Abstract
1. This study investigated the effects of 7 weeks of sprint training on gas exchange across the lungs and active skeletal muscle during and following maximal cycling exercise in eight healthy males. 2. Pulmonary oxygen uptake (VO2) and carbon dioxide output (VCO2) were measured before and after training during incremental exercise (n = 8) and during and in recovery from a maximal 30 s sprint exercise bout by breath-by-breath analysis (n = 6). To determine gas exchange by the exercising leg muscles, brachial arterial and femoral venous blood O2 and CO2 contents and lactate concentration were measured at rest, during the final 10 s of exercise and during 10 min of recovery. 3. Training increased (P < 0.05) the maximal incremental exercise values of ventilation (VE, by 15.7 +/- 7.1%), VCO2 (by 9.3 +/- 2.1%) and VO2 (by 15.0 +/- 4.2%). Sprint exercise peak power (3.9 +/- 1.0% increase) and cumulative 30 s work (11.7 +/- 2.8% increase) were increased and fatigue index was reduced (by -9.2 +/- 1.5%) after training (P < 0.05). The highest VE, VCO2 and VO2 values attained during sprint exercise were not significantly changed after training, but a significant (P < 0.05) training effect indicated increased VE (by 19.2 +/- 7.9%), VCO2 (by 9.3 +/- 2.1%) and VO2 (by 12.7 +/- 6.5%), primarily reflecting elevated post-exercise values after training. 4. Arterial O2 and CO2 contents were lower after training, by respective mean differences of 3.4 and 21.9 ml l-1 (P < 0.05), whereas the arteriovenous O2 and CO2 content differences and the respiratory exchange ratio across the leg were unchanged by training. 5. Arterial whole blood lactate concentration and the net lactate release by exercising muscle were unchanged by training. 6. The greater peak pulmonary VO2 and VCO2 with sprint exercise, the increased maximal incremental values, unchanged arterial blood lactate concentration and greater sprint performance all point strongly towards enhanced gas exchange across the lungs and in active muscles after sprint training. Enhanced aerobic metabolism after sprint training may contribute to reduced fatigability during maximal exercise, whilst greater pulmonary CO2 output may improve acid-base control after training.
Full text
PDF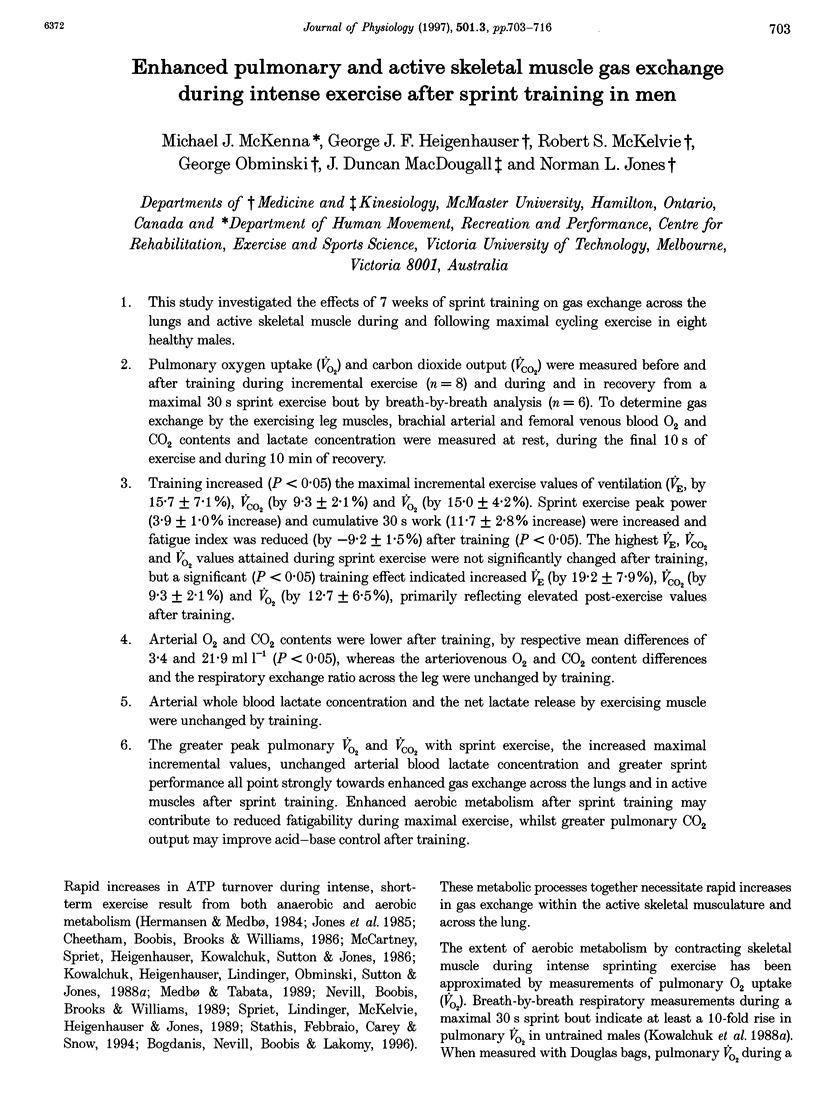
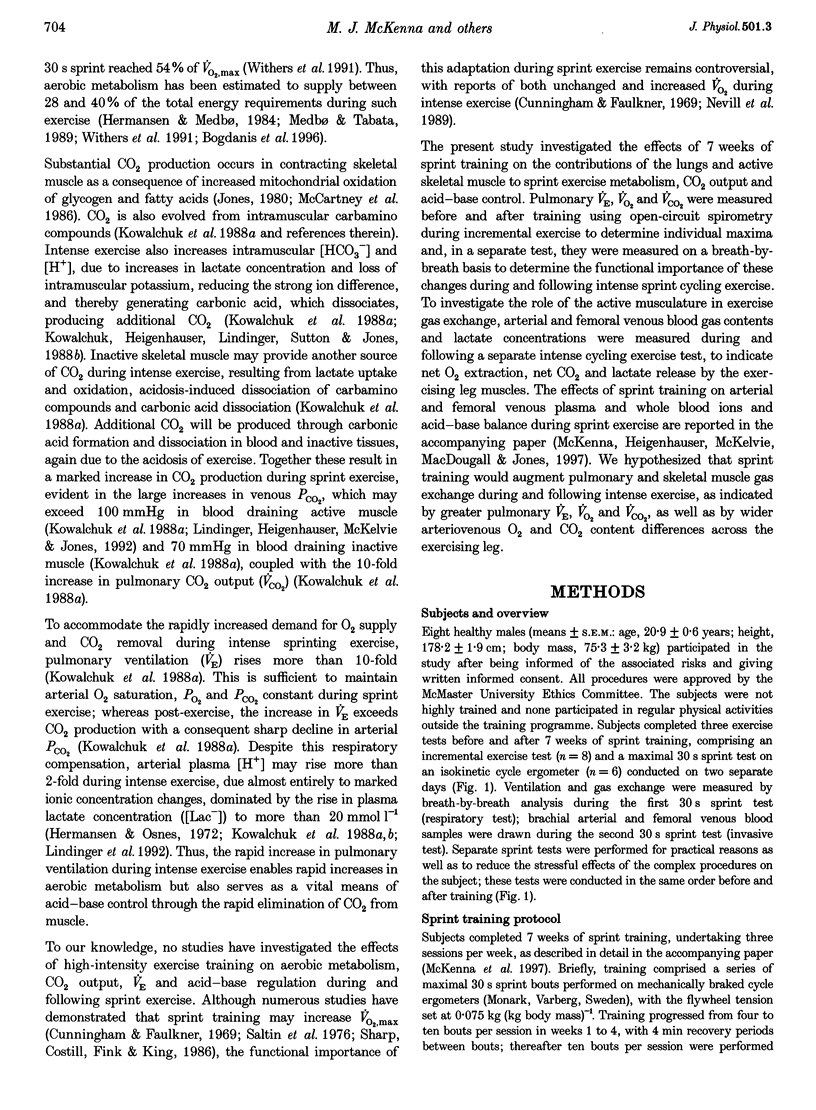
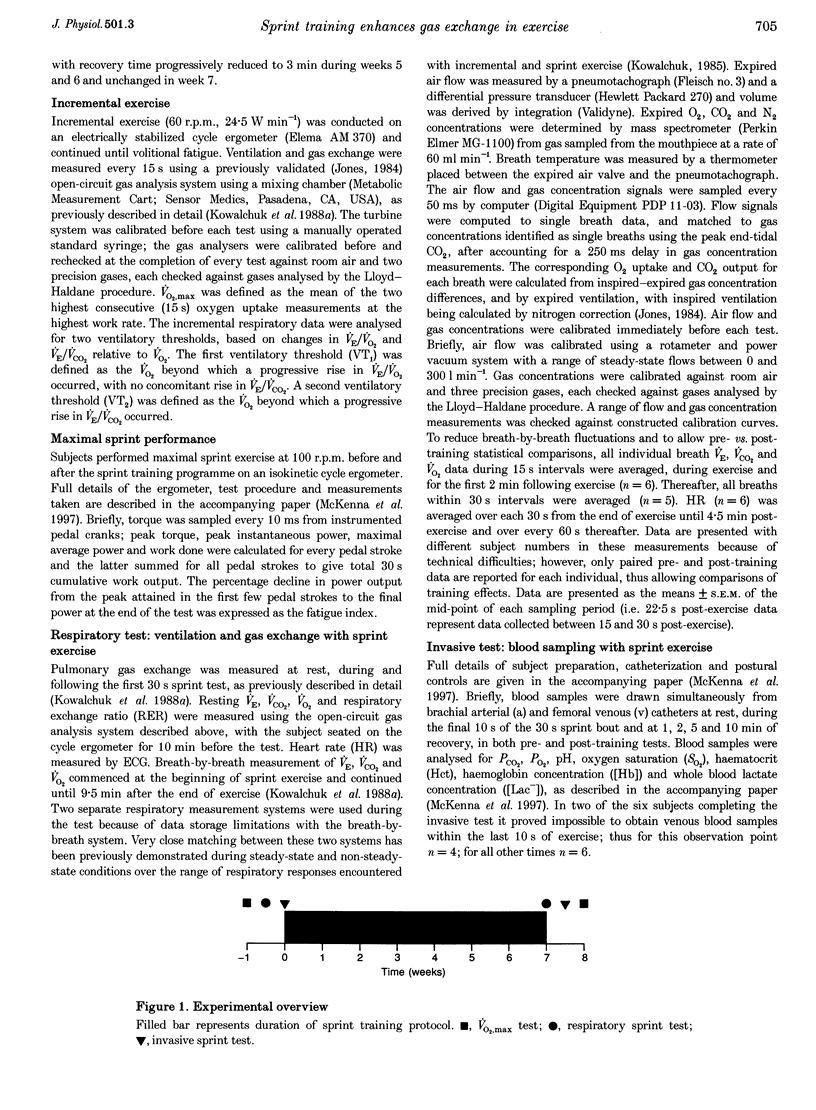
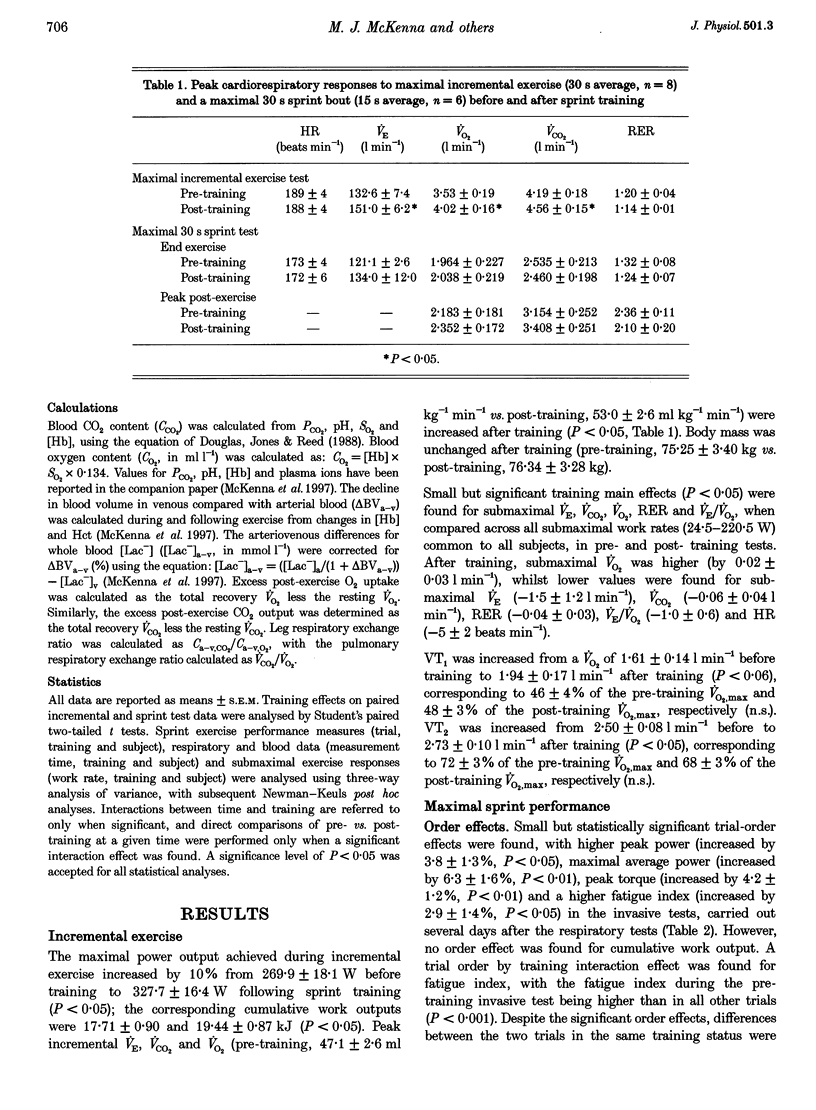
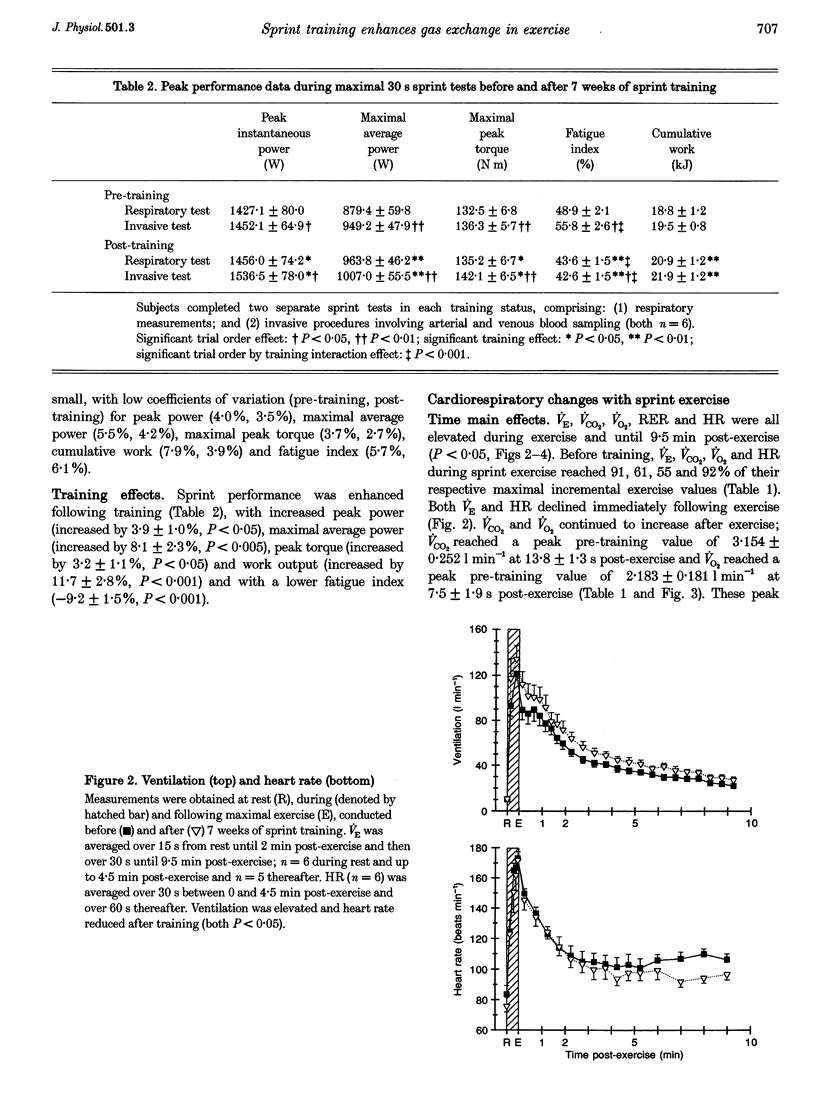
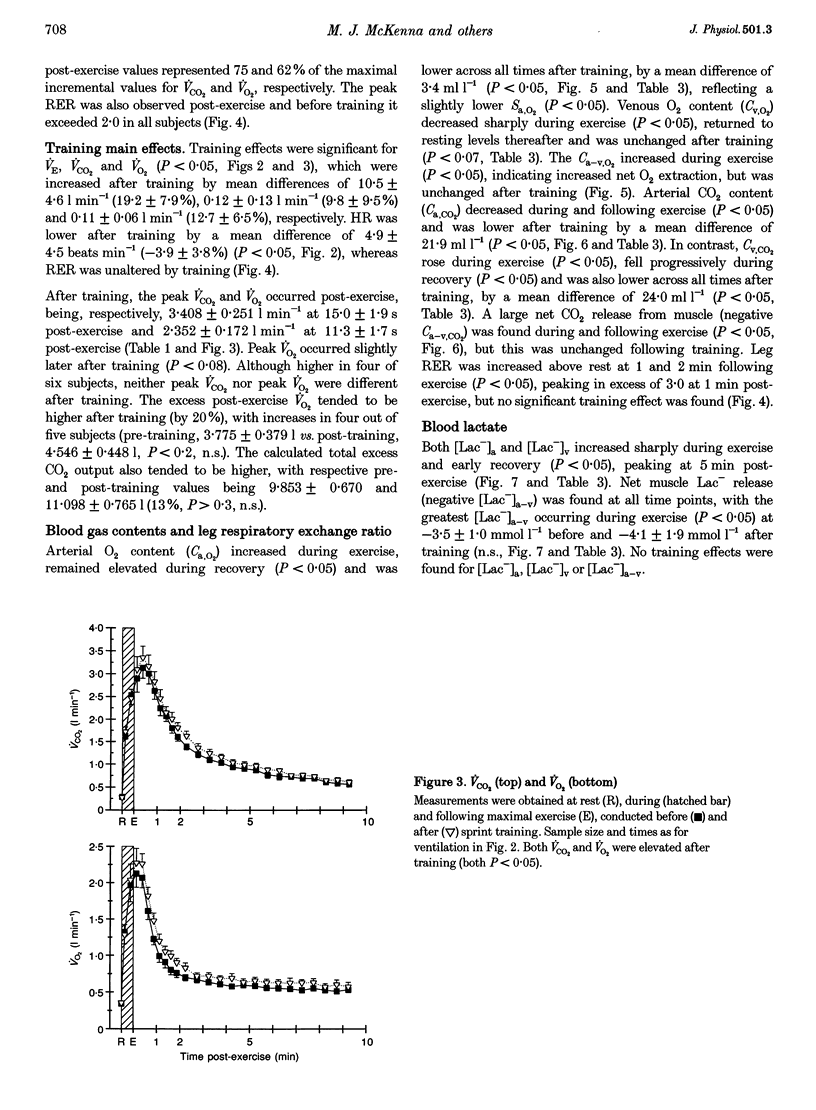
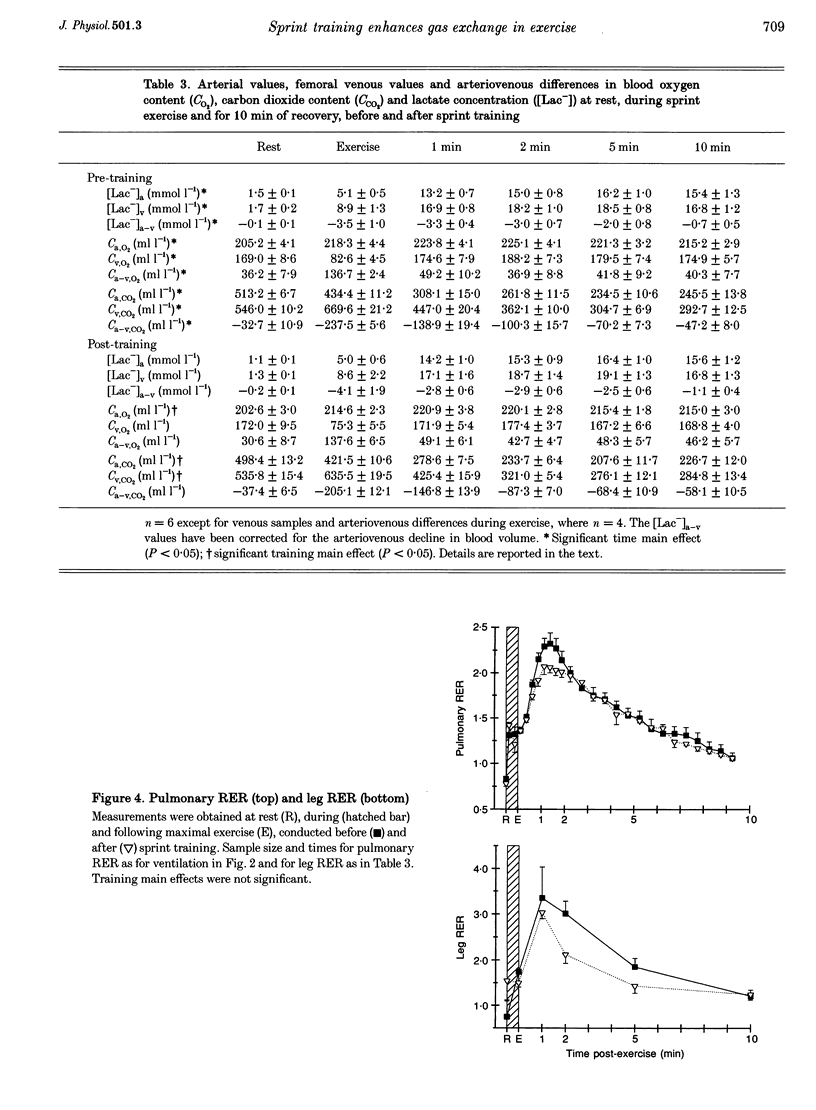
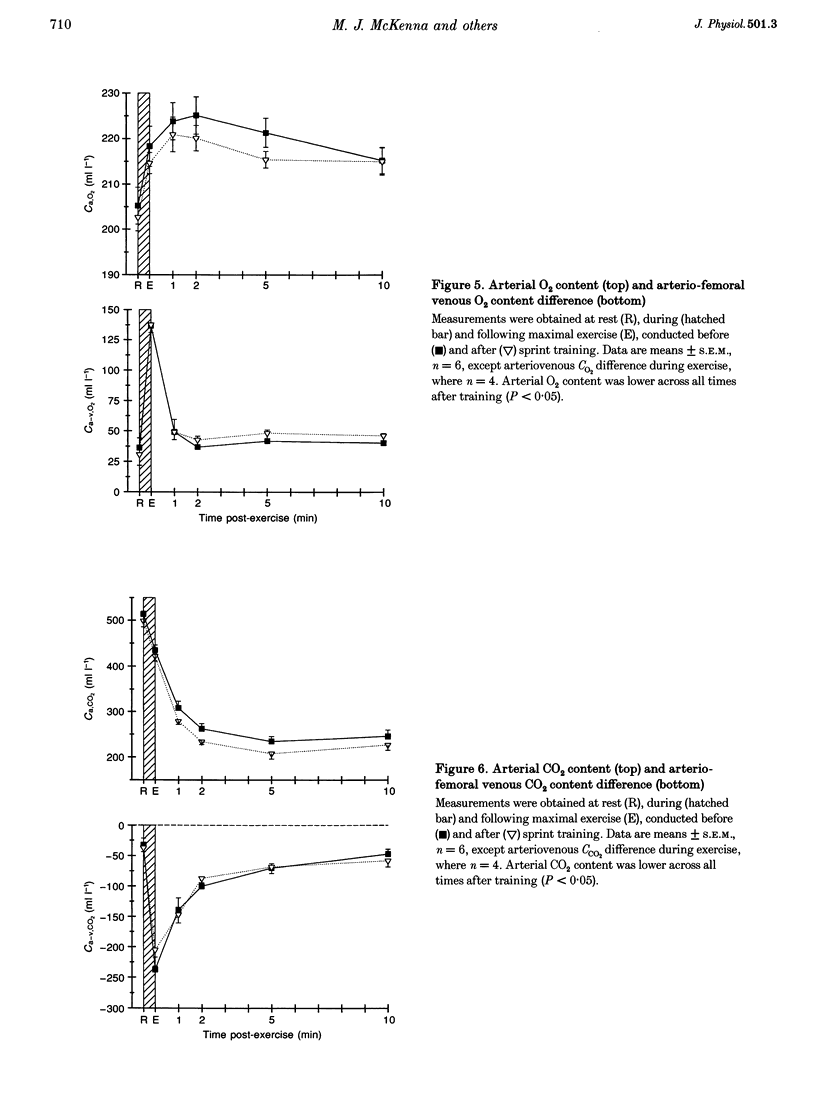
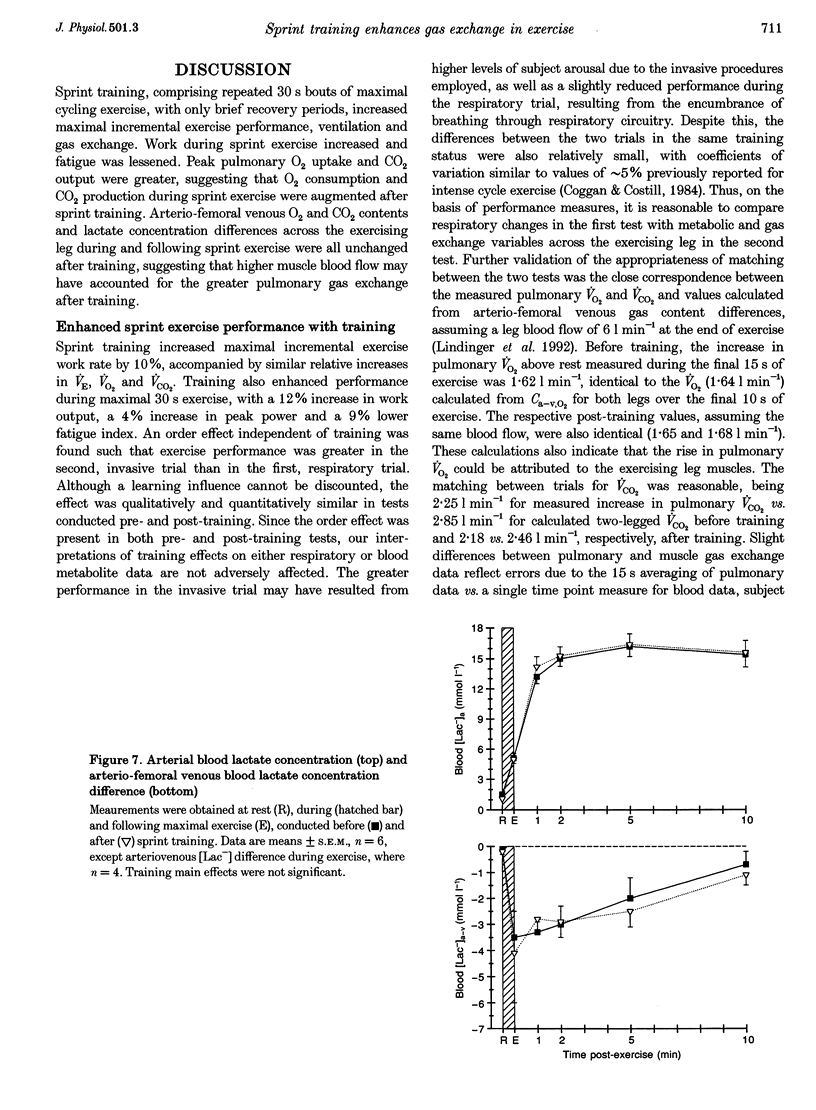
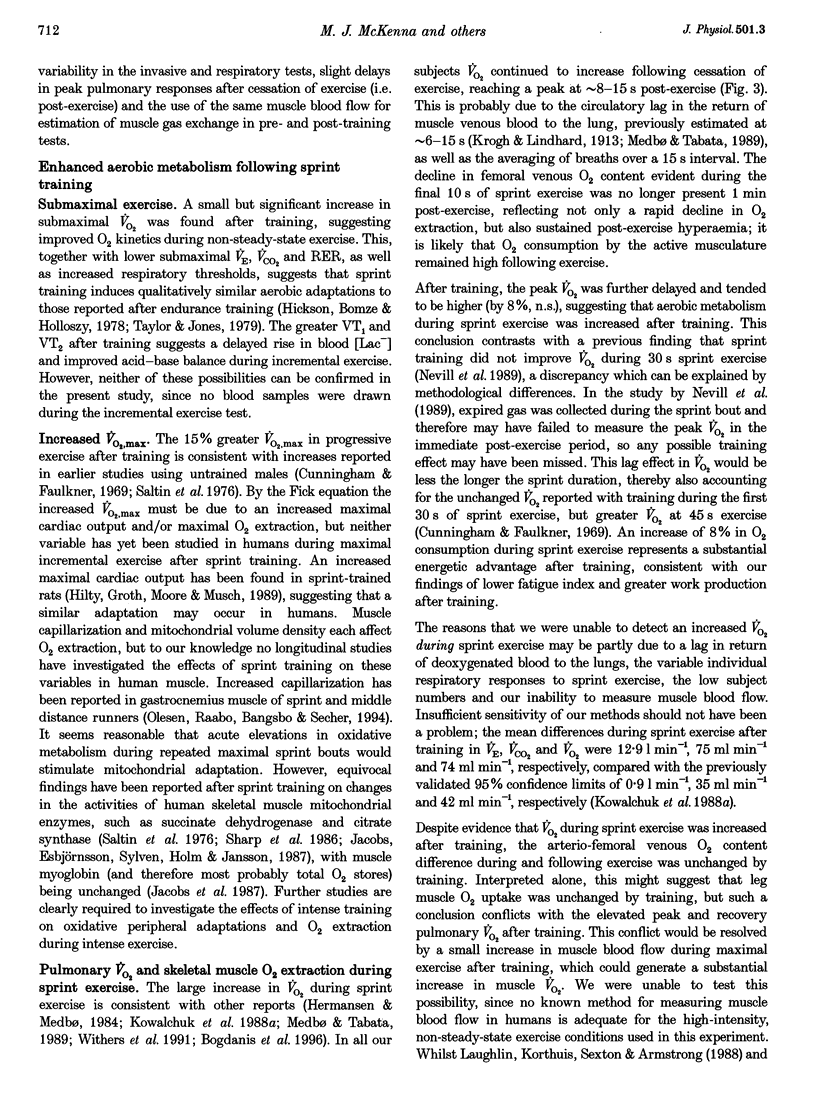
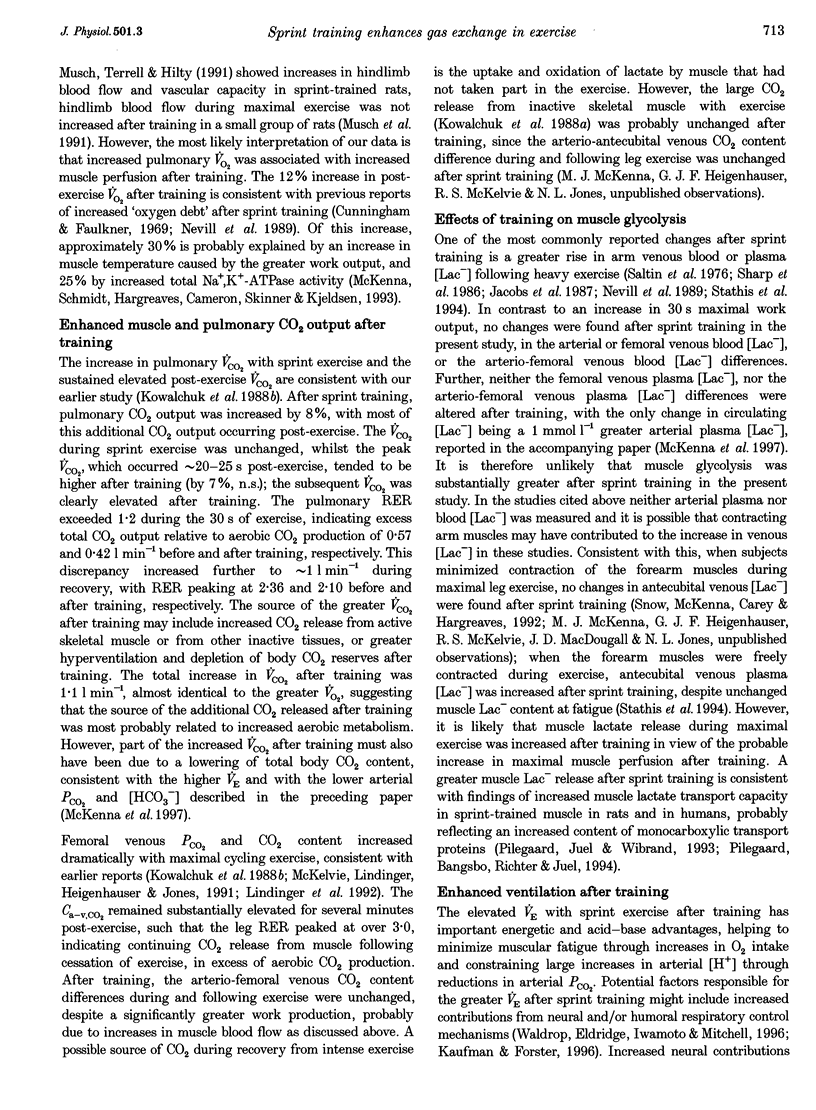
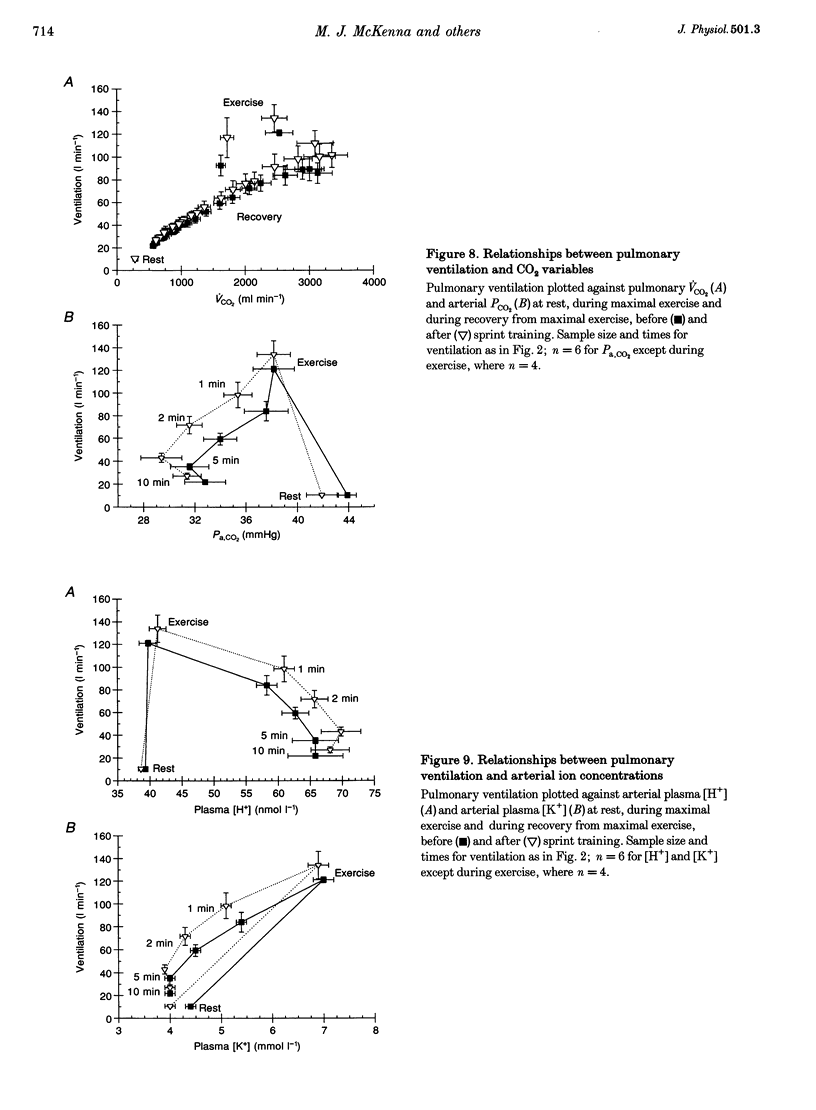
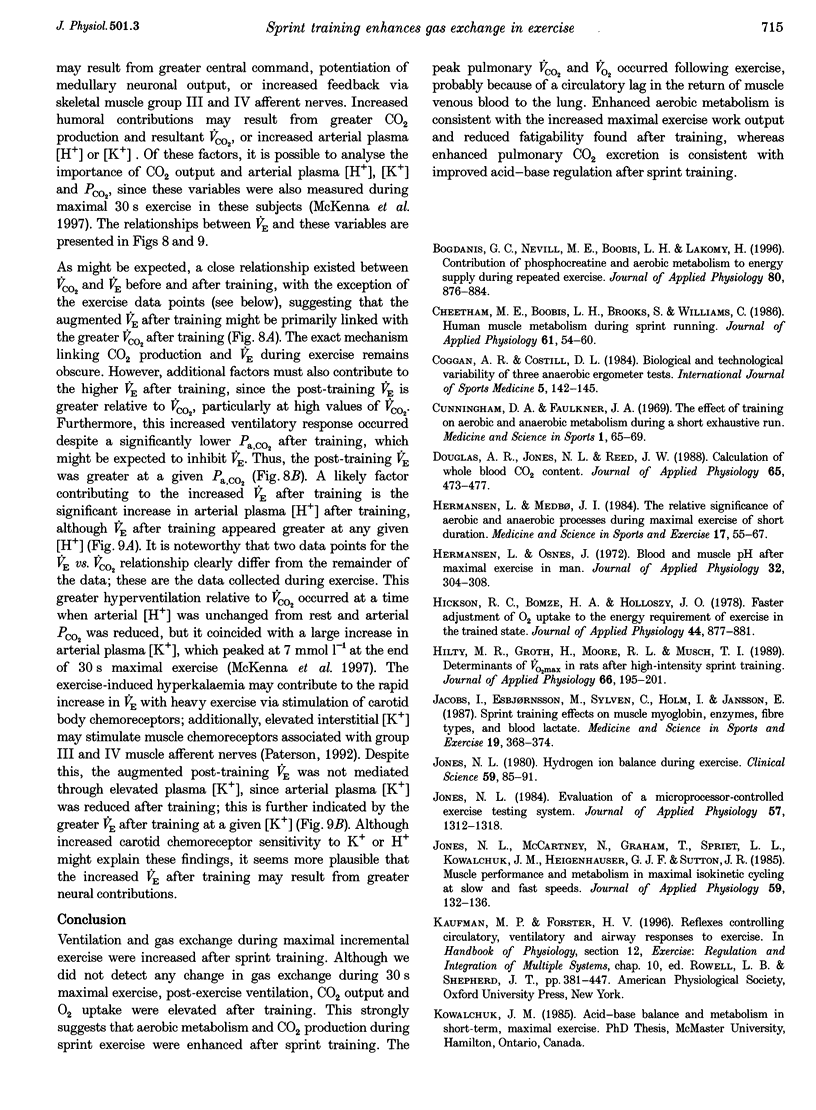
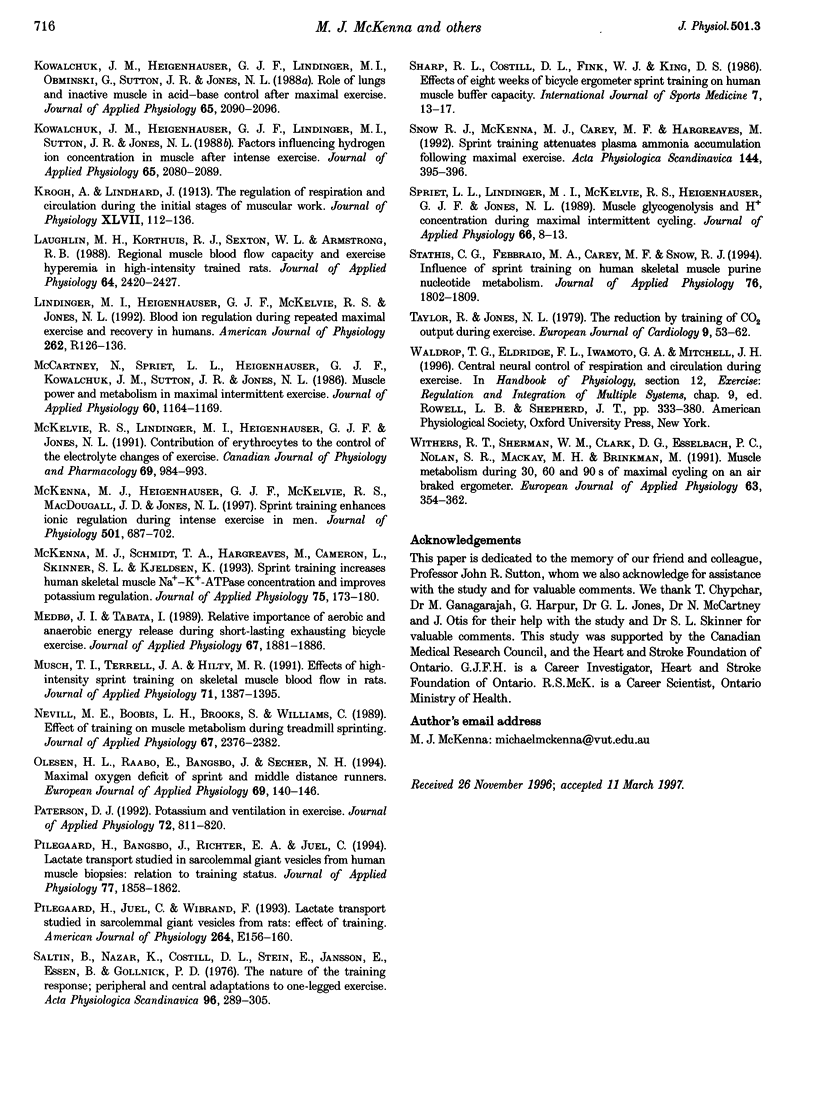
Selected References
These references are in PubMed. This may not be the complete list of references from this article.
- Aharon S., Bercovier M., Parnas H. Parallel computation enables precise description of Ca2+ distribution in nerve terminals. Bull Math Biol. 1996 Nov;58(6):1075–1097. doi: 10.1007/BF02458384. [DOI] [PubMed] [Google Scholar]
- Aharon S., Parnas H., Parnas I. The magnitude and significance of Ca2+ domains for release of neurotransmitter. Bull Math Biol. 1994 Nov;56(6):1095–1119. doi: 10.1007/BF02460288. [DOI] [PubMed] [Google Scholar]
- Augustine G. J., Charlton M. P., Smith S. J. Calcium action in synaptic transmitter release. Annu Rev Neurosci. 1987;10:633–693. doi: 10.1146/annurev.ne.10.030187.003221. [DOI] [PubMed] [Google Scholar]
- Augustine G. J., Neher E. Calcium requirements for secretion in bovine chromaffin cells. J Physiol. 1992 May;450:247–271. doi: 10.1113/jphysiol.1992.sp019126. [DOI] [PMC free article] [PubMed] [Google Scholar]
- Bers D. M. A simple method for the accurate determination of free [Ca] in Ca-EGTA solutions. Am J Physiol. 1982 May;242(5):C404–C408. doi: 10.1152/ajpcell.1982.242.5.C404. [DOI] [PubMed] [Google Scholar]
- Bogdanis G. C., Nevill M. E., Boobis L. H., Lakomy H. K. Contribution of phosphocreatine and aerobic metabolism to energy supply during repeated sprint exercise. J Appl Physiol (1985) 1996 Mar;80(3):876–884. doi: 10.1152/jappl.1996.80.3.876. [DOI] [PubMed] [Google Scholar]
- Cheetham M. E., Boobis L. H., Brooks S., Williams C. Human muscle metabolism during sprint running. J Appl Physiol (1985) 1986 Jul;61(1):54–60. doi: 10.1152/jappl.1986.61.1.54. [DOI] [PubMed] [Google Scholar]
- Coggan A. R., Costill D. L. Biological and technological variability of three anaerobic ergometer tests. Int J Sports Med. 1984 Jun;5(3):142–145. doi: 10.1055/s-2008-1025896. [DOI] [PubMed] [Google Scholar]
- Cooper R. L., Marin L., Atwood H. L. Synaptic differentiation of a single motor neuron: conjoint definition of transmitter release, presynaptic calcium signals, and ultrastructure. J Neurosci. 1995 Jun;15(6):4209–4222. doi: 10.1523/JNEUROSCI.15-06-04209.1995. [DOI] [PMC free article] [PubMed] [Google Scholar]
- Delaney K. R., Zucker R. S., Tank D. W. Calcium in motor nerve terminals associated with posttetanic potentiation. J Neurosci. 1989 Oct;9(10):3558–3567. doi: 10.1523/JNEUROSCI.09-10-03558.1989. [DOI] [PMC free article] [PubMed] [Google Scholar]
- Delaney K., Tank D. W., Zucker R. S. Presynaptic calcium and serotonin-mediated enhancement of transmitter release at crayfish neuromuscular junction. J Neurosci. 1991 Sep;11(9):2631–2643. doi: 10.1523/JNEUROSCI.11-09-02631.1991. [DOI] [PMC free article] [PubMed] [Google Scholar]
- Douglas A. R., Jones N. L., Reed J. W. Calculation of whole blood CO2 content. J Appl Physiol (1985) 1988 Jul;65(1):473–477. doi: 10.1152/jappl.1988.65.1.473. [DOI] [PubMed] [Google Scholar]
- Dudel J. The effect of reduced calcium on quantal unit current and release at the crayfish neuromuscular junction. Pflugers Arch. 1981 Jul;391(1):35–40. doi: 10.1007/BF00580691. [DOI] [PubMed] [Google Scholar]
- Fischer Y., Parnas I. Differential activation of two distinct mechanisms for presynaptic inhibition by a single inhibitory axon. J Neurophysiol. 1996 Dec;76(6):3807–3816. doi: 10.1152/jn.1996.76.6.3807. [DOI] [PubMed] [Google Scholar]
- Fogelson A. L., Zucker R. S. Presynaptic calcium diffusion from various arrays of single channels. Implications for transmitter release and synaptic facilitation. Biophys J. 1985 Dec;48(6):1003–1017. doi: 10.1016/S0006-3495(85)83863-7. [DOI] [PMC free article] [PubMed] [Google Scholar]
- Geppert M., Goda Y., Hammer R. E., Li C., Rosahl T. W., Stevens C. F., Südhof T. C. Synaptotagmin I: a major Ca2+ sensor for transmitter release at a central synapse. Cell. 1994 Nov 18;79(4):717–727. doi: 10.1016/0092-8674(94)90556-8. [DOI] [PubMed] [Google Scholar]
- Grynkiewicz G., Poenie M., Tsien R. Y. A new generation of Ca2+ indicators with greatly improved fluorescence properties. J Biol Chem. 1985 Mar 25;260(6):3440–3450. [PubMed] [Google Scholar]
- Heinemann C., Chow R. H., Neher E., Zucker R. S. Kinetics of the secretory response in bovine chromaffin cells following flash photolysis of caged Ca2+. Biophys J. 1994 Dec;67(6):2546–2557. doi: 10.1016/S0006-3495(94)80744-1. [DOI] [PMC free article] [PubMed] [Google Scholar]
- Hermansen L., Osnes J. B. Blood and muscle pH after maximal exercise in man. J Appl Physiol. 1972 Mar;32(3):304–308. doi: 10.1152/jappl.1972.32.3.304. [DOI] [PubMed] [Google Scholar]
- Hickson R. C., Bomze H. A., Hollozy J. O. Faster adjustment of O2 uptake to the energy requirement of exercise in the trained state. J Appl Physiol Respir Environ Exerc Physiol. 1978 Jun;44(6):877–881. doi: 10.1152/jappl.1978.44.6.877. [DOI] [PubMed] [Google Scholar]
- Hilty M. R., Groth H., Moore R. L., Musch T. I. Determinants of VO2max in rats after high-intensity sprint training. J Appl Physiol (1985) 1989 Jan;66(1):195–201. doi: 10.1152/jappl.1989.66.1.195. [DOI] [PubMed] [Google Scholar]
- Jacobs I., Esbjörnsson M., Sylvén C., Holm I., Jansson E. Sprint training effects on muscle myoglobin, enzymes, fiber types, and blood lactate. Med Sci Sports Exerc. 1987 Aug;19(4):368–374. [PubMed] [Google Scholar]
- Jones N. L. Evaluation of a microprocessor-controlled exercise testing system. J Appl Physiol Respir Environ Exerc Physiol. 1984 Nov;57(5):1312–1318. doi: 10.1152/jappl.1984.57.5.1312. [DOI] [PubMed] [Google Scholar]
- Jones N. L. Hydrogen ion balance during exercise. Clin Sci (Lond) 1980 Aug;59(2):85–91. doi: 10.1042/cs0590085. [DOI] [PubMed] [Google Scholar]
- Jones N. L., McCartney N., Graham T., Spriet L. L., Kowalchuk J. M., Heigenhauser G. J., Sutton J. R. Muscle performance and metabolism in maximal isokinetic cycling at slow and fast speeds. J Appl Physiol (1985) 1985 Jul;59(1):132–136. doi: 10.1152/jappl.1985.59.1.132. [DOI] [PubMed] [Google Scholar]
- Kowalchuk J. M., Heigenhauser G. J., Lindinger M. I., Obminski G., Sutton J. R., Jones N. L. Role of lungs and inactive muscle in acid-base control after maximal exercise. J Appl Physiol (1985) 1988 Nov;65(5):2090–2096. doi: 10.1152/jappl.1988.65.5.2090. [DOI] [PubMed] [Google Scholar]
- Krogh A., Lindhard J. The regulation of respiration and circulation during the initial stages of muscular work. J Physiol. 1913 Oct 17;47(1-2):112–136. doi: 10.1113/jphysiol.1913.sp001616. [DOI] [PMC free article] [PubMed] [Google Scholar]
- Landò L., Zucker R. S. Ca2+ cooperativity in neurosecretion measured using photolabile Ca2+ chelators. J Neurophysiol. 1994 Aug;72(2):825–830. doi: 10.1152/jn.1994.72.2.825. [DOI] [PubMed] [Google Scholar]
- Laughlin M. H., Korthuis R. J., Sexton W. L., Armstrong R. B. Regional muscle blood flow capacity and exercise hyperemia in high-intensity trained rats. J Appl Physiol (1985) 1988 Jun;64(6):2420–2427. doi: 10.1152/jappl.1988.64.6.2420. [DOI] [PubMed] [Google Scholar]
- Linial M., Parnas D. Deciphering neuronal secretion: tools of the trade. Biochim Biophys Acta. 1996 Jun 10;1286(2):117–152. doi: 10.1016/0304-4157(96)00007-x. [DOI] [PubMed] [Google Scholar]
- Llinás R., Steinberg I. Z., Walton K. Relationship between presynaptic calcium current and postsynaptic potential in squid giant synapse. Biophys J. 1981 Mar;33(3):323–351. doi: 10.1016/S0006-3495(81)84899-0. [DOI] [PMC free article] [PubMed] [Google Scholar]
- Llinás R., Sugimori M., Silver R. B. Time resolved calcium microdomains and synaptic transmission. J Physiol Paris. 1995;89(2):77–81. doi: 10.1016/0928-4257(96)80554-7. [DOI] [PubMed] [Google Scholar]
- McCartney N., Spriet L. L., Heigenhauser G. J., Kowalchuk J. M., Sutton J. R., Jones N. L. Muscle power and metabolism in maximal intermittent exercise. J Appl Physiol (1985) 1986 Apr;60(4):1164–1169. doi: 10.1152/jappl.1986.60.4.1164. [DOI] [PubMed] [Google Scholar]
- McKelvie R. S., Lindinger M. I., Heigenhauser G. J., Jones N. L. Contribution of erythrocytes to the control of the electrolyte changes of exercise. Can J Physiol Pharmacol. 1991 Jul;69(7):984–993. doi: 10.1139/y91-148. [DOI] [PubMed] [Google Scholar]
- McKenna M. J., Schmidt T. A., Hargreaves M., Cameron L., Skinner S. L., Kjeldsen K. Sprint training increases human skeletal muscle Na(+)-K(+)-ATPase concentration and improves K+ regulation. J Appl Physiol (1985) 1993 Jul;75(1):173–180. doi: 10.1152/jappl.1993.75.1.173. [DOI] [PubMed] [Google Scholar]
- Medbø J. I., Tabata I. Relative importance of aerobic and anaerobic energy release during short-lasting exhausting bicycle exercise. J Appl Physiol (1985) 1989 Nov;67(5):1881–1886. doi: 10.1152/jappl.1989.67.5.1881. [DOI] [PubMed] [Google Scholar]
- Miledi R. Transmitter release induced by injection of calcium ions into nerve terminals. Proc R Soc Lond B Biol Sci. 1973 Jul 3;183(1073):421–425. doi: 10.1098/rspb.1973.0026. [DOI] [PubMed] [Google Scholar]
- Mulkey R. M., Zucker R. S. Calcium released by photolysis of DM-nitrophen triggers transmitter release at the crayfish neuromuscular junction. J Physiol. 1993 Mar;462:243–260. doi: 10.1113/jphysiol.1993.sp019553. [DOI] [PMC free article] [PubMed] [Google Scholar]
- Musch T. I., Terrell J. A., Hilty M. R. Effects of high-intensity sprint training on skeletal muscle blood flow in rats. J Appl Physiol (1985) 1991 Oct;71(4):1387–1395. doi: 10.1152/jappl.1991.71.4.1387. [DOI] [PubMed] [Google Scholar]
- Neher E., Augustine G. J. Calcium gradients and buffers in bovine chromaffin cells. J Physiol. 1992 May;450:273–301. doi: 10.1113/jphysiol.1992.sp019127. [DOI] [PMC free article] [PubMed] [Google Scholar]
- Nevill M. E., Boobis L. H., Brooks S., Williams C. Effect of training on muscle metabolism during treadmill sprinting. J Appl Physiol (1985) 1989 Dec;67(6):2376–2382. doi: 10.1152/jappl.1989.67.6.2376. [DOI] [PubMed] [Google Scholar]
- Olesen H. L., Raabo E., Bangsbo J., Secher N. H. Maximal oxygen deficit of sprint and middle distance runners. Eur J Appl Physiol Occup Physiol. 1994;69(2):140–146. doi: 10.1007/BF00609406. [DOI] [PubMed] [Google Scholar]
- Parnas H., Segel L. A. A theoretical explanation for some effects of calcium on the facilitation of neurotransmitter release. J Theor Biol. 1980 May 7;84(1):3–29. doi: 10.1016/s0022-5193(80)81035-6. [DOI] [PubMed] [Google Scholar]
- Parnas I., Dudel J., Parnas H., Ravin R. Glutamate depresses release by activating non-conventional glutamate receptors at crayfish nerve terminals. Eur J Neurosci. 1996 Jan;8(1):116–126. doi: 10.1111/j.1460-9568.1996.tb01172.x. [DOI] [PubMed] [Google Scholar]
- Parnas I., Parnas H., Dudel J. Neurotransmitter release and its facilitation in crayfish muscle. V. Basis for synapse differentiation of the fast and slow type in one axon. Pflugers Arch. 1982 Dec;395(4):261–270. doi: 10.1007/BF00580788. [DOI] [PubMed] [Google Scholar]
- Paterson D. J. Potassium and ventilation in exercise. J Appl Physiol (1985) 1992 Mar;72(3):811–820. doi: 10.1152/jappl.1992.72.3.811. [DOI] [PubMed] [Google Scholar]
- Pilegaard H., Bangsbo J., Richter E. A., Juel C. Lactate transport studied in sarcolemmal giant vesicles from human muscle biopsies: relation to training status. J Appl Physiol (1985) 1994 Oct;77(4):1858–1862. doi: 10.1152/jappl.1994.77.4.1858. [DOI] [PubMed] [Google Scholar]
- Pilegaard H., Juel C., Wibrand F. Lactate transport studied in sarcolemmal giant vesicles from rats: effect of training. Am J Physiol. 1993 Feb;264(2 Pt 1):E156–E160. doi: 10.1152/ajpendo.1993.264.2.E156. [DOI] [PubMed] [Google Scholar]
- Rahamimoff R., Meiri H., Erulkar S. D., Barenholz Y. Changes in transmitter release induced by ion-containing liposomes. Proc Natl Acad Sci U S A. 1978 Oct;75(10):5214–5216. doi: 10.1073/pnas.75.10.5214. [DOI] [PMC free article] [PubMed] [Google Scholar]
- Saltin B., Nazar K., Costill D. L., Stein E., Jansson E., Essén B., Gollnick D. The nature of the training response; peripheral and central adaptations of one-legged exercise. Acta Physiol Scand. 1976 Mar;96(3):289–305. doi: 10.1111/j.1748-1716.1976.tb10200.x. [DOI] [PubMed] [Google Scholar]
- Sharp R. L., Costill D. L., Fink W. J., King D. S. Effects of eight weeks of bicycle ergometer sprint training on human muscle buffer capacity. Int J Sports Med. 1986 Feb;7(1):13–17. doi: 10.1055/s-2008-1025727. [DOI] [PubMed] [Google Scholar]
- Simon S. M., Llinás R. R. Compartmentalization of the submembrane calcium activity during calcium influx and its significance in transmitter release. Biophys J. 1985 Sep;48(3):485–498. doi: 10.1016/S0006-3495(85)83804-2. [DOI] [PMC free article] [PubMed] [Google Scholar]
- Smith S. J., Augustine G. J., Charlton M. P. Transmission at voltage-clamped giant synapse of the squid: evidence for cooperativity of presynaptic calcium action. Proc Natl Acad Sci U S A. 1985 Jan;82(2):622–625. doi: 10.1073/pnas.82.2.622. [DOI] [PMC free article] [PubMed] [Google Scholar]
- Snow R. J., McKenna M. J., Carey M. F., Hargreaves M. Sprint training attenuates plasma ammonia accumulation following maximal exercise. Acta Physiol Scand. 1992 Mar;144(3):395–396. doi: 10.1111/j.1748-1716.1992.tb09310.x. [DOI] [PubMed] [Google Scholar]
- Spriet L. L., Lindinger M. I., McKelvie R. S., Heigenhauser G. J., Jones N. L. Muscle glycogenolysis and H+ concentration during maximal intermittent cycling. J Appl Physiol (1985) 1989 Jan;66(1):8–13. doi: 10.1152/jappl.1989.66.1.8. [DOI] [PubMed] [Google Scholar]
- Stathis C. G., Febbraio M. A., Carey M. F., Snow R. J. Influence of sprint training on human skeletal muscle purine nucleotide metabolism. J Appl Physiol (1985) 1994 Apr;76(4):1802–1809. doi: 10.1152/jappl.1994.76.4.1802. [DOI] [PubMed] [Google Scholar]
- Tank D. W., Regehr W. G., Delaney K. R. A quantitative analysis of presynaptic calcium dynamics that contribute to short-term enhancement. J Neurosci. 1995 Dec;15(12):7940–7952. doi: 10.1523/JNEUROSCI.15-12-07940.1995. [DOI] [PMC free article] [PubMed] [Google Scholar]
- Taylor R., Jones N. L. The reduction by training of CO2 output during exercise. Eur J Cardiol. 1979 Jan;9(1):53–62. [PubMed] [Google Scholar]
- Wallin B. G. Intracellular ion concentrations in single crayfish axons. Acta Physiol Scand. 1967 Jul-Aug;70(3):419–430. doi: 10.1111/j.1748-1716.1967.tb03640.x. [DOI] [PubMed] [Google Scholar]
- Withers R. T., Sherman W. M., Clark D. G., Esselbach P. C., Nolan S. R., Mackay M. H., Brinkman M. Muscle metabolism during 30, 60 and 90 s of maximal cycling on an air-braked ergometer. Eur J Appl Physiol Occup Physiol. 1991;63(5):354–362. doi: 10.1007/BF00364462. [DOI] [PubMed] [Google Scholar]
- Wojtowicz J. M., Atwood H. L. Presynaptic membrane potential and transmitter release at the crayfish neuromuscular junction. J Neurophysiol. 1984 Jul;52(1):99–113. doi: 10.1152/jn.1984.52.1.99. [DOI] [PubMed] [Google Scholar]
- Yamada W. M., Zucker R. S. Time course of transmitter release calculated from simulations of a calcium diffusion model. Biophys J. 1992 Mar;61(3):671–682. doi: 10.1016/S0006-3495(92)81872-6. [DOI] [PMC free article] [PubMed] [Google Scholar]
- Ziv N. E., Spira M. E. Axotomy induces a transient and localized elevation of the free intracellular calcium concentration to the millimolar range. J Neurophysiol. 1995 Dec;74(6):2625–2637. doi: 10.1152/jn.1995.74.6.2625. [DOI] [PubMed] [Google Scholar]
- Ziv N. E., Spira M. E. Spatiotemporal distribution of Ca2+ following axotomy and throughout the recovery process of cultured Aplysia neurons. Eur J Neurosci. 1993 Jun 1;5(6):657–668. doi: 10.1111/j.1460-9568.1993.tb00531.x. [DOI] [PubMed] [Google Scholar]
- Zucker R. S., Delaney K. R., Mulkey R., Tank D. W. Presynaptic calcium in transmitter release and posttetanic potentiation. Ann N Y Acad Sci. 1991;635:191–207. doi: 10.1111/j.1749-6632.1991.tb36492.x. [DOI] [PubMed] [Google Scholar]