Abstract
1. In newborn mammals, systemic hypoxia provokes catecholamine secretion from the adrenal medulla. In contrast to adults, this release is independent of sympathetic innervation. We have studied the cellular processes involved in hypoxia-induced catecholamine secretion, employing fluorimetric techniques to measure changes in [Ca2+]i, NADH and mitochondrial potential, and voltammetric techniques to record changes in PO2 and catecholamine secretion. 2. In adrenal chromaffin cells freshly dissociated from newborn rats, severe hypoxia increased [Ca2+]i and secretion of catecholamines, indicating that the response of the newborn adrenal medulla to hypoxia is an intrinsic property of these cells. Discrete quantal secretory events were identifiable, suggesting an exocytotic mechanism of secretion. 3. Hypoxia-induced secretion was only seen when PO2 fell below 5 mmHg, similar to the threshold arterial PO2 reported to stimulate release in vivo. Such oxygen tensions also inhibited mitochondrial metabolism, shown by an increase in NADH autofluorescence. We therefore explored the involvement of mitochondria in oxygen sensing. Inhibition of mitochondrial respiration either by CN- at complex IV or by rotenone at complex I mimicked severe hypoxia, reversibly increasing both [Ca2+]i and catecholamine secretion. The CN(-)-induced depolarization of the mitochondrial inner membrane potential preceded the increase in [Ca2+]i by approximately 6 s. 4. The effects of severe hypoxia and CN- on [Ca2+]i and catecholamine secretion were not additive, suggesting a common mechanism. 5. Chemical anoxia failed to increase [Ca2+]i in a significant proportion of cells dissociated from 2- to 4-week-old rats. Thus, the sensitivity to hypoxia is specific to adrenal chromaffin cells dissociated from newborn rats. 6. These data indicate that hypoxia-induced catecholamine secretion in the newborn adrenal medulla is mediated by reversible inhibition of mitochondrial respiration, leading to an increase in [Ca2+]i and catecholamine secretion.
Full text
PDF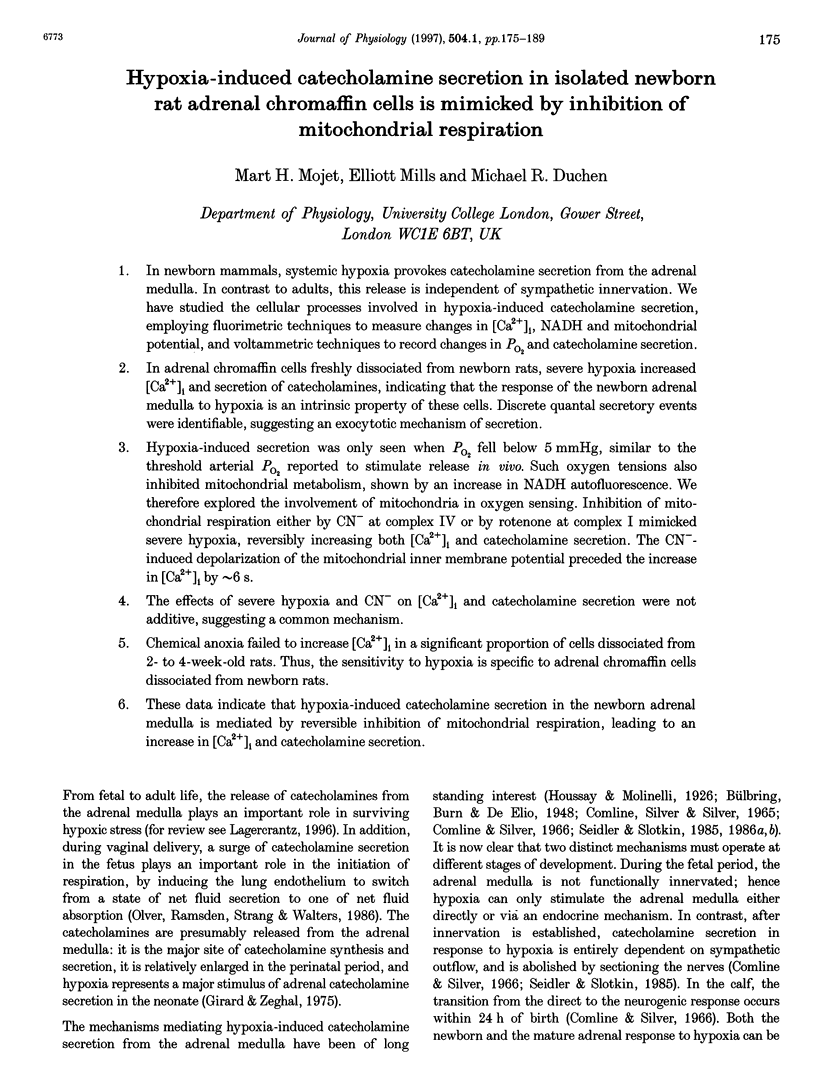
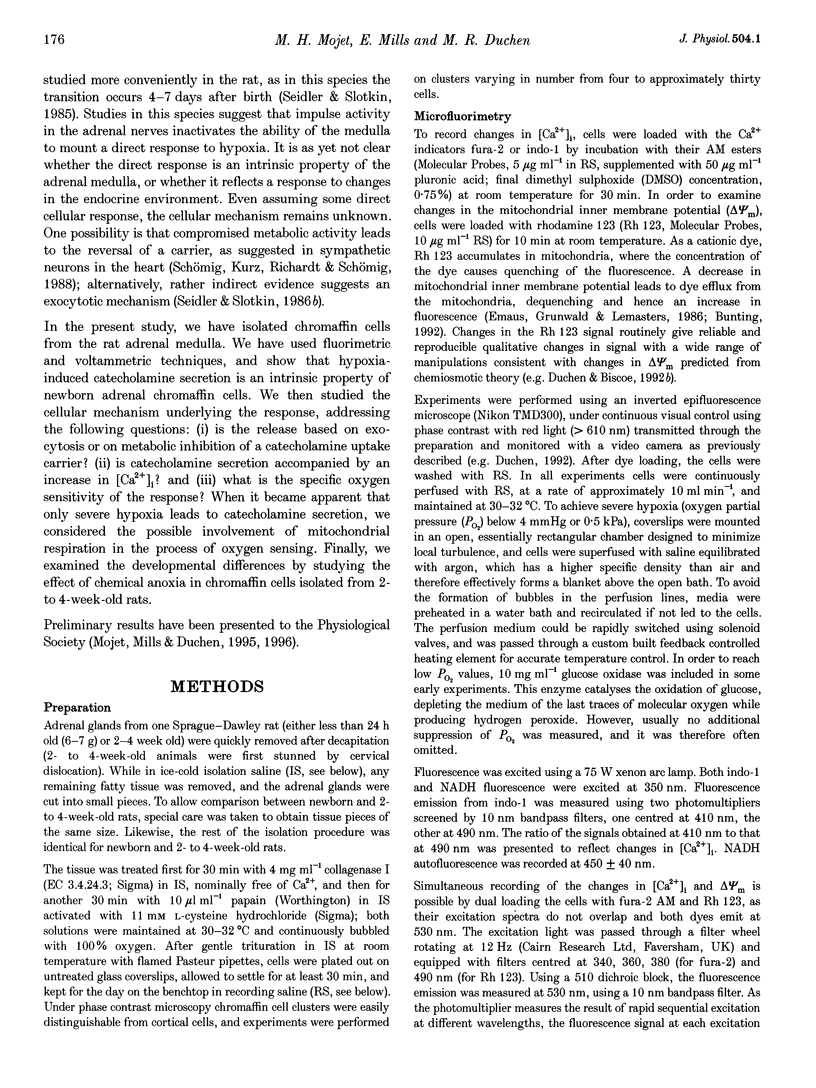
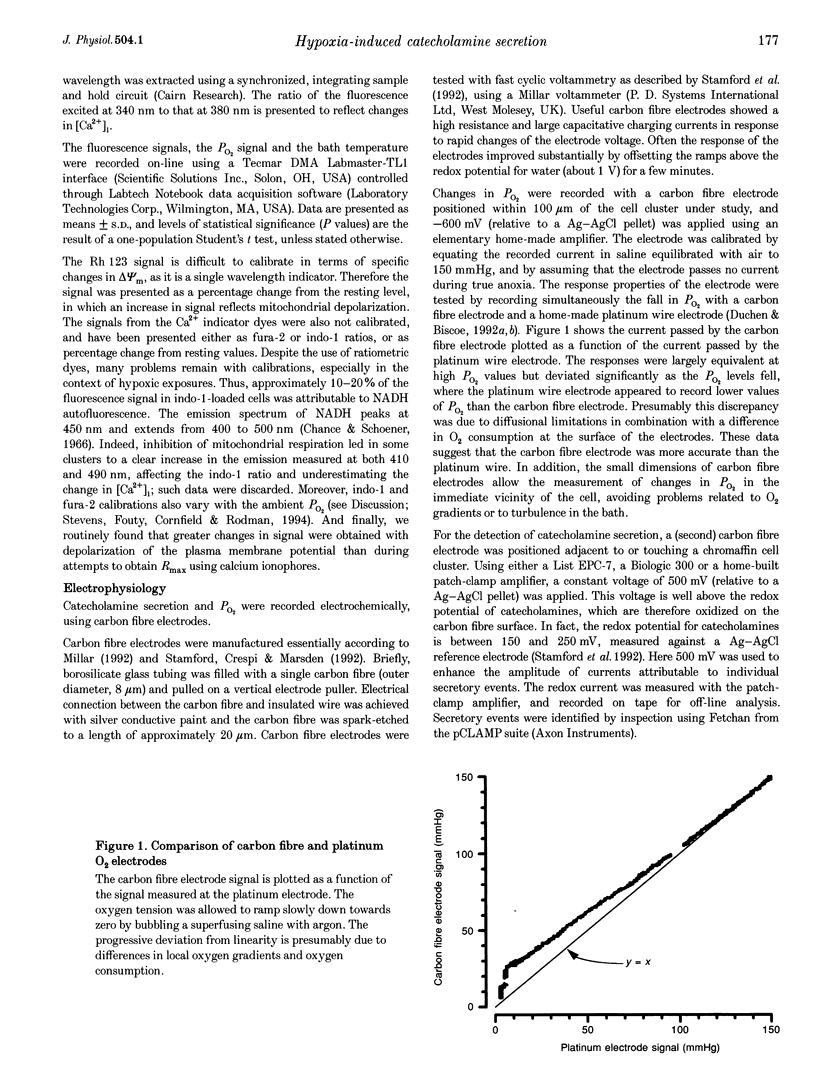
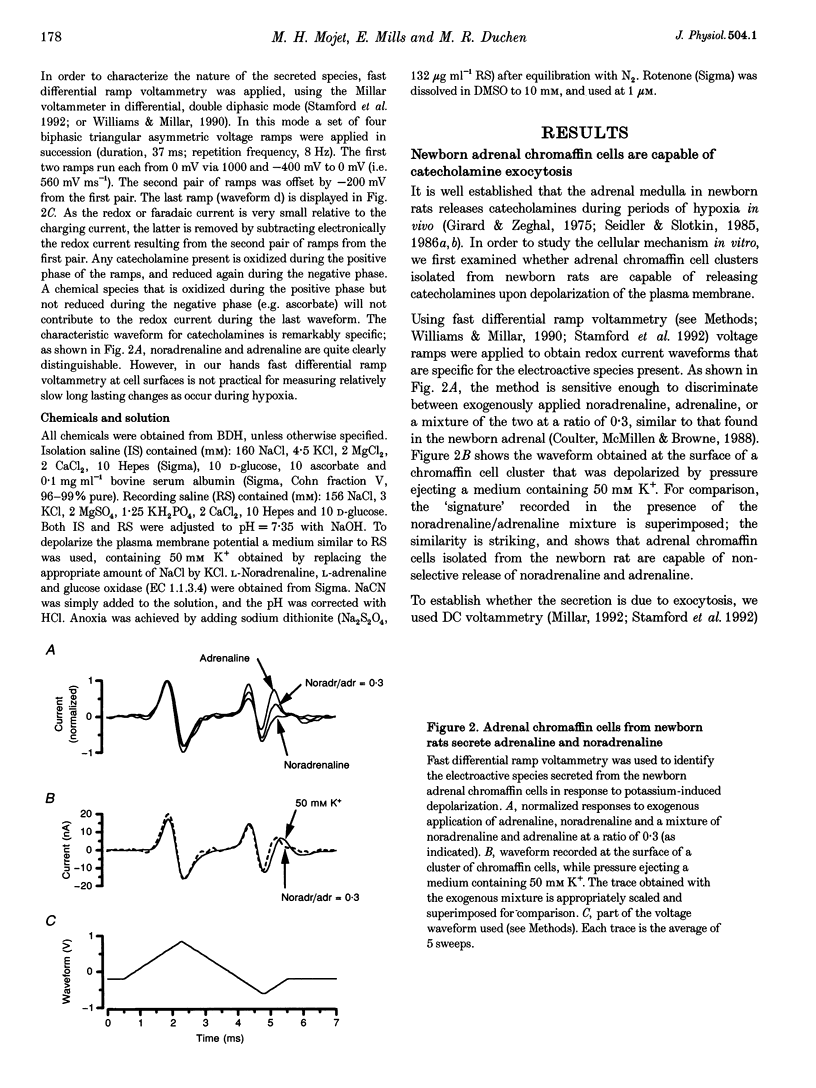
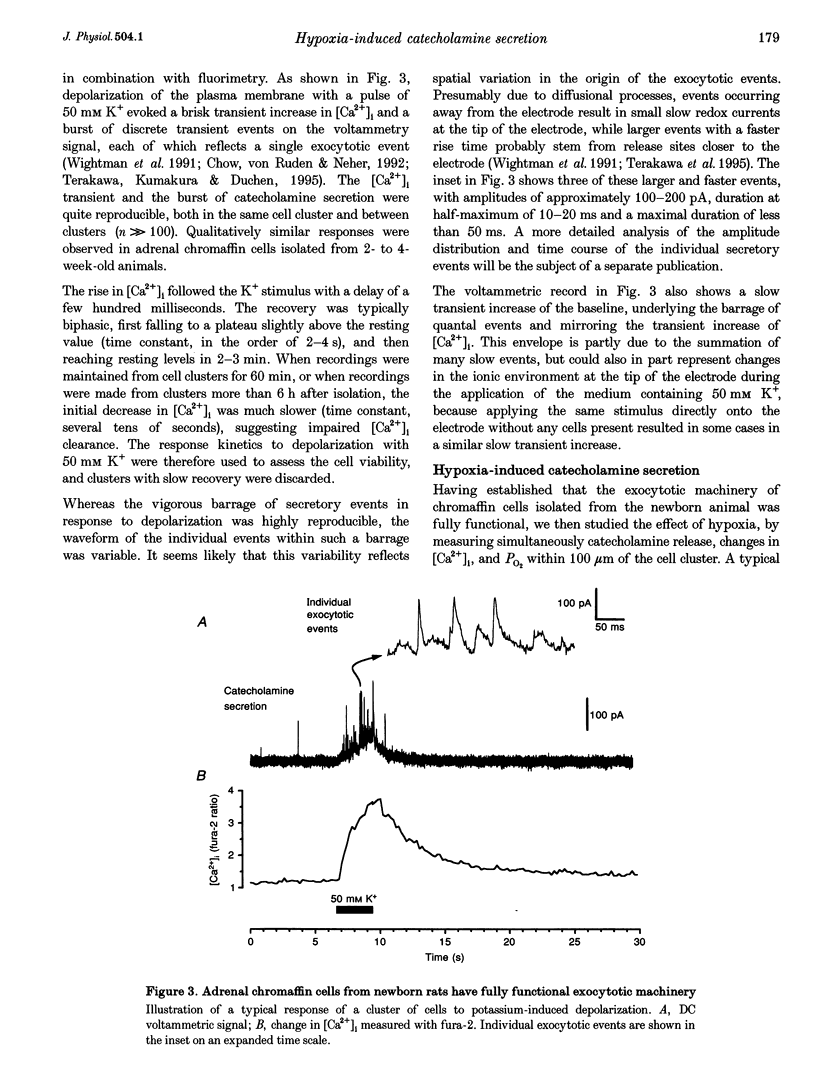
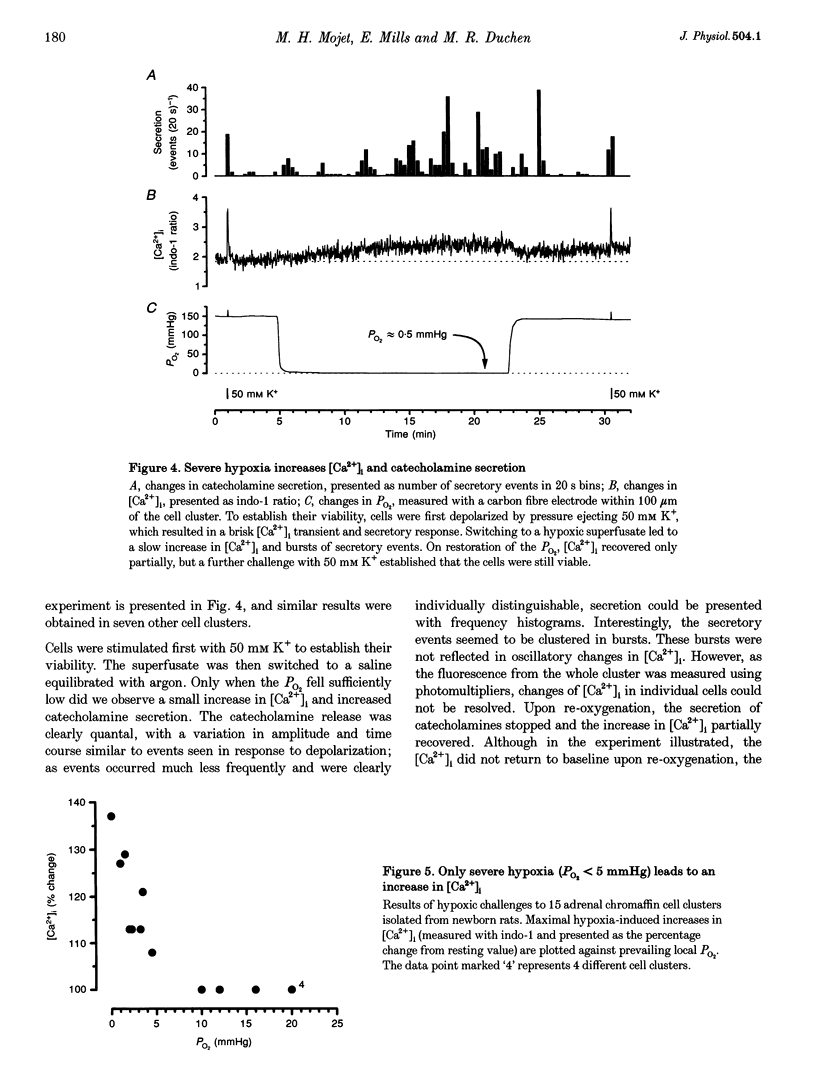
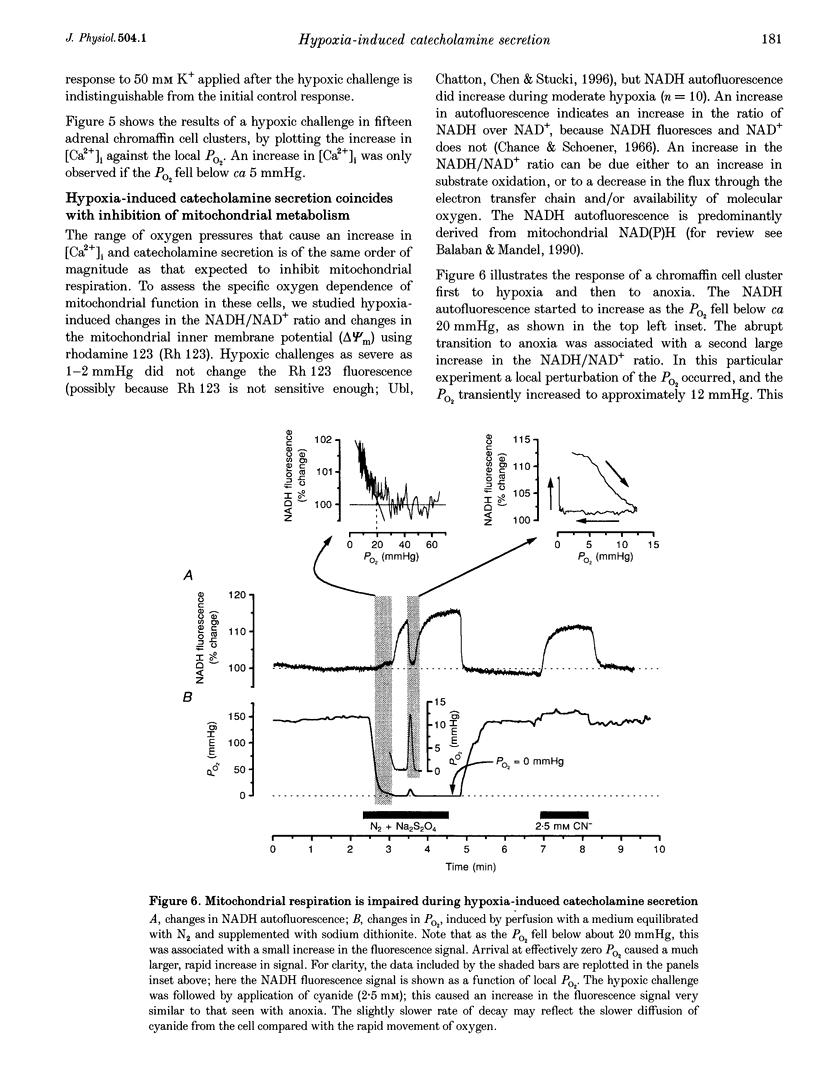
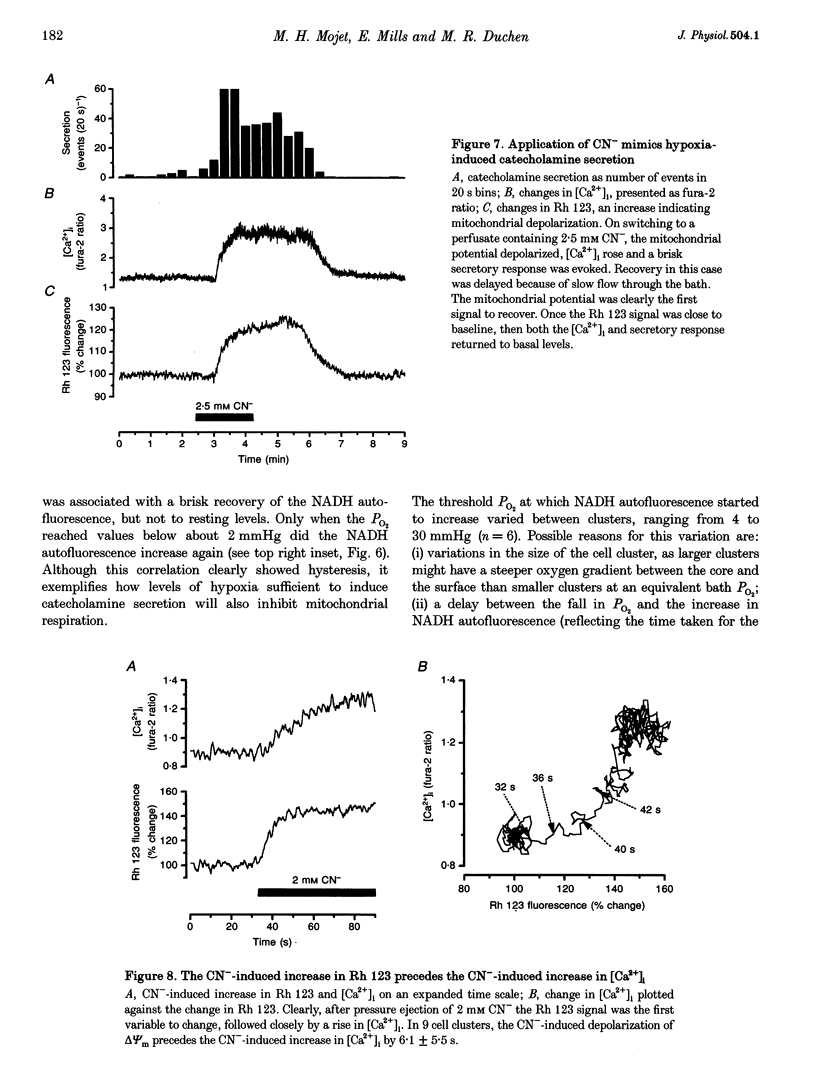
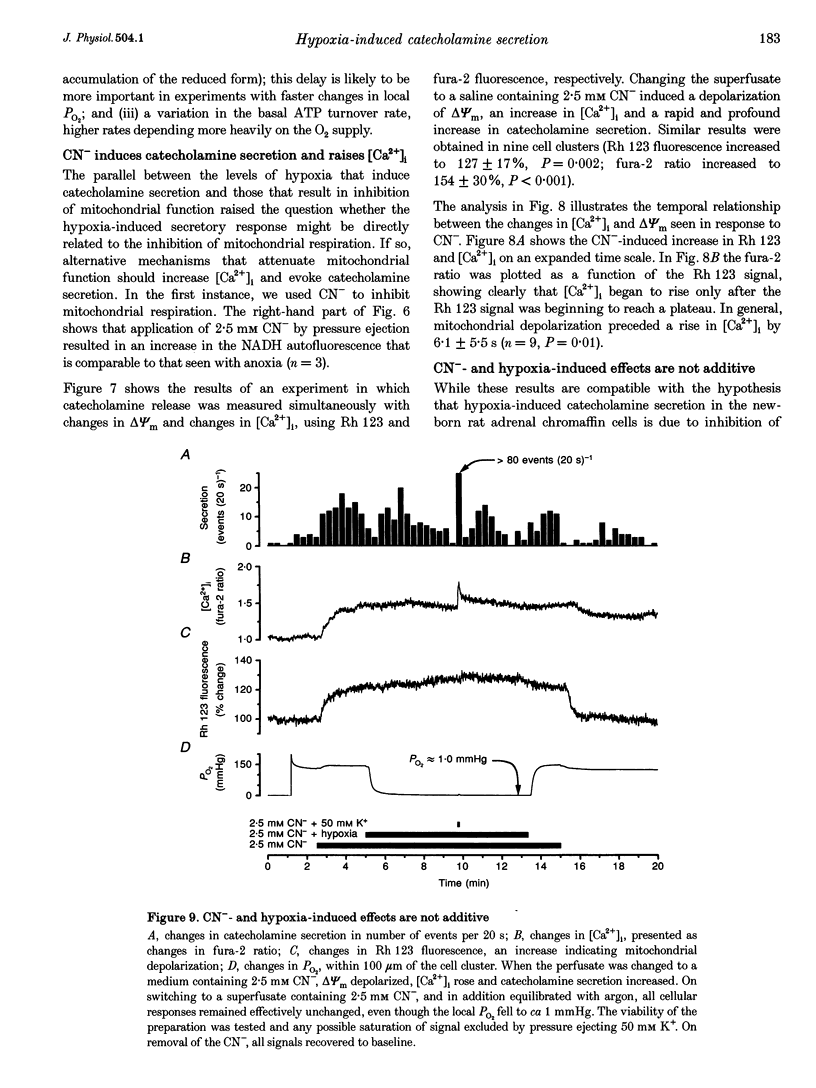
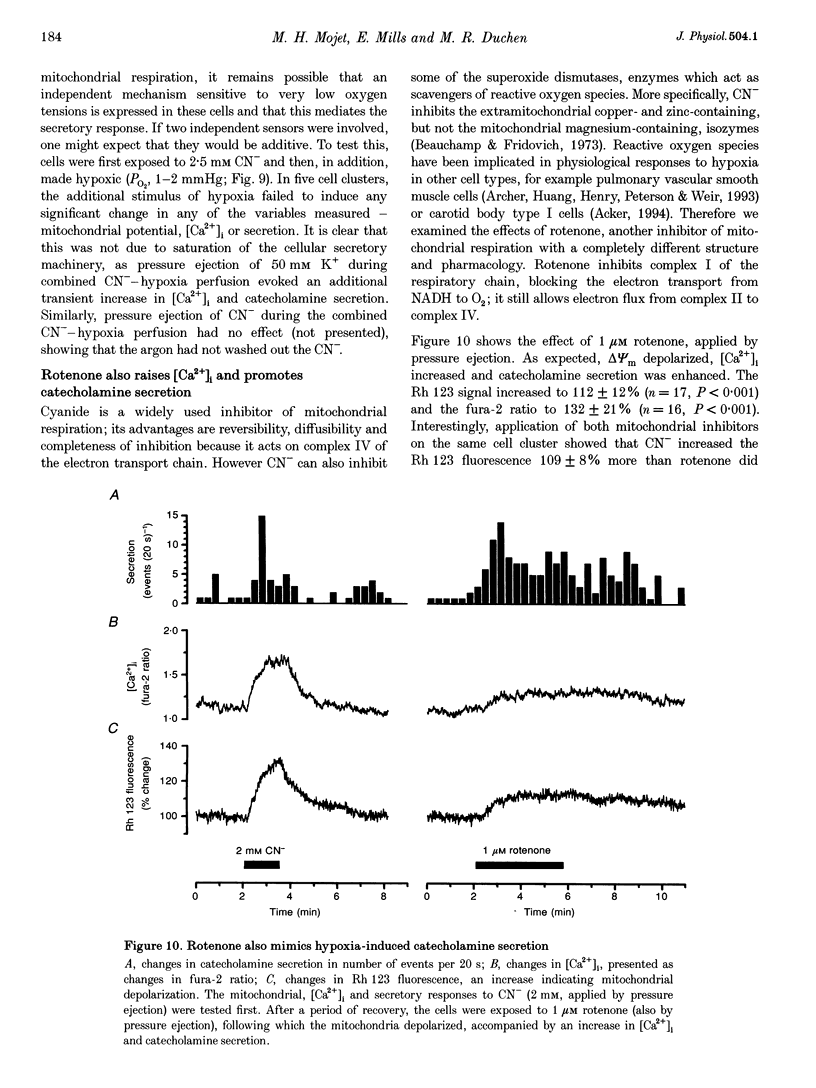
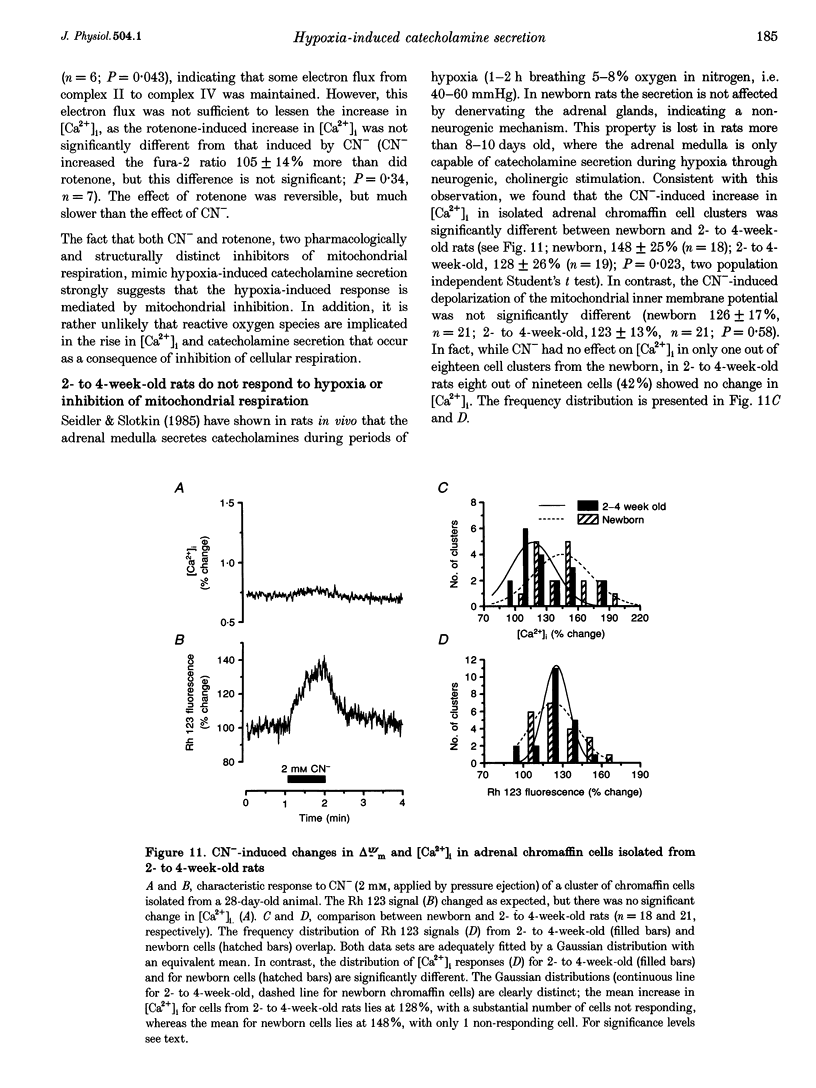
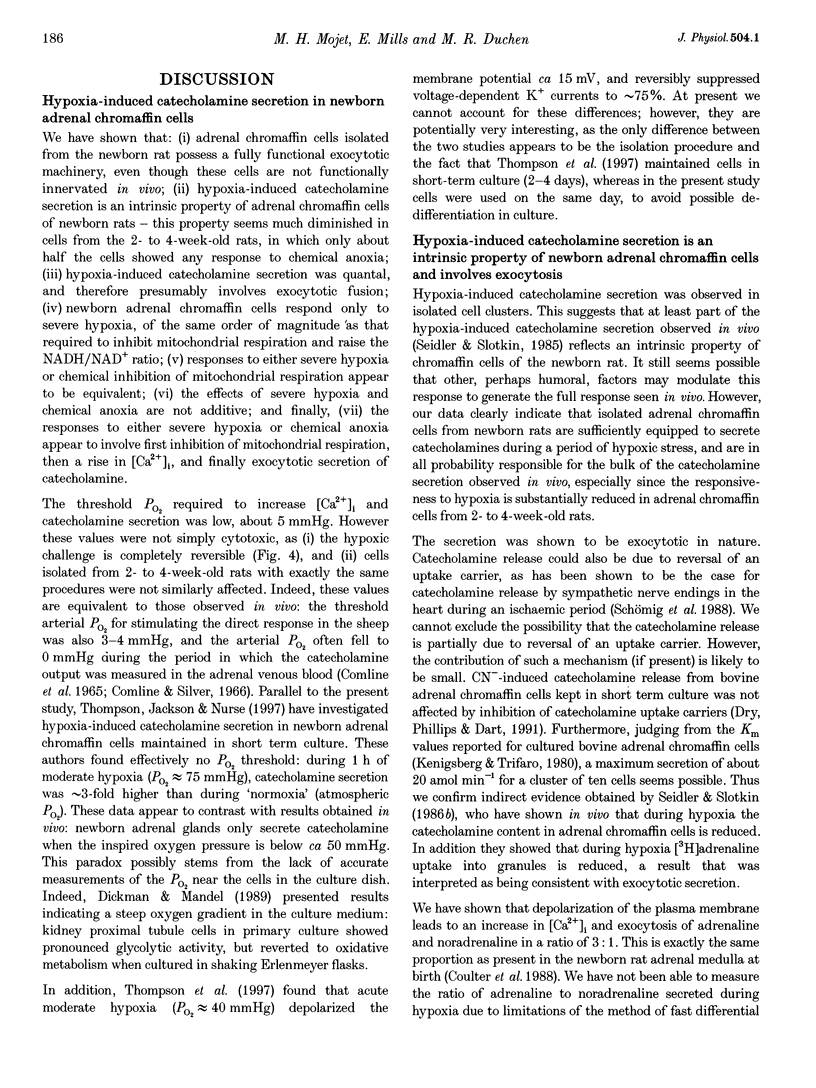
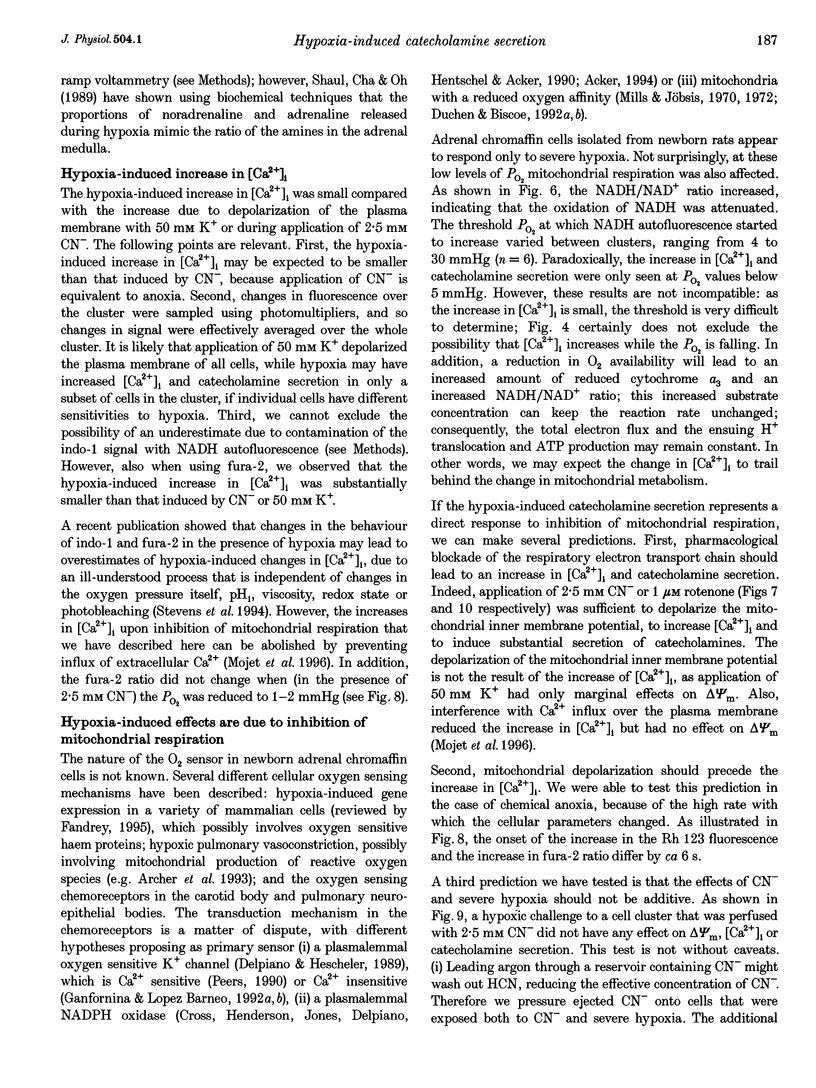
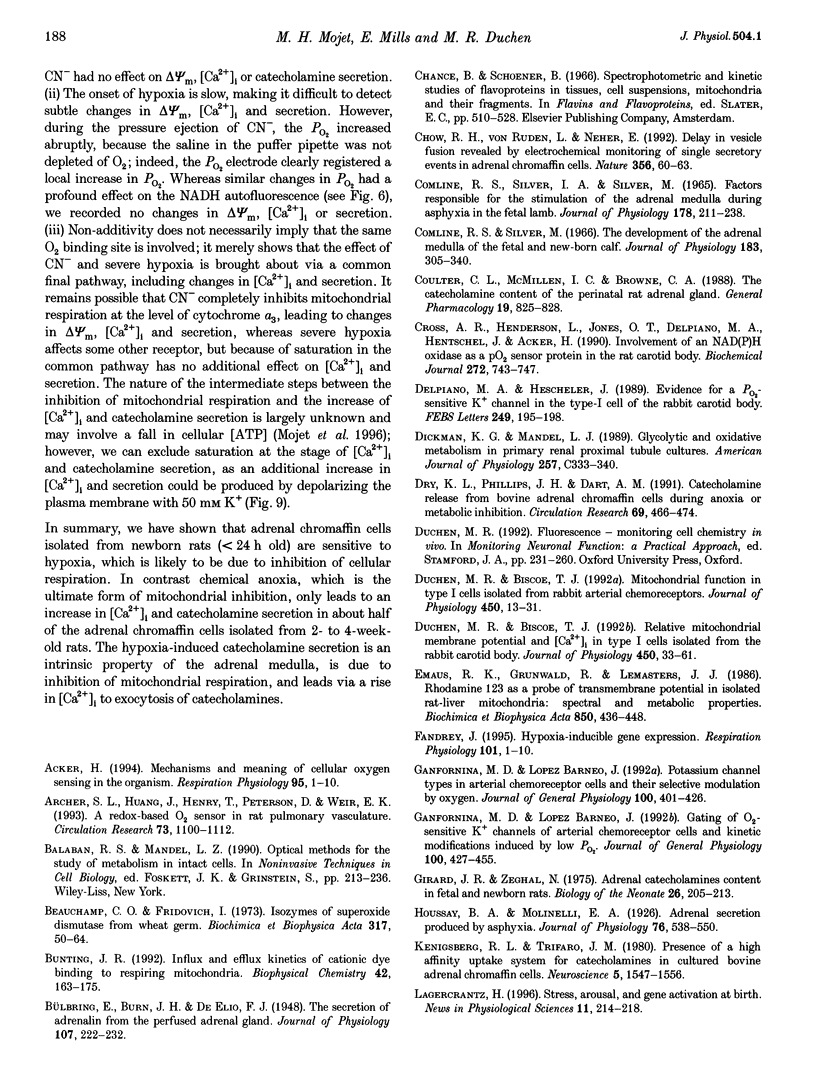
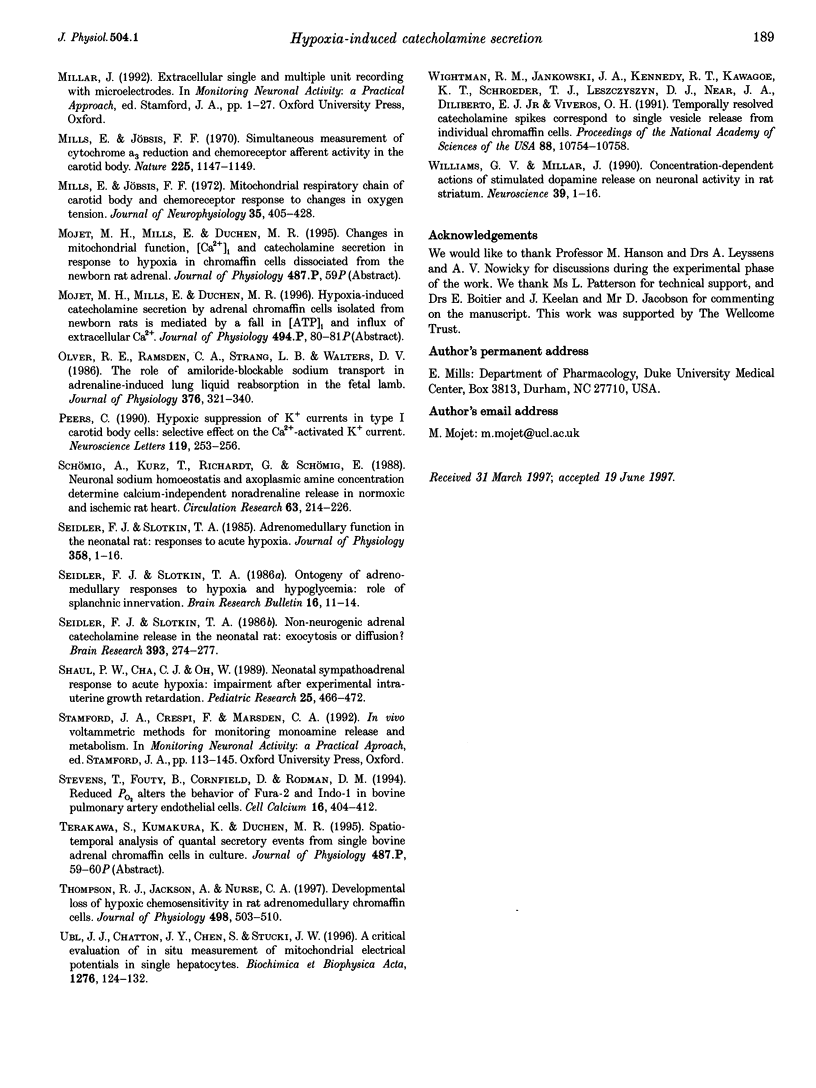
Images in this article
Selected References
These references are in PubMed. This may not be the complete list of references from this article.
- Acker H. Mechanisms and meaning of cellular oxygen sensing in the organism. Respir Physiol. 1994 Jan;95(1):1–10. doi: 10.1016/0034-5687(94)90043-4. [DOI] [PubMed] [Google Scholar]
- Beauchamp C. O., Fridovich I. Isozymes of superoxide dismutase from wheat germ. Biochim Biophys Acta. 1973 Jul 12;317(1):50–64. doi: 10.1016/0005-2795(73)90198-0. [DOI] [PubMed] [Google Scholar]
- Bunting J. R. Influx and efflux kinetics of cationic dye binding to respiring mitochondria. Biophys Chem. 1992 Feb;42(2):163–175. doi: 10.1016/0301-4622(92)85006-p. [DOI] [PubMed] [Google Scholar]
- Bülbring E., Burn J. H., De Elio F. J. The secretion of adrenaline from the perfused suprarenal gland. J Physiol. 1948 Mar 15;107(2):222–232. doi: 10.1113/jphysiol.1948.sp004265. [DOI] [PMC free article] [PubMed] [Google Scholar]
- COMLINE R. S., SILVER I. A., SILVER M. FACTORS RESPONSIBLE FOR THE STIMULATION OF THE ADRENAL MEDULLA DURING ASPHYXIA IN THE FOETAL LAMB. J Physiol. 1965 May;178:211–238. doi: 10.1113/jphysiol.1965.sp007624. [DOI] [PMC free article] [PubMed] [Google Scholar]
- Chow R. H., von Rüden L., Neher E. Delay in vesicle fusion revealed by electrochemical monitoring of single secretory events in adrenal chromaffin cells. Nature. 1992 Mar 5;356(6364):60–63. doi: 10.1038/356060a0. [DOI] [PubMed] [Google Scholar]
- Comline R. S., Silver M. The development of the adrenal medulla of the foetal and new-born calf. J Physiol. 1966 Mar;183(2):305–340. doi: 10.1113/jphysiol.1966.sp007868. [DOI] [PMC free article] [PubMed] [Google Scholar]
- Coulter C. L., McMillen I. C., Browne C. A. The catecholamine content of the perinatal rat adrenal gland. Gen Pharmacol. 1988;19(6):825–828. [PubMed] [Google Scholar]
- Cross A. R., Henderson L., Jones O. T., Delpiano M. A., Hentschel J., Acker H. Involvement of an NAD(P)H oxidase as a pO2 sensor protein in the rat carotid body. Biochem J. 1990 Dec 15;272(3):743–747. doi: 10.1042/bj2720743. [DOI] [PMC free article] [PubMed] [Google Scholar]
- Dickman K. G., Mandel L. J. Glycolytic and oxidative metabolism in primary renal proximal tubule cultures. Am J Physiol. 1989 Aug;257(2 Pt 1):C333–C340. doi: 10.1152/ajpcell.1989.257.2.C333. [DOI] [PubMed] [Google Scholar]
- Dry K. L., Phillips J. H., Dart A. M. Catecholamine release from bovine adrenal chromaffin cells during anoxia or metabolic inhibition. Circ Res. 1991 Aug;69(2):466–474. doi: 10.1161/01.res.69.2.466. [DOI] [PubMed] [Google Scholar]
- Duchen M. R., Biscoe T. J. Mitochondrial function in type I cells isolated from rabbit arterial chemoreceptors. J Physiol. 1992 May;450:13–31. doi: 10.1113/jphysiol.1992.sp019114. [DOI] [PMC free article] [PubMed] [Google Scholar]
- Duchen M. R., Biscoe T. J. Relative mitochondrial membrane potential and [Ca2+]i in type I cells isolated from the rabbit carotid body. J Physiol. 1992 May;450:33–61. doi: 10.1113/jphysiol.1992.sp019115. [DOI] [PMC free article] [PubMed] [Google Scholar]
- Emaus R. K., Grunwald R., Lemasters J. J. Rhodamine 123 as a probe of transmembrane potential in isolated rat-liver mitochondria: spectral and metabolic properties. Biochim Biophys Acta. 1986 Jul 23;850(3):436–448. doi: 10.1016/0005-2728(86)90112-x. [DOI] [PubMed] [Google Scholar]
- Fandrey J. Hypoxia-inducible gene expression. Respir Physiol. 1995 Jul;101(1):1–10. doi: 10.1016/0034-5687(95)00013-4. [DOI] [PubMed] [Google Scholar]
- Ganfornina M. D., López-Barneo J. Gating of O2-sensitive K+ channels of arterial chemoreceptor cells and kinetic modifications induced by low PO2. J Gen Physiol. 1992 Sep;100(3):427–455. doi: 10.1085/jgp.100.3.427. [DOI] [PMC free article] [PubMed] [Google Scholar]
- Ganfornina M. D., López-Barneo J. Potassium channel types in arterial chemoreceptor cells and their selective modulation by oxygen. J Gen Physiol. 1992 Sep;100(3):401–426. doi: 10.1085/jgp.100.3.401. [DOI] [PMC free article] [PubMed] [Google Scholar]
- Girard J. R., Zeghal N. Adrenal catecholamines content in fetal and newborn rats. Biol Neonate. 1975;26(3-4):205–213. doi: 10.1159/000240731. [DOI] [PubMed] [Google Scholar]
- Kenigsberg R. L., Trifaró J. M. Presence of a high affinity uptake system for catecholamines in cultured bovine adrenal chromaffin cells. Neuroscience. 1980;5(9):1547–1556. doi: 10.1016/0306-4522(80)90019-6. [DOI] [PubMed] [Google Scholar]
- Mills E., Jöbsis F. F. Mitochondrial respiratory chain of carotid body and chemoreceptor response to changes in oxygen tension. J Neurophysiol. 1972 Jul;35(4):405–428. doi: 10.1152/jn.1972.35.4.405. [DOI] [PubMed] [Google Scholar]
- Mills E., Jöbsis F. F. Simultaneous measurement of cytochrome a3 reduction and chemoreceptor afferent activity in the carotid body. Nature. 1970 Mar 21;225(5238):1147–1149. doi: 10.1038/2251147a0. [DOI] [PubMed] [Google Scholar]
- Olver R. E., Ramsden C. A., Strang L. B., Walters D. V. The role of amiloride-blockable sodium transport in adrenaline-induced lung liquid reabsorption in the fetal lamb. J Physiol. 1986 Jul;376:321–340. doi: 10.1113/jphysiol.1986.sp016156. [DOI] [PMC free article] [PubMed] [Google Scholar]
- Peers C. Hypoxic suppression of K+ currents in type I carotid body cells: selective effect on the Ca2(+)-activated K+ current. Neurosci Lett. 1990 Nov 13;119(2):253–256. doi: 10.1016/0304-3940(90)90846-2. [DOI] [PubMed] [Google Scholar]
- Schömig A., Kurz T., Richardt G., Schömig E. Neuronal sodium homoeostatis and axoplasmic amine concentration determine calcium-independent noradrenaline release in normoxic and ischemic rat heart. Circ Res. 1988 Jul;63(1):214–226. doi: 10.1161/01.res.63.1.214. [DOI] [PubMed] [Google Scholar]
- Seidler F. J., Slotkin T. A. Adrenomedullary function in the neonatal rat: responses to acute hypoxia. J Physiol. 1985 Jan;358:1–16. doi: 10.1113/jphysiol.1985.sp015536. [DOI] [PMC free article] [PubMed] [Google Scholar]
- Seidler F. J., Slotkin T. A. Non-neurogenic adrenal catecholamine release in the neonatal rat: exocytosis or diffusion? Brain Res. 1986 Aug;393(2):274–277. doi: 10.1016/0165-3806(86)90031-3. [DOI] [PubMed] [Google Scholar]
- Seidler F. J., Slotkin T. A. Ontogeny of adrenomedullary responses to hypoxia and hypoglycemia: role of splanchnic innervation. Brain Res Bull. 1986 Jan;16(1):11–14. doi: 10.1016/0361-9230(86)90005-5. [DOI] [PubMed] [Google Scholar]
- Shaul P. W., Cha C. J., Oh W. Neonatal sympathoadrenal response to acute hypoxia: impairment after experimental intrauterine growth retardation. Pediatr Res. 1989 May;25(5):466–472. doi: 10.1203/00006450-198905000-00008. [DOI] [PubMed] [Google Scholar]
- Stevens T., Fouty B., Cornfield D., Rodman D. M. Reduced PO2 alters the behavior of Fura-2 and Indo-1 in bovine pulmonary artery endothelial cells. Cell Calcium. 1994 Nov;16(5):404–412. doi: 10.1016/0143-4160(94)90033-7. [DOI] [PubMed] [Google Scholar]
- Thompson R. J., Jackson A., Nurse C. A. Developmental loss of hypoxic chemosensitivity in rat adrenomedullary chromaffin cells. J Physiol. 1997 Jan 15;498(Pt 2):503–510. doi: 10.1113/jphysiol.1997.sp021876. [DOI] [PMC free article] [PubMed] [Google Scholar]
- Ubl J. J., Chatton J. Y., Chen S., Stucki J. W. A critical evaluation of in situ measurement of mitochondrial electrical potentials in single hepatocytes. Biochim Biophys Acta. 1996 Sep 12;1276(2):124–132. doi: 10.1016/0005-2728(96)00067-9. [DOI] [PubMed] [Google Scholar]
- Wightman R. M., Jankowski J. A., Kennedy R. T., Kawagoe K. T., Schroeder T. J., Leszczyszyn D. J., Near J. A., Diliberto E. J., Jr, Viveros O. H. Temporally resolved catecholamine spikes correspond to single vesicle release from individual chromaffin cells. Proc Natl Acad Sci U S A. 1991 Dec 1;88(23):10754–10758. doi: 10.1073/pnas.88.23.10754. [DOI] [PMC free article] [PubMed] [Google Scholar]
- Williams G. V., Millar J. Concentration-dependent actions of stimulated dopamine release on neuronal activity in rat striatum. Neuroscience. 1990;39(1):1–16. doi: 10.1016/0306-4522(90)90217-r. [DOI] [PubMed] [Google Scholar]