Abstract
1. We examined the hypothesis that in skeletal muscle the steep relationship between twitch tension and sarcomere length (SL) within the range 2.30 to 1.85 microns involves SL-dependent alterations in the rate of tension development. 2. In skinned preparations of both rat slow-twitch and rabbit fast-twitch skeletal muscle fibres the rate of tension redevelopment (ktr) at 15 degrees C was reduced at short SL (approximately 2.00 microns) compared with a longer SL (approximately 2.30 microns). In submaximally activated fibres, the decrease in ktr over this range of lengths was greater in fast-twitch fibres (38% reduction) than in slow-twitch fibres (14% reduction). 3. Ca2+ sensitivity of tension, as assessed as the pCa (-log[Ca2+]) for half-maximal activation, or pCa50, decreased to a greater extent in rabbit fast-twitch skeletal muscle fibres than in slow-twitch fibres from both rabbit and rat when SL was reduced from approximately 2.30 to approximately 1.85 microns. The delta pCa50 over this SL range was 0.24 +/- 0.07 pCa units in fast-twitch fibres from rabbit psoas muscle. The delta pCa50 for slow-twitch fibres from rabbit and rat soleus muscle was 0.08 +/- 0.02 and 0.10 +/- 0.04 pCa units, respectively. 4. Osmotic compression of both slow-twitch and fast-twitch fibres at a SL of 2.00 microns increased ktr to values similar to those obtained at a SL of 2.30 microns in the absence of dextran. This result indicates that the slower rate of tension redevelopment at short SL is due in large part to the increase in interfilament lattice spacing associated with shorter SL. 5. Taken together, these results suggest that length dependence of twitch tension is, in part, due to length dependence of isometric cross-bridge interaction kinetics, an effect that is mediated by length-dependent changes in interfilament lattice spacing.
Full text
PDF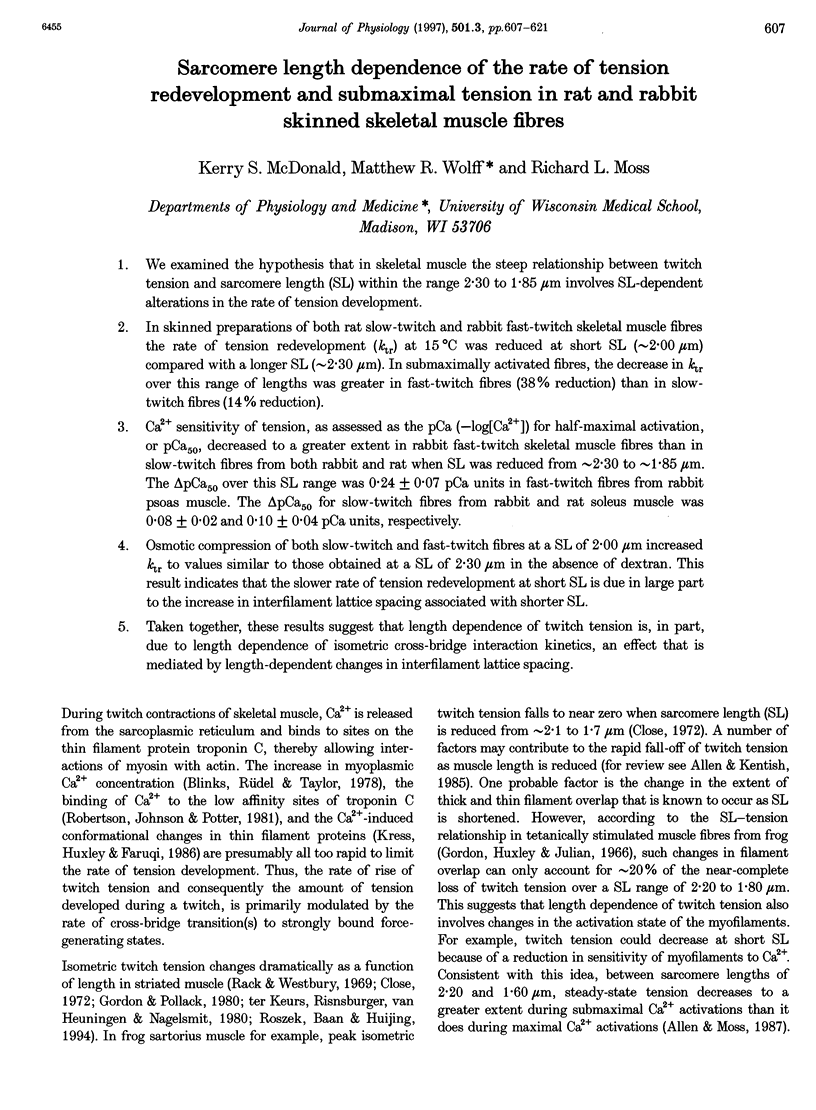
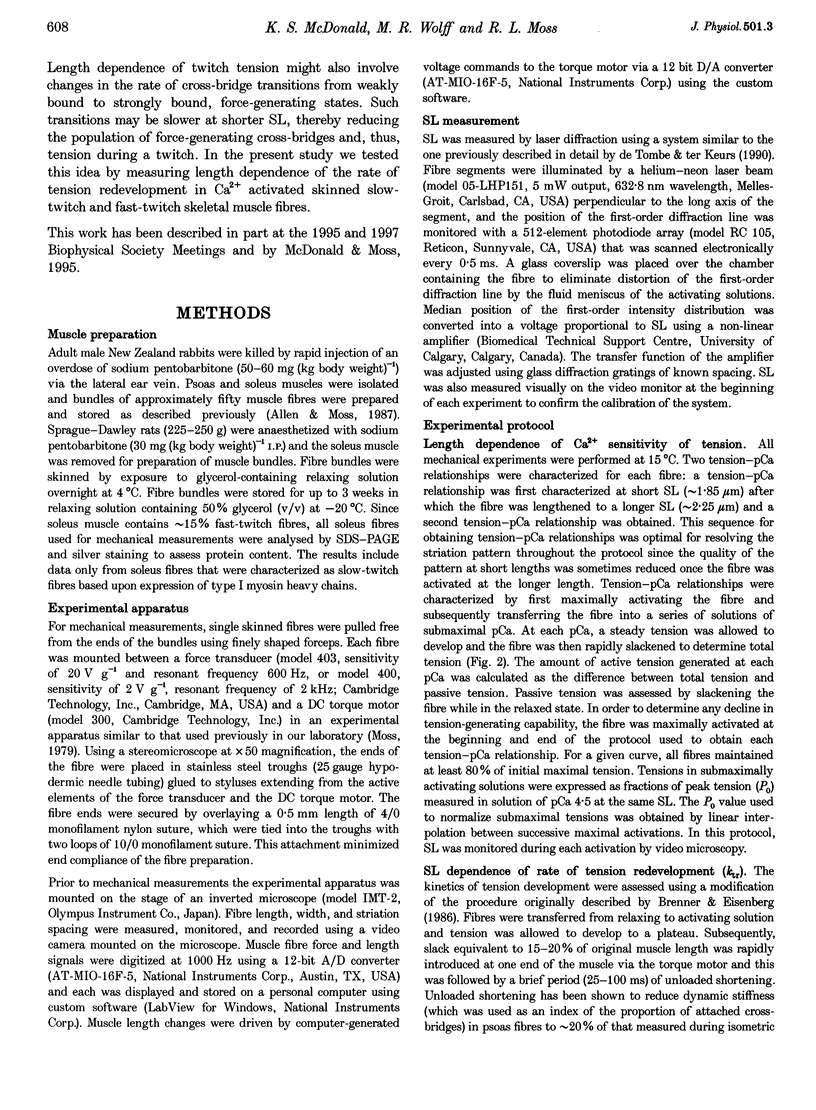
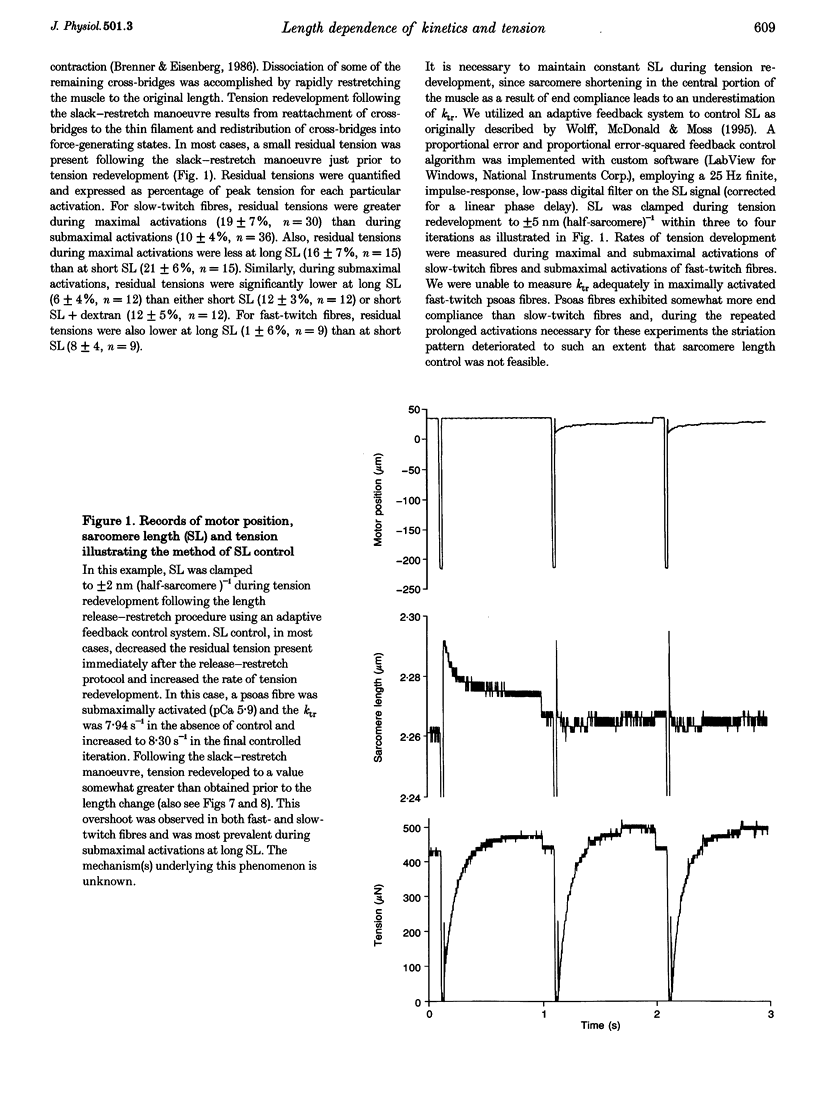
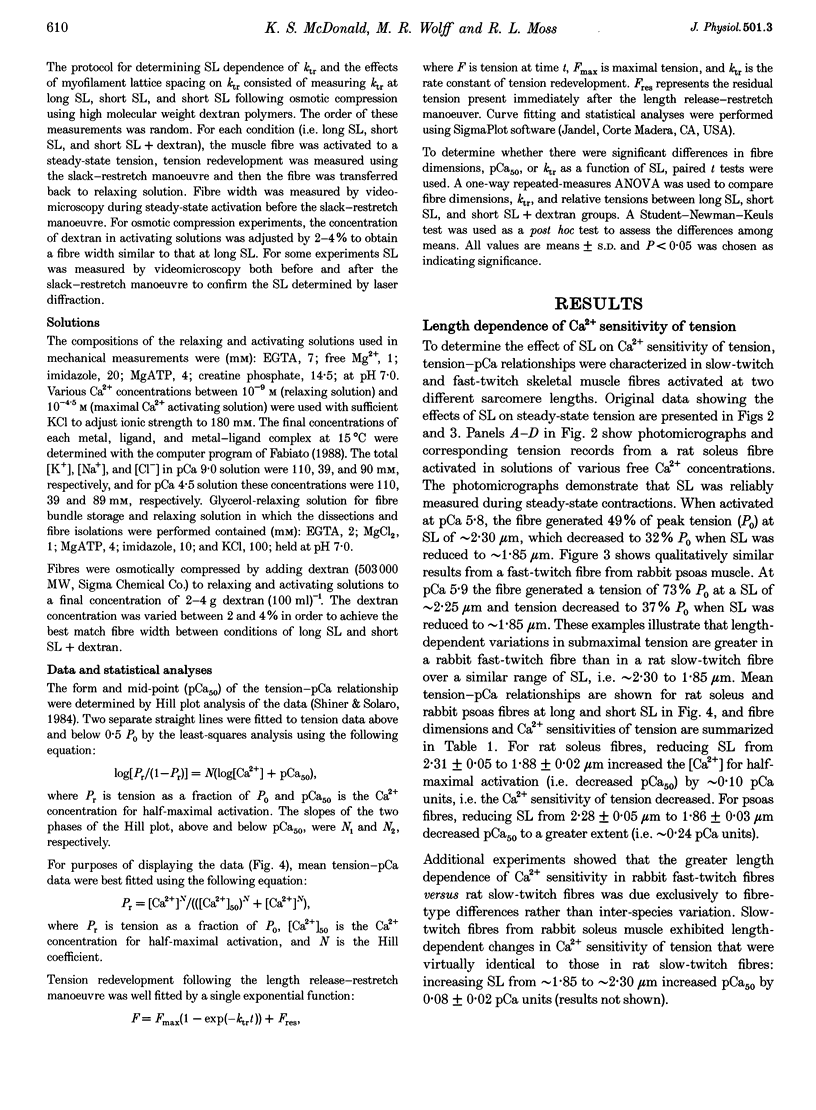
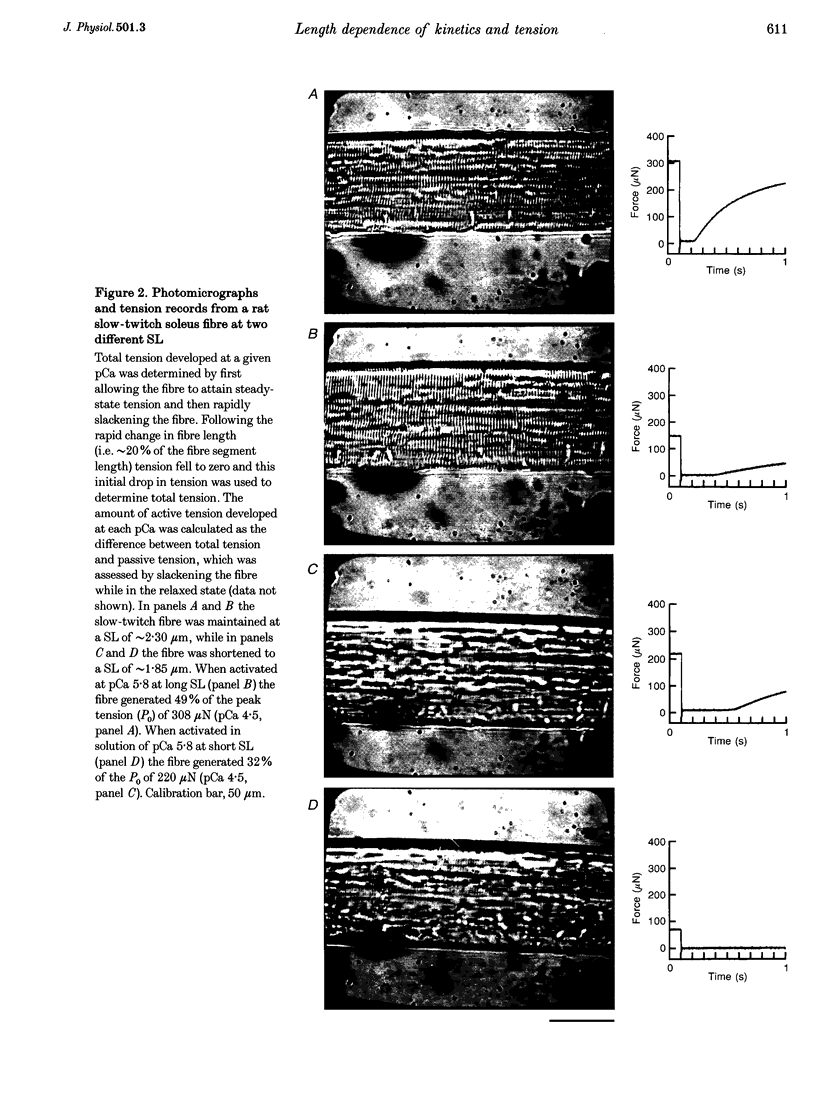
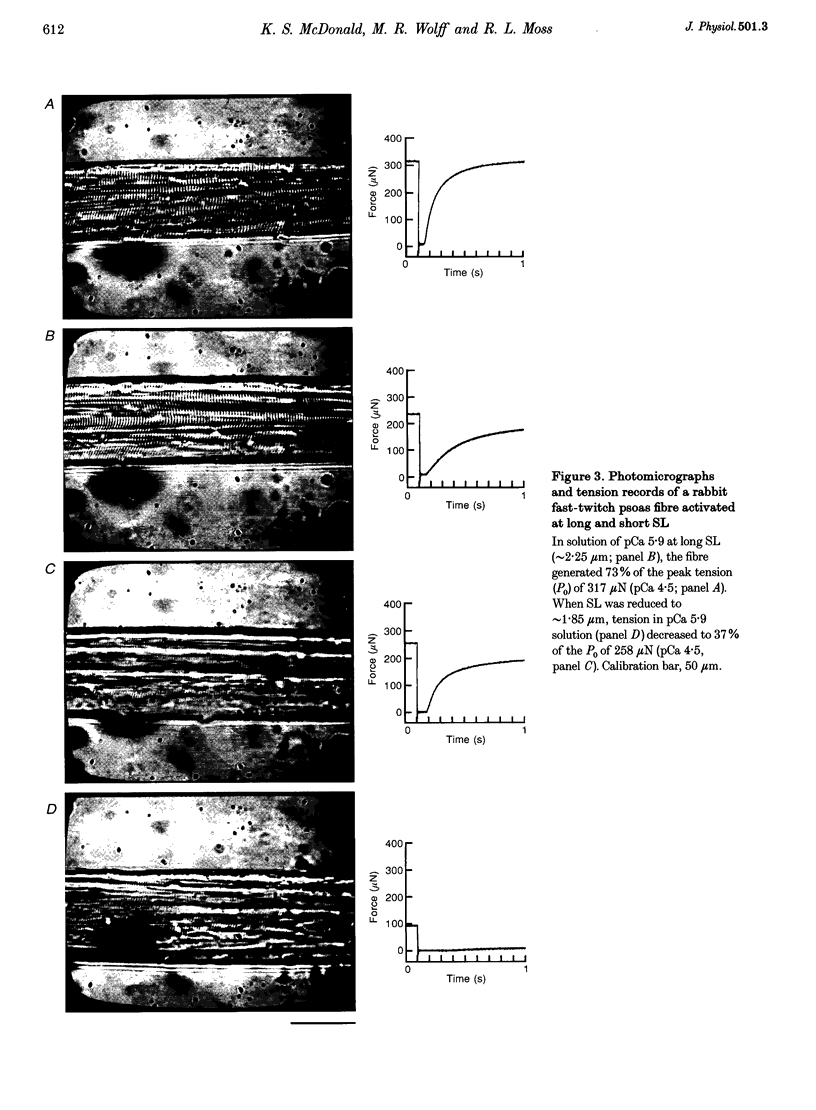
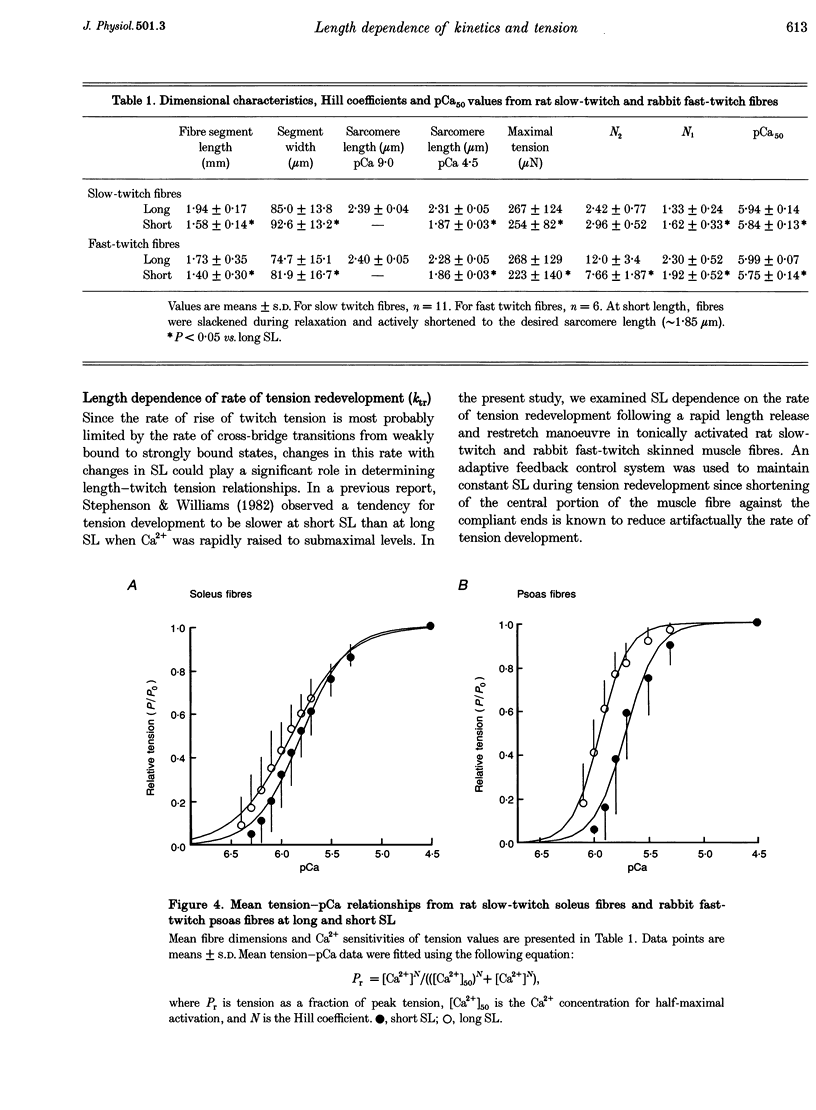
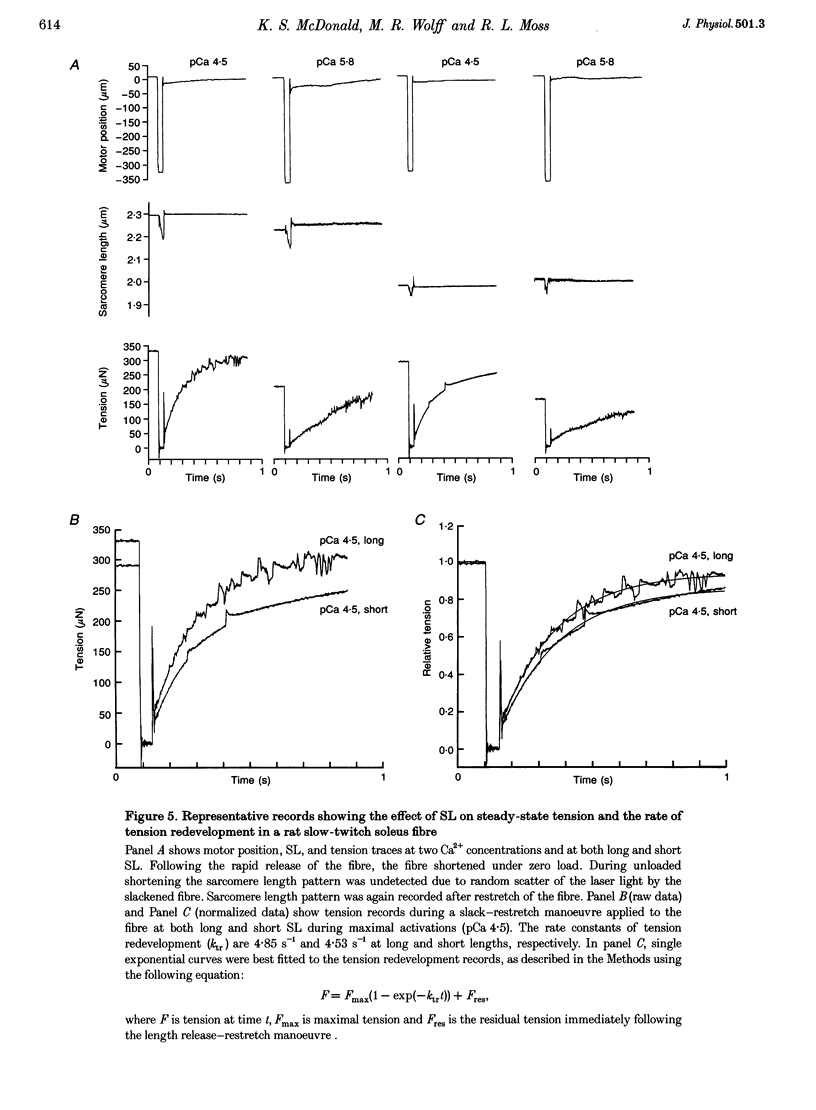
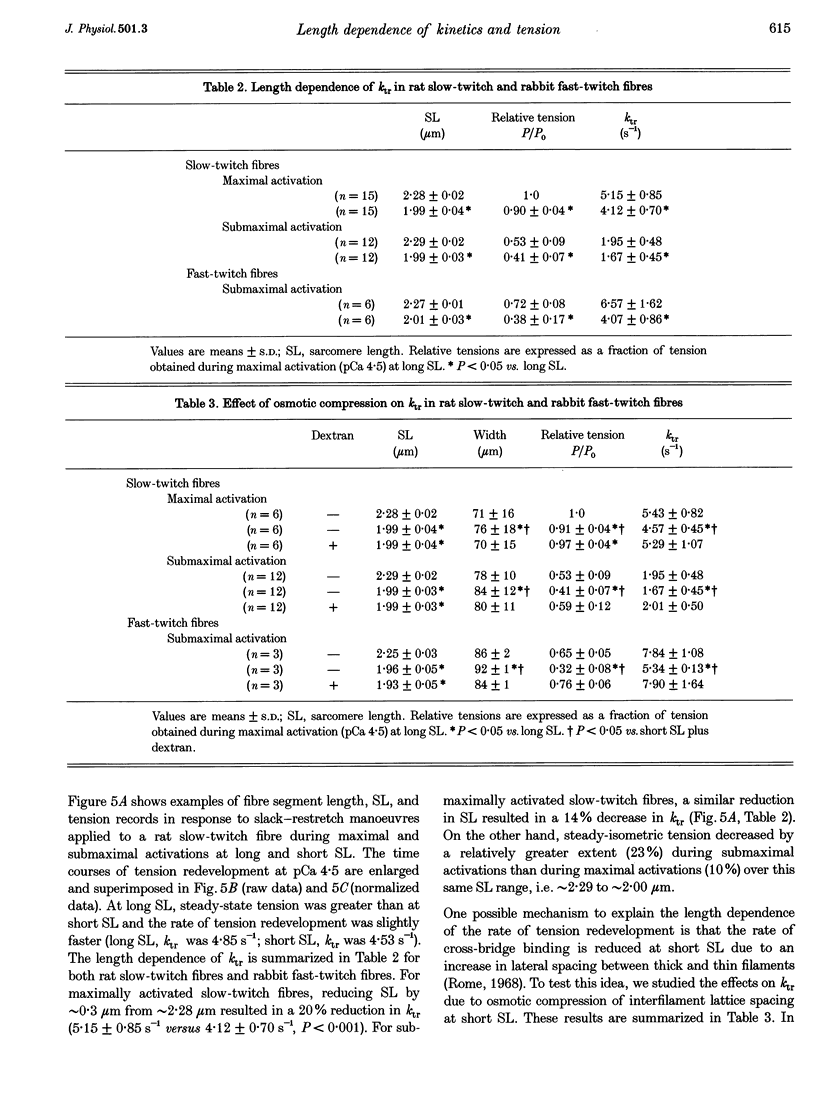
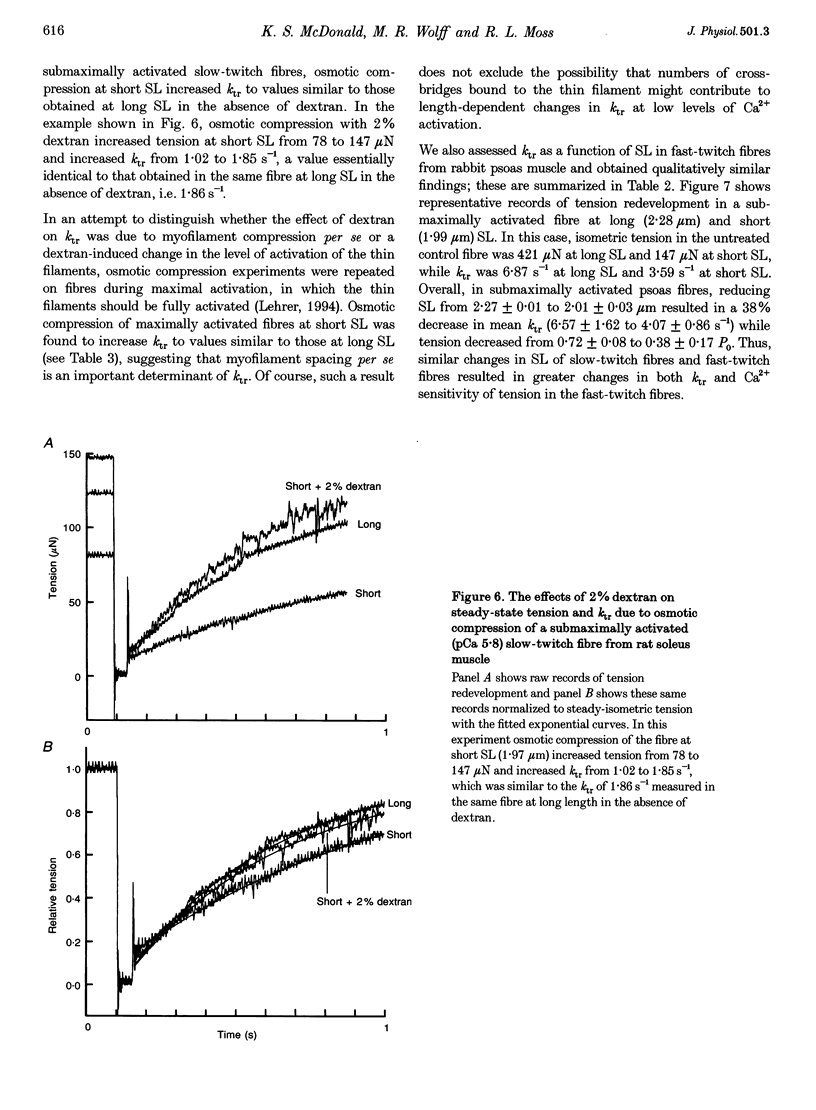
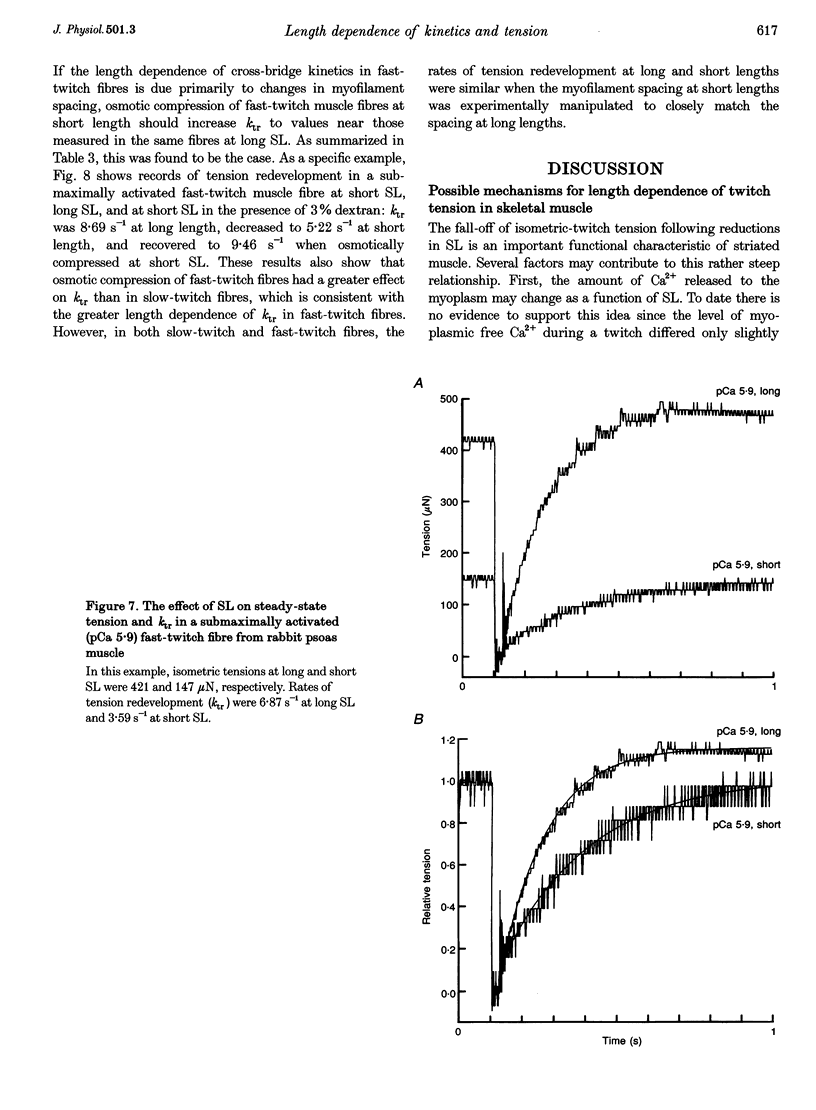
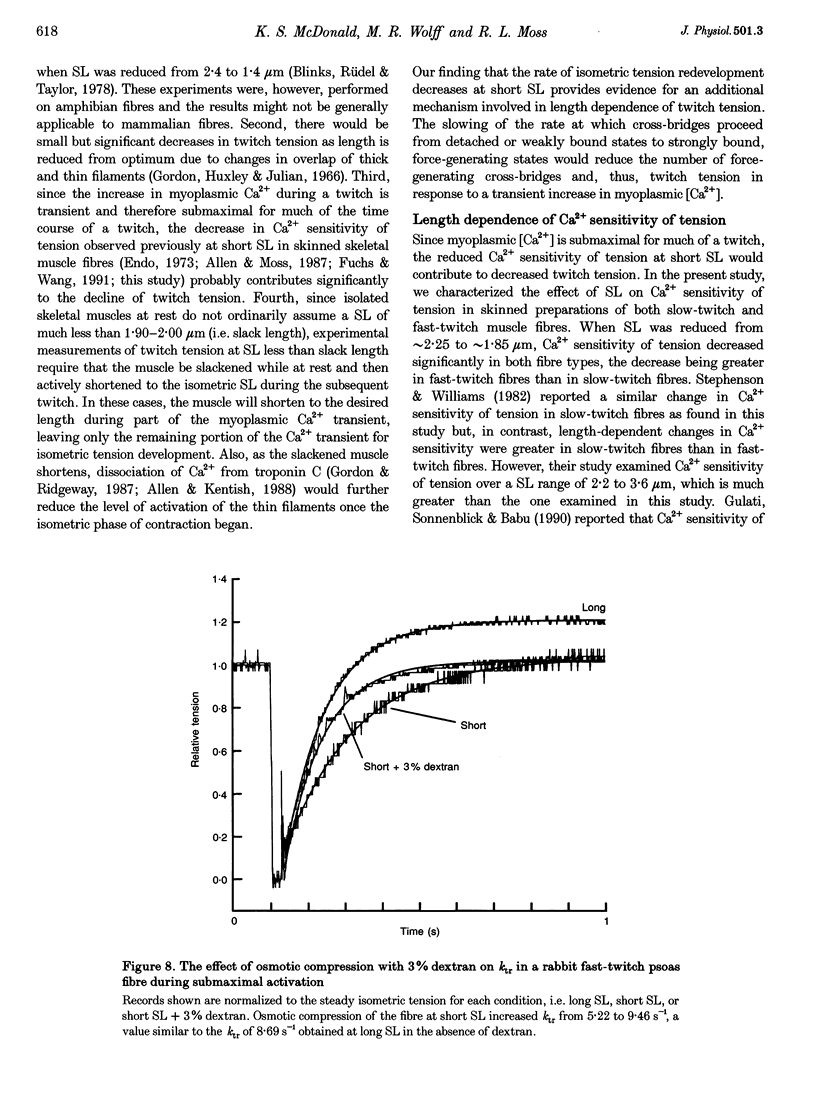
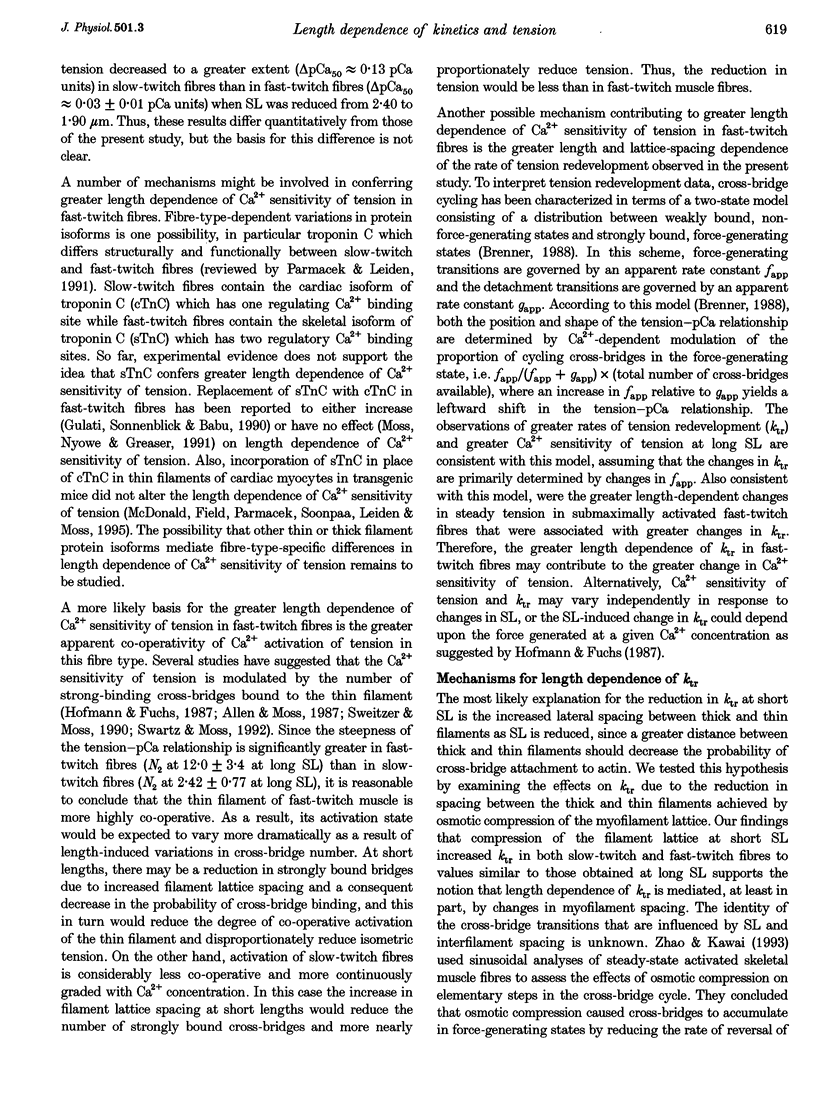
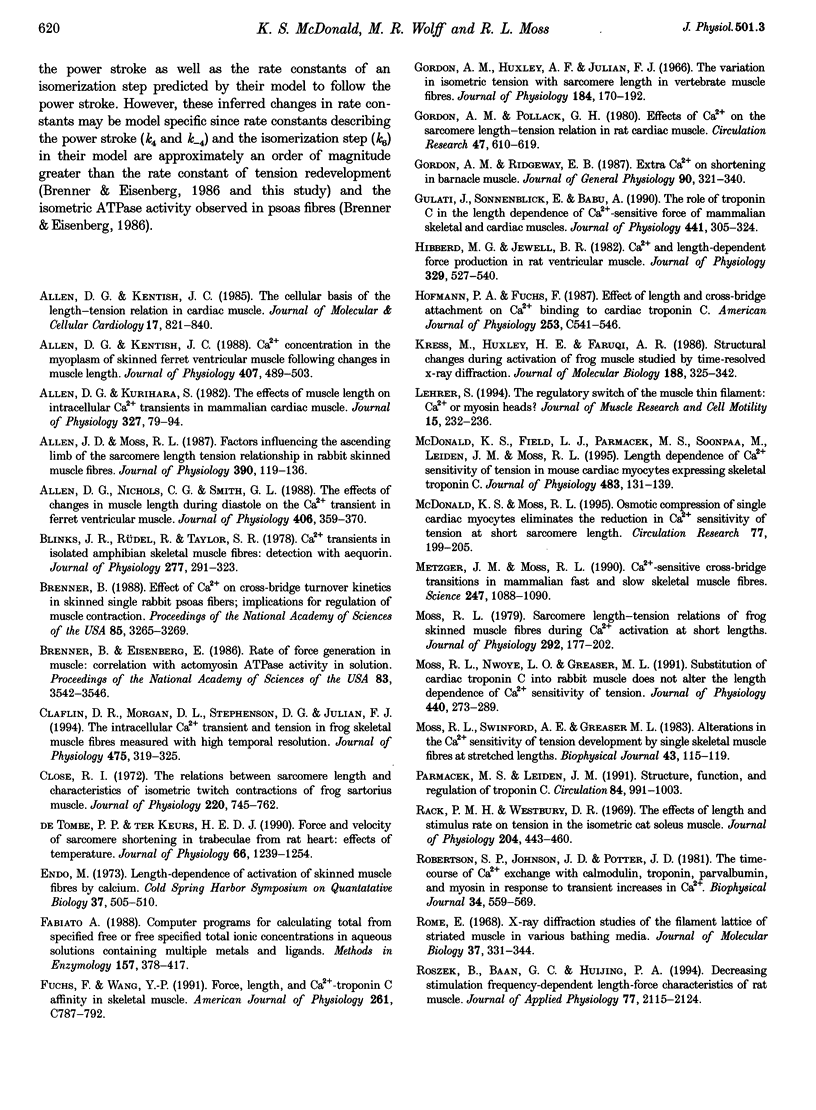
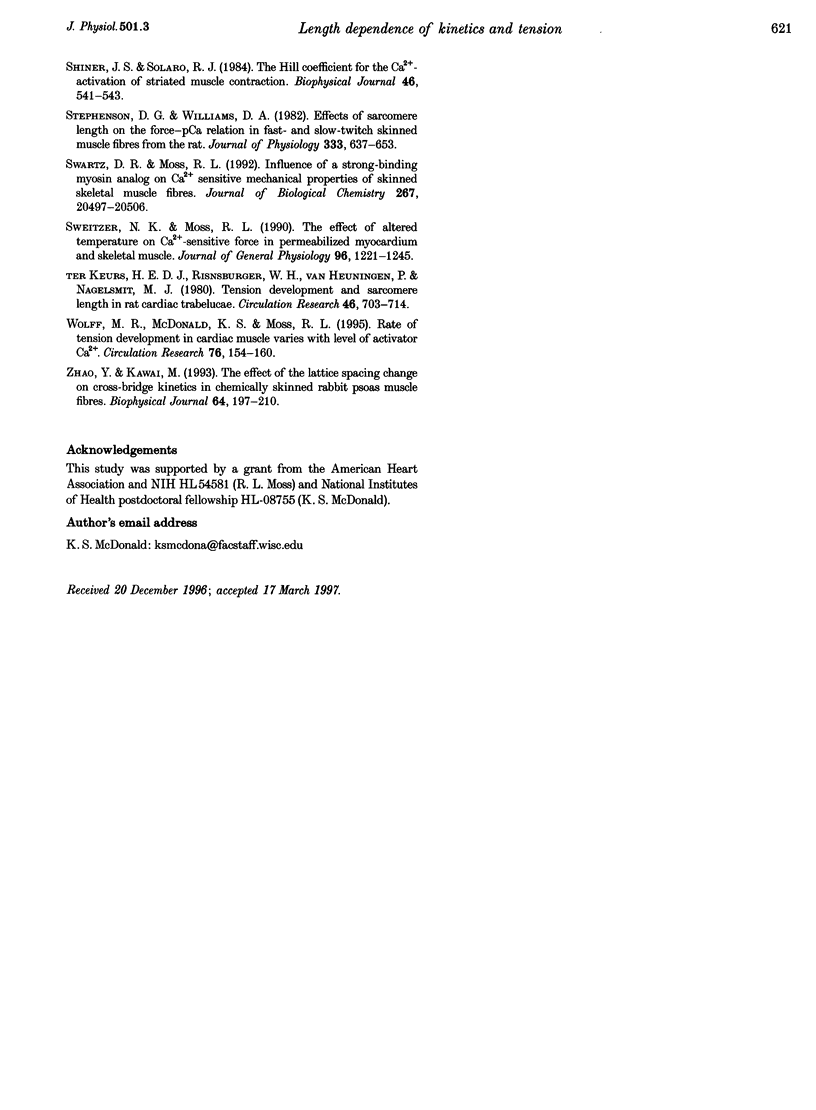
Images in this article
Selected References
These references are in PubMed. This may not be the complete list of references from this article.
- Allen D. G., Kentish J. C. Calcium concentration in the myoplasm of skinned ferret ventricular muscle following changes in muscle length. J Physiol. 1988 Dec;407:489–503. doi: 10.1113/jphysiol.1988.sp017427. [DOI] [PMC free article] [PubMed] [Google Scholar]
- Allen D. G., Kentish J. C. The cellular basis of the length-tension relation in cardiac muscle. J Mol Cell Cardiol. 1985 Sep;17(9):821–840. doi: 10.1016/s0022-2828(85)80097-3. [DOI] [PubMed] [Google Scholar]
- Allen D. G., Kurihara S. The effects of muscle length on intracellular calcium transients in mammalian cardiac muscle. J Physiol. 1982 Jun;327:79–94. doi: 10.1113/jphysiol.1982.sp014221. [DOI] [PMC free article] [PubMed] [Google Scholar]
- Allen D. G., Nichols C. G., Smith G. L. The effects of changes in muscle length during diastole on the calcium transient in ferret ventricular muscle. J Physiol. 1988 Dec;406:359–370. doi: 10.1113/jphysiol.1988.sp017385. [DOI] [PMC free article] [PubMed] [Google Scholar]
- Allen J. D., Moss R. L. Factors influencing the ascending limb of the sarcomere length-tension relationship in rabbit skinned muscle fibres. J Physiol. 1987 Sep;390:119–136. doi: 10.1113/jphysiol.1987.sp016689. [DOI] [PMC free article] [PubMed] [Google Scholar]
- Blinks J. R., Rüdel R., Taylor S. R. Calcium transients in isolated amphibian skeletal muscle fibres: detection with aequorin. J Physiol. 1978 Apr;277:291–323. doi: 10.1113/jphysiol.1978.sp012273. [DOI] [PMC free article] [PubMed] [Google Scholar]
- Brenner B. Effect of Ca2+ on cross-bridge turnover kinetics in skinned single rabbit psoas fibers: implications for regulation of muscle contraction. Proc Natl Acad Sci U S A. 1988 May;85(9):3265–3269. doi: 10.1073/pnas.85.9.3265. [DOI] [PMC free article] [PubMed] [Google Scholar]
- Brenner B., Eisenberg E. Rate of force generation in muscle: correlation with actomyosin ATPase activity in solution. Proc Natl Acad Sci U S A. 1986 May;83(10):3542–3546. doi: 10.1073/pnas.83.10.3542. [DOI] [PMC free article] [PubMed] [Google Scholar]
- Claflin D. R., Morgan D. L., Stephenson D. G., Julian F. J. The intracellular Ca2+ transient and tension in frog skeletal muscle fibres measured with high temporal resolution. J Physiol. 1994 Mar 1;475(2):319–325. doi: 10.1113/jphysiol.1994.sp020072. [DOI] [PMC free article] [PubMed] [Google Scholar]
- Close R. I. The relations between sarcomere length and characteristics of isometric twitch contractions of frog sartorius muscle. J Physiol. 1972 Feb;220(3):745–762. doi: 10.1113/jphysiol.1972.sp009733. [DOI] [PMC free article] [PubMed] [Google Scholar]
- Fabiato A. Computer programs for calculating total from specified free or free from specified total ionic concentrations in aqueous solutions containing multiple metals and ligands. Methods Enzymol. 1988;157:378–417. doi: 10.1016/0076-6879(88)57093-3. [DOI] [PubMed] [Google Scholar]
- Fuchs F., Wang Y. P. Force, length, and Ca(2+)-troponin C affinity in skeletal muscle. Am J Physiol. 1991 Nov;261(5 Pt 1):C787–C792. doi: 10.1152/ajpcell.1991.261.5.C787. [DOI] [PubMed] [Google Scholar]
- Gordon A. M., Huxley A. F., Julian F. J. The variation in isometric tension with sarcomere length in vertebrate muscle fibres. J Physiol. 1966 May;184(1):170–192. doi: 10.1113/jphysiol.1966.sp007909. [DOI] [PMC free article] [PubMed] [Google Scholar]
- Gordon A. M., Pollack G. H. Effects of calcium on the sarcomere length-tension relation in rat cardiac muscle. Implications for the Frank-Starling mechanism. Circ Res. 1980 Oct;47(4):610–619. doi: 10.1161/01.res.47.4.610. [DOI] [PubMed] [Google Scholar]
- Gordon A. M., Ridgway E. B. Extra calcium on shortening in barnacle muscle. Is the decrease in calcium binding related to decreased cross-bridge attachment, force, or length? J Gen Physiol. 1987 Sep;90(3):321–340. doi: 10.1085/jgp.90.3.321. [DOI] [PMC free article] [PubMed] [Google Scholar]
- Gulati J., Sonnenblick E., Babu A. The role of troponin C in the length dependence of Ca(2+)-sensitive force of mammalian skeletal and cardiac muscles. J Physiol. 1991 Sep;441:305–324. doi: 10.1113/jphysiol.1991.sp018753. [DOI] [PMC free article] [PubMed] [Google Scholar]
- Hibberd M. G., Jewell B. R. Calcium- and length-dependent force production in rat ventricular muscle. J Physiol. 1982 Aug;329:527–540. doi: 10.1113/jphysiol.1982.sp014317. [DOI] [PMC free article] [PubMed] [Google Scholar]
- Kress M., Huxley H. E., Faruqi A. R., Hendrix J. Structural changes during activation of frog muscle studied by time-resolved X-ray diffraction. J Mol Biol. 1986 Apr 5;188(3):325–342. doi: 10.1016/0022-2836(86)90158-0. [DOI] [PubMed] [Google Scholar]
- Lehrer S. S. The regulatory switch of the muscle thin filament: Ca2+ or myosin heads? J Muscle Res Cell Motil. 1994 Jun;15(3):232–236. doi: 10.1007/BF00123476. [DOI] [PubMed] [Google Scholar]
- McDonald K. S., Field L. J., Parmacek M. S., Soonpaa M., Leiden J. M., Moss R. L. Length dependence of Ca2+ sensitivity of tension in mouse cardiac myocytes expressing skeletal troponin C. J Physiol. 1995 Feb 15;483(Pt 1):131–139. doi: 10.1113/jphysiol.1995.sp020573. [DOI] [PMC free article] [PubMed] [Google Scholar]
- McDonald K. S., Moss R. L. Osmotic compression of single cardiac myocytes eliminates the reduction in Ca2+ sensitivity of tension at short sarcomere length. Circ Res. 1995 Jul;77(1):199–205. doi: 10.1161/01.res.77.1.199. [DOI] [PubMed] [Google Scholar]
- Metzger J. M., Moss R. L. Calcium-sensitive cross-bridge transitions in mammalian fast and slow skeletal muscle fibers. Science. 1990 Mar 2;247(4946):1088–1090. doi: 10.1126/science.2309121. [DOI] [PubMed] [Google Scholar]
- Moss R. L., Nwoye L. O., Greaser M. L. Substitution of cardiac troponin C into rabbit muscle does not alter the length dependence of Ca2+ sensitivity of tension. J Physiol. 1991;440:273–289. doi: 10.1113/jphysiol.1991.sp018708. [DOI] [PMC free article] [PubMed] [Google Scholar]
- Moss R. L. Sarcomere length-tension relations of frog skinned muscle fibres during calcium activation at short lengths. J Physiol. 1979 Jul;292:177–192. doi: 10.1113/jphysiol.1979.sp012845. [DOI] [PMC free article] [PubMed] [Google Scholar]
- Moss R. L., Swinford A. E., Greaser M. L. Alterations in the Ca2+ sensitivity of tension development by single skeletal muscle fibers at stretched lengths. Biophys J. 1983 Jul;43(1):115–119. doi: 10.1016/S0006-3495(83)84329-X. [DOI] [PMC free article] [PubMed] [Google Scholar]
- Parmacek M. S., Leiden J. M. Structure, function, and regulation of troponin C. Circulation. 1991 Sep;84(3):991–1003. doi: 10.1161/01.cir.84.3.991. [DOI] [PubMed] [Google Scholar]
- Rack P. M., Westbury D. R. The effects of length and stimulus rate on tension in the isometric cat soleus muscle. J Physiol. 1969 Oct;204(2):443–460. doi: 10.1113/jphysiol.1969.sp008923. [DOI] [PMC free article] [PubMed] [Google Scholar]
- Robertson S. P., Johnson J. D., Potter J. D. The time-course of Ca2+ exchange with calmodulin, troponin, parvalbumin, and myosin in response to transient increases in Ca2+. Biophys J. 1981 Jun;34(3):559–569. doi: 10.1016/S0006-3495(81)84868-0. [DOI] [PMC free article] [PubMed] [Google Scholar]
- Rome E. X-ray diffraction studies of the filament lattice of striated muscle in various bathing media. J Mol Biol. 1968 Oct 28;37(2):331–344. doi: 10.1016/0022-2836(68)90272-6. [DOI] [PubMed] [Google Scholar]
- Roszek B., Baan G. C., Huijing P. A. Decreasing stimulation frequency-dependent length-force characteristics of rat muscle. J Appl Physiol (1985) 1994 Nov;77(5):2115–2124. doi: 10.1152/jappl.1994.77.5.2115. [DOI] [PubMed] [Google Scholar]
- Shiner J. S., Solaro R. J. The hill coefficient for the Ca2+-activation of striated muscle contraction. Biophys J. 1984 Oct;46(4):541–543. doi: 10.1016/S0006-3495(84)84051-5. [DOI] [PMC free article] [PubMed] [Google Scholar]
- Stephenson D. G., Williams D. A. Effects of sarcomere length on the force-pCa relation in fast- and slow-twitch skinned muscle fibres from the rat. J Physiol. 1982 Dec;333:637–653. doi: 10.1113/jphysiol.1982.sp014473. [DOI] [PMC free article] [PubMed] [Google Scholar]
- Swartz D. R., Moss R. L. Influence of a strong-binding myosin analogue on calcium-sensitive mechanical properties of skinned skeletal muscle fibers. J Biol Chem. 1992 Oct 5;267(28):20497–20506. [PubMed] [Google Scholar]
- Sweitzer N. K., Moss R. L. The effect of altered temperature on Ca2(+)-sensitive force in permeabilized myocardium and skeletal muscle. Evidence for force dependence of thin filament activation. J Gen Physiol. 1990 Dec;96(6):1221–1245. doi: 10.1085/jgp.96.6.1221. [DOI] [PMC free article] [PubMed] [Google Scholar]
- Wolff M. R., McDonald K. S., Moss R. L. Rate of tension development in cardiac muscle varies with level of activator calcium. Circ Res. 1995 Jan;76(1):154–160. doi: 10.1161/01.res.76.1.154. [DOI] [PubMed] [Google Scholar]
- Zhao Y., Kawai M. The effect of the lattice spacing change on cross-bridge kinetics in chemically skinned rabbit psoas muscle fibers. II. Elementary steps affected by the spacing change. Biophys J. 1993 Jan;64(1):197–210. doi: 10.1016/S0006-3495(93)81357-2. [DOI] [PMC free article] [PubMed] [Google Scholar]
- de Tombe P. P., ter Keurs H. E. Force and velocity of sarcomere shortening in trabeculae from rat heart. Effects of temperature. Circ Res. 1990 May;66(5):1239–1254. doi: 10.1161/01.res.66.5.1239. [DOI] [PubMed] [Google Scholar]
- ter Keurs H. E., Rijnsburger W. H., van Heuningen R., Nagelsmit M. J. Tension development and sarcomere length in rat cardiac trabeculae. Evidence of length-dependent activation. Circ Res. 1980 May;46(5):703–714. doi: 10.1161/01.res.46.5.703. [DOI] [PubMed] [Google Scholar]