Abstract
1. Initiation and propagation of action potentials evoked by extracellular synaptic stimulation was studied using simultaneous dual and triple patch pipette recordings from different locations on neocortical layer 5 pyramidal neurons in brain slices from 4-week-old rats (P26-30) at physiological temperatures. 2. Simultaneous cell-attached and whole-cell voltage recordings from the apical trunk (up to 700 microns distal to the soma) and the soma indicated that proximal synaptic stimulation (layer 4) initiated action potentials first at the soma, whereas distal stimulation (upper layer 2/3) could initiate dendritic regenerative potentials prior to somatic action potentials following stimulation at higher intensity. 3. Somatic action potentials, once initiated, propagated back into the apical dendrites in a decremented manner which was frequency dependent. The half-width of back propagating action potentials increased and their maximum rate of rise decreased with distance from the soma, with the peak of these action potentials propagating with a conduction velocity of approximately 0.5 m s-1. 4. Back-propagation of action potentials into the dendritic tree was associated with dendritic calcium electrogenesis, which was particularly prominent during bursts of somatic action potentials. 5. When dendritic regenerative potentials were evoked prior to somatic action potentials, the more distal the dendritic recording was made from the soma the longer the time between the onset of the dendritic regenerative potential relative to somatic action potential. This suggested that dendritic regenerative potentials were initiated in the distal apical dendrites, possibly in the apical tuft. 6. At any one stimulus intensity, the initiation of dendritic regenerative potentials prior to somatic action potentials could fluctuate, and was modulated by depolarizing somatic or hyperpolarizing dendritic current injection. 7. Dendritic regenerative potentials could be initiated prior to somatic action potentials by dendritic current injections used to simulate the membrane voltage change that occurs during an EPSP. Initiation of these dendritic potentials was not affected by cadmium (200 microM), but was blocked by TTX (1 microM). 8. Dendritic regenerative potentials in some experiments were initiated in isolated from somatic action potentials. The voltage change at the soma in response to these dendritic regenerative events was small and subthreshold, showing that dendritic regenerative events are strongly attenuated as they spread to the soma. 9. Simultaneous whole-cell recordings from the axon initial segment and the soma indicated that synaptic stimulation always initiated action potentials first in the axon. The further the axonal recording was made from the soma the greater the time delay between axonal and somatic action potentials, indicating a site of action potential initiation in the axon at least 30 microns distal to the soma. 10. Simultaneous whole-cell recordings from the apical dendrite, soma and axon initial segment showed that action potentials were always initiated in the axon prior to the soma, and with the same latency difference, independent of whether dendritic regenerative potentials were initiated or not. 11. It is concluded that both the apical dendrites and the axon of neocortical layer 5 pyramidal neurons in P26-30 animals are capable of initiating regenerative potentials. Regenerative potentials initiated in dendrites, however, are significantly attenuated as they spread to the soma and axon. As a consequence, action potentials are always initiated in the axon before the soma, even when synaptic activation is intense enough to initiate dendritic regenerative potentials. Once initiated, the axonal action potentials are conducted orthogradely into the axonal arbor and retrogradely into the dendritic tree.
Full text
PDF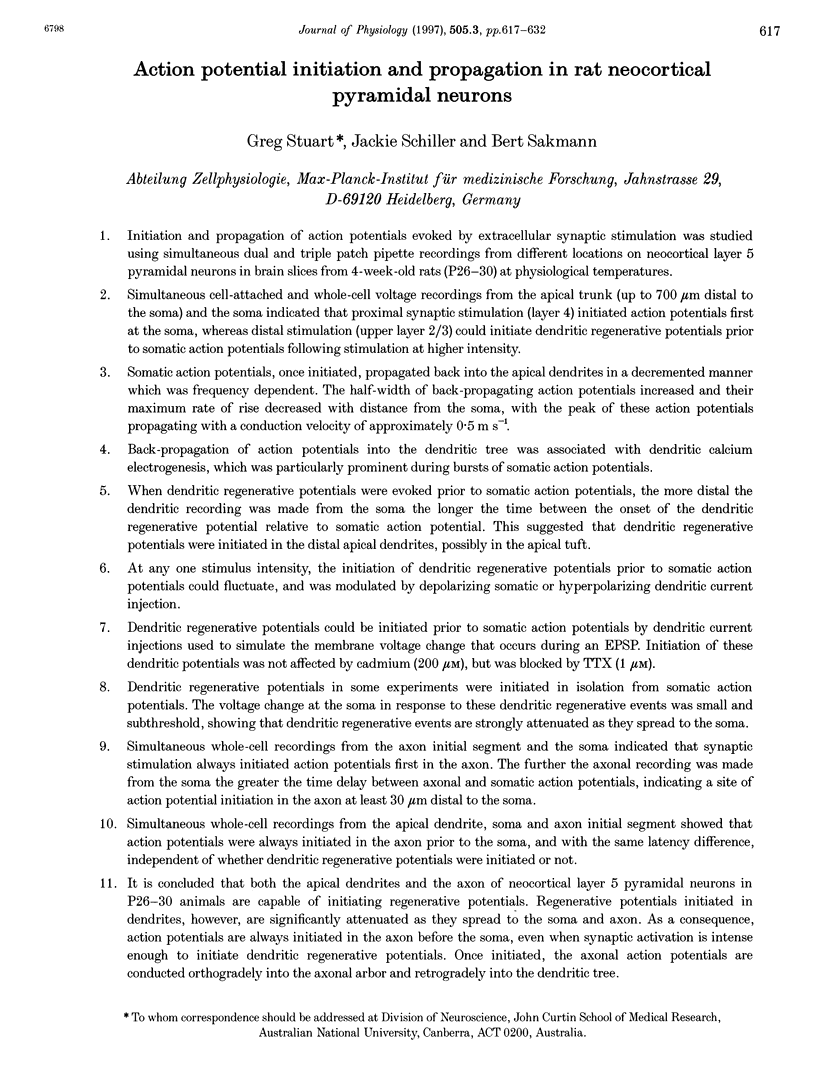
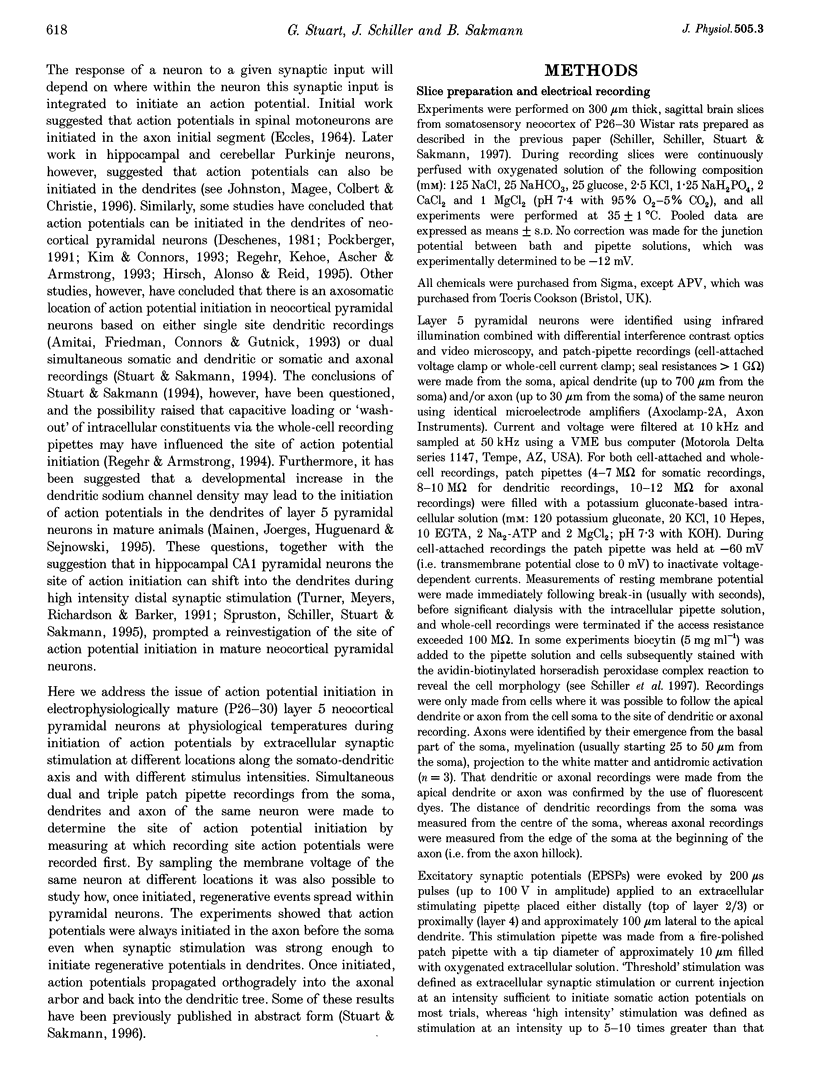
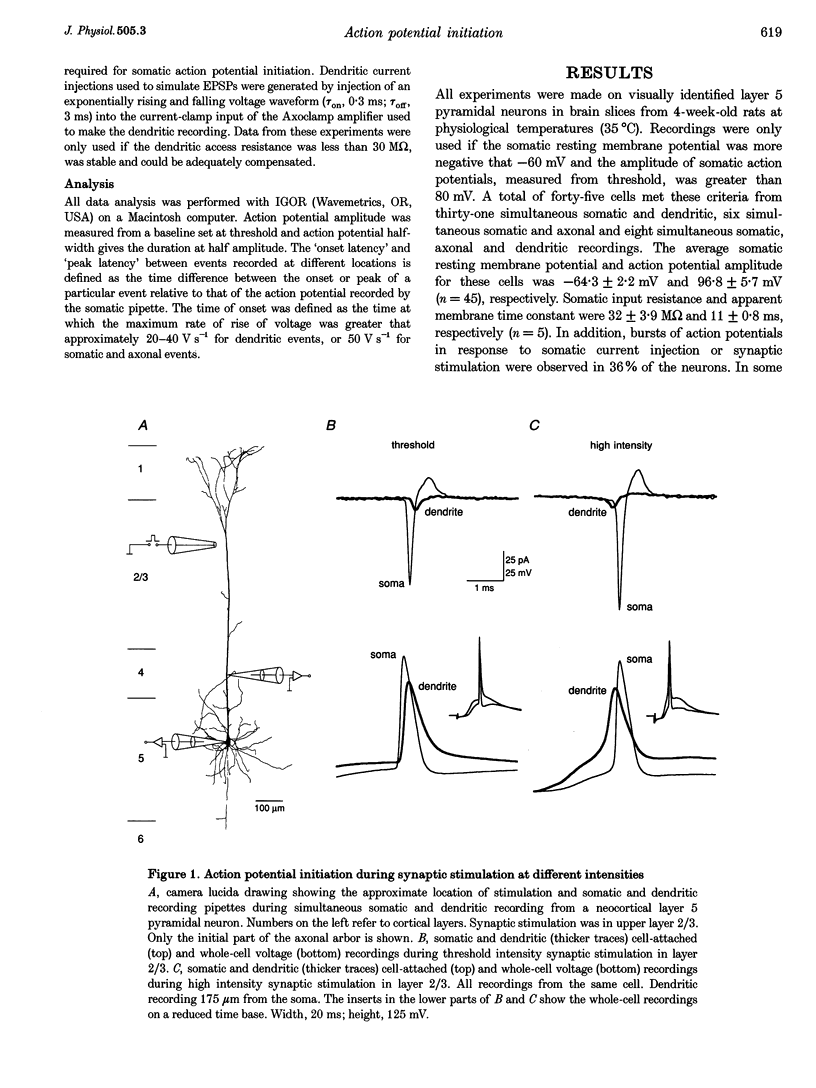
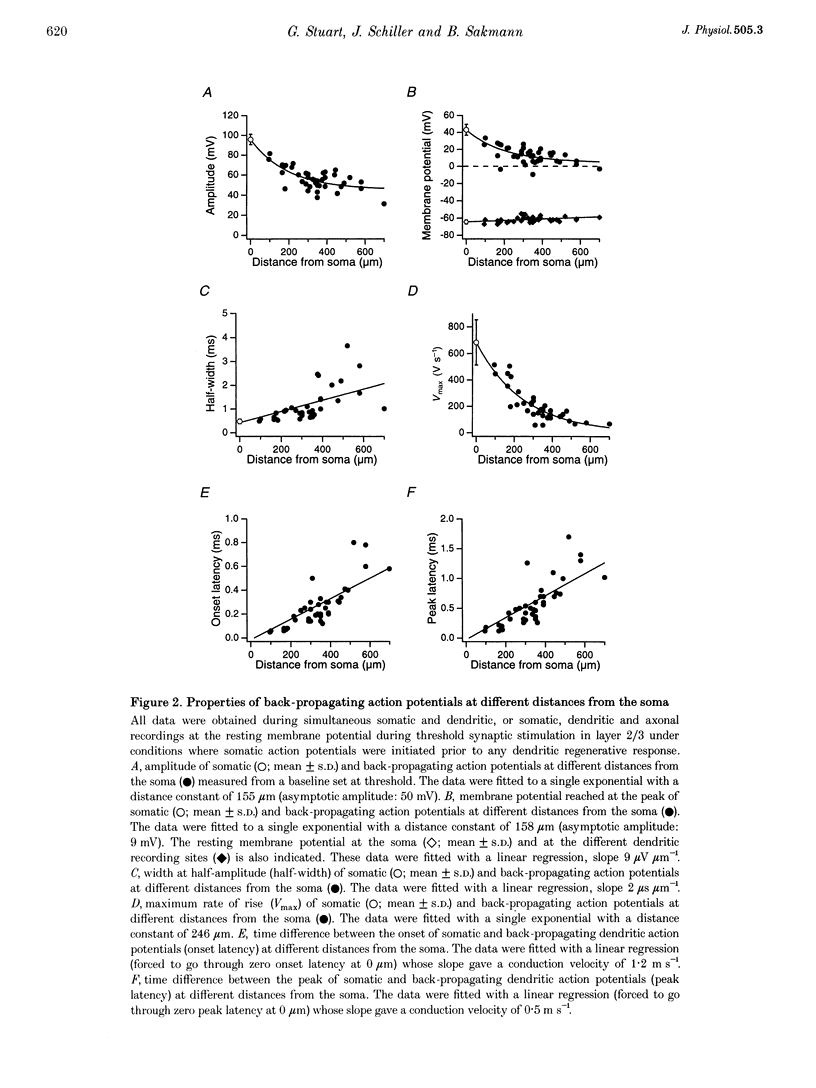
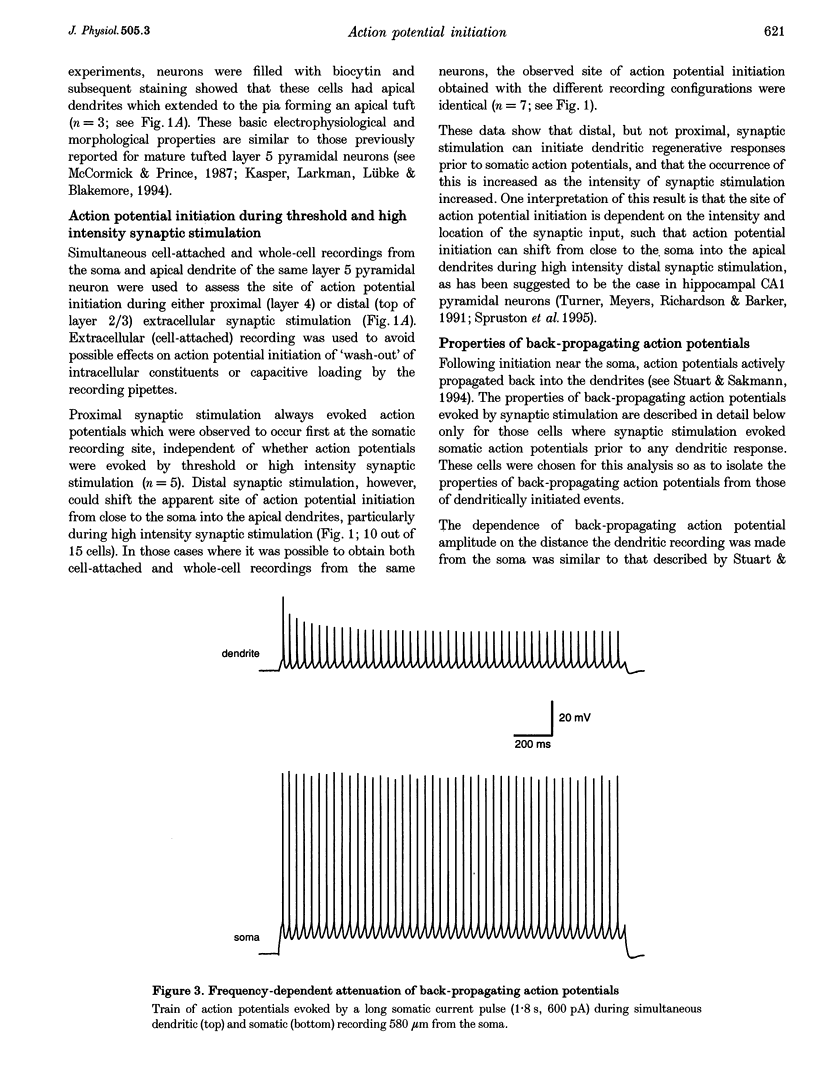
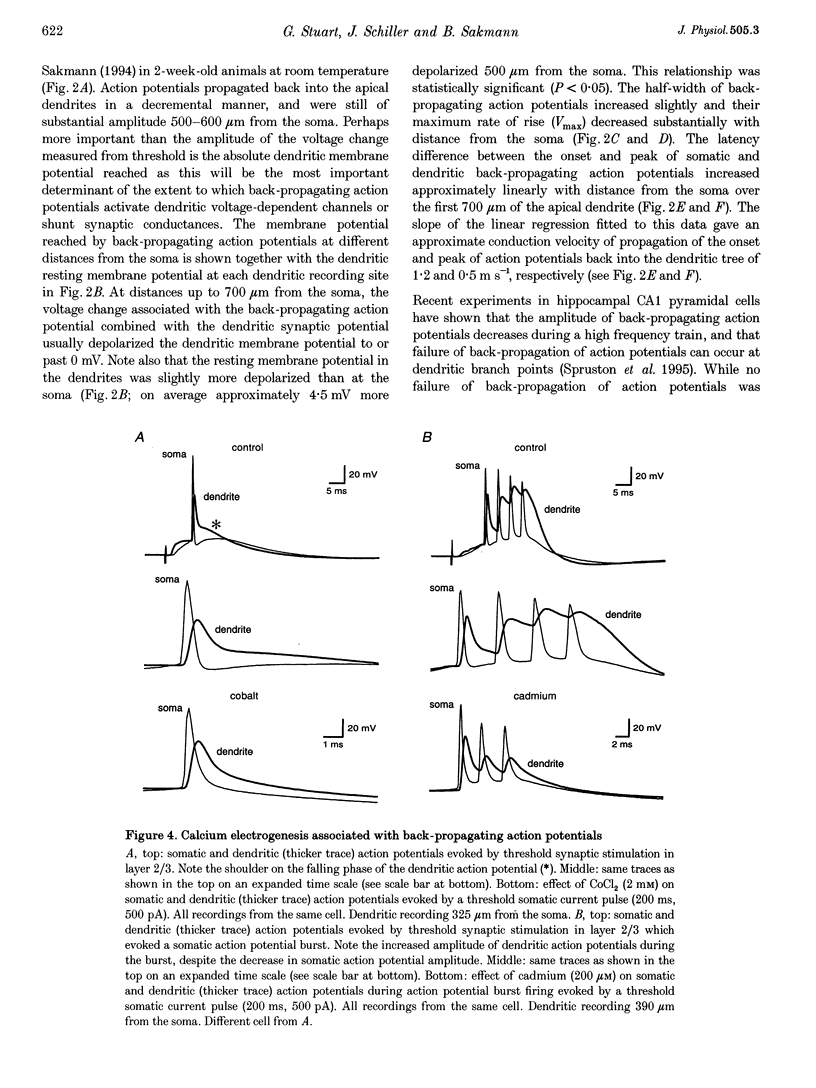
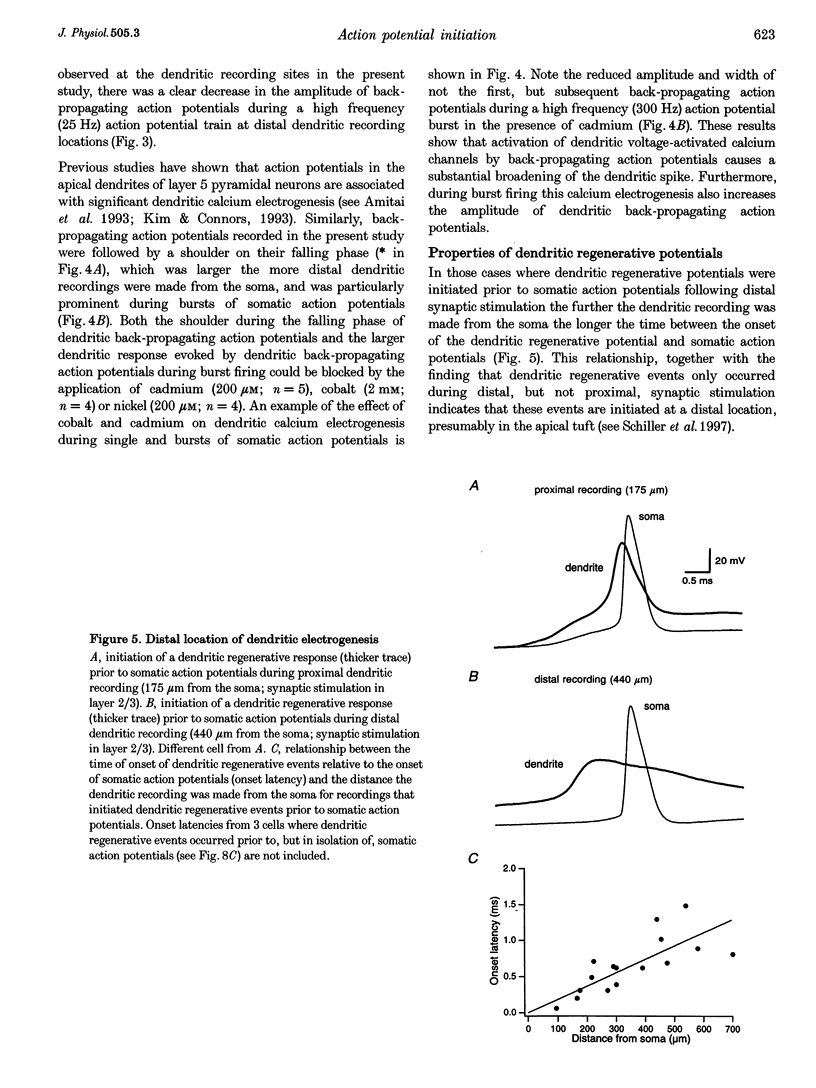
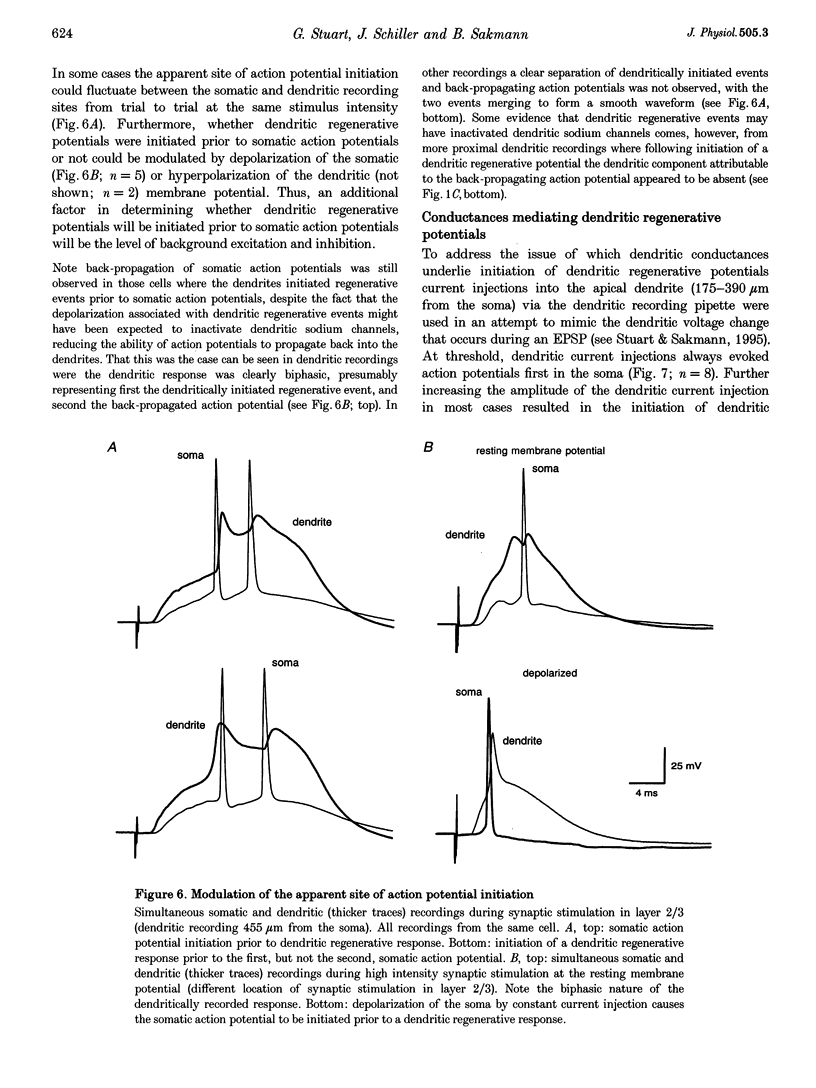
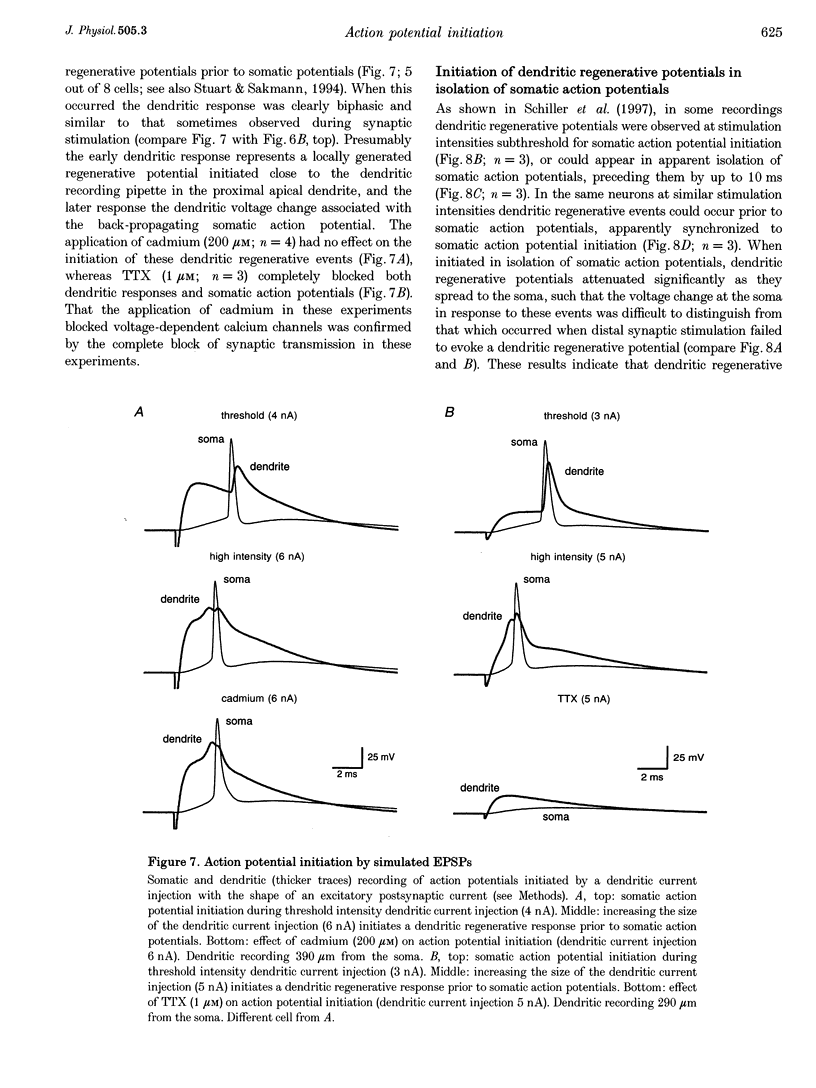
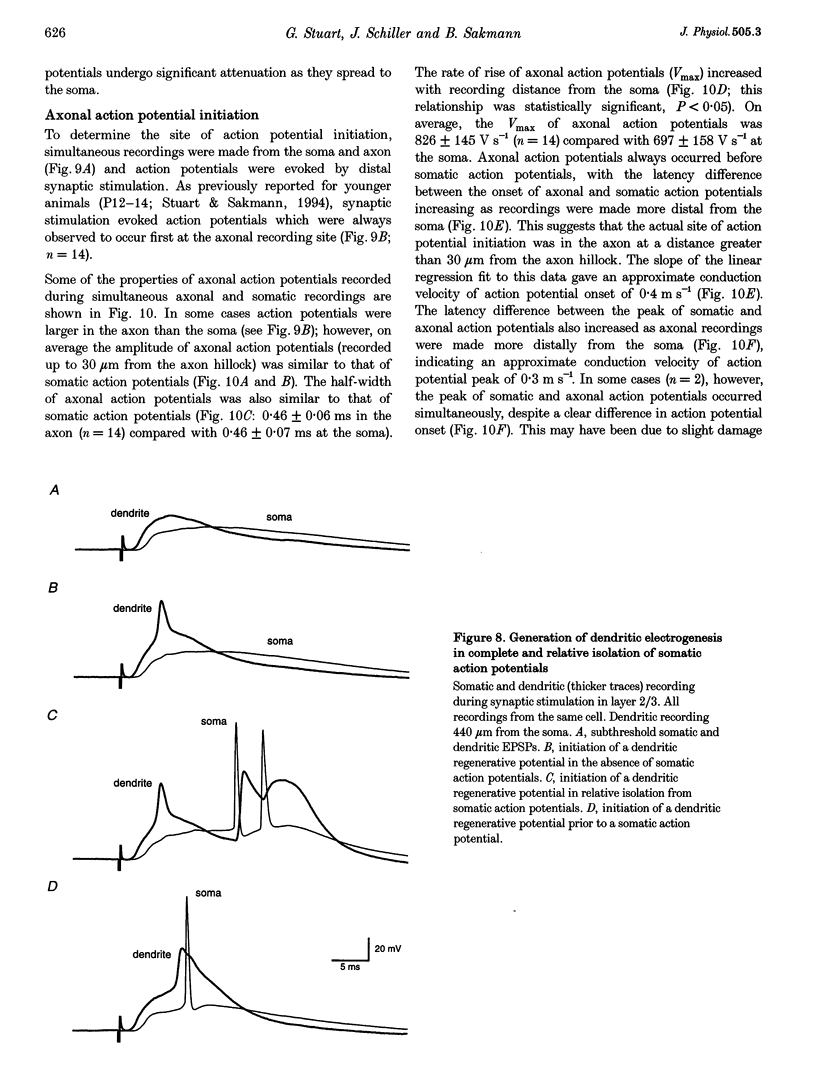
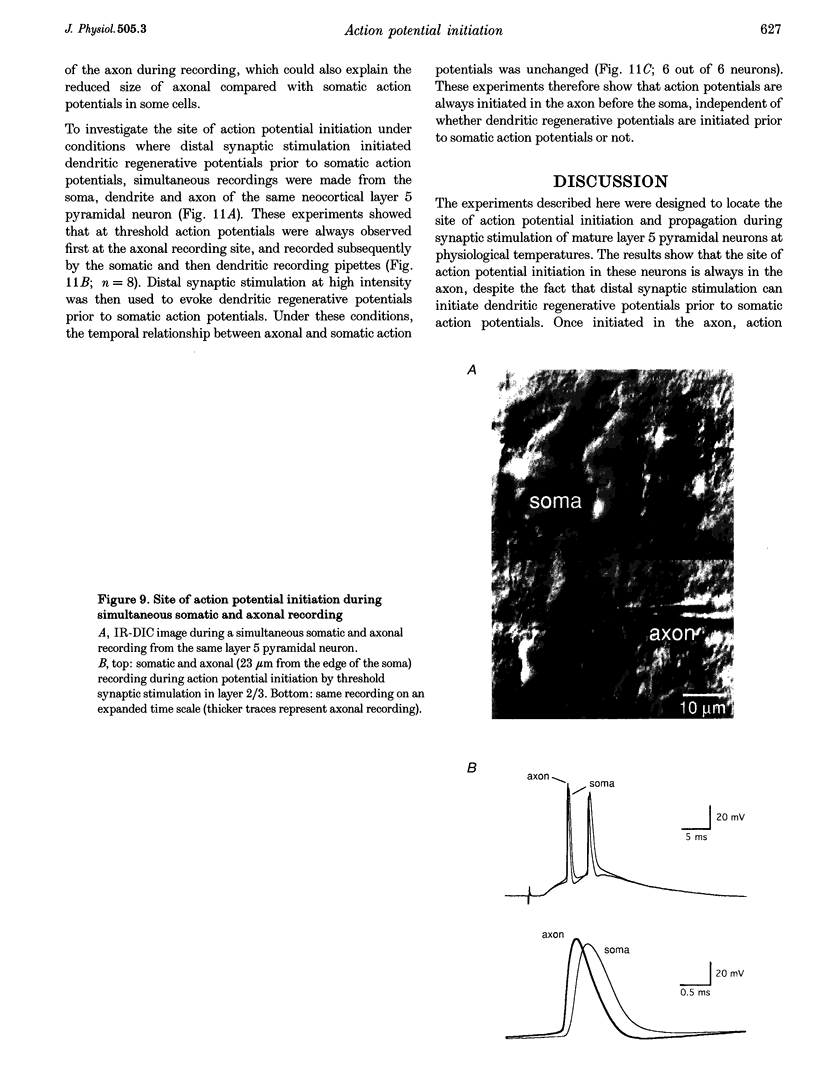
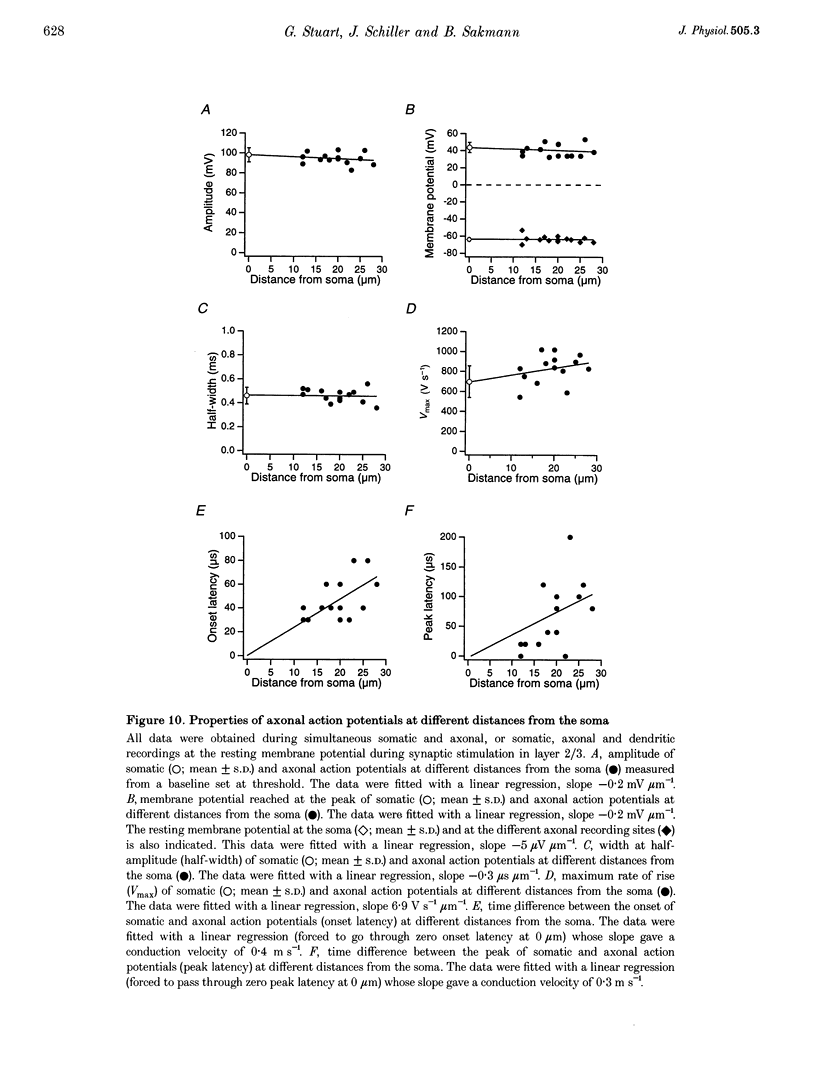
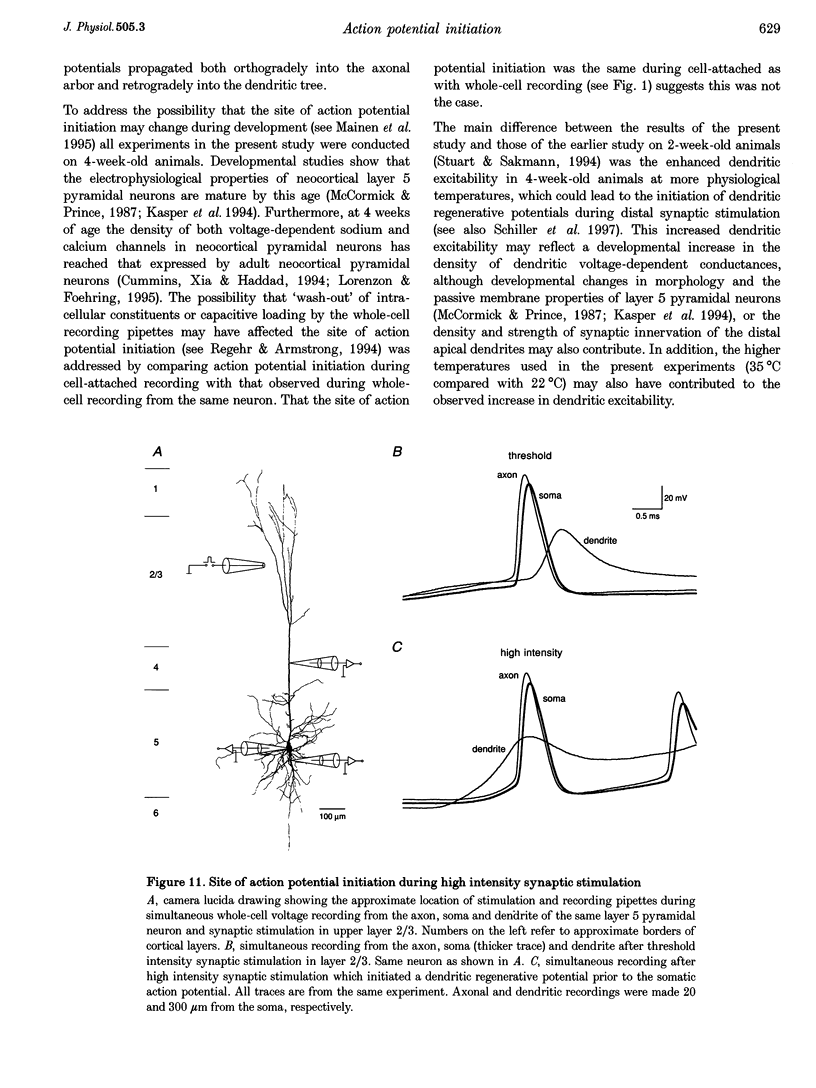
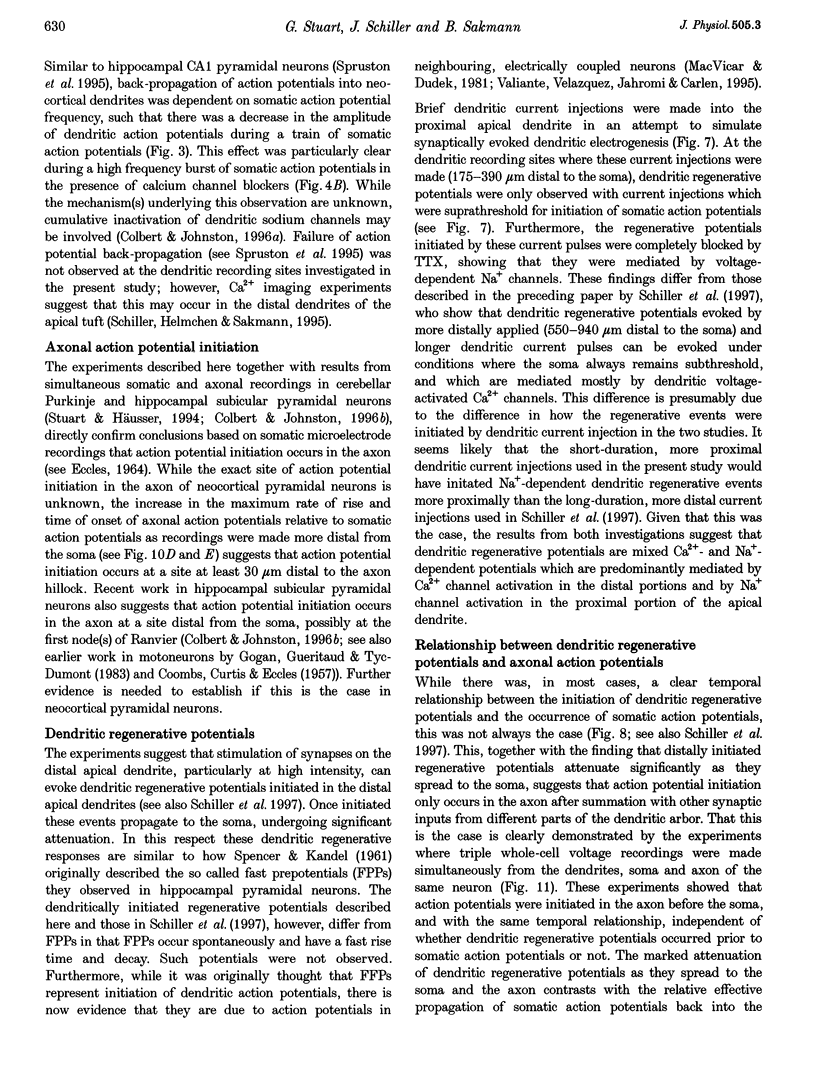
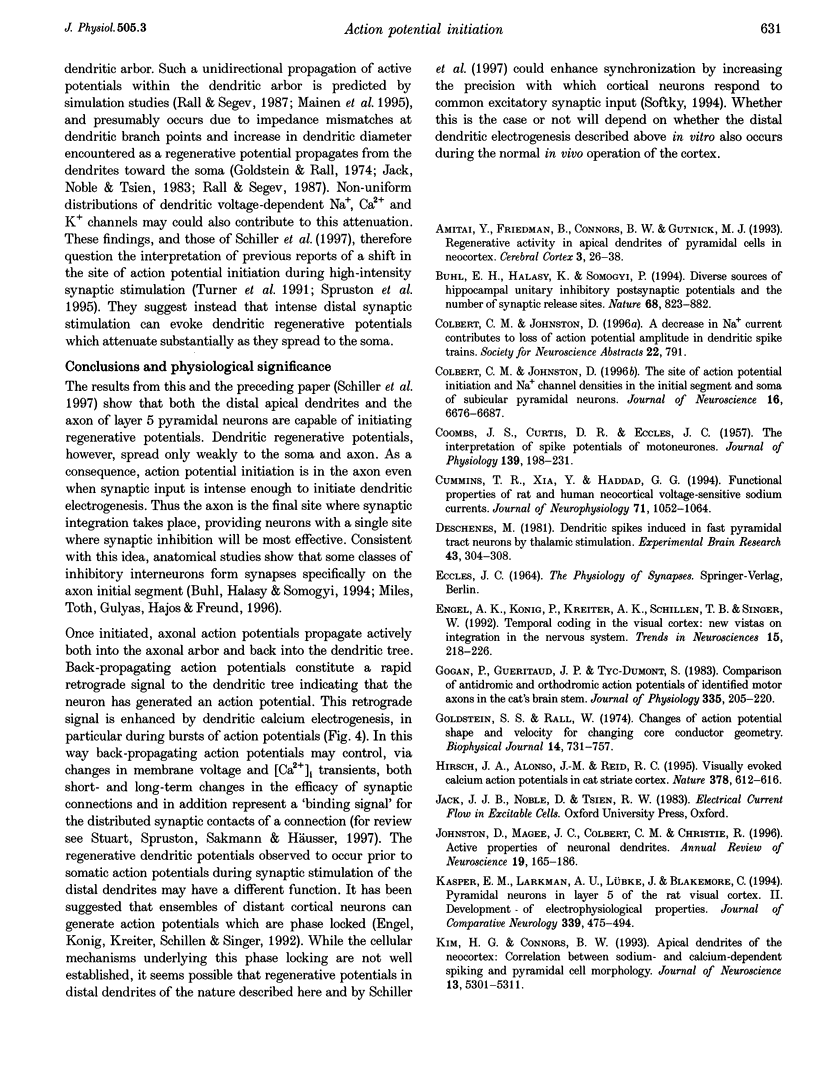
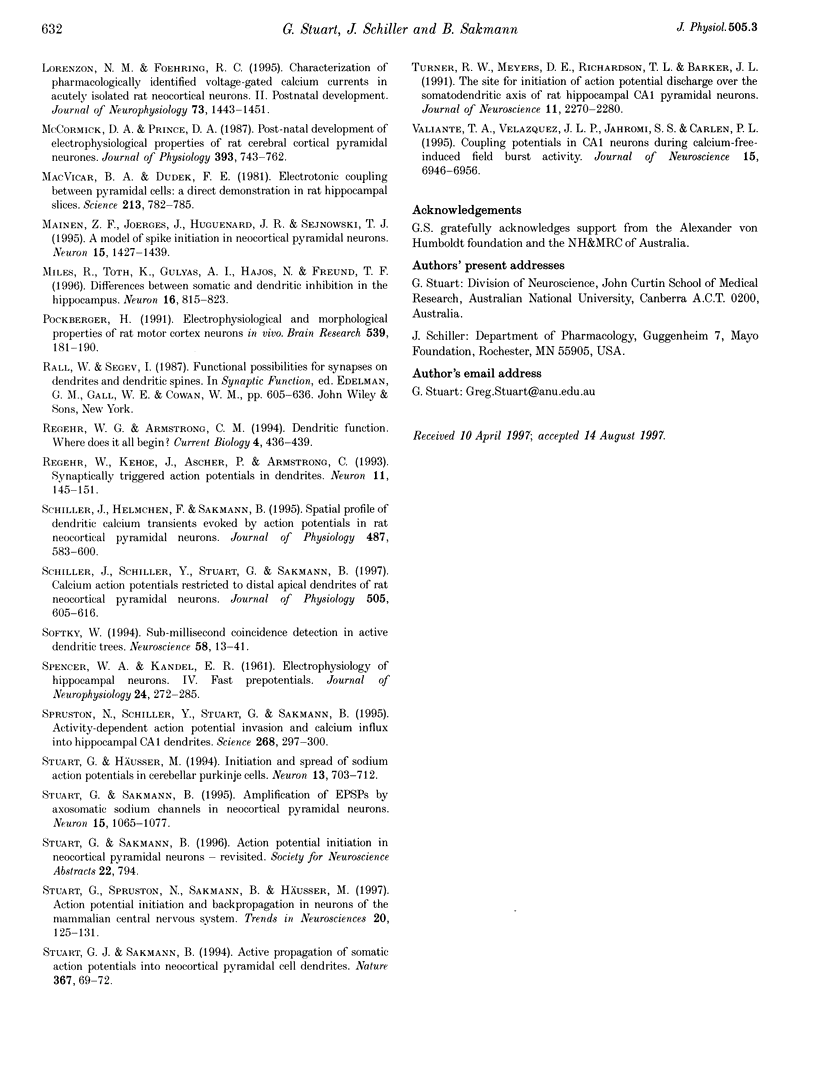
Images in this article
Selected References
These references are in PubMed. This may not be the complete list of references from this article.
- Amitai Y., Friedman A., Connors B. W., Gutnick M. J. Regenerative activity in apical dendrites of pyramidal cells in neocortex. Cereb Cortex. 1993 Jan-Feb;3(1):26–38. doi: 10.1093/cercor/3.1.26. [DOI] [PubMed] [Google Scholar]
- Buhl E. H., Halasy K., Somogyi P. Diverse sources of hippocampal unitary inhibitory postsynaptic potentials and the number of synaptic release sites. Nature. 1994 Apr 28;368(6474):823–828. doi: 10.1038/368823a0. [DOI] [PubMed] [Google Scholar]
- COOMBS J. S., CURTIS D. R., ECCLES J. C. The interpretation of spike potentials of motoneurones. J Physiol. 1957 Dec 3;139(2):198–231. doi: 10.1113/jphysiol.1957.sp005887. [DOI] [PMC free article] [PubMed] [Google Scholar]
- Colbert C. M., Johnston D. Axonal action-potential initiation and Na+ channel densities in the soma and axon initial segment of subicular pyramidal neurons. J Neurosci. 1996 Nov 1;16(21):6676–6686. doi: 10.1523/JNEUROSCI.16-21-06676.1996. [DOI] [PMC free article] [PubMed] [Google Scholar]
- Cummins T. R., Xia Y., Haddad G. G. Functional properties of rat and human neocortical voltage-sensitive sodium currents. J Neurophysiol. 1994 Mar;71(3):1052–1064. doi: 10.1152/jn.1994.71.3.1052. [DOI] [PubMed] [Google Scholar]
- Deschênes M. Dendritic spikes induced in fast pyramidal tract neurons by thalamic stimulation. Exp Brain Res. 1981;43(3-4):304–308. doi: 10.1007/BF00238371. [DOI] [PubMed] [Google Scholar]
- Engel A. K., König P., Kreiter A. K., Schillen T. B., Singer W. Temporal coding in the visual cortex: new vistas on integration in the nervous system. Trends Neurosci. 1992 Jun;15(6):218–226. doi: 10.1016/0166-2236(92)90039-b. [DOI] [PubMed] [Google Scholar]
- Gogan P., Gueritaud J. P., Tyc-Dumont S. Comparison of antidromic and orthodromic action potentials of identified motor axons in the cat's brain stem. J Physiol. 1983 Feb;335:205–220. doi: 10.1113/jphysiol.1983.sp014529. [DOI] [PMC free article] [PubMed] [Google Scholar]
- Goldstein S. S., Rall W. Changes of action potential shape and velocity for changing core conductor geometry. Biophys J. 1974 Oct;14(10):731–757. doi: 10.1016/S0006-3495(74)85947-3. [DOI] [PMC free article] [PubMed] [Google Scholar]
- Hirsch J. A., Alonso J. M., Reid R. C. Visually evoked calcium action potentials in cat striate cortex. Nature. 1995 Dec 7;378(6557):612–616. doi: 10.1038/378612a0. [DOI] [PubMed] [Google Scholar]
- Johnston D., Magee J. C., Colbert C. M., Cristie B. R. Active properties of neuronal dendrites. Annu Rev Neurosci. 1996;19:165–186. doi: 10.1146/annurev.ne.19.030196.001121. [DOI] [PubMed] [Google Scholar]
- Kasper E. M., Larkman A. U., Lübke J., Blakemore C. Pyramidal neurons in layer 5 of the rat visual cortex. II. Development of electrophysiological properties. J Comp Neurol. 1994 Jan 22;339(4):475–494. doi: 10.1002/cne.903390403. [DOI] [PubMed] [Google Scholar]
- Kim H. G., Connors B. W. Apical dendrites of the neocortex: correlation between sodium- and calcium-dependent spiking and pyramidal cell morphology. J Neurosci. 1993 Dec;13(12):5301–5311. doi: 10.1523/JNEUROSCI.13-12-05301.1993. [DOI] [PMC free article] [PubMed] [Google Scholar]
- Lorenzon N. M., Foehring R. C. Characterization of pharmacologically identified voltage-gated calcium channel currents in acutely isolated rat neocortical neurons. II. Postnatal development. J Neurophysiol. 1995 Apr;73(4):1443–1451. doi: 10.1152/jn.1995.73.4.1443. [DOI] [PubMed] [Google Scholar]
- MacVicar B. A., Dudek F. E. Electrotonic coupling between pyramidal cells: a direct demonstration in rat hippocampal slices. Science. 1981 Aug 14;213(4509):782–785. doi: 10.1126/science.6266013. [DOI] [PubMed] [Google Scholar]
- Mainen Z. F., Joerges J., Huguenard J. R., Sejnowski T. J. A model of spike initiation in neocortical pyramidal neurons. Neuron. 1995 Dec;15(6):1427–1439. doi: 10.1016/0896-6273(95)90020-9. [DOI] [PubMed] [Google Scholar]
- McCormick D. A., Prince D. A. Post-natal development of electrophysiological properties of rat cerebral cortical pyramidal neurones. J Physiol. 1987 Dec;393:743–762. doi: 10.1113/jphysiol.1987.sp016851. [DOI] [PMC free article] [PubMed] [Google Scholar]
- Miles R., Tóth K., Gulyás A. I., Hájos N., Freund T. F. Differences between somatic and dendritic inhibition in the hippocampus. Neuron. 1996 Apr;16(4):815–823. doi: 10.1016/s0896-6273(00)80101-4. [DOI] [PubMed] [Google Scholar]
- Pockberger H. Electrophysiological and morphological properties of rat motor cortex neurons in vivo. Brain Res. 1991 Jan 25;539(2):181–190. doi: 10.1016/0006-8993(91)91619-c. [DOI] [PubMed] [Google Scholar]
- Regehr W. G., Armstrong C. M. Dendritic function. Where does it all begin? Curr Biol. 1994 May 1;4(5):436–439. doi: 10.1016/s0960-9822(00)00096-8. [DOI] [PubMed] [Google Scholar]
- Regehr W., Kehoe J. S., Ascher P., Armstrong C. Synaptically triggered action potentials in dendrites. Neuron. 1993 Jul;11(1):145–151. doi: 10.1016/0896-6273(93)90278-y. [DOI] [PubMed] [Google Scholar]
- Schiller J., Helmchen F., Sakmann B. Spatial profile of dendritic calcium transients evoked by action potentials in rat neocortical pyramidal neurones. J Physiol. 1995 Sep 15;487(Pt 3):583–600. doi: 10.1113/jphysiol.1995.sp020902. [DOI] [PMC free article] [PubMed] [Google Scholar]
- Schiller J., Schiller Y., Stuart G., Sakmann B. Calcium action potentials restricted to distal apical dendrites of rat neocortical pyramidal neurons. J Physiol. 1997 Dec 15;505(Pt 3):605–616. doi: 10.1111/j.1469-7793.1997.605ba.x. [DOI] [PMC free article] [PubMed] [Google Scholar]
- Spruston N., Schiller Y., Stuart G., Sakmann B. Activity-dependent action potential invasion and calcium influx into hippocampal CA1 dendrites. Science. 1995 Apr 14;268(5208):297–300. doi: 10.1126/science.7716524. [DOI] [PubMed] [Google Scholar]
- Stuart G. J., Sakmann B. Active propagation of somatic action potentials into neocortical pyramidal cell dendrites. Nature. 1994 Jan 6;367(6458):69–72. doi: 10.1038/367069a0. [DOI] [PubMed] [Google Scholar]
- Stuart G., Häusser M. Initiation and spread of sodium action potentials in cerebellar Purkinje cells. Neuron. 1994 Sep;13(3):703–712. doi: 10.1016/0896-6273(94)90037-x. [DOI] [PubMed] [Google Scholar]
- Stuart G., Sakmann B. Amplification of EPSPs by axosomatic sodium channels in neocortical pyramidal neurons. Neuron. 1995 Nov;15(5):1065–1076. doi: 10.1016/0896-6273(95)90095-0. [DOI] [PubMed] [Google Scholar]
- Stuart G., Spruston N., Sakmann B., Häusser M. Action potential initiation and backpropagation in neurons of the mammalian CNS. Trends Neurosci. 1997 Mar;20(3):125–131. doi: 10.1016/s0166-2236(96)10075-8. [DOI] [PubMed] [Google Scholar]
- Turner R. W., Meyers D. E., Richardson T. L., Barker J. L. The site for initiation of action potential discharge over the somatodendritic axis of rat hippocampal CA1 pyramidal neurons. J Neurosci. 1991 Jul;11(7):2270–2280. doi: 10.1523/JNEUROSCI.11-07-02270.1991. [DOI] [PMC free article] [PubMed] [Google Scholar]
- Valiante T. A., Perez Velazquez J. L., Jahromi S. S., Carlen P. L. Coupling potentials in CA1 neurons during calcium-free-induced field burst activity. J Neurosci. 1995 Oct;15(10):6946–6956. doi: 10.1523/JNEUROSCI.15-10-06946.1995. [DOI] [PMC free article] [PubMed] [Google Scholar]