Abstract
1. The relationship between force and velocity of shortening was studied during fused tetani of single fibres isolated from the anterior tibialis muscle of Rana temporaria (1.5-3.3 degrees C; sarcomere length, 2.20 microns). Stiffness was measured as the change in force that occurred in response to a 4 kHz length oscillation of the fibre. 2. The results confirmed the existence of two distinct curvatures of the force-velocity relationship located on either side of a breakpoint in the high-force, low-velocity range. Reduction of the isometric force (P0) to 83.4 +/- 1.7% (mean +/- S.E.M., n = 5) of the control value by dantrolene did not affect the relative shape of the force-velocity relationship. The breakpoint between the two curvatures was located at 75.9 +/- 0.9% of P0 and 11.4 +/- 0.6% of maximum velocity of shortening (Vmax) in control Ringer solution and at 75.6 +/- 0.7% of P0 and 12.2 +/- 0.7% of Vmax in the presence of dantrolene. These results provide evidence that the transition between the two curvatures of the force-velocity relationship is primarily related to the speed of shortening, not to the actual force within the fibre. 3. The instantaneous stiffness varied with the speed of shortening forming a biphasic relationship with a breakpoint near 0.15 Vmax and 0.8 P0, respectively. The force/stiffness ratio (probably reflecting the average force per cross-bridge), increased with force during shortening. The increase of the force/stiffness ratio with force was less steep at forces exceeding 0.8 P0 than below this point. 4. A four-state cross-bridge model (described in the Appendix) was used to evaluate the experimental results. The model reproduces with great precision the characteristic features of the force-stiffness-velocity relationships recorded in intact muscle fibres.
Full text
PDF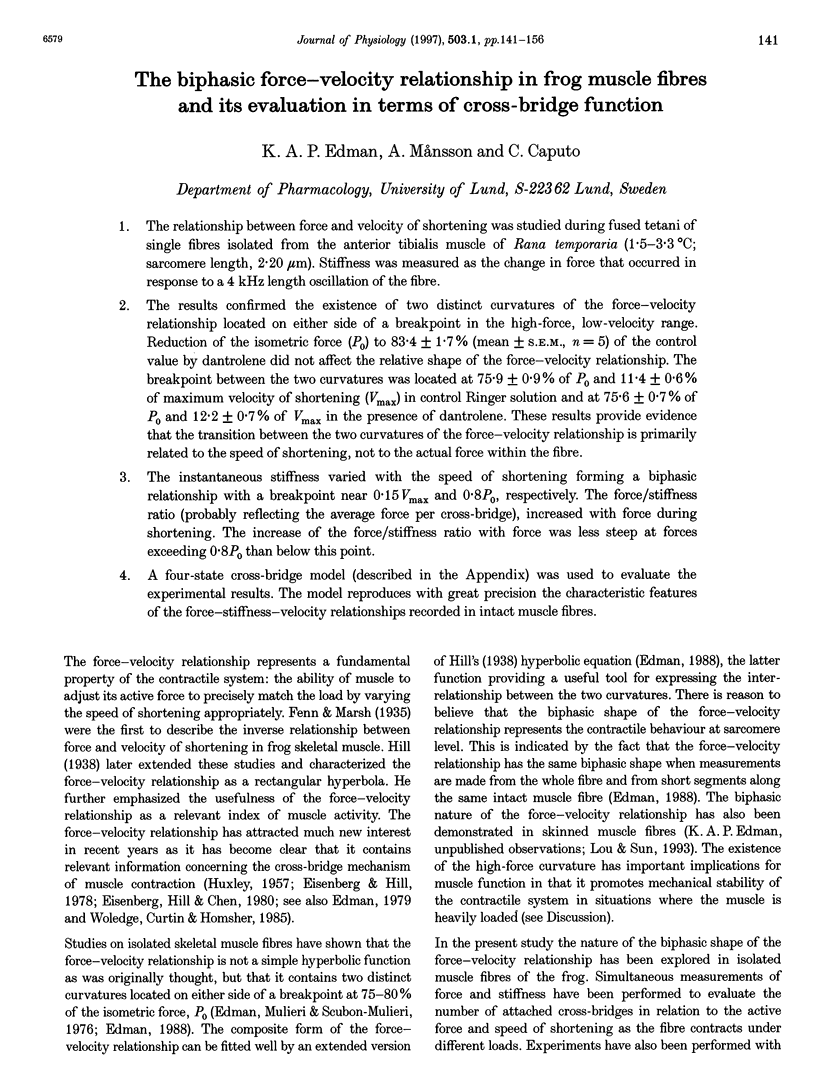
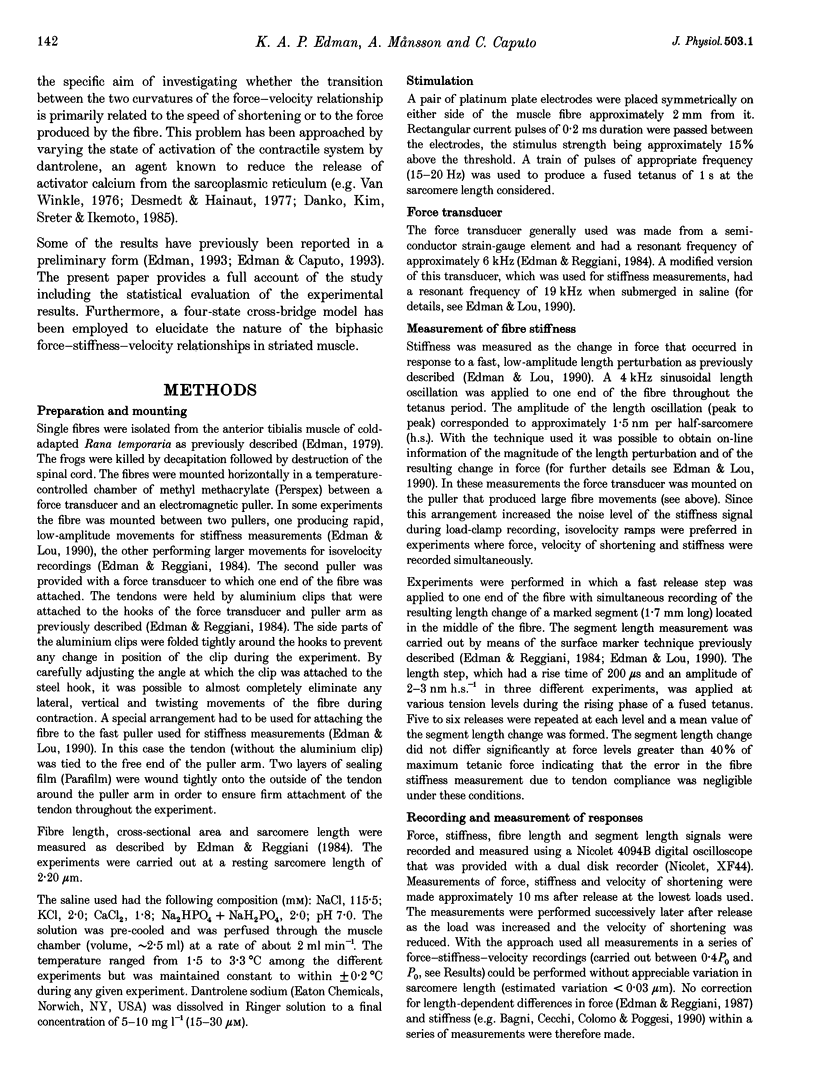
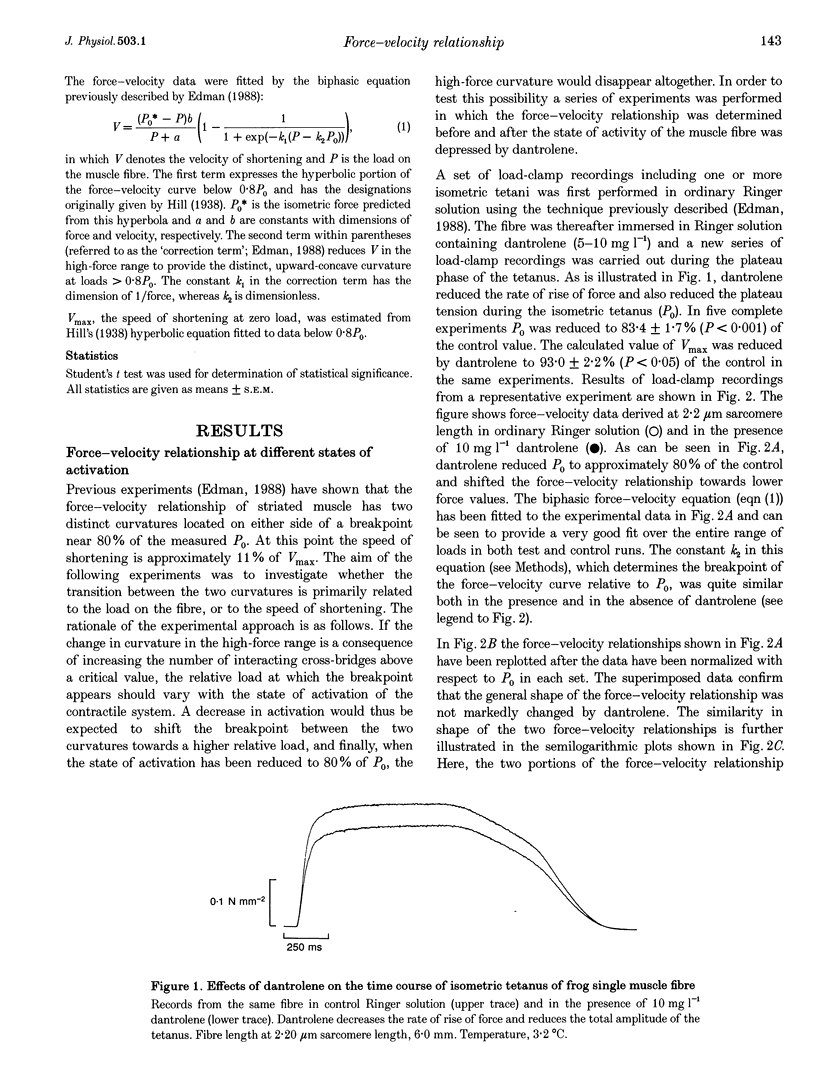
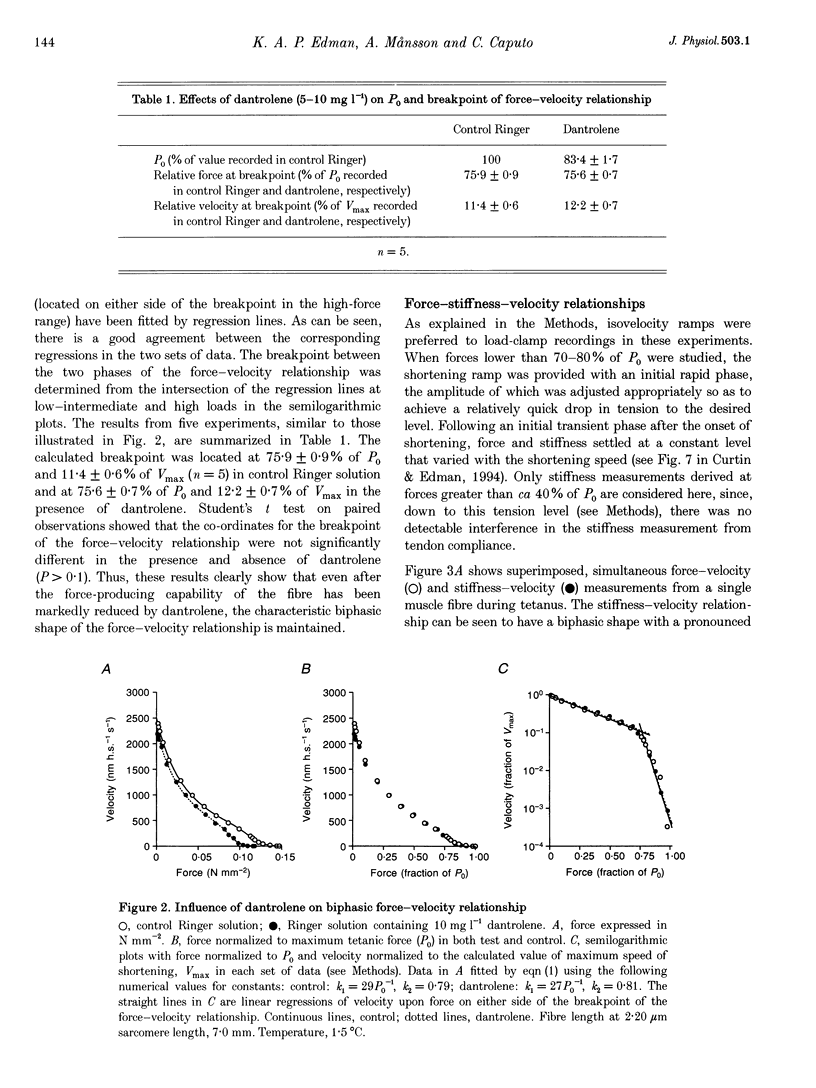
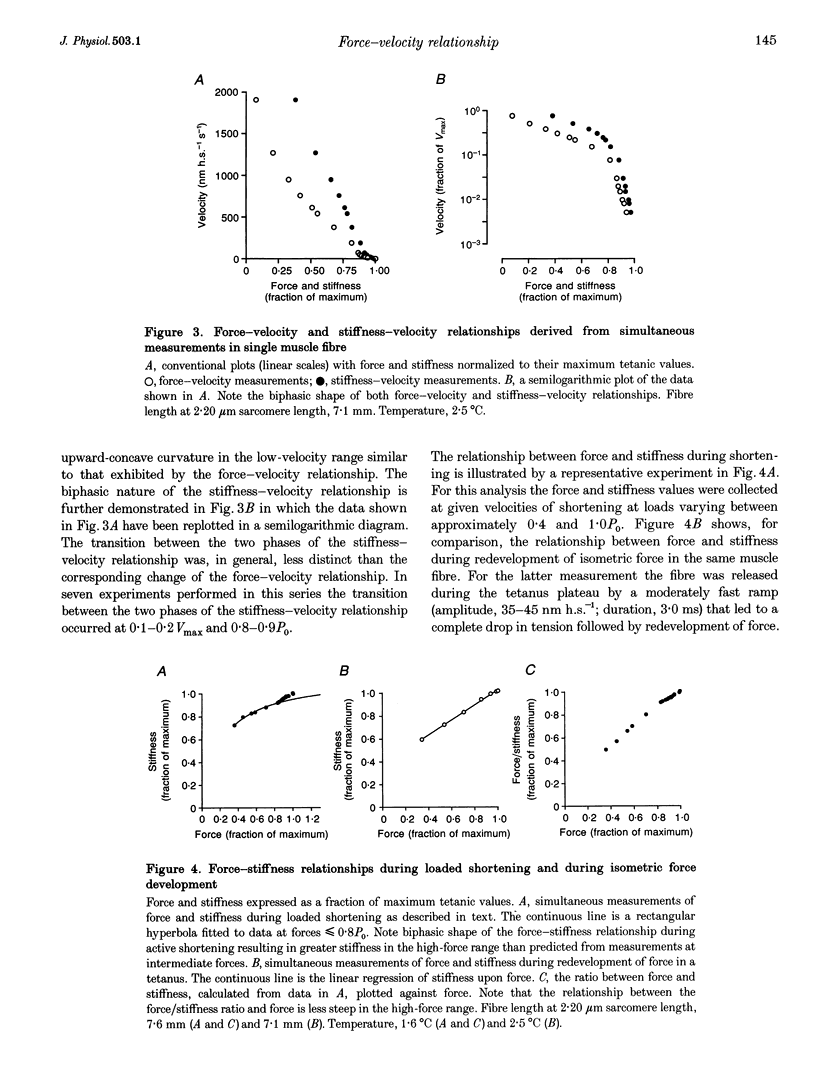
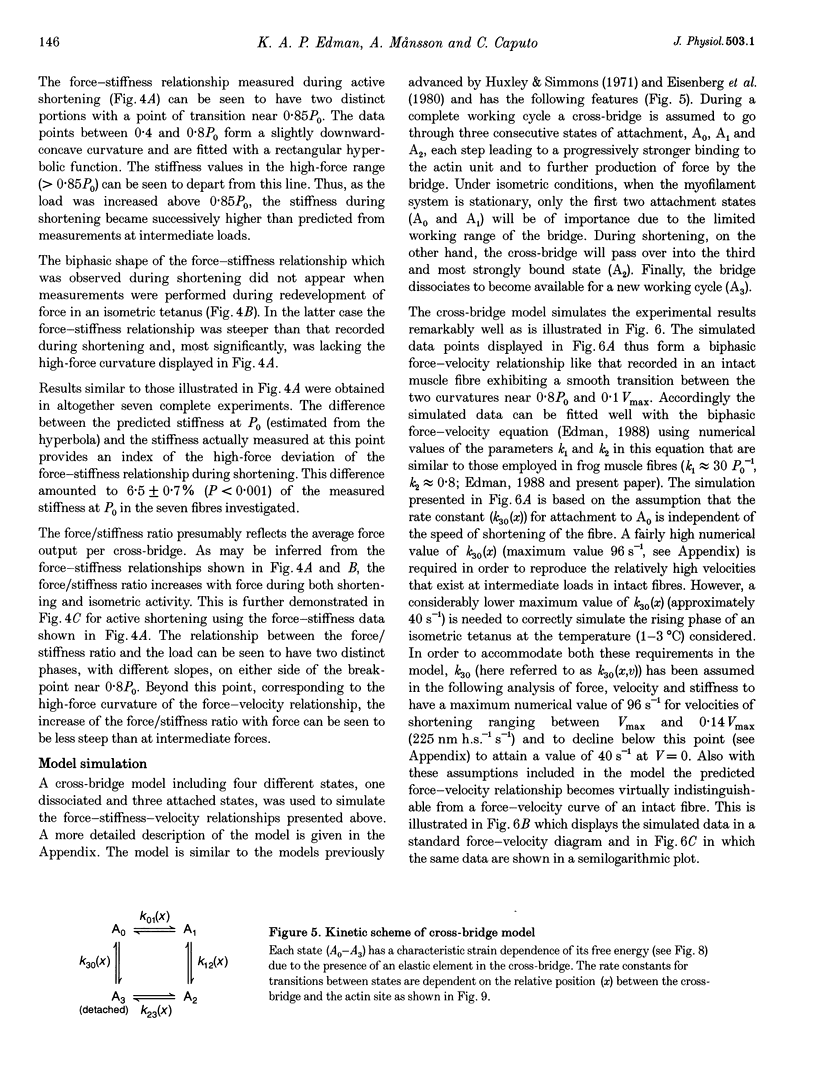
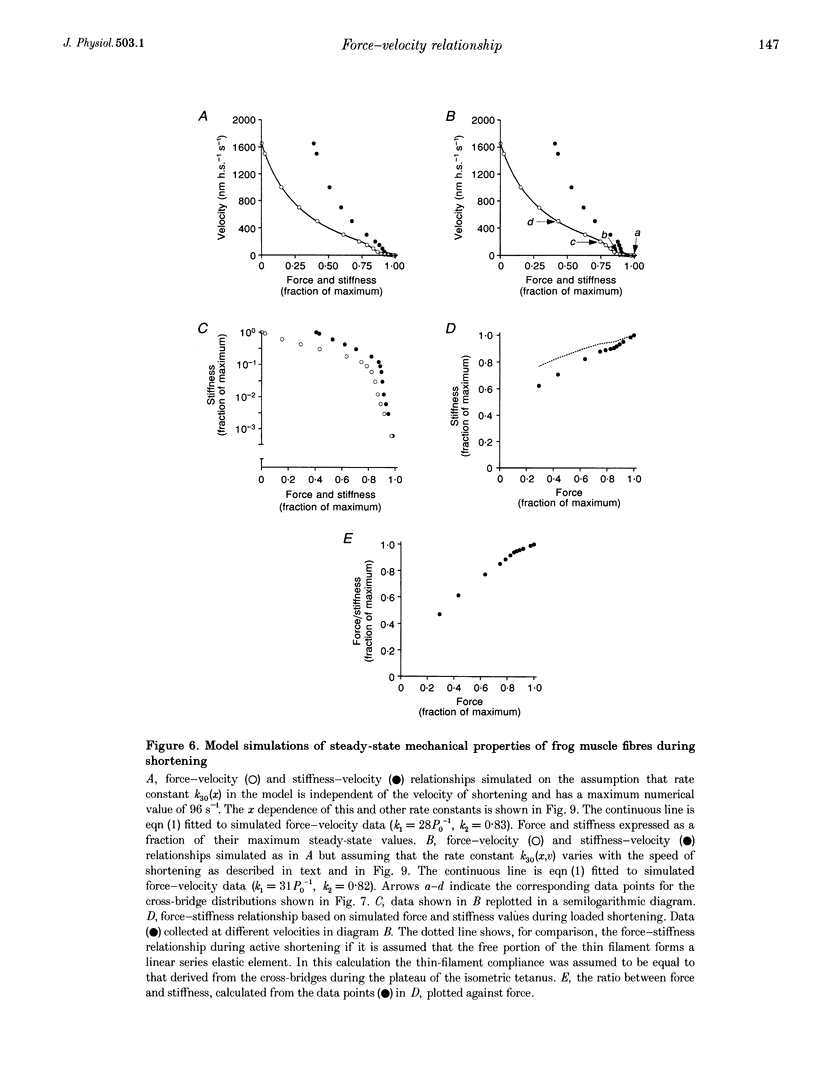
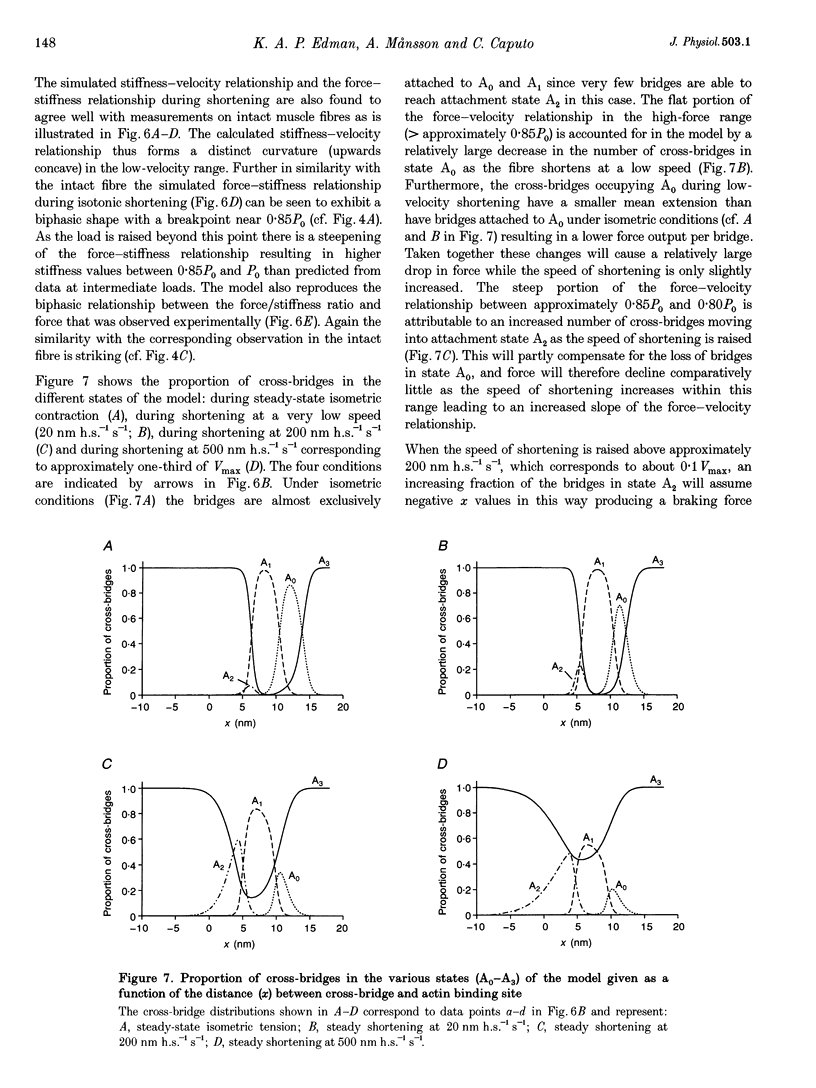
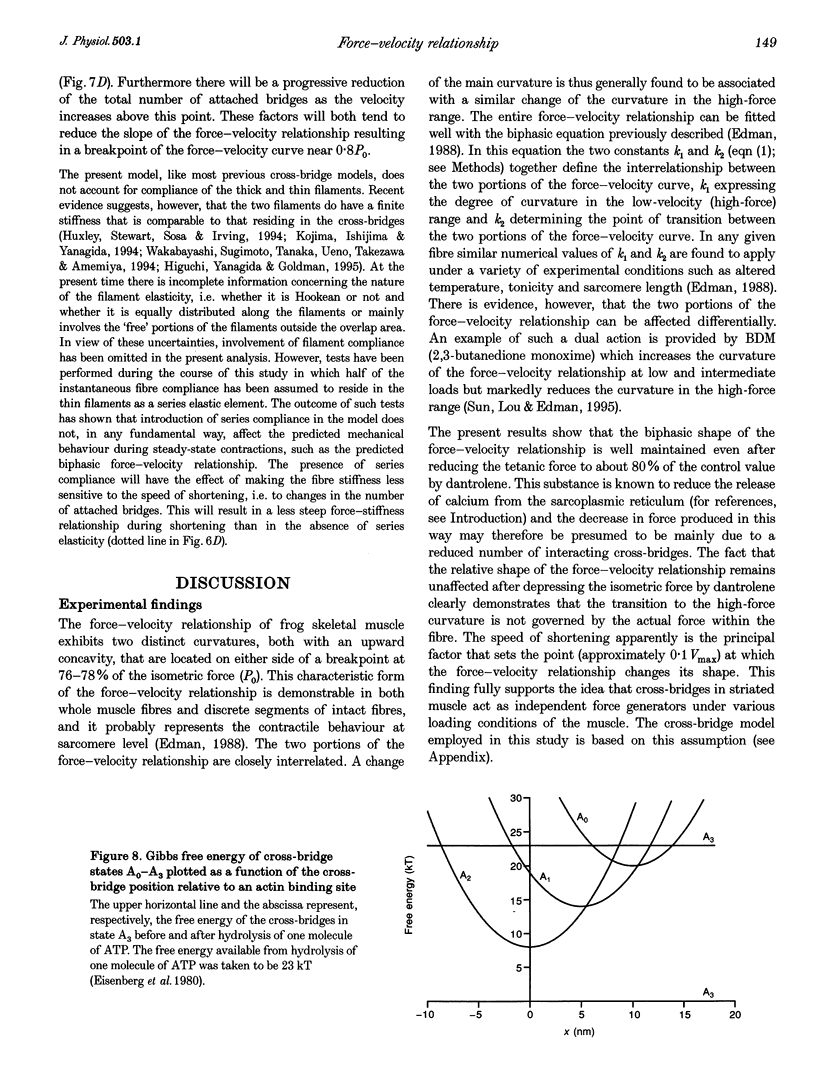
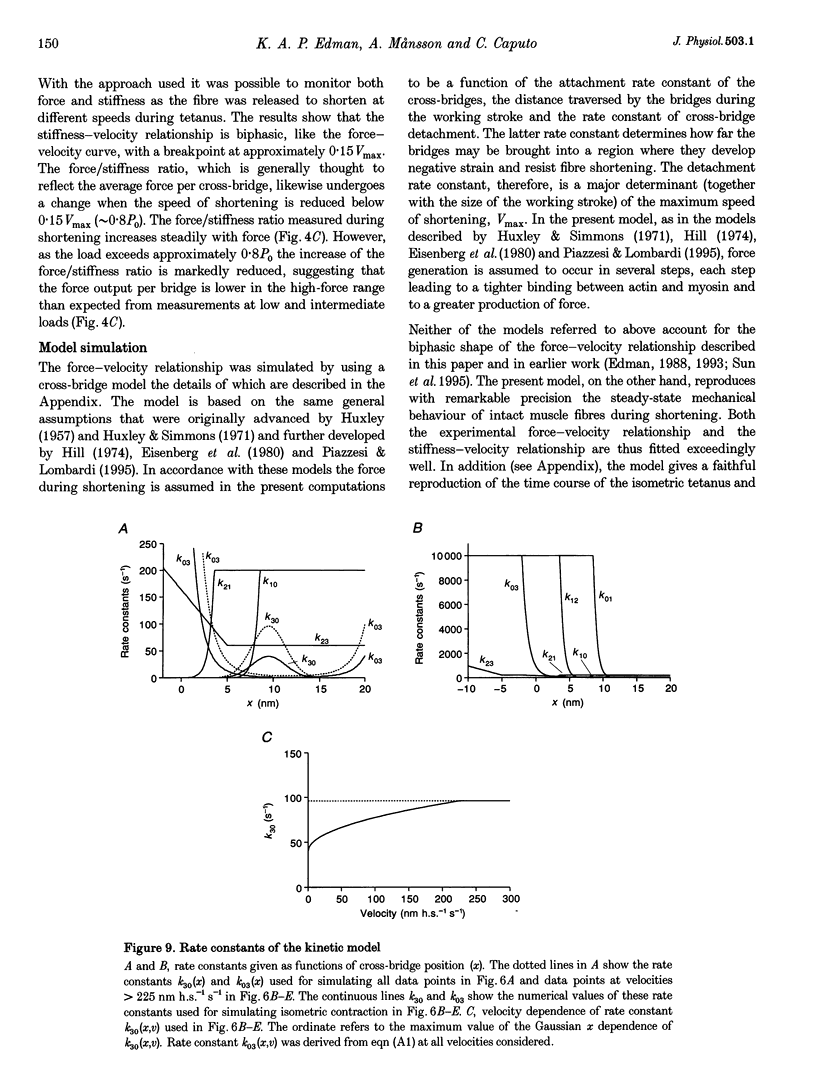
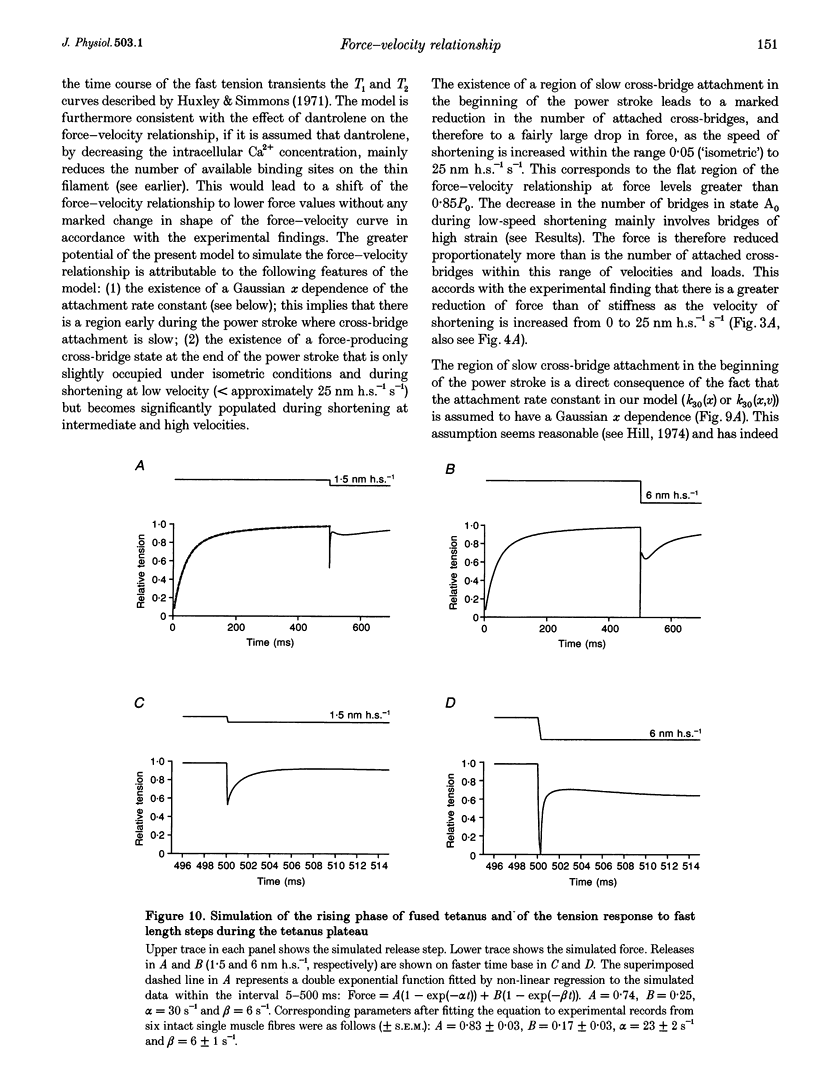
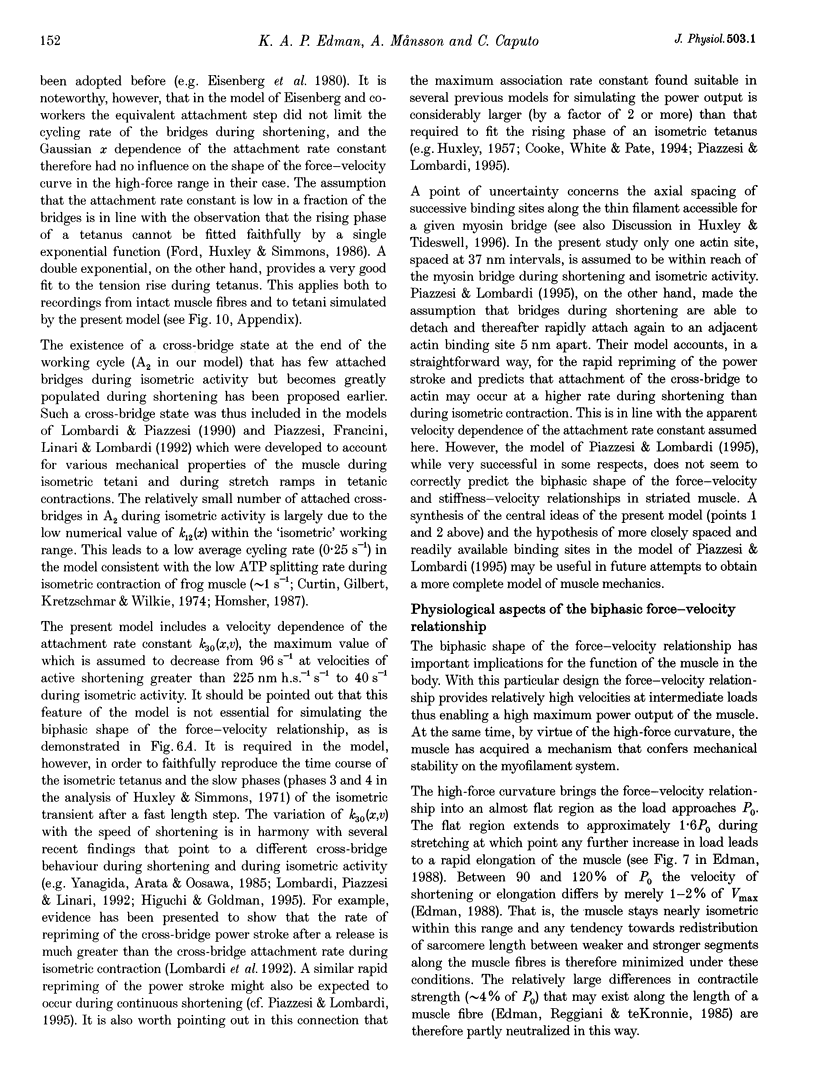
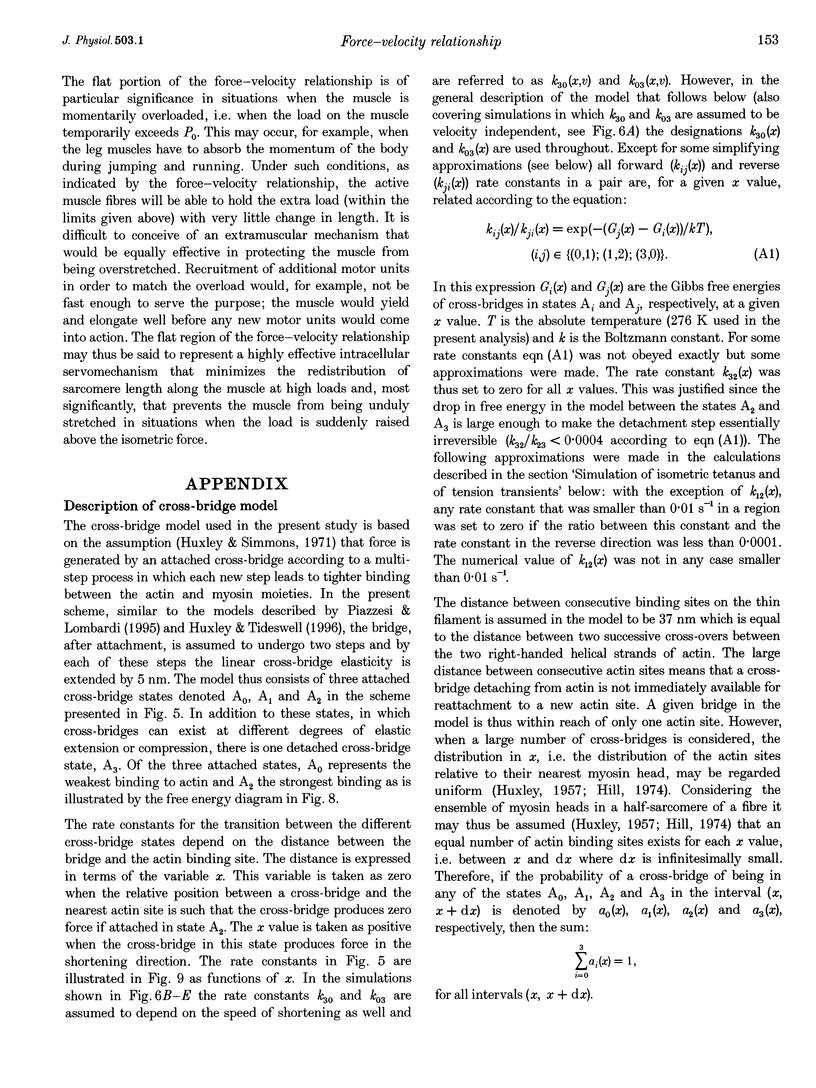
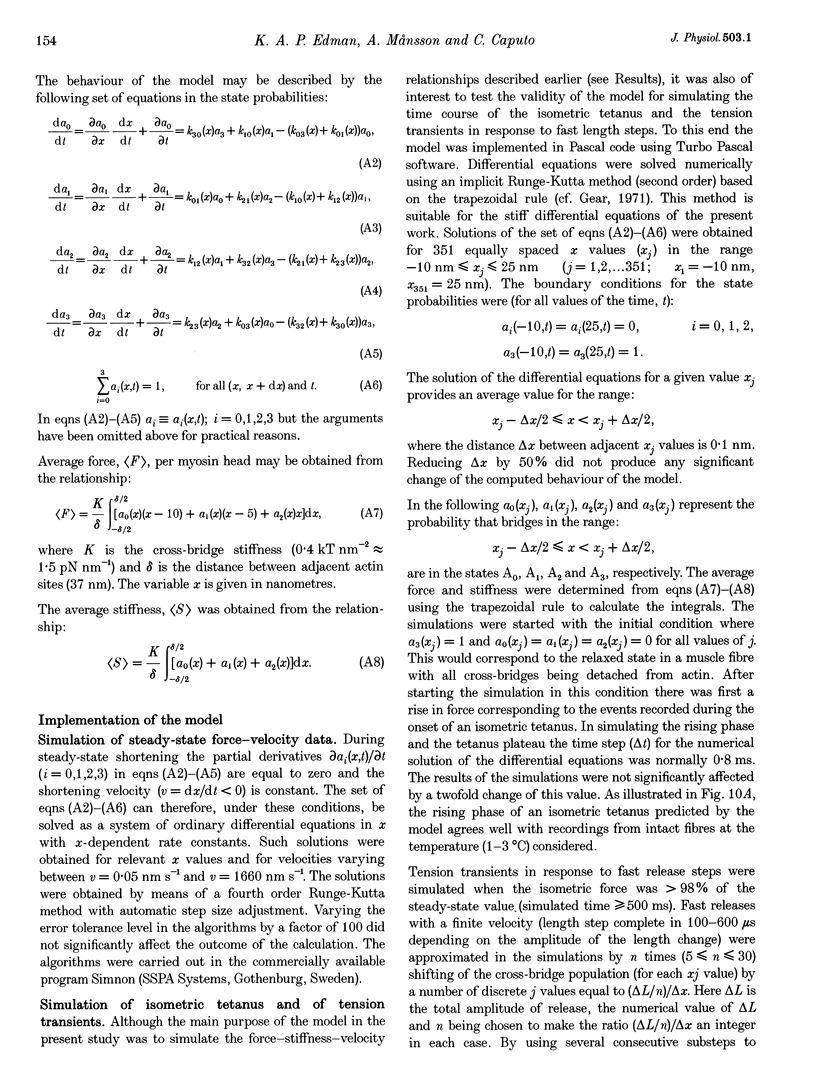
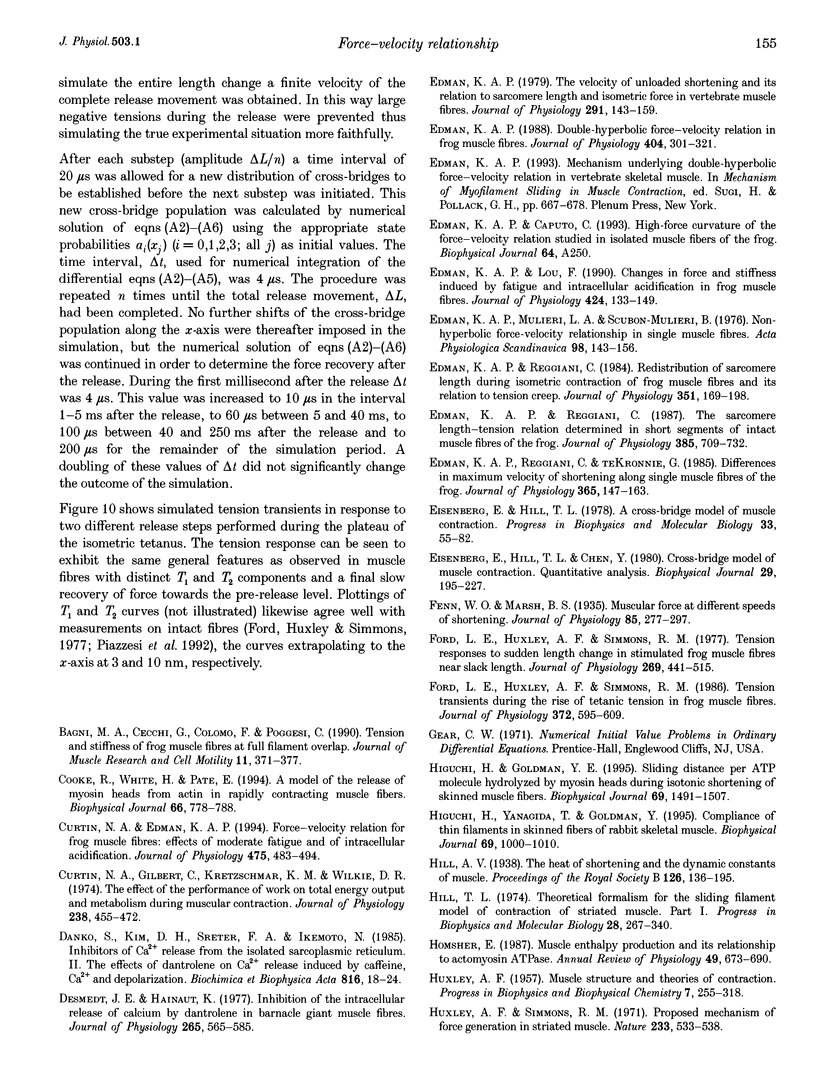
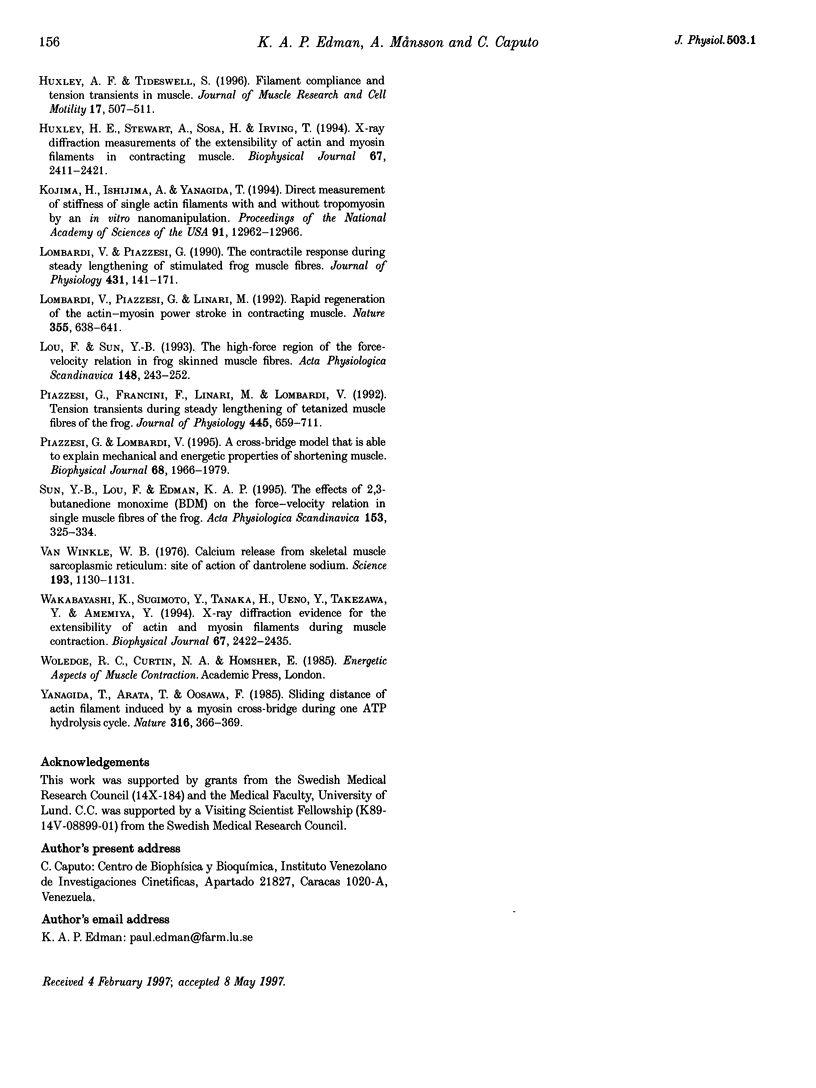
Selected References
These references are in PubMed. This may not be the complete list of references from this article.
- Bagni M. A., Cecchi G., Colomo F., Poggesi C. Tension and stiffness of frog muscle fibres at full filament overlap. J Muscle Res Cell Motil. 1990 Oct;11(5):371–377. doi: 10.1007/BF01739758. [DOI] [PubMed] [Google Scholar]
- Cooke R., White H., Pate E. A model of the release of myosin heads from actin in rapidly contracting muscle fibers. Biophys J. 1994 Mar;66(3 Pt 1):778–788. doi: 10.1016/s0006-3495(94)80854-9. [DOI] [PMC free article] [PubMed] [Google Scholar]
- Curtin N. A., Edman K. A. Force-velocity relation for frog muscle fibres: effects of moderate fatigue and of intracellular acidification. J Physiol. 1994 Mar 15;475(3):483–494. doi: 10.1113/jphysiol.1994.sp020087. [DOI] [PMC free article] [PubMed] [Google Scholar]
- Curtin N. A., Gilbert C., Kretzschmar K. M., Wilkie D. R. The effect of the performance of work on total energy output and metabolism during muscular contraction. J Physiol. 1974 May;238(3):455–472. doi: 10.1113/jphysiol.1974.sp010537. [DOI] [PMC free article] [PubMed] [Google Scholar]
- Danko S., Kim D. H., Sreter F. A., Ikemoto N. Inhibitors of Ca2+ release from the isolated sarcoplasmic reticulum. II. The effects of dantrolene on Ca2+ release induced by caffeine, Ca2+ and depolarization. Biochim Biophys Acta. 1985 Jun 11;816(1):18–24. doi: 10.1016/0005-2736(85)90388-8. [DOI] [PubMed] [Google Scholar]
- Desmedt J. E., Hainaut K. Inhibition of the intracellular release of calcium by Dantrolene in barnacle giant muscle fibres. J Physiol. 1977 Feb;265(2):565–585. doi: 10.1113/jphysiol.1977.sp011731. [DOI] [PMC free article] [PubMed] [Google Scholar]
- Edman K. A. Double-hyperbolic force-velocity relation in frog muscle fibres. J Physiol. 1988 Oct;404:301–321. doi: 10.1113/jphysiol.1988.sp017291. [DOI] [PMC free article] [PubMed] [Google Scholar]
- Edman K. A., Lou F. Changes in force and stiffness induced by fatigue and intracellular acidification in frog muscle fibres. J Physiol. 1990 May;424:133–149. doi: 10.1113/jphysiol.1990.sp018059. [DOI] [PMC free article] [PubMed] [Google Scholar]
- Edman K. A. Mechanism underlying double-hyperbolic force-velocity relation in vertebrate skeletal muscle. Adv Exp Med Biol. 1993;332:667–678. doi: 10.1007/978-1-4615-2872-2_59. [DOI] [PubMed] [Google Scholar]
- Edman K. A., Mulieri L. A., Scubon-Mulieri B. Non-hyperbolic force-velocity relationship in single muscle fibres. Acta Physiol Scand. 1976 Oct;98(2):143–156. doi: 10.1111/j.1748-1716.1976.tb00234.x. [DOI] [PubMed] [Google Scholar]
- Edman K. A., Reggiani C. Redistribution of sarcomere length during isometric contraction of frog muscle fibres and its relation to tension creep. J Physiol. 1984 Jun;351:169–198. doi: 10.1113/jphysiol.1984.sp015240. [DOI] [PMC free article] [PubMed] [Google Scholar]
- Edman K. A., Reggiani C. The sarcomere length-tension relation determined in short segments of intact muscle fibres of the frog. J Physiol. 1987 Apr;385:709–732. doi: 10.1113/jphysiol.1987.sp016516. [DOI] [PMC free article] [PubMed] [Google Scholar]
- Edman K. A., Reggiani C., te Kronnie G. Differences in maximum velocity of shortening along single muscle fibres of the frog. J Physiol. 1985 Aug;365:147–163. doi: 10.1113/jphysiol.1985.sp015764. [DOI] [PMC free article] [PubMed] [Google Scholar]
- Edman K. A. The velocity of unloaded shortening and its relation to sarcomere length and isometric force in vertebrate muscle fibres. J Physiol. 1979 Jun;291:143–159. doi: 10.1113/jphysiol.1979.sp012804. [DOI] [PMC free article] [PubMed] [Google Scholar]
- Eisenberg E., Hill T. L. A cross-bridge model of muscle contraction. Prog Biophys Mol Biol. 1978;33(1):55–82. doi: 10.1016/0079-6107(79)90025-7. [DOI] [PubMed] [Google Scholar]
- Eisenberg E., Hill T. L., Chen Y. Cross-bridge model of muscle contraction. Quantitative analysis. Biophys J. 1980 Feb;29(2):195–227. doi: 10.1016/S0006-3495(80)85126-5. [DOI] [PMC free article] [PubMed] [Google Scholar]
- Fenn W. O., Marsh B. S. Muscular force at different speeds of shortening. J Physiol. 1935 Nov 22;85(3):277–297. doi: 10.1113/jphysiol.1935.sp003318. [DOI] [PMC free article] [PubMed] [Google Scholar]
- Ford L. E., Huxley A. F., Simmons R. M. Tension responses to sudden length change in stimulated frog muscle fibres near slack length. J Physiol. 1977 Jul;269(2):441–515. doi: 10.1113/jphysiol.1977.sp011911. [DOI] [PMC free article] [PubMed] [Google Scholar]
- Ford L. E., Huxley A. F., Simmons R. M. Tension transients during the rise of tetanic tension in frog muscle fibres. J Physiol. 1986 Mar;372:595–609. doi: 10.1113/jphysiol.1986.sp016027. [DOI] [PMC free article] [PubMed] [Google Scholar]
- HUXLEY A. F. Muscle structure and theories of contraction. Prog Biophys Biophys Chem. 1957;7:255–318. [PubMed] [Google Scholar]
- Higuchi H., Goldman Y. E. Sliding distance per ATP molecule hydrolyzed by myosin heads during isotonic shortening of skinned muscle fibers. Biophys J. 1995 Oct;69(4):1491–1507. doi: 10.1016/S0006-3495(95)80020-2. [DOI] [PMC free article] [PubMed] [Google Scholar]
- Higuchi H., Yanagida T., Goldman Y. E. Compliance of thin filaments in skinned fibers of rabbit skeletal muscle. Biophys J. 1995 Sep;69(3):1000–1010. doi: 10.1016/S0006-3495(95)79975-1. [DOI] [PMC free article] [PubMed] [Google Scholar]
- Hill T. L. Theoretical formalism for the sliding filament model of contraction of striated muscle. Part I. Prog Biophys Mol Biol. 1974;28:267–340. doi: 10.1016/0079-6107(74)90020-0. [DOI] [PubMed] [Google Scholar]
- Homsher E. Muscle enthalpy production and its relationship to actomyosin ATPase. Annu Rev Physiol. 1987;49:673–690. doi: 10.1146/annurev.ph.49.030187.003325. [DOI] [PubMed] [Google Scholar]
- Huxley A. F., Simmons R. M. Proposed mechanism of force generation in striated muscle. Nature. 1971 Oct 22;233(5321):533–538. doi: 10.1038/233533a0. [DOI] [PubMed] [Google Scholar]
- Huxley A. F., Tideswell S. Filament compliance and tension transients in muscle. J Muscle Res Cell Motil. 1996 Aug;17(4):507–511. doi: 10.1007/BF00123366. [DOI] [PubMed] [Google Scholar]
- Huxley H. E., Stewart A., Sosa H., Irving T. X-ray diffraction measurements of the extensibility of actin and myosin filaments in contracting muscle. Biophys J. 1994 Dec;67(6):2411–2421. doi: 10.1016/S0006-3495(94)80728-3. [DOI] [PMC free article] [PubMed] [Google Scholar]
- Kojima H., Ishijima A., Yanagida T. Direct measurement of stiffness of single actin filaments with and without tropomyosin by in vitro nanomanipulation. Proc Natl Acad Sci U S A. 1994 Dec 20;91(26):12962–12966. doi: 10.1073/pnas.91.26.12962. [DOI] [PMC free article] [PubMed] [Google Scholar]
- Lombardi V., Piazzesi G., Linari M. Rapid regeneration of the actin-myosin power stroke in contracting muscle. Nature. 1992 Feb 13;355(6361):638–641. doi: 10.1038/355638a0. [DOI] [PubMed] [Google Scholar]
- Lombardi V., Piazzesi G. The contractile response during steady lengthening of stimulated frog muscle fibres. J Physiol. 1990 Dec;431:141–171. doi: 10.1113/jphysiol.1990.sp018324. [DOI] [PMC free article] [PubMed] [Google Scholar]
- Lou F., Sun Y. B. The high-force region of the force-velocity relation in frog skinned muscle fibres. Acta Physiol Scand. 1993 Jul;148(3):243–252. doi: 10.1111/j.1748-1716.1993.tb09555.x. [DOI] [PubMed] [Google Scholar]
- Piazzesi G., Francini F., Linari M., Lombardi V. Tension transients during steady lengthening of tetanized muscle fibres of the frog. J Physiol. 1992 Jan;445:659–711. doi: 10.1113/jphysiol.1992.sp018945. [DOI] [PMC free article] [PubMed] [Google Scholar]
- Piazzesi G., Lombardi V. A cross-bridge model that is able to explain mechanical and energetic properties of shortening muscle. Biophys J. 1995 May;68(5):1966–1979. doi: 10.1016/S0006-3495(95)80374-7. [DOI] [PMC free article] [PubMed] [Google Scholar]
- Sun Y. B., Lou F., Edman K. A. The effects of 2,3-butanedione monoxime (BDM) on the force-velocity relation in single muscle fibres of the frog. Acta Physiol Scand. 1995 Apr;153(4):325–334. doi: 10.1111/j.1748-1716.1995.tb09870.x. [DOI] [PubMed] [Google Scholar]
- Van Winkle W. B. Calcium release from skeletal muscle sarcoplasmic reticulum: site of action of dantrolene sodium. Science. 1976 Sep 17;193(4258):1130–1131. doi: 10.1126/science.959824. [DOI] [PubMed] [Google Scholar]
- Wakabayashi K., Sugimoto Y., Tanaka H., Ueno Y., Takezawa Y., Amemiya Y. X-ray diffraction evidence for the extensibility of actin and myosin filaments during muscle contraction. Biophys J. 1994 Dec;67(6):2422–2435. doi: 10.1016/S0006-3495(94)80729-5. [DOI] [PMC free article] [PubMed] [Google Scholar]
- Yanagida T., Arata T., Oosawa F. Sliding distance of actin filament induced by a myosin crossbridge during one ATP hydrolysis cycle. Nature. 1985 Jul 25;316(6026):366–369. doi: 10.1038/316366a0. [DOI] [PubMed] [Google Scholar]