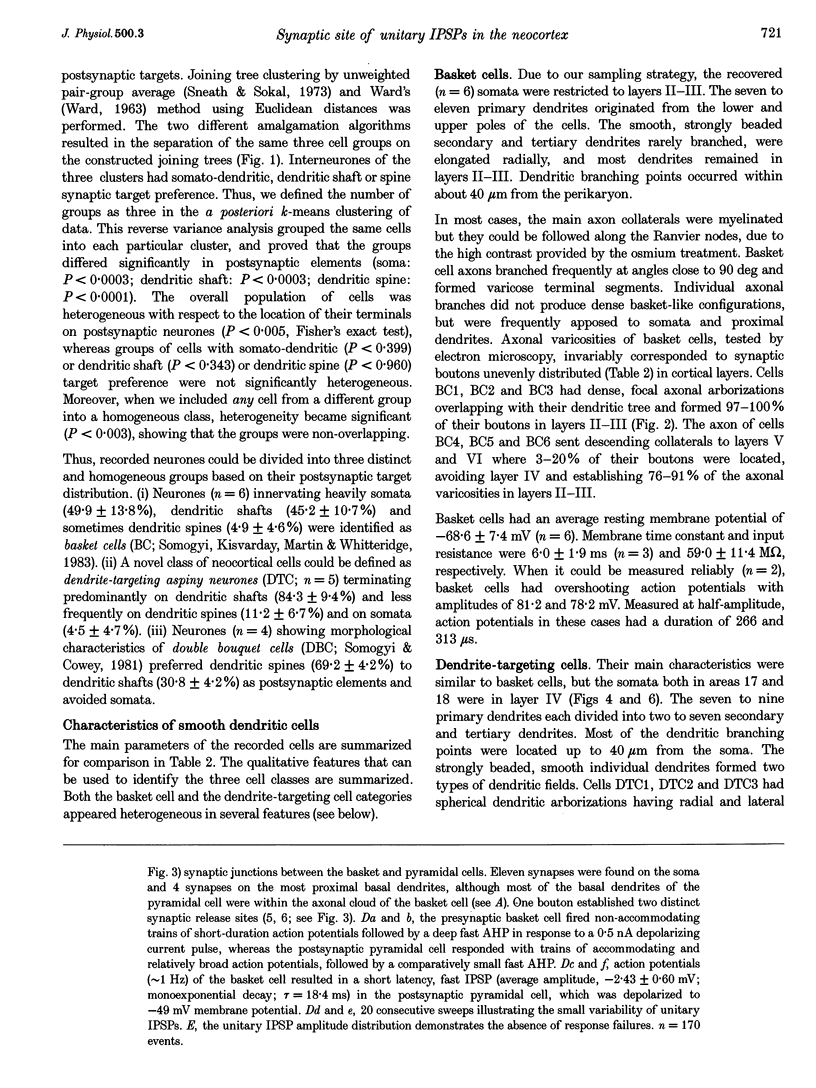
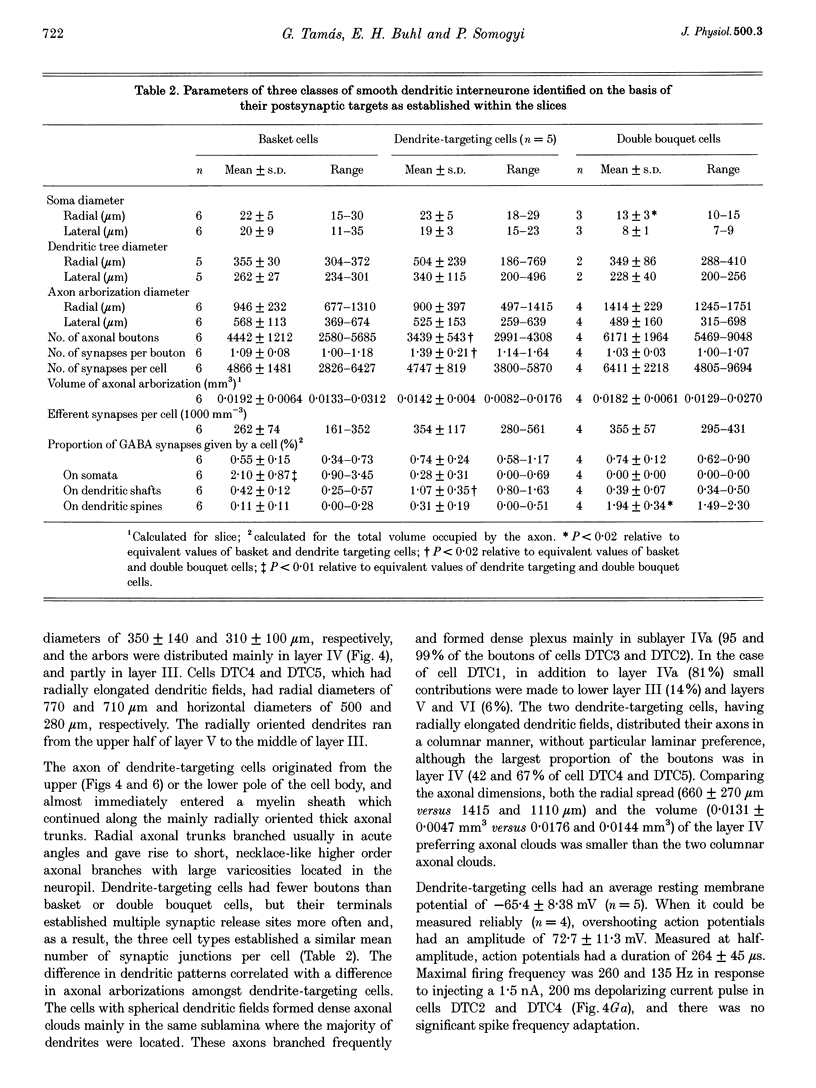
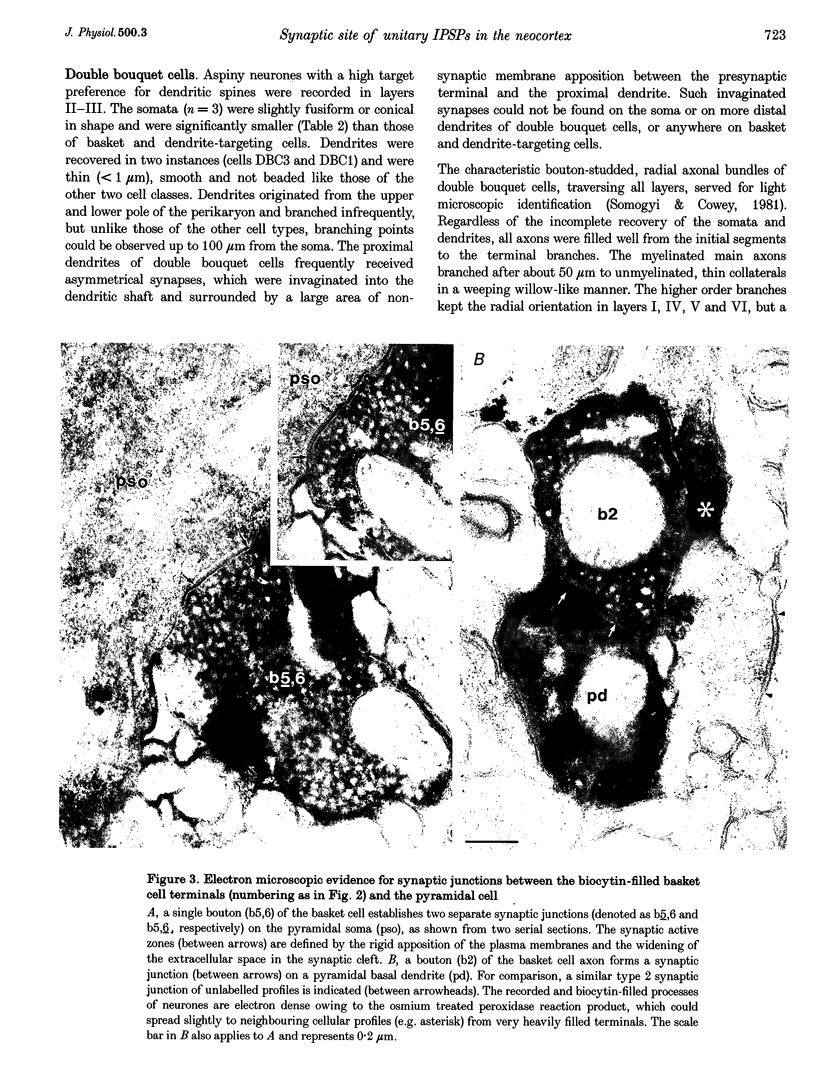
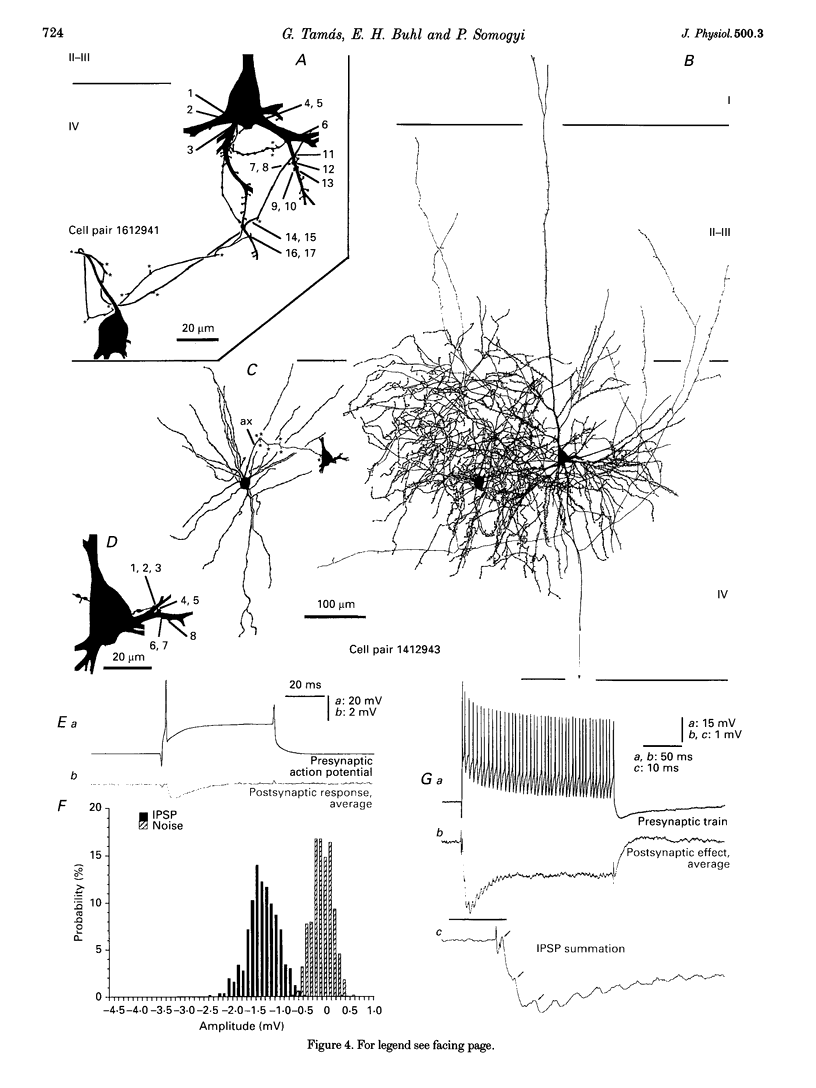
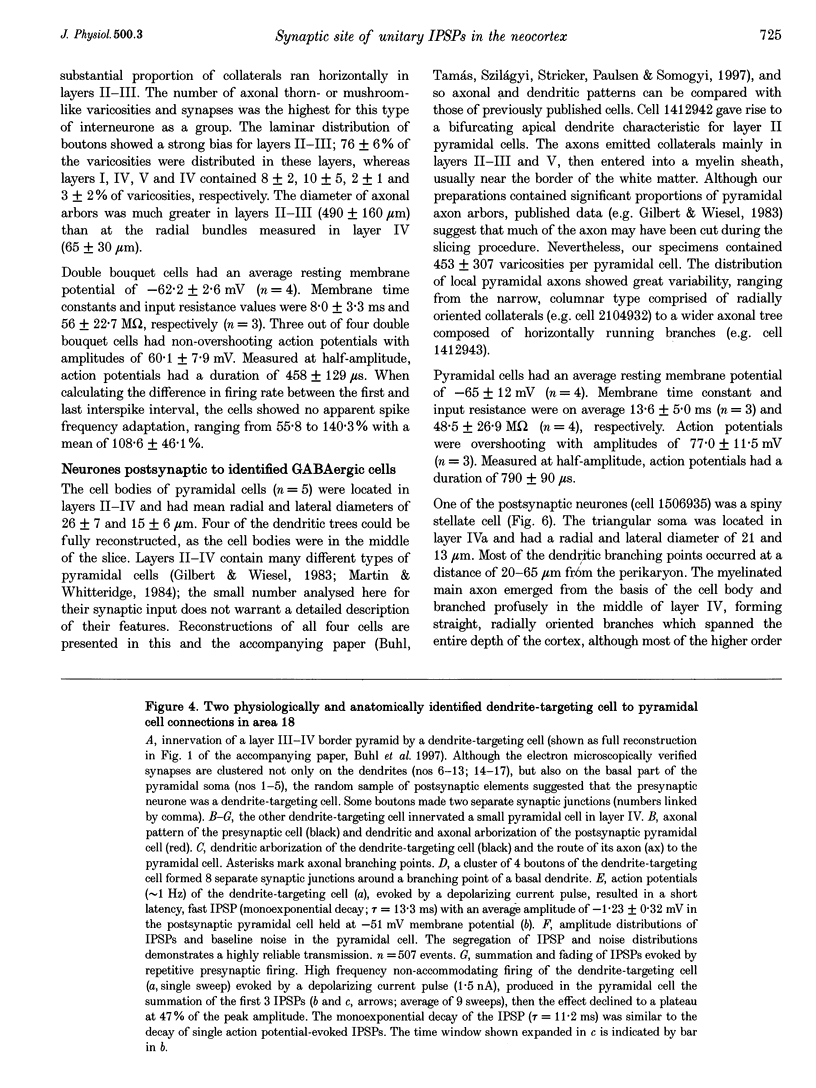
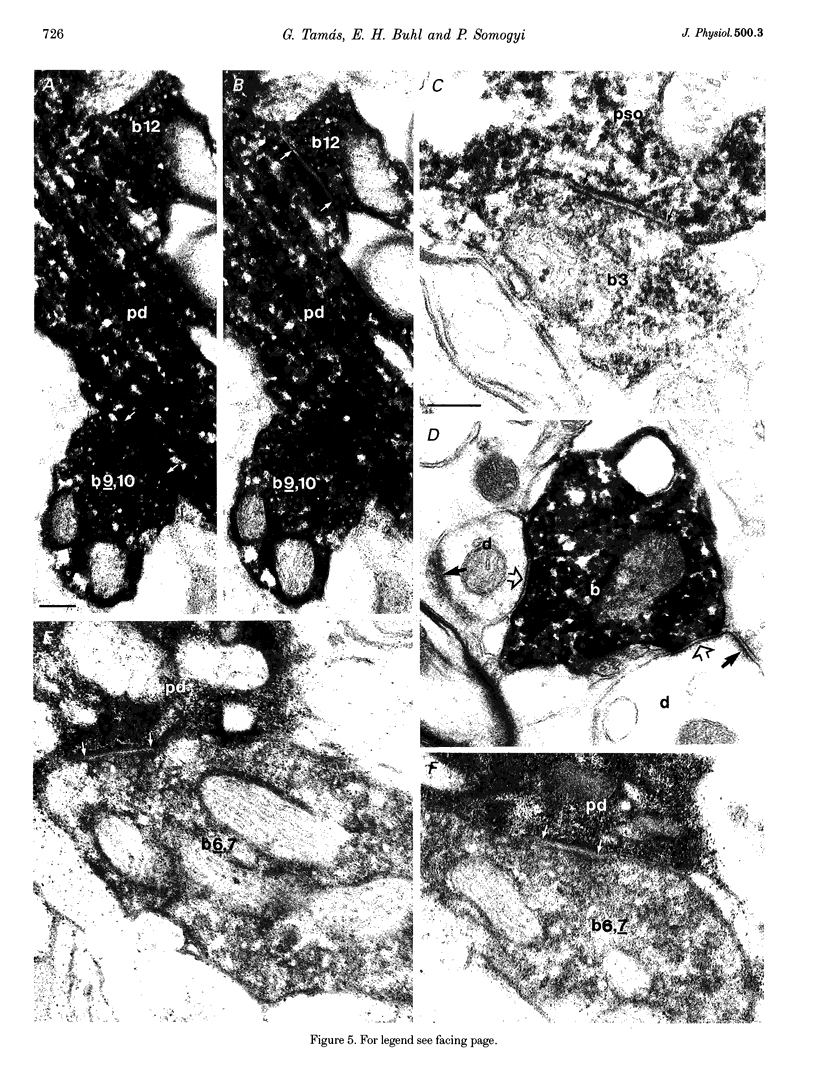
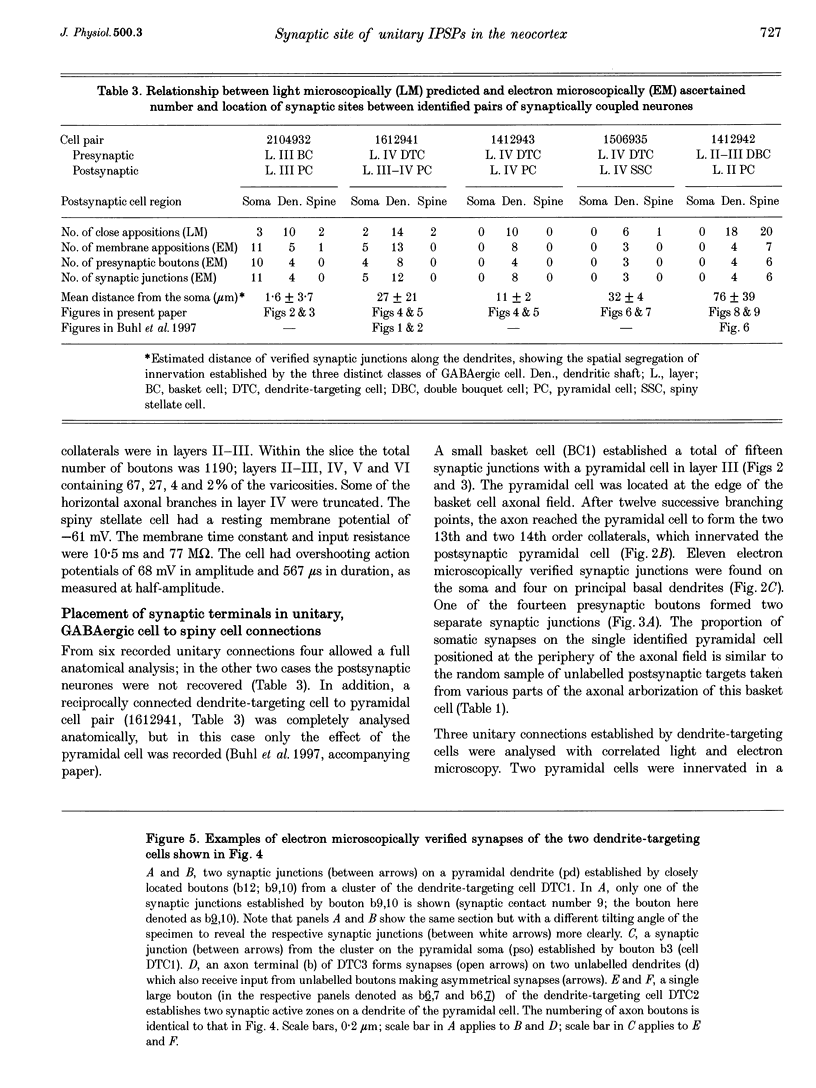
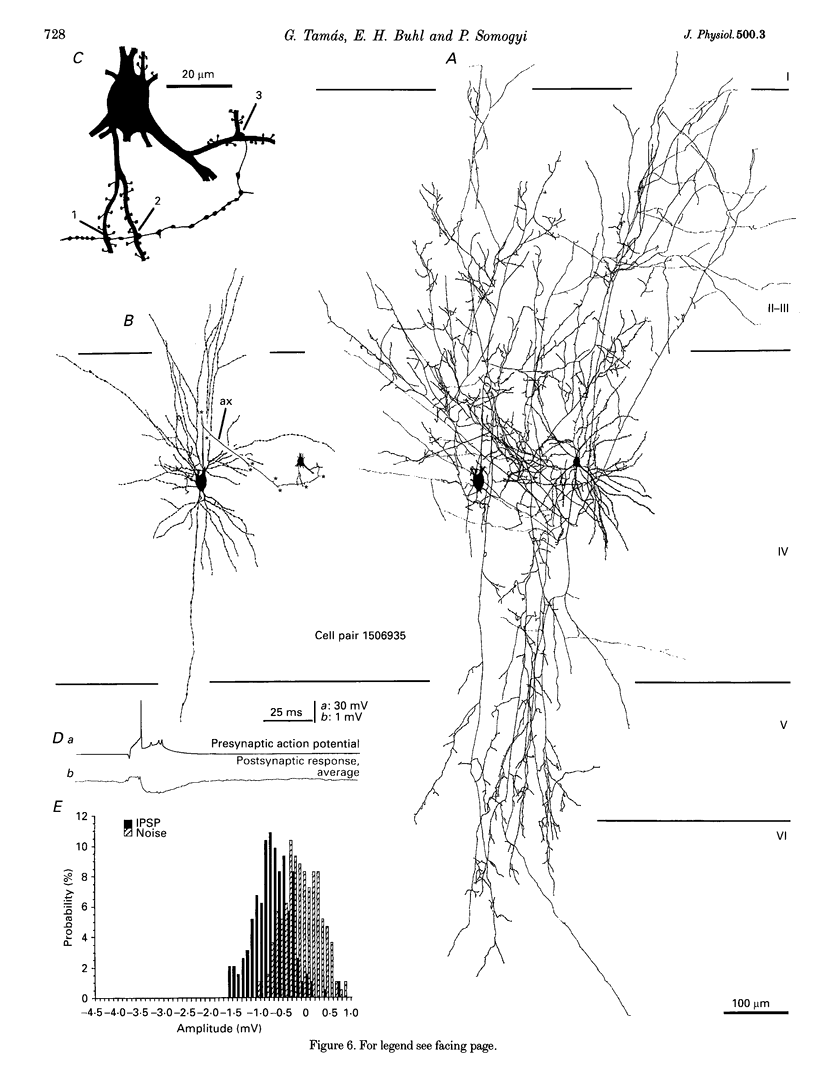
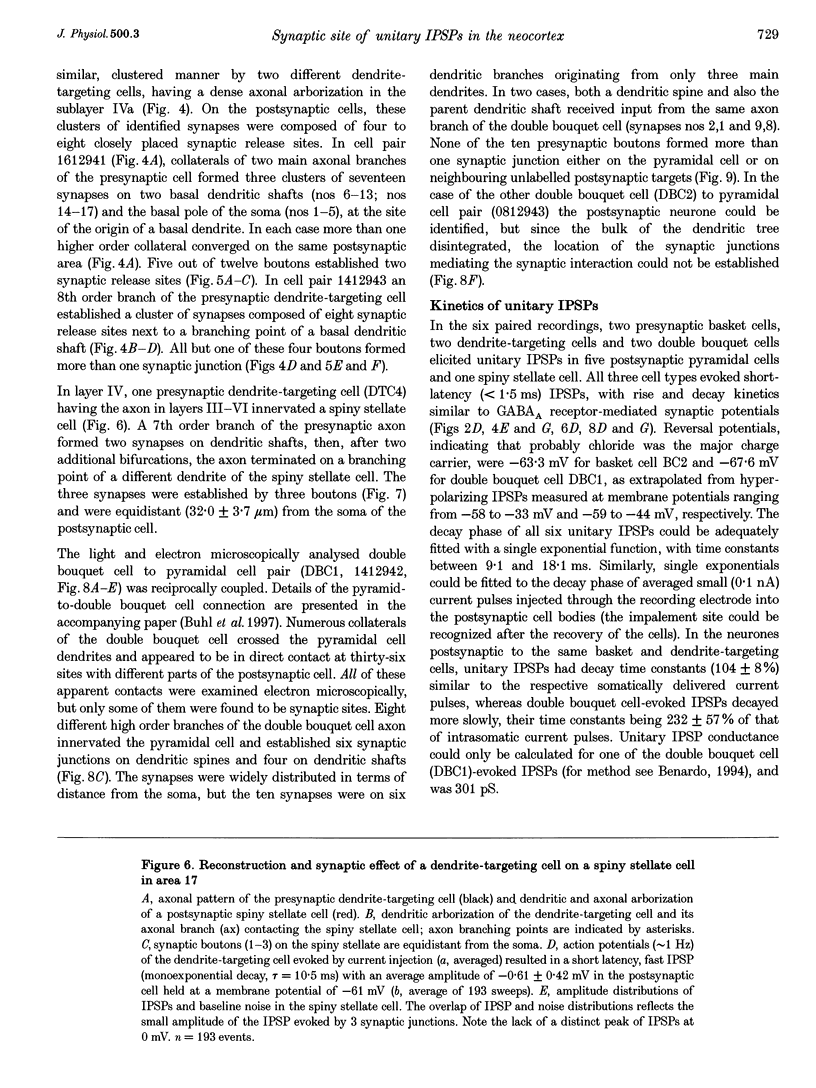
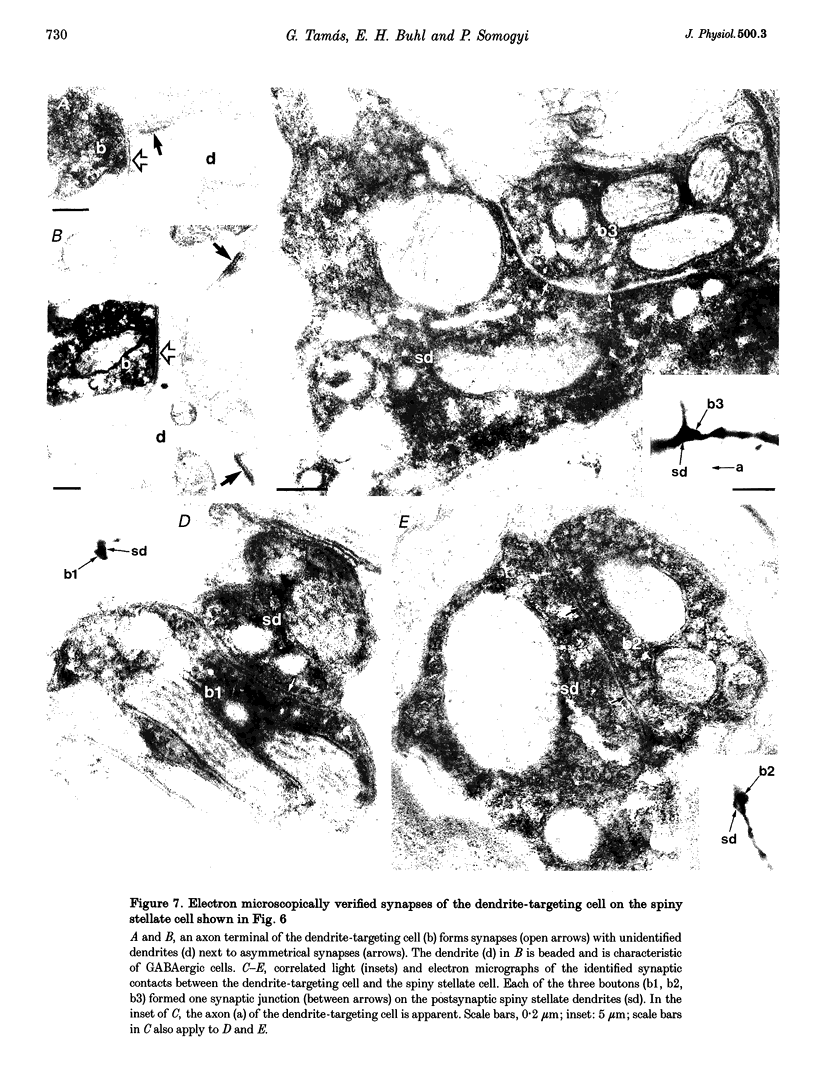
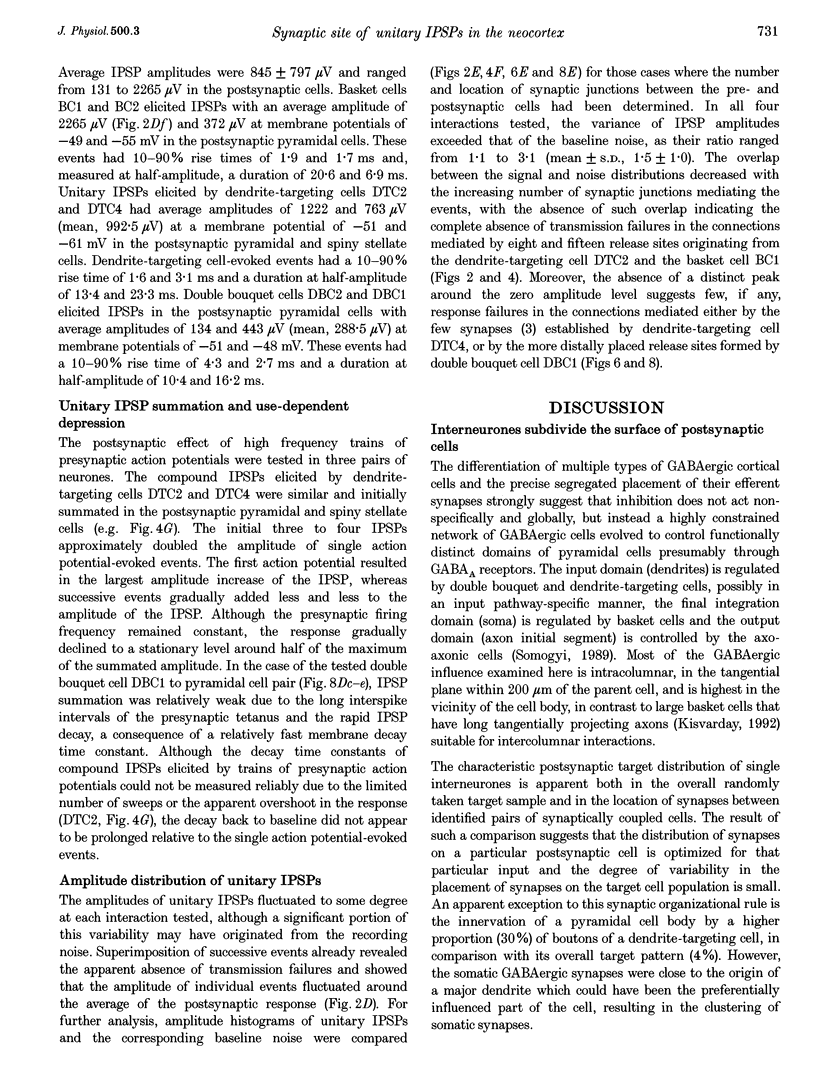
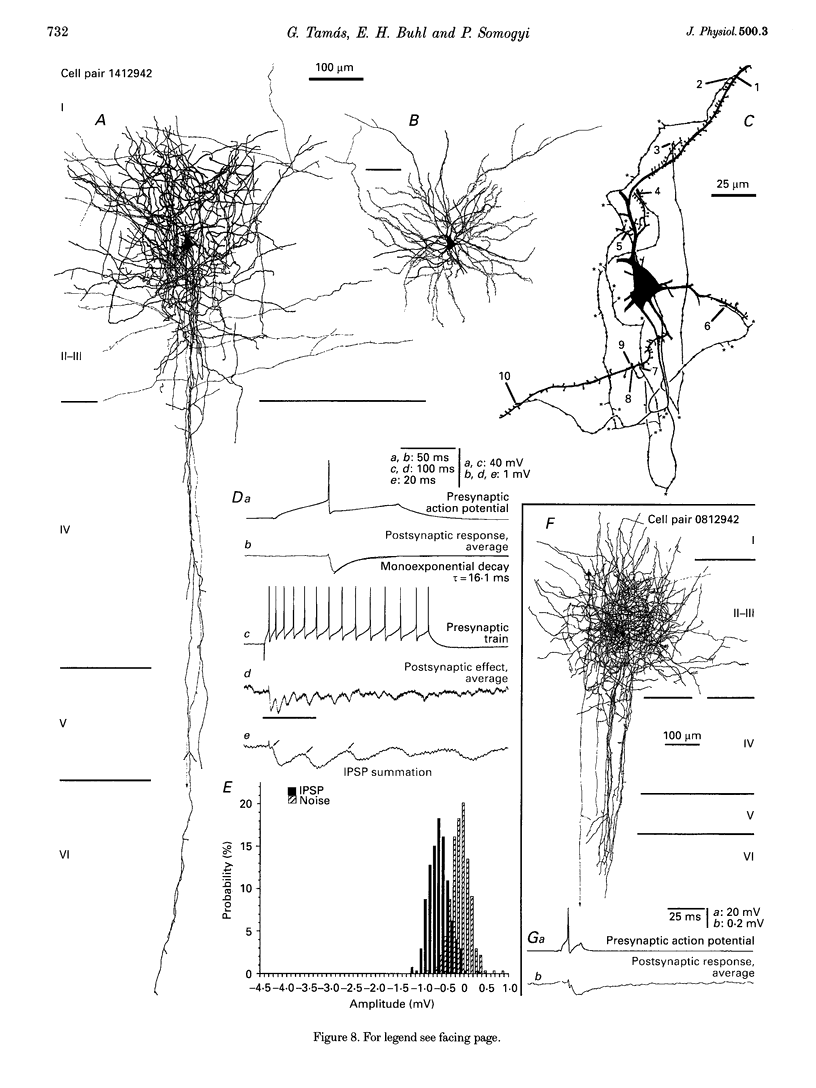
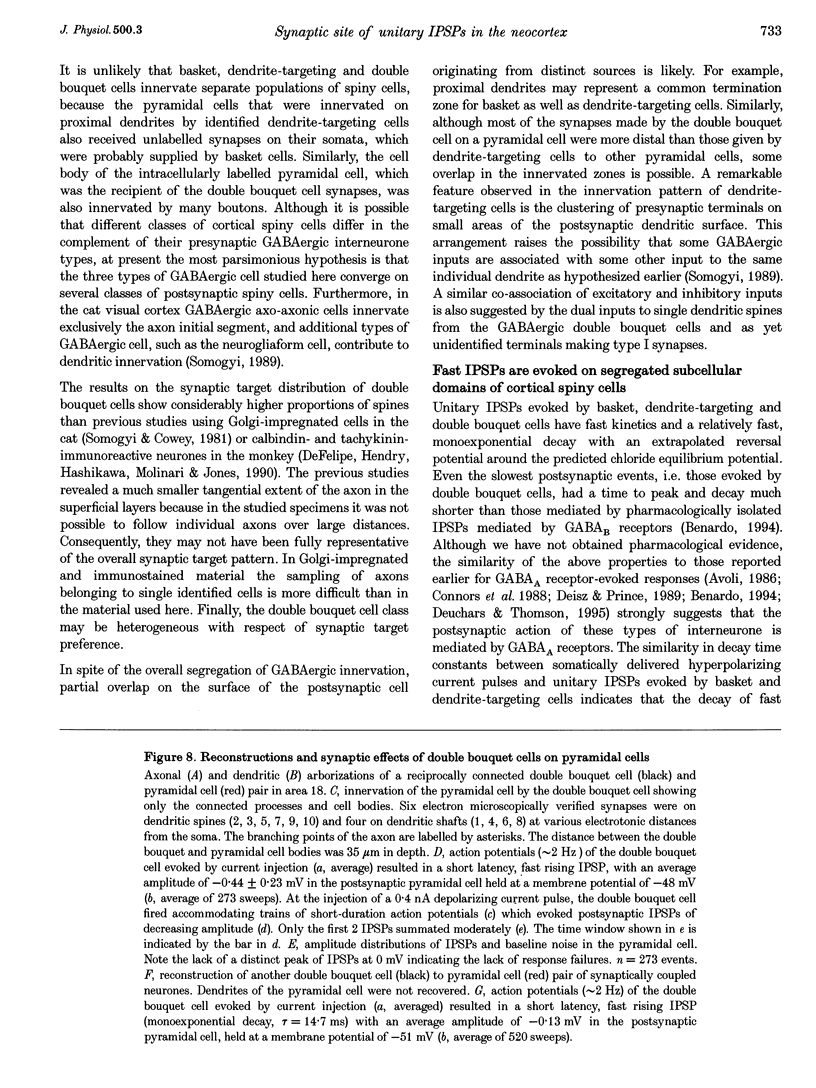
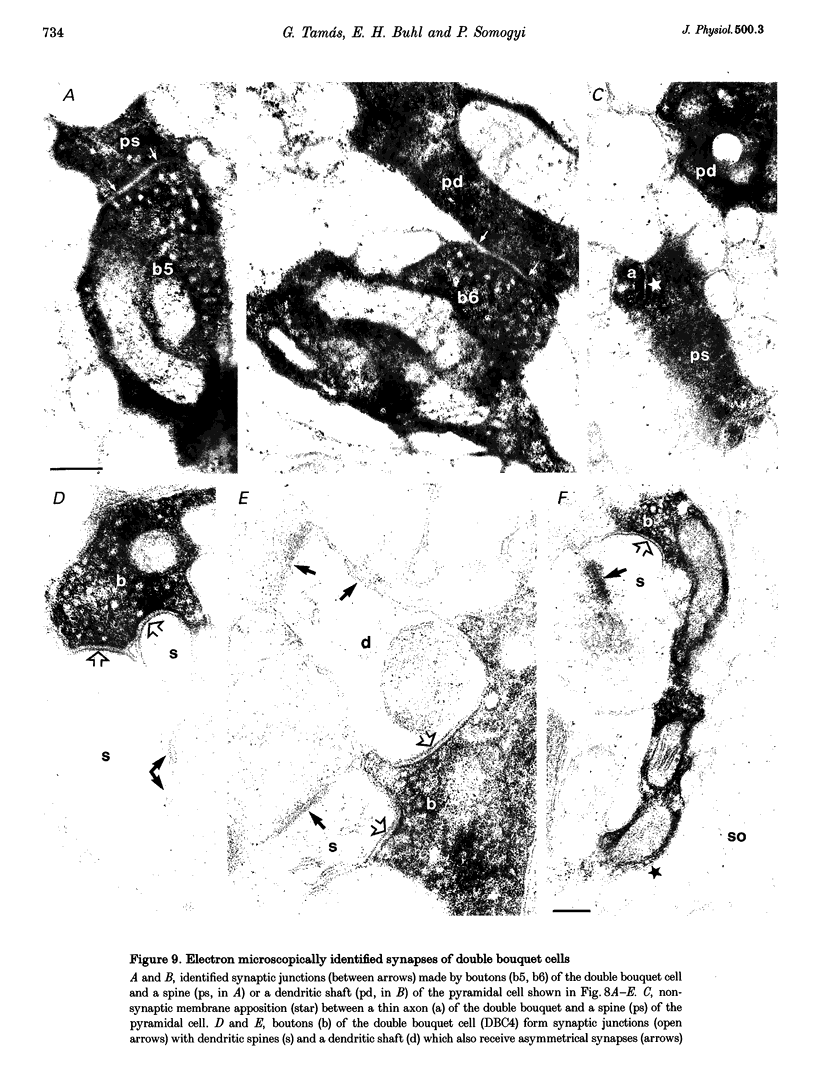
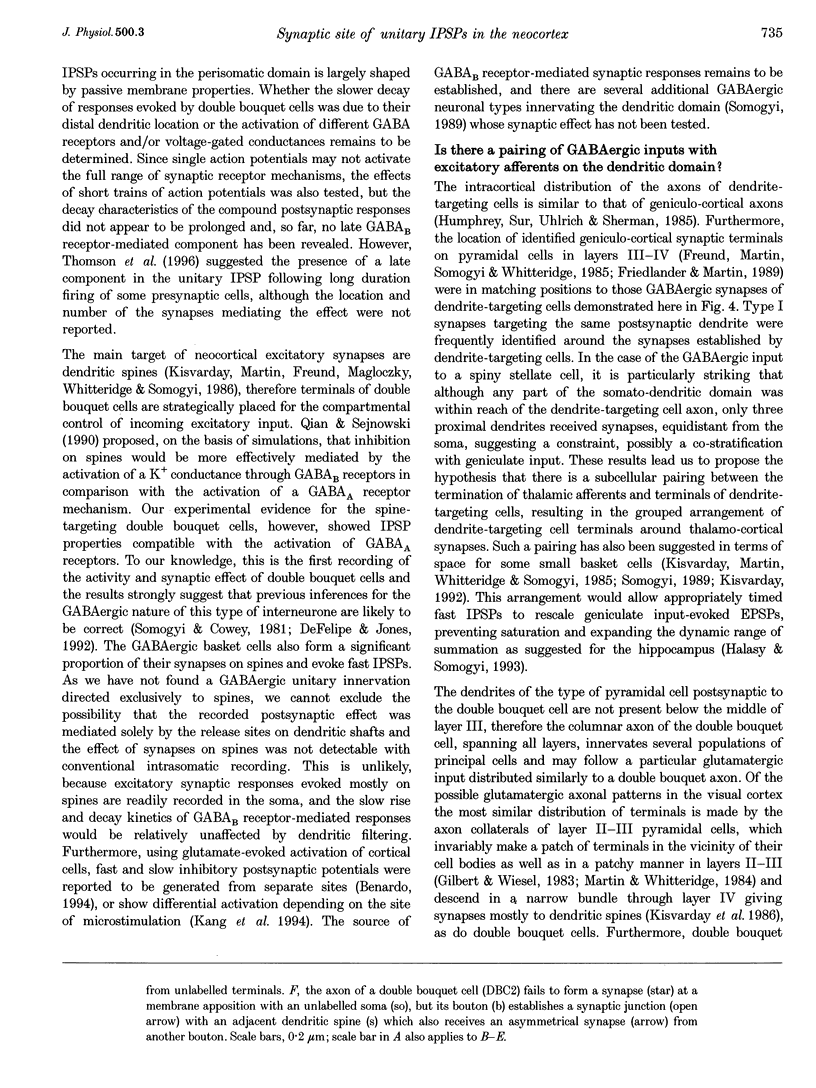
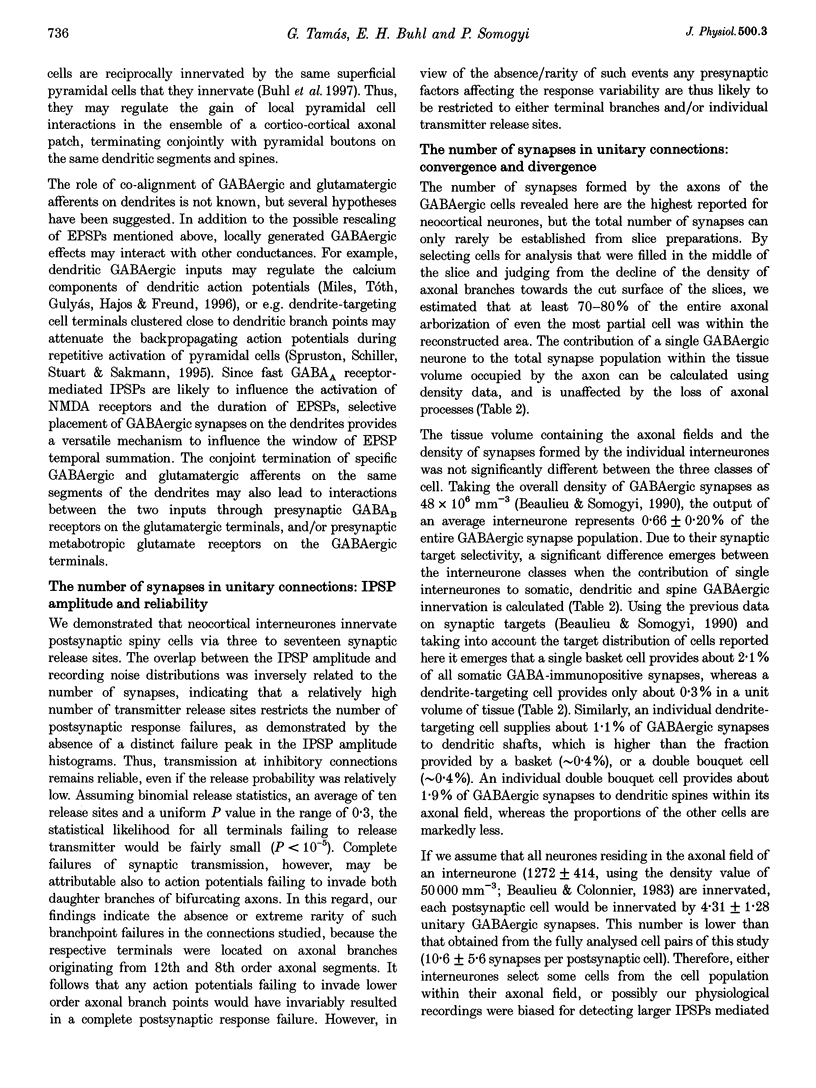
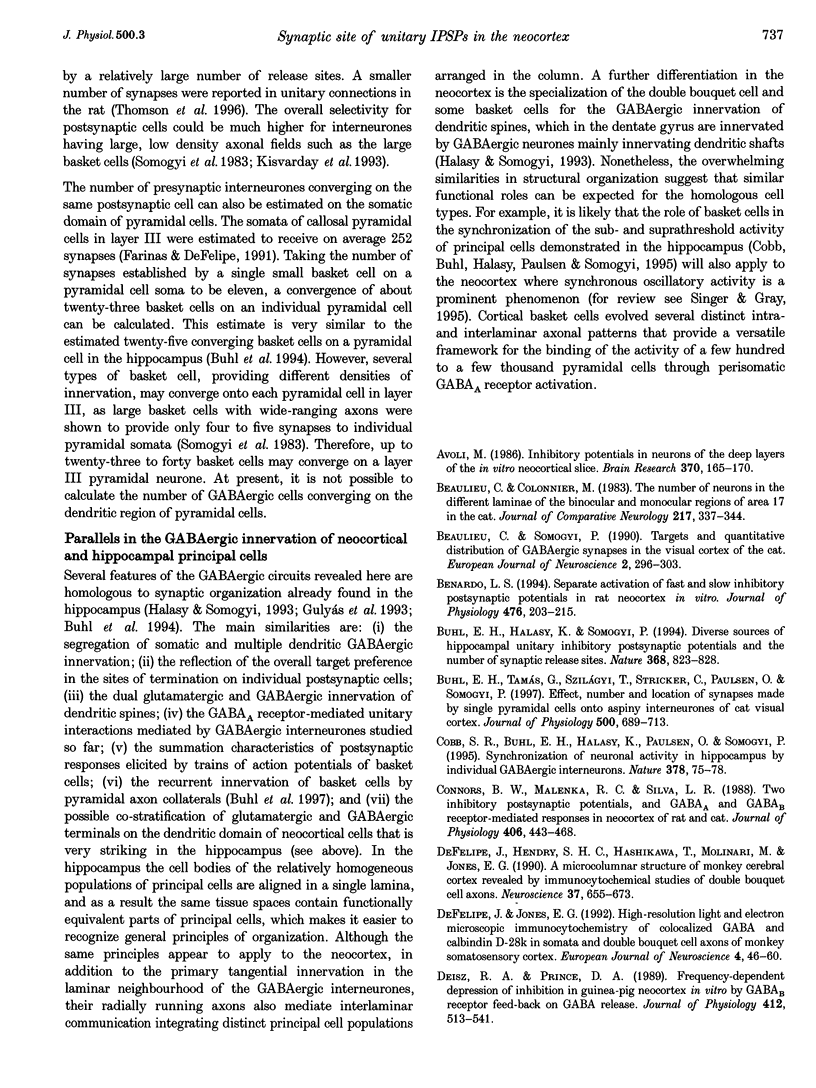
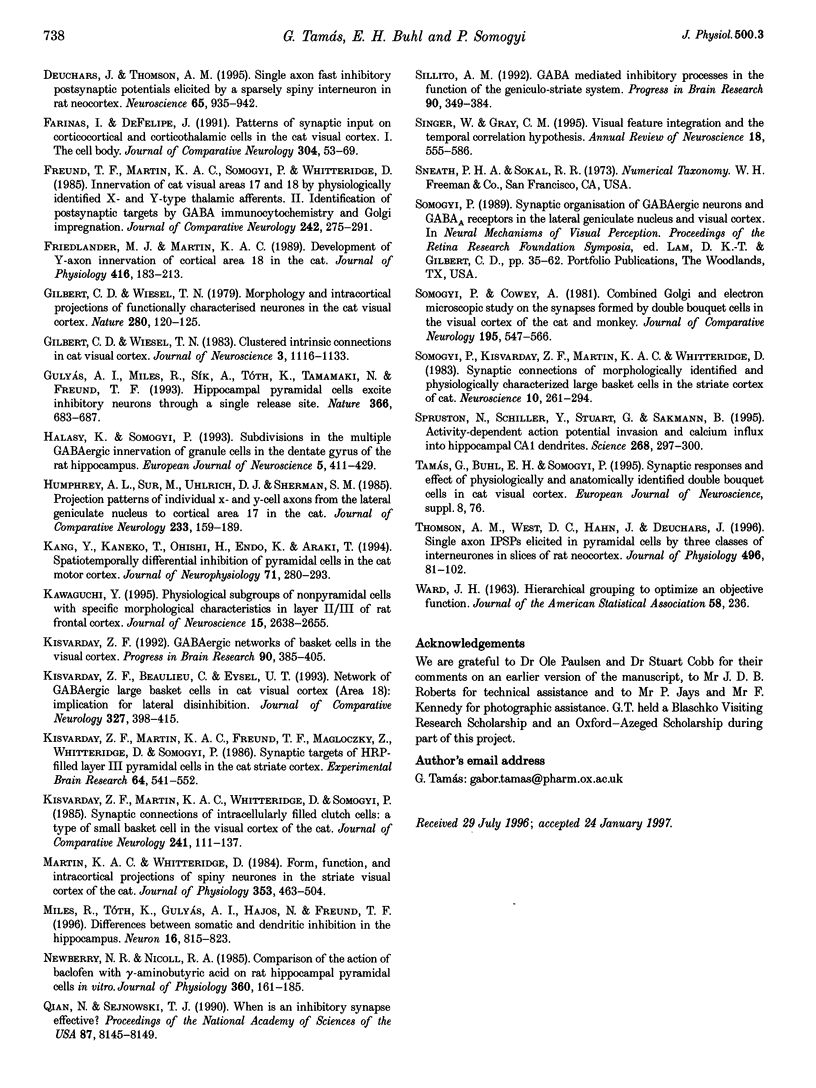
Images in this article
Selected References
These references are in PubMed. This may not be the complete list of references from this article.
- Avoli M. Inhibitory potentials in neurons of the deep layers of the in vitro neocortical slice. Brain Res. 1986 Apr 2;370(1):165–170. doi: 10.1016/0006-8993(86)91118-2. [DOI] [PubMed] [Google Scholar]
- Beaulieu C., Colonnier M. The number of neurons in the different laminae of the binocular and monocular regions of area 17 in the cat, Canada. J Comp Neurol. 1983 Jul 1;217(3):337–344. doi: 10.1002/cne.902170308. [DOI] [PubMed] [Google Scholar]
- Beaulieu C., Somogyi P. Targets and Quantitative Distribution of GABAergic Synapses in the Visual Cortex of the Cat. Eur J Neurosci. 1990;2(4):296–303. doi: 10.1111/j.1460-9568.1990.tb00421.x. [DOI] [PubMed] [Google Scholar]
- Benardo L. S. Separate activation of fast and slow inhibitory postsynaptic potentials in rat neocortex in vitro. J Physiol. 1994 Apr 15;476(2):203–215. doi: 10.1113/jphysiol.1994.sp020124. [DOI] [PMC free article] [PubMed] [Google Scholar]
- Buhl E. H., Halasy K., Somogyi P. Diverse sources of hippocampal unitary inhibitory postsynaptic potentials and the number of synaptic release sites. Nature. 1994 Apr 28;368(6474):823–828. doi: 10.1038/368823a0. [DOI] [PubMed] [Google Scholar]
- Buhl E. H., Tamás G., Szilágyi T., Stricker C., Paulsen O., Somogyi P. Effect, number and location of synapses made by single pyramidal cells onto aspiny interneurones of cat visual cortex. J Physiol. 1997 May 1;500(Pt 3):689–713. doi: 10.1113/jphysiol.1997.sp022053. [DOI] [PMC free article] [PubMed] [Google Scholar]
- Cobb S. R., Buhl E. H., Halasy K., Paulsen O., Somogyi P. Synchronization of neuronal activity in hippocampus by individual GABAergic interneurons. Nature. 1995 Nov 2;378(6552):75–78. doi: 10.1038/378075a0. [DOI] [PubMed] [Google Scholar]
- Connors B. W., Malenka R. C., Silva L. R. Two inhibitory postsynaptic potentials, and GABAA and GABAB receptor-mediated responses in neocortex of rat and cat. J Physiol. 1988 Dec;406:443–468. doi: 10.1113/jphysiol.1988.sp017390. [DOI] [PMC free article] [PubMed] [Google Scholar]
- DeFelipe J., Hendry S. H., Hashikawa T., Molinari M., Jones E. G. A microcolumnar structure of monkey cerebral cortex revealed by immunocytochemical studies of double bouquet cell axons. Neuroscience. 1990;37(3):655–673. doi: 10.1016/0306-4522(90)90097-n. [DOI] [PubMed] [Google Scholar]
- DeFelipe J., Jones E. G. High-Resolution Light and Electron Microscopic Immunocytochemistry of Colocalized GABA and Calbindin D-28k in Somata and Double Bouquet Cell Axons of Monkey Somatosensory Cortex. Eur J Neurosci. 1992 Oct;4(1):46–60. doi: 10.1111/j.1460-9568.1992.tb00108.x. [DOI] [PubMed] [Google Scholar]
- Deisz R. A., Prince D. A. Frequency-dependent depression of inhibition in guinea-pig neocortex in vitro by GABAB receptor feed-back on GABA release. J Physiol. 1989 May;412:513–541. doi: 10.1113/jphysiol.1989.sp017629. [DOI] [PMC free article] [PubMed] [Google Scholar]
- Deuchars J., Thomson A. M. Single axon fast inhibitory postsynaptic potentials elicited by a sparsely spiny interneuron in rat neocortex. Neuroscience. 1995 Apr;65(4):935–942. doi: 10.1016/0306-4522(95)00020-j. [DOI] [PubMed] [Google Scholar]
- Fariñas I., DeFelipe J. Patterns of synaptic input on corticocortical and corticothalamic cells in the cat visual cortex. I. The cell body. J Comp Neurol. 1991 Feb 1;304(1):53–69. doi: 10.1002/cne.903040105. [DOI] [PubMed] [Google Scholar]
- Freund T. F., Martin K. A., Somogyi P., Whitteridge D. Innervation of cat visual areas 17 and 18 by physiologically identified X- and Y- type thalamic afferents. II. Identification of postsynaptic targets by GABA immunocytochemistry and Golgi impregnation. J Comp Neurol. 1985 Dec 8;242(2):275–291. doi: 10.1002/cne.902420209. [DOI] [PubMed] [Google Scholar]
- Friedlander M. J., Martin K. A. Development of Y-axon innervation of cortical area 18 in the cat. J Physiol. 1989 Sep;416:183–213. doi: 10.1113/jphysiol.1989.sp017756. [DOI] [PMC free article] [PubMed] [Google Scholar]
- Gilbert C. D., Wiesel T. N. Clustered intrinsic connections in cat visual cortex. J Neurosci. 1983 May;3(5):1116–1133. doi: 10.1523/JNEUROSCI.03-05-01116.1983. [DOI] [PMC free article] [PubMed] [Google Scholar]
- Gilbert C. D., Wiesel T. N. Morphology and intracortical projections of functionally characterised neurones in the cat visual cortex. Nature. 1979 Jul 12;280(5718):120–125. doi: 10.1038/280120a0. [DOI] [PubMed] [Google Scholar]
- Gulyás A. I., Miles R., Sík A., Tóth K., Tamamaki N., Freund T. F. Hippocampal pyramidal cells excite inhibitory neurons through a single release site. Nature. 1993 Dec 16;366(6456):683–687. doi: 10.1038/366683a0. [DOI] [PubMed] [Google Scholar]
- Halasy K., Somogyi P. Subdivisions in the multiple GABAergic innervation of granule cells in the dentate gyrus of the rat hippocampus. Eur J Neurosci. 1993 May 1;5(5):411–429. doi: 10.1111/j.1460-9568.1993.tb00508.x. [DOI] [PubMed] [Google Scholar]
- Humphrey A. L., Sur M., Uhlrich D. J., Sherman S. M. Projection patterns of individual X- and Y-cell axons from the lateral geniculate nucleus to cortical area 17 in the cat. J Comp Neurol. 1985 Mar 8;233(2):159–189. doi: 10.1002/cne.902330203. [DOI] [PubMed] [Google Scholar]
- Kang Y., Kaneko T., Ohishi H., Endo K., Araki T. Spatiotemporally differential inhibition of pyramidal cells in the cat motor cortex. J Neurophysiol. 1994 Jan;71(1):280–293. doi: 10.1152/jn.1994.71.1.280. [DOI] [PubMed] [Google Scholar]
- Kawaguchi Y. Physiological subgroups of nonpyramidal cells with specific morphological characteristics in layer II/III of rat frontal cortex. J Neurosci. 1995 Apr;15(4):2638–2655. doi: 10.1523/JNEUROSCI.15-04-02638.1995. [DOI] [PMC free article] [PubMed] [Google Scholar]
- Kisvárday Z. F., Beaulieu C., Eysel U. T. Network of GABAergic large basket cells in cat visual cortex (area 18): implication for lateral disinhibition. J Comp Neurol. 1993 Jan 15;327(3):398–415. doi: 10.1002/cne.903270307. [DOI] [PubMed] [Google Scholar]
- Kisvárday Z. F. GABAergic networks of basket cells in the visual cortex. Prog Brain Res. 1992;90:385–405. doi: 10.1016/s0079-6123(08)63623-7. [DOI] [PubMed] [Google Scholar]
- Kisvárday Z. F., Martin K. A., Freund T. F., Maglóczky Z., Whitteridge D., Somogyi P. Synaptic targets of HRP-filled layer III pyramidal cells in the cat striate cortex. Exp Brain Res. 1986;64(3):541–552. doi: 10.1007/BF00340492. [DOI] [PubMed] [Google Scholar]
- Kisvárday Z. F., Martin K. A., Whitteridge D., Somogyi P. Synaptic connections of intracellularly filled clutch cells: a type of small basket cell in the visual cortex of the cat. J Comp Neurol. 1985 Nov 8;241(2):111–137. doi: 10.1002/cne.902410202. [DOI] [PubMed] [Google Scholar]
- Martin K. A., Whitteridge D. Form, function and intracortical projections of spiny neurones in the striate visual cortex of the cat. J Physiol. 1984 Aug;353:463–504. doi: 10.1113/jphysiol.1984.sp015347. [DOI] [PMC free article] [PubMed] [Google Scholar]
- Miles R., Tóth K., Gulyás A. I., Hájos N., Freund T. F. Differences between somatic and dendritic inhibition in the hippocampus. Neuron. 1996 Apr;16(4):815–823. doi: 10.1016/s0896-6273(00)80101-4. [DOI] [PubMed] [Google Scholar]
- Newberry N. R., Nicoll R. A. Comparison of the action of baclofen with gamma-aminobutyric acid on rat hippocampal pyramidal cells in vitro. J Physiol. 1985 Mar;360:161–185. doi: 10.1113/jphysiol.1985.sp015610. [DOI] [PMC free article] [PubMed] [Google Scholar]
- Qian N., Sejnowski T. J. When is an inhibitory synapse effective? Proc Natl Acad Sci U S A. 1990 Oct;87(20):8145–8149. doi: 10.1073/pnas.87.20.8145. [DOI] [PMC free article] [PubMed] [Google Scholar]
- Sillito A. M. GABA mediated inhibitory processes in the function of the geniculo-striate system. Prog Brain Res. 1992;90:349–384. doi: 10.1016/s0079-6123(08)63622-5. [DOI] [PubMed] [Google Scholar]
- Singer W., Gray C. M. Visual feature integration and the temporal correlation hypothesis. Annu Rev Neurosci. 1995;18:555–586. doi: 10.1146/annurev.ne.18.030195.003011. [DOI] [PubMed] [Google Scholar]
- Somogyi P., Cowey A. Combined Golgi and electron microscopic study on the synapses formed by double bouquet cells in the visual cortex of the cat and monkey. J Comp Neurol. 1981 Feb 1;195(4):547–566. doi: 10.1002/cne.901950402. [DOI] [PubMed] [Google Scholar]
- Somogyi P., Kisvárday Z. F., Martin K. A., Whitteridge D. Synaptic connections of morphologically identified and physiologically characterized large basket cells in the striate cortex of cat. Neuroscience. 1983 Oct;10(2):261–294. doi: 10.1016/0306-4522(83)90133-1. [DOI] [PubMed] [Google Scholar]
- Spruston N., Schiller Y., Stuart G., Sakmann B. Activity-dependent action potential invasion and calcium influx into hippocampal CA1 dendrites. Science. 1995 Apr 14;268(5208):297–300. doi: 10.1126/science.7716524. [DOI] [PubMed] [Google Scholar]
- Thomson A. M., West D. C., Hahn J., Deuchars J. Single axon IPSPs elicited in pyramidal cells by three classes of interneurones in slices of rat neocortex. J Physiol. 1996 Oct 1;496(Pt 1):81–102. doi: 10.1113/jphysiol.1996.sp021667. [DOI] [PMC free article] [PubMed] [Google Scholar]