Abstract
1. The morphological (n = 66) and electrophysiological (n = 41) properties of eighty-six thalamocortical (TC) neurones and those of one interneurone in the cat ventrobasal (VB) thalamus were examined using an in vitro slice preparation. The resting membrane potential for thirty-seven TC neurones was -61.9 +/- 0.7 mV, with thirteen neurones exhibiting delta oscillation with and without DC injection. 2. The voltage-current relationships of TC neurones were highly non-linear, with a mean peak input resistance of 254.4 M omega and a mean steady-state input resistance of 80.6 M omega between -60 and -75 mV. At potentials more positive than -60 mV, outward rectification led to a mean steady-state input resistance of 13.3 M omega. At potentials more negative than -75 mV, there was inward rectification, consisting of a fast component leading to a mean peak input resistance of 14.5 M omega, and a slow time-dependent component leading to a mean steady-state input resistance of 10.6 M omega. 3. Above -60 mV, three types of firing were exhibited by TC neurones. The first was an accelerating pattern associated with little spike broadening and a late component in the spike after-hyperpolarization. The second was an accommodating or intermittent pattern associated with spike broadening, while the third was a burst-suppressed pattern of firing also associated with spike broadening, but with broader spikes of a smaller amplitude. All TC neurones evoked high frequency (310-520 Hz) burst firing mediated by a low threshold Ca2+ potential. 4. Morphologically TC neurones were divided into two groups: Type I (n = 31 neurones) which had larger soma, dendritic arbors that occupied more space, thicker primary dendrites and daughter dendrites that followed a more direct course than Type II (n = 35). The only electrophysiological differences were that Type I neurones (n = 16) had smaller peak input and outward rectification resistance and spike after-hyperpolarization, but greater peak inward rectification resistance, and exhibited delta oscillation less often than Type II (n = 13). 5. The morphologically identified interneurone exhibited no outward rectification, only moderate inward rectification, and no high frequency firing associated with the offset of negative current steps below -55 mV. This interneurone had a regular accommodating firing pattern, but the spike after-hyperpolarization had a late component, unlike the accommodating firing in TC neurones. 6. Therefore, the differentiation of TC neuronal types in the cat VB thalamus based on their morphology was reflected by differences in peak input resistance, outward rectification and spike after-hyperpolarization, which could be accounted for by their difference in soma size. More importantly, the firing pattern of the majority of TC neurones in the cat VB thalamus were different from those of TC neurones in other sensory thalamic nuclei. 7. Thalamocortical neurones in the cat VB thalamus were also clearly distinguishable from the interneurone based on the presence of their prominent outward rectification, peak inward rectification and robust low threshold Ca2+ potentials.
Full text
PDF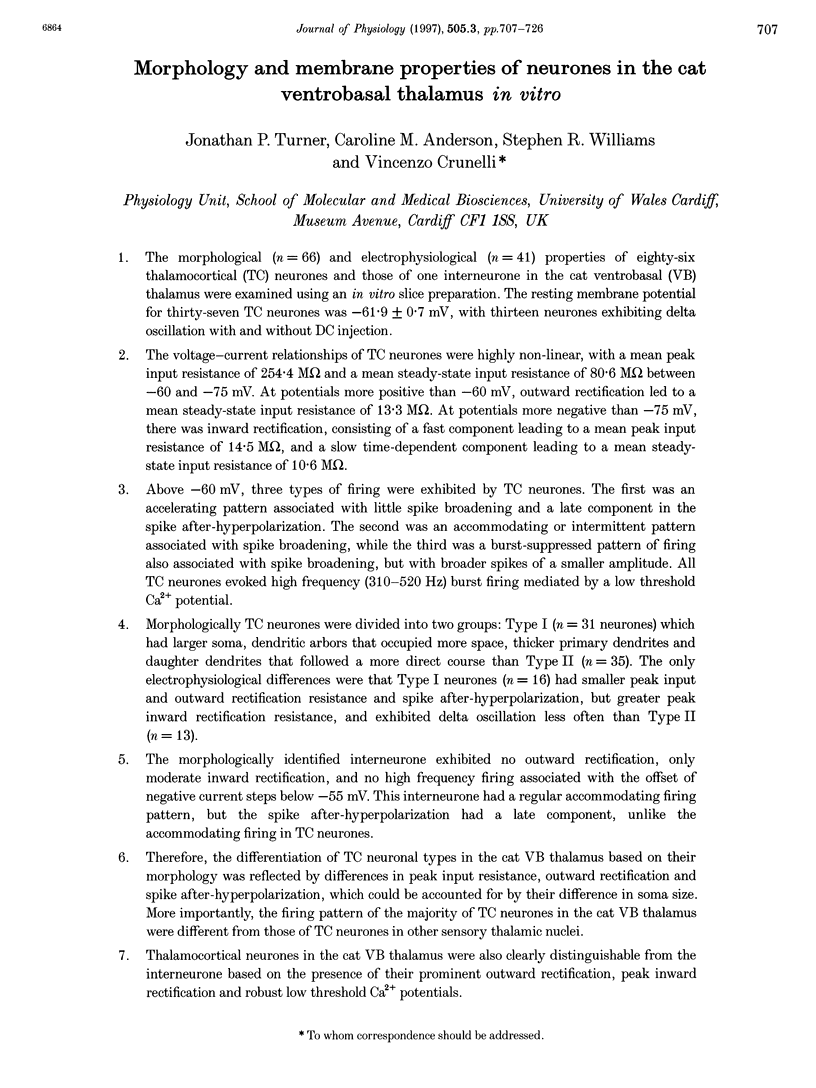
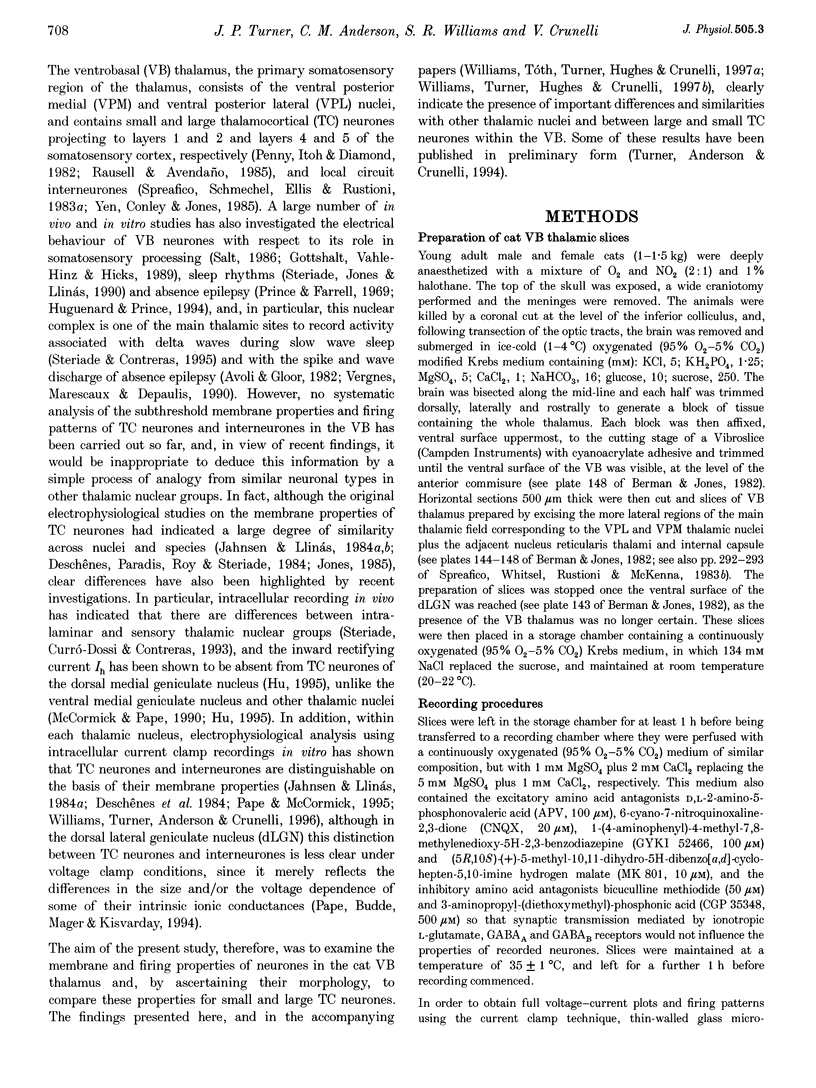
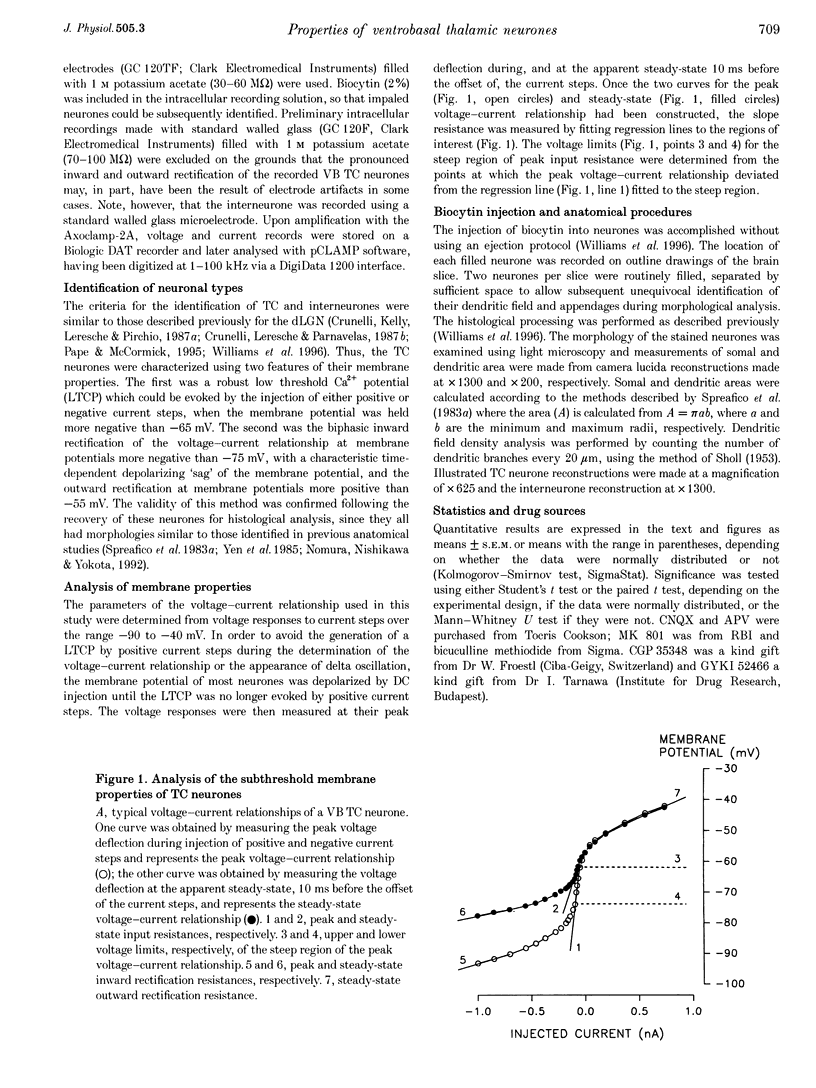
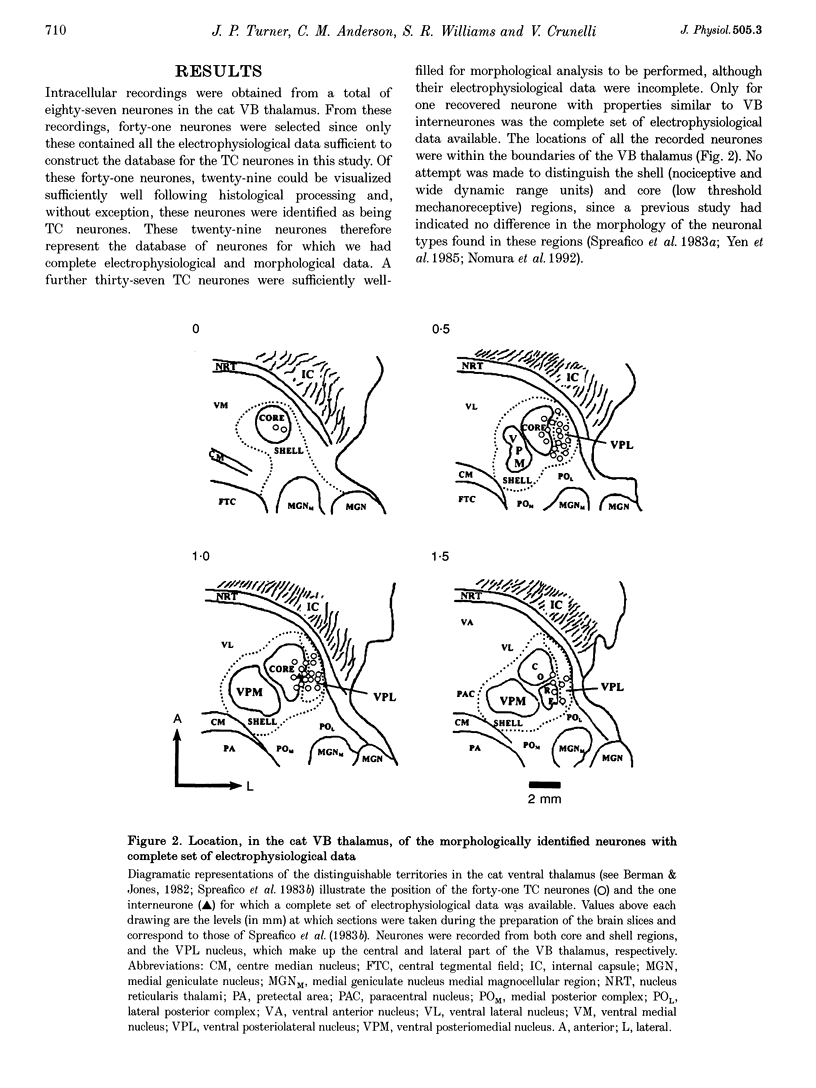
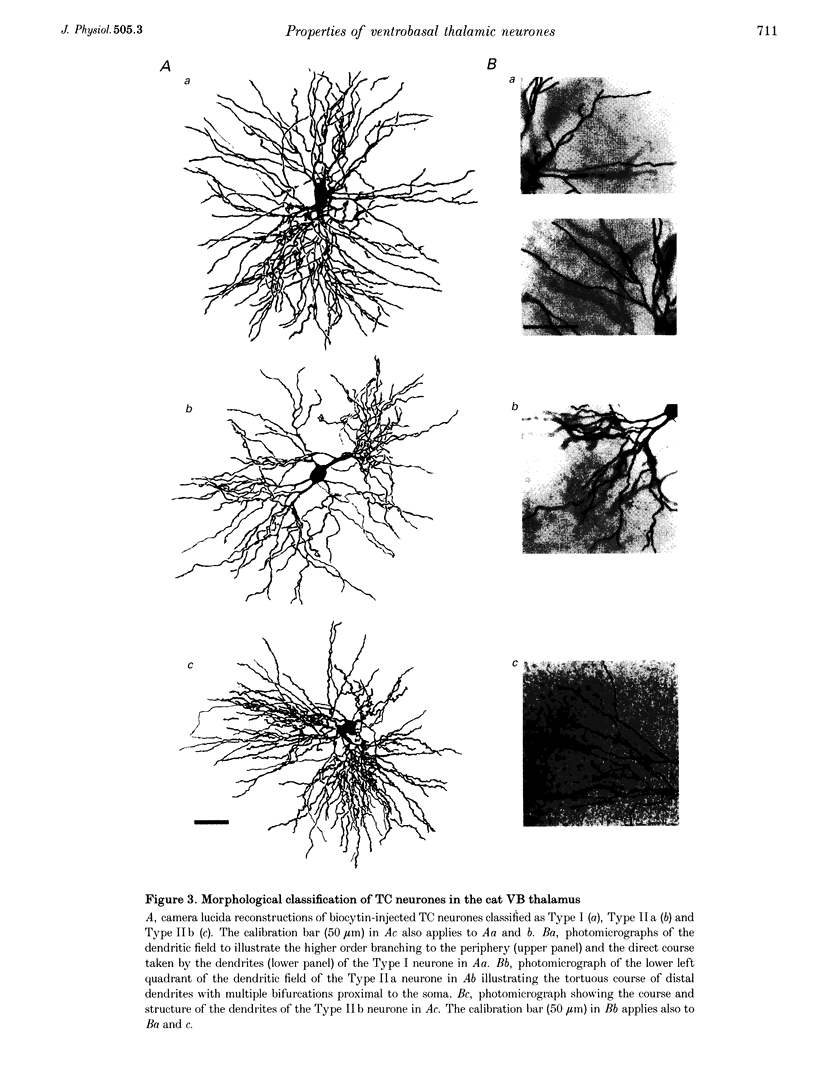
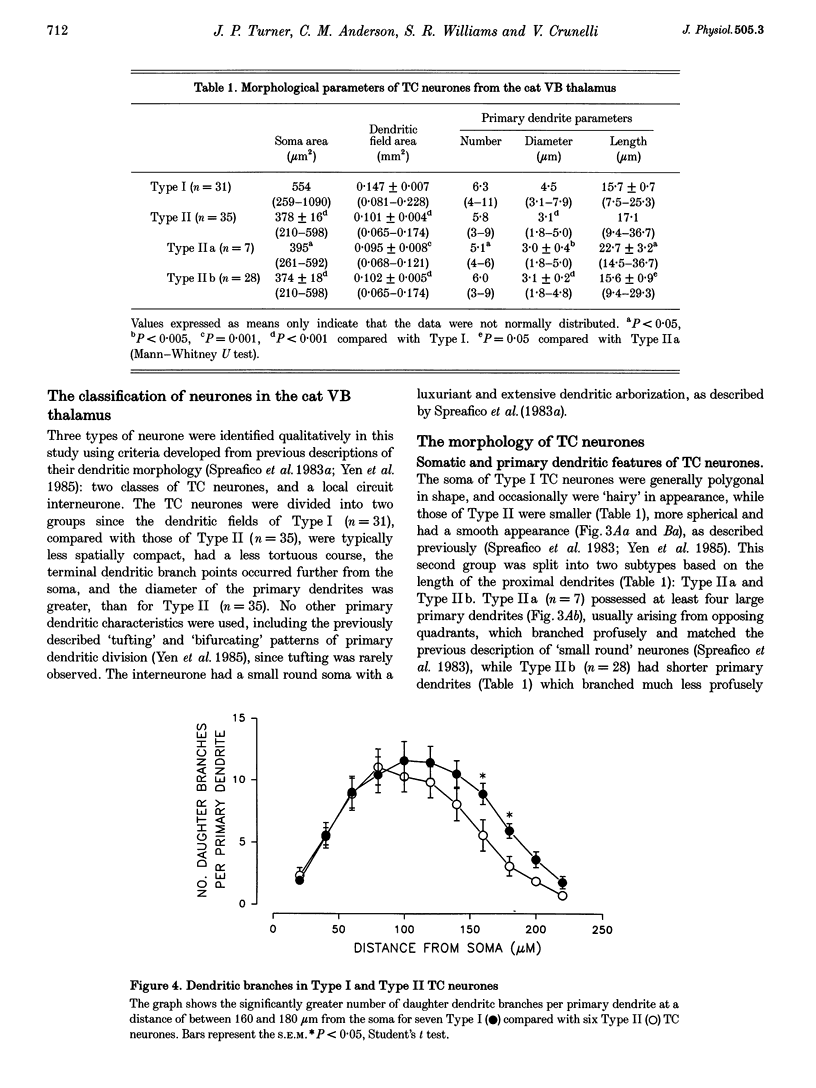
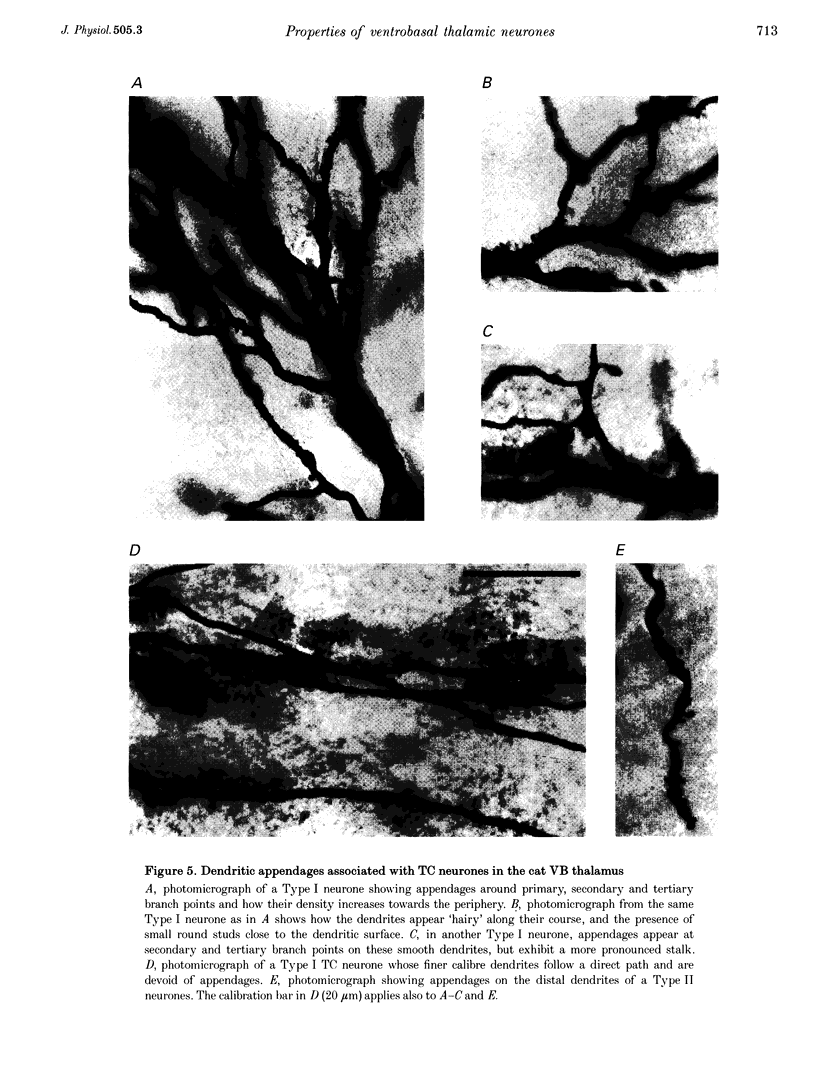
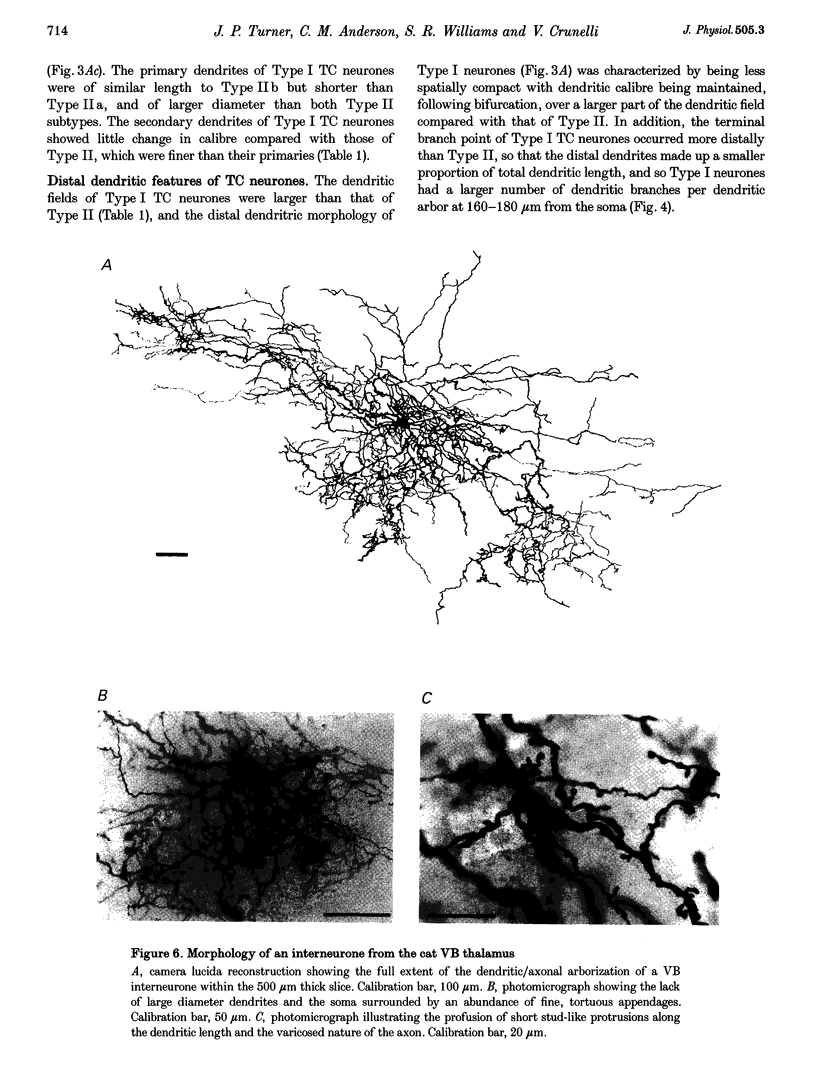
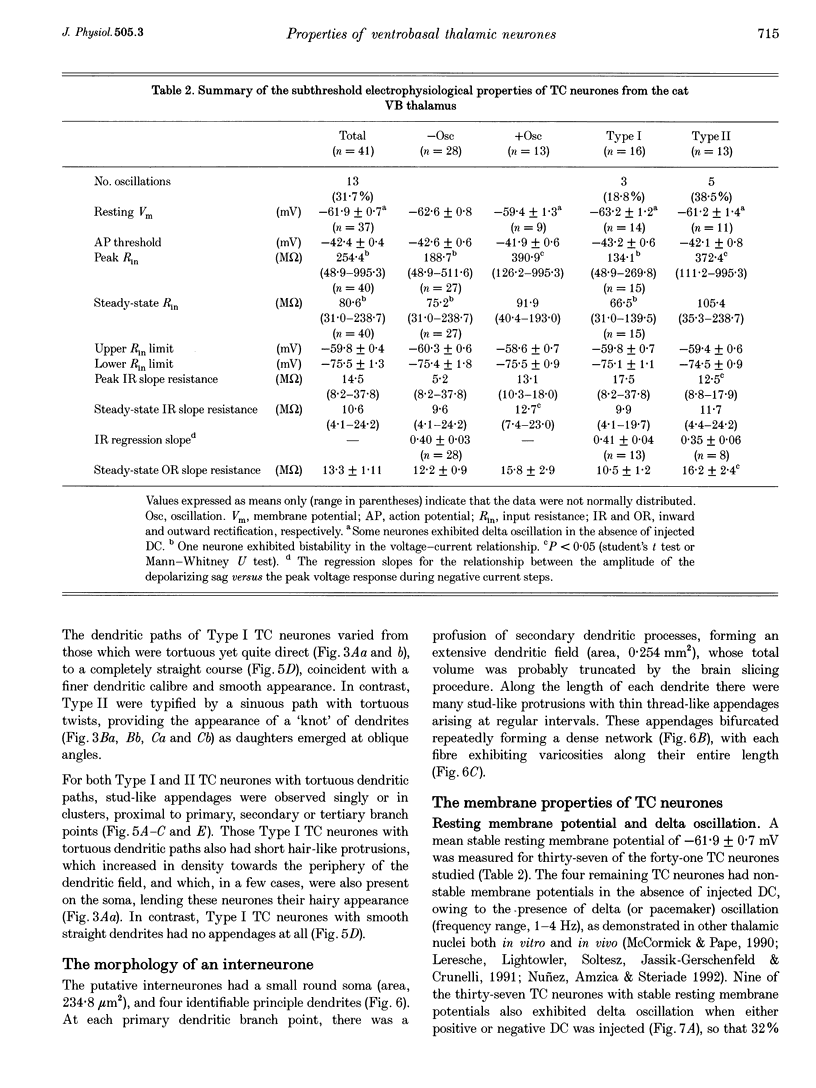
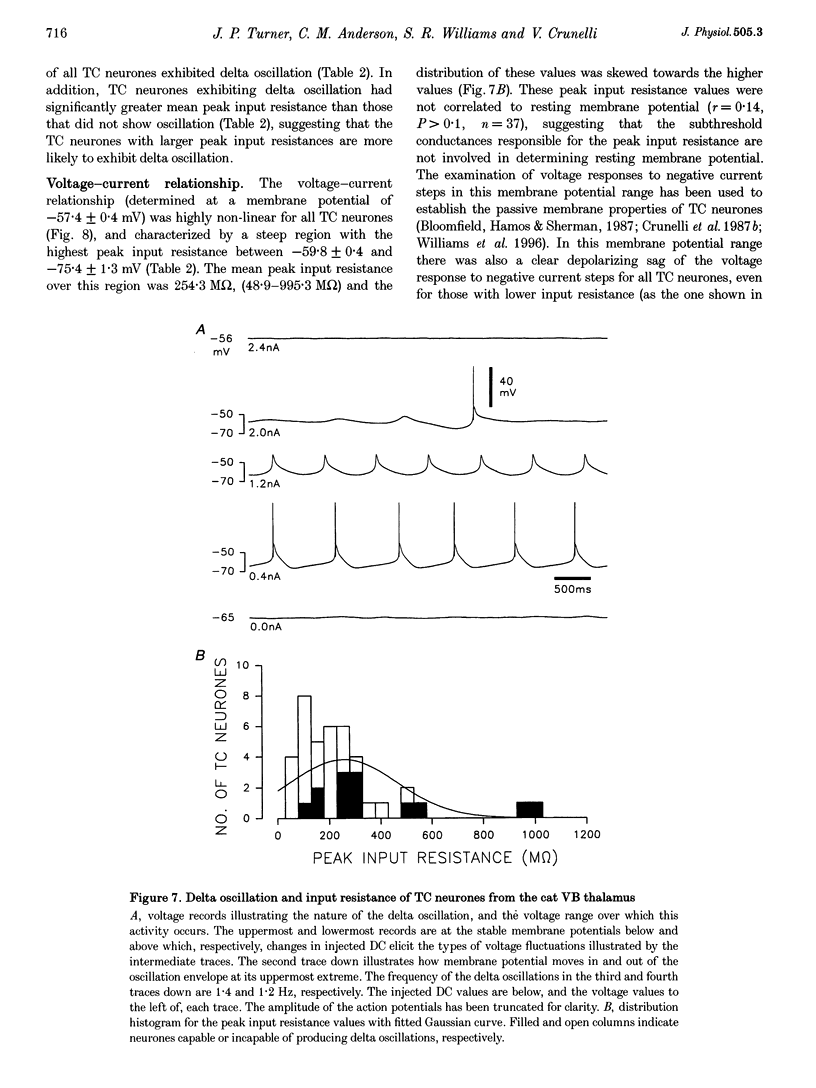
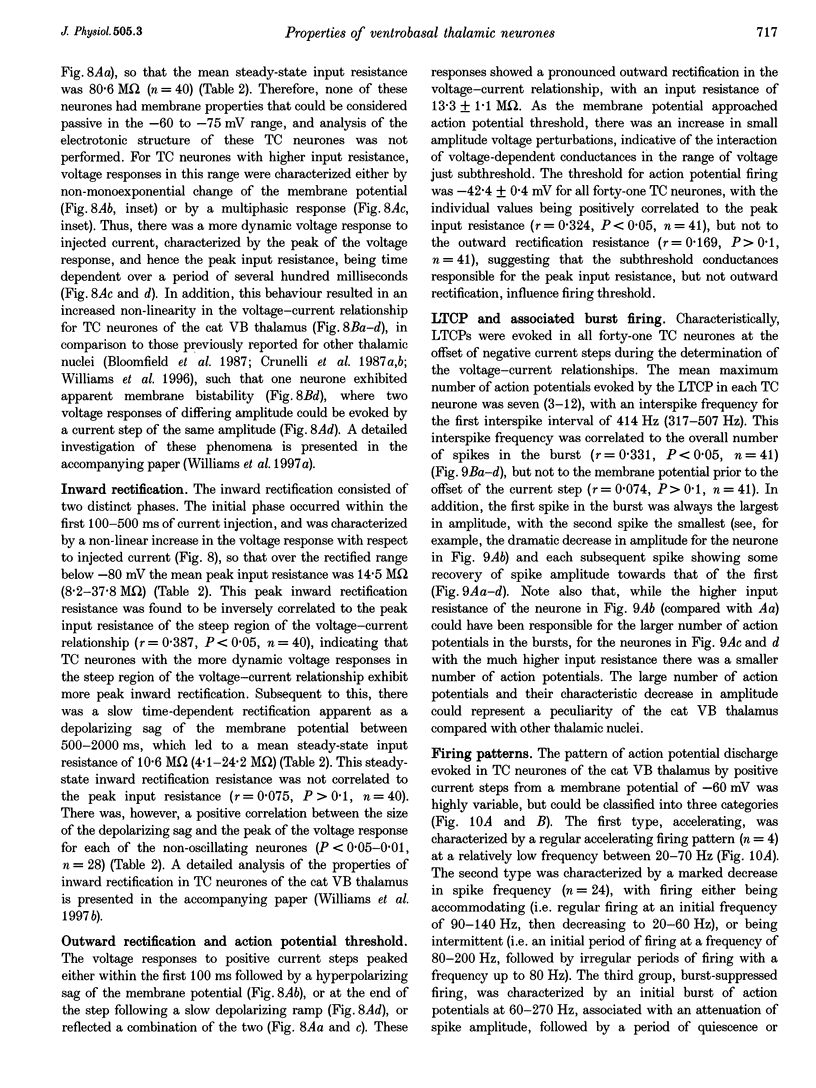
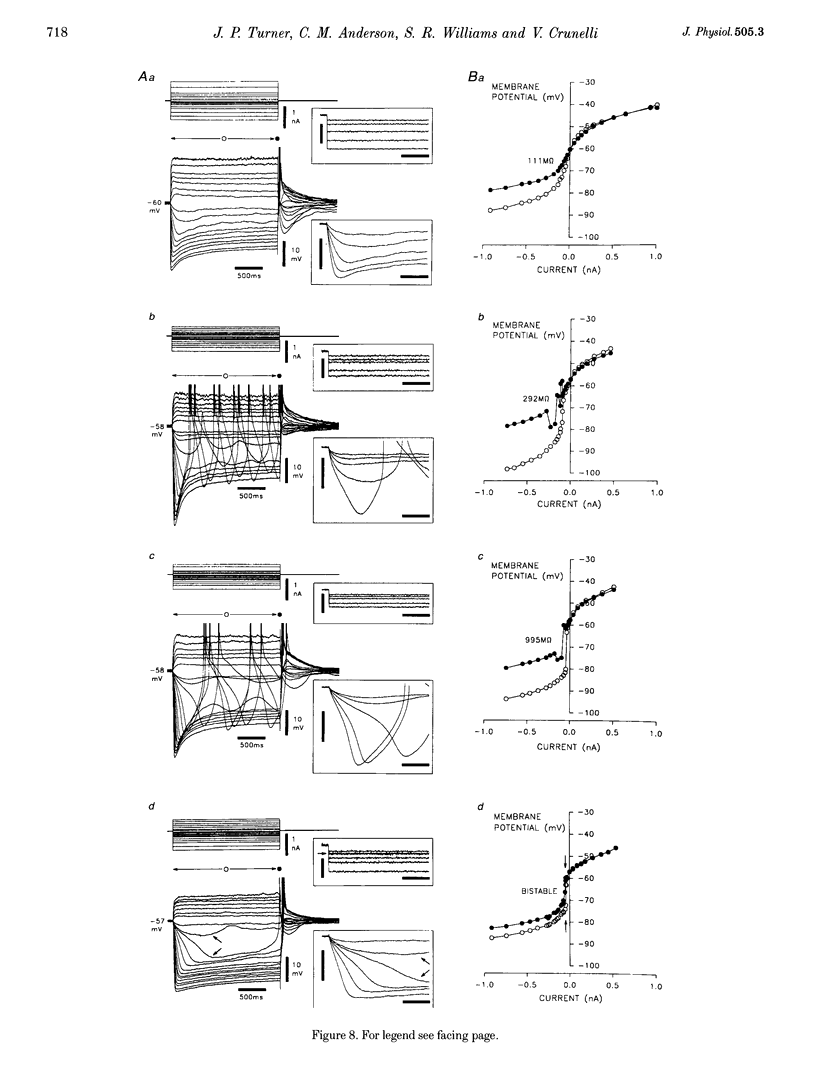
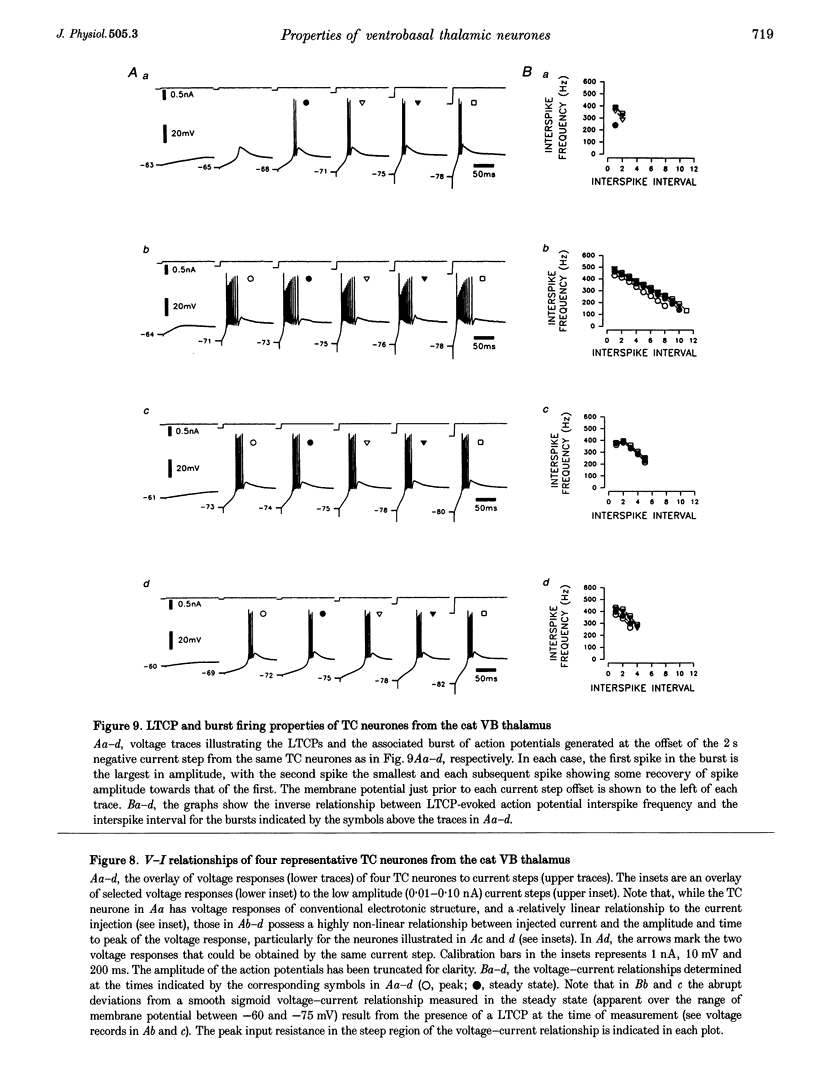
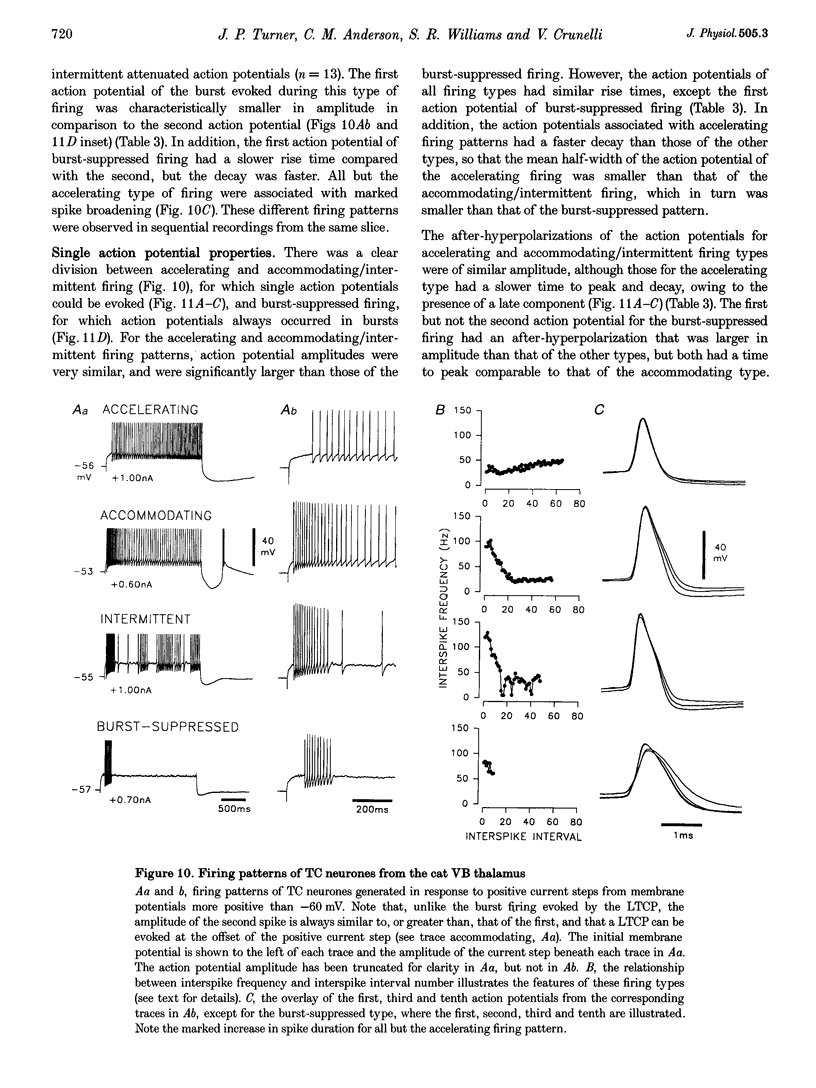
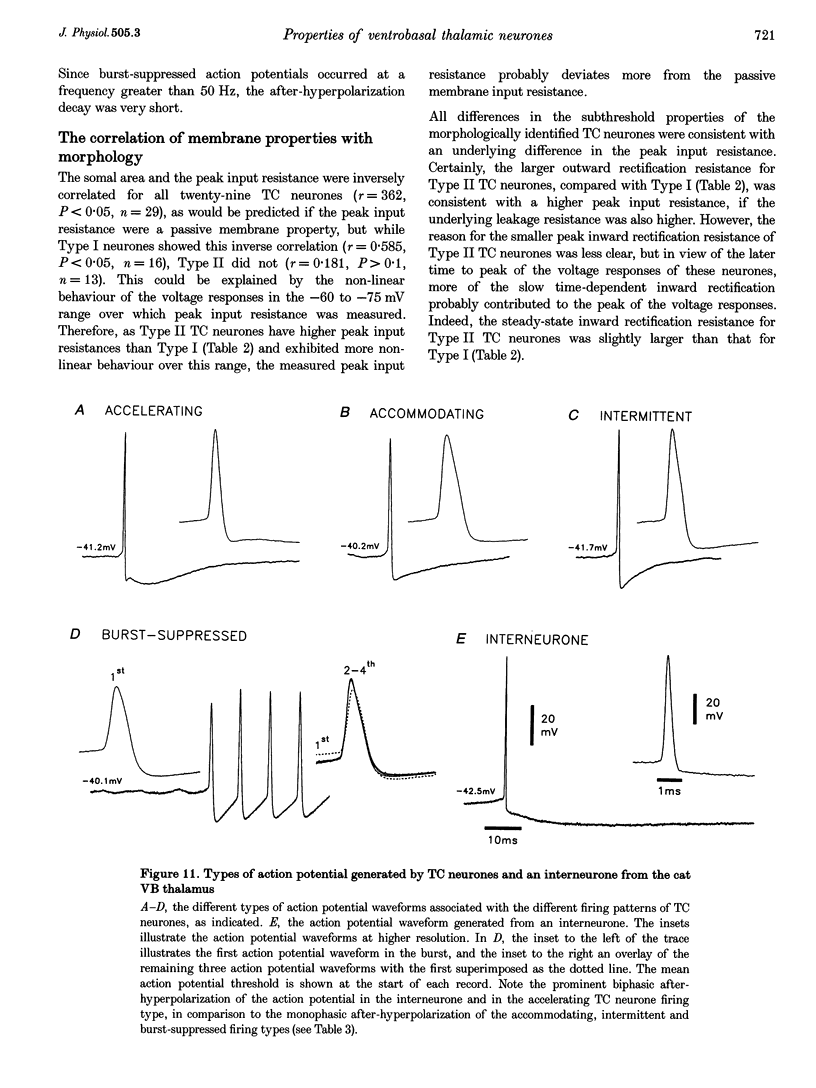
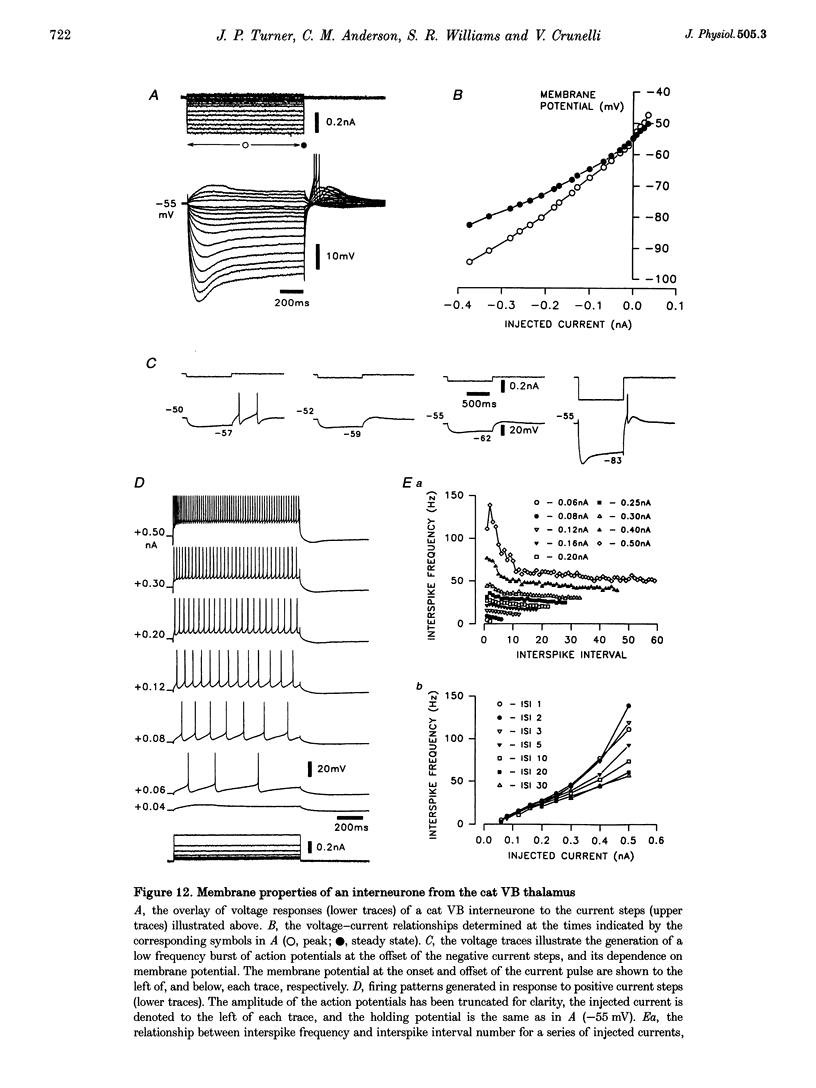
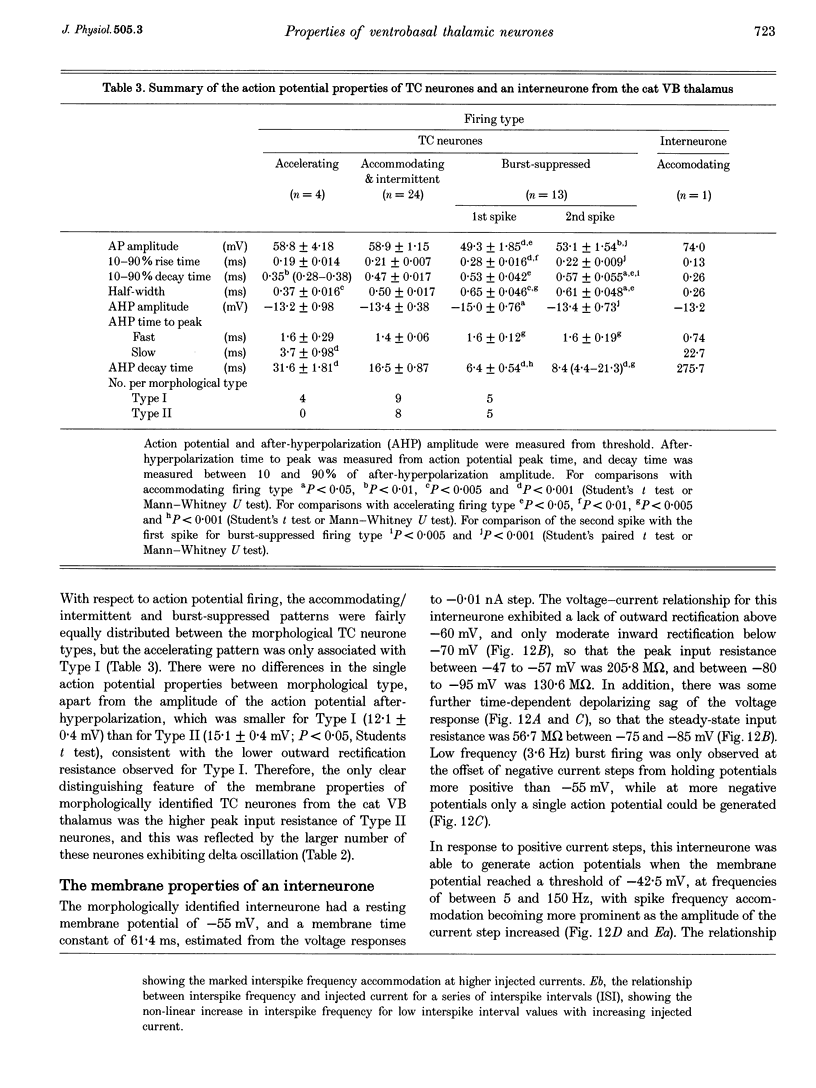
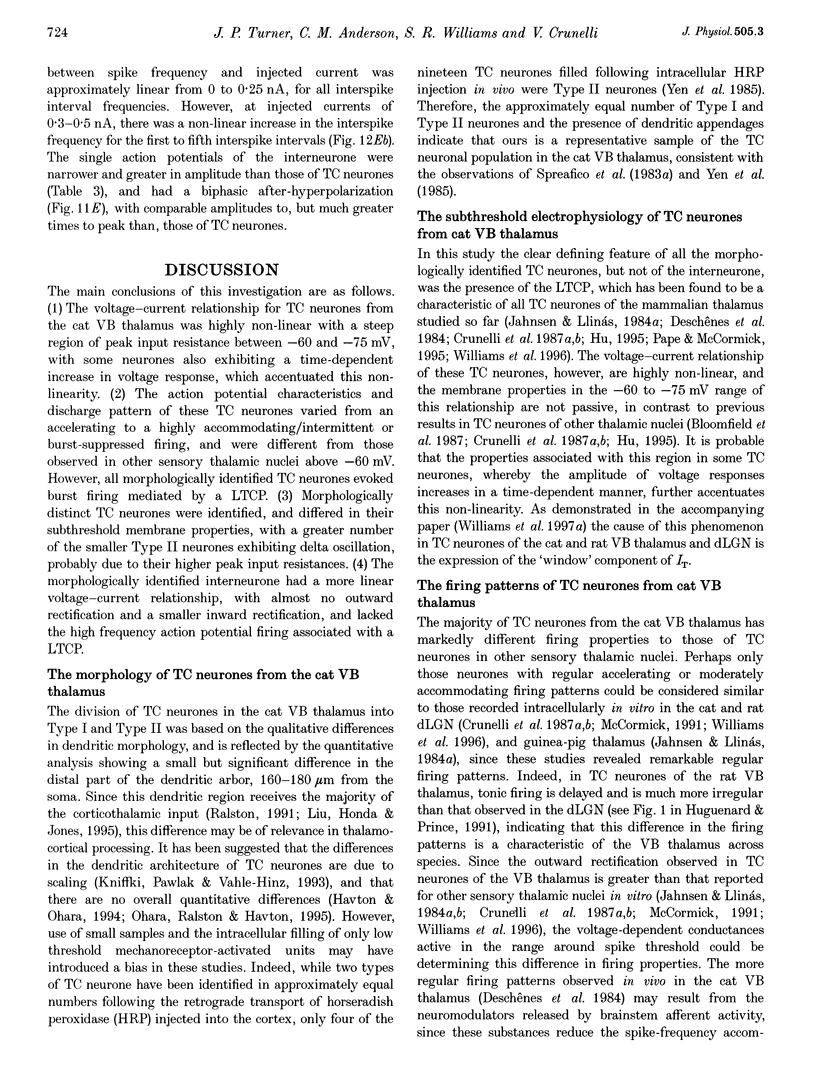
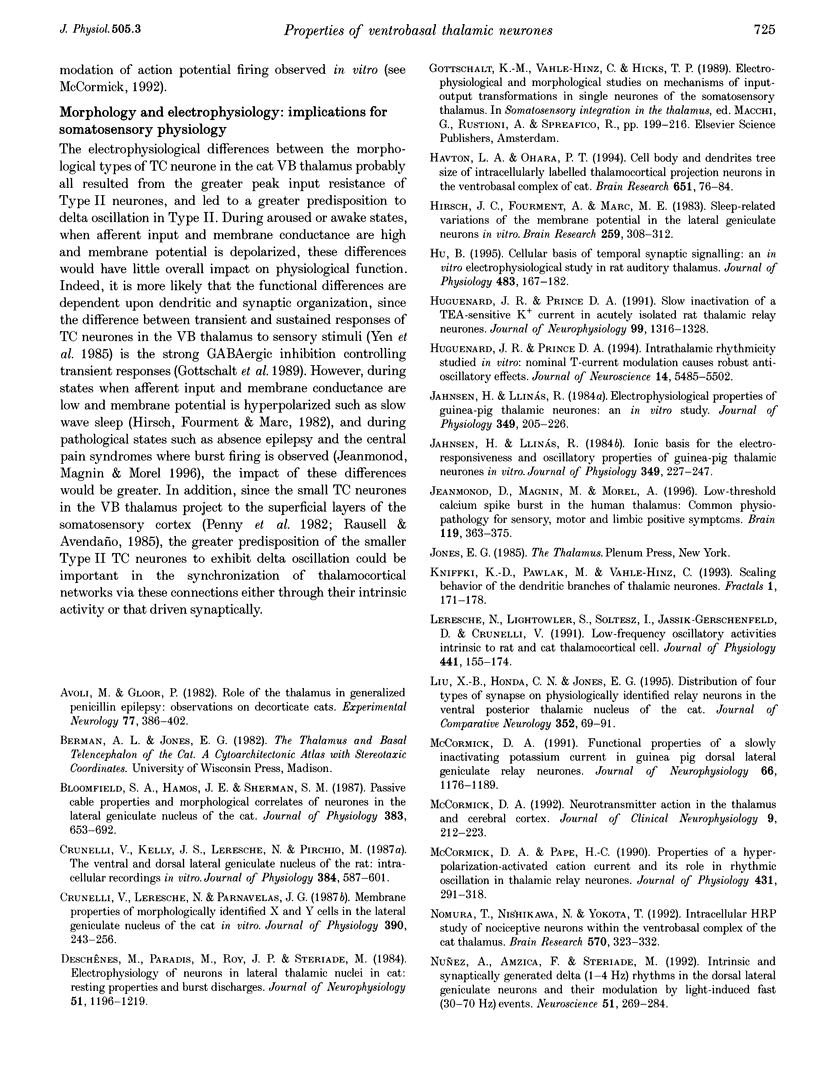
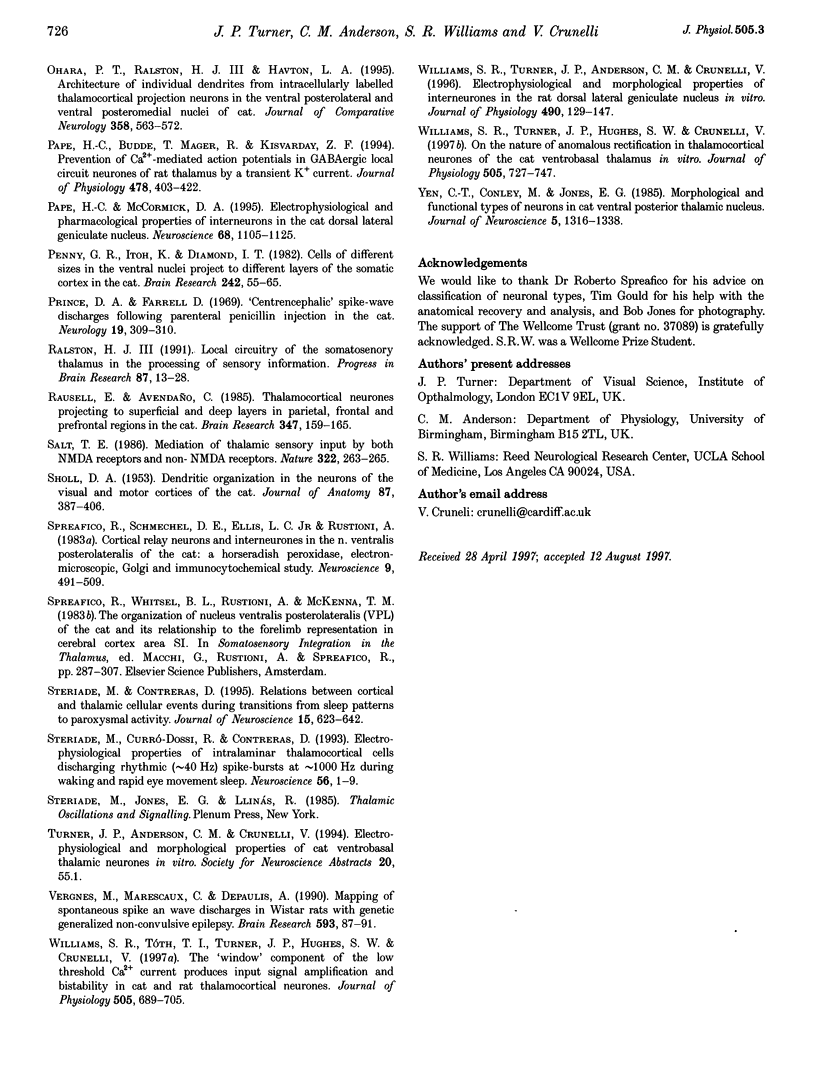
Images in this article
Selected References
These references are in PubMed. This may not be the complete list of references from this article.
- Avoli M., Gloor P. Role of the thalamus in generalized penicillin epilepsy: observations on decorticated cats. Exp Neurol. 1982 Aug;77(2):386–402. doi: 10.1016/0014-4886(82)90252-7. [DOI] [PubMed] [Google Scholar]
- Bloomfield S. A., Hamos J. E., Sherman S. M. Passive cable properties and morphological correlates of neurones in the lateral geniculate nucleus of the cat. J Physiol. 1987 Feb;383:653–692. doi: 10.1113/jphysiol.1987.sp016435. [DOI] [PMC free article] [PubMed] [Google Scholar]
- Crunelli V., Kelly J. S., Leresche N., Pirchio M. The ventral and dorsal lateral geniculate nucleus of the rat: intracellular recordings in vitro. J Physiol. 1987 Mar;384:587–601. doi: 10.1113/jphysiol.1987.sp016471. [DOI] [PMC free article] [PubMed] [Google Scholar]
- Crunelli V., Leresche N., Parnavelas J. G. Membrane properties of morphologically identified X and Y cells in the lateral geniculate nucleus of the cat in vitro. J Physiol. 1987 Sep;390:243–256. doi: 10.1113/jphysiol.1987.sp016697. [DOI] [PMC free article] [PubMed] [Google Scholar]
- Deschênes M., Paradis M., Roy J. P., Steriade M. Electrophysiology of neurons of lateral thalamic nuclei in cat: resting properties and burst discharges. J Neurophysiol. 1984 Jun;51(6):1196–1219. doi: 10.1152/jn.1984.51.6.1196. [DOI] [PubMed] [Google Scholar]
- Havton L. A., Ohara P. T. Cell body and dendritic tree size of intracellularly labeled thalamocortical projection neurons in the ventrobasal complex of cat. Brain Res. 1994 Jul 18;651(1-2):76–84. doi: 10.1016/0006-8993(94)90681-5. [DOI] [PubMed] [Google Scholar]
- Hirsch J. C., Fourment A., Marc M. E. Sleep-related variations of membrane potential in the lateral geniculate body relay neurons of the cat. Brain Res. 1983 Jan 24;259(2):308–312. doi: 10.1016/0006-8993(83)91264-7. [DOI] [PubMed] [Google Scholar]
- Hu B. Cellular basis of temporal synaptic signalling: an in vitro electrophysiological study in rat auditory thalamus. J Physiol. 1995 Feb 15;483(Pt 1):167–182. doi: 10.1113/jphysiol.1995.sp020576. [DOI] [PMC free article] [PubMed] [Google Scholar]
- Huguenard J. R., Prince D. A. Intrathalamic rhythmicity studied in vitro: nominal T-current modulation causes robust antioscillatory effects. J Neurosci. 1994 Sep;14(9):5485–5502. doi: 10.1523/JNEUROSCI.14-09-05485.1994. [DOI] [PMC free article] [PubMed] [Google Scholar]
- Huguenard J. R., Prince D. A. Slow inactivation of a TEA-sensitive K current in acutely isolated rat thalamic relay neurons. J Neurophysiol. 1991 Oct;66(4):1316–1328. doi: 10.1152/jn.1991.66.4.1316. [DOI] [PubMed] [Google Scholar]
- Jahnsen H., Llinás R. Electrophysiological properties of guinea-pig thalamic neurones: an in vitro study. J Physiol. 1984 Apr;349:205–226. doi: 10.1113/jphysiol.1984.sp015153. [DOI] [PMC free article] [PubMed] [Google Scholar]
- Jahnsen H., Llinás R. Ionic basis for the electro-responsiveness and oscillatory properties of guinea-pig thalamic neurones in vitro. J Physiol. 1984 Apr;349:227–247. doi: 10.1113/jphysiol.1984.sp015154. [DOI] [PMC free article] [PubMed] [Google Scholar]
- Jeanmonod D., Magnin M., Morel A. Low-threshold calcium spike bursts in the human thalamus. Common physiopathology for sensory, motor and limbic positive symptoms. Brain. 1996 Apr;119(Pt 2):363–375. doi: 10.1093/brain/119.2.363. [DOI] [PubMed] [Google Scholar]
- Leresche N., Lightowler S., Soltesz I., Jassik-Gerschenfeld D., Crunelli V. Low-frequency oscillatory activities intrinsic to rat and cat thalamocortical cells. J Physiol. 1991 Sep;441:155–174. doi: 10.1113/jphysiol.1991.sp018744. [DOI] [PMC free article] [PubMed] [Google Scholar]
- Liu X. B., Honda C. N., Jones E. G. Distribution of four types of synapse on physiologically identified relay neurons in the ventral posterior thalamic nucleus of the cat. J Comp Neurol. 1995 Jan 30;352(1):69–91. doi: 10.1002/cne.903520106. [DOI] [PubMed] [Google Scholar]
- McCormick D. A. Functional properties of a slowly inactivating potassium current in guinea pig dorsal lateral geniculate relay neurons. J Neurophysiol. 1991 Oct;66(4):1176–1189. doi: 10.1152/jn.1991.66.4.1176. [DOI] [PubMed] [Google Scholar]
- McCormick D. A. Neurotransmitter actions in the thalamus and cerebral cortex. J Clin Neurophysiol. 1992 Apr;9(2):212–223. doi: 10.1097/00004691-199204010-00004. [DOI] [PubMed] [Google Scholar]
- McCormick D. A., Pape H. C. Properties of a hyperpolarization-activated cation current and its role in rhythmic oscillation in thalamic relay neurones. J Physiol. 1990 Dec;431:291–318. doi: 10.1113/jphysiol.1990.sp018331. [DOI] [PMC free article] [PubMed] [Google Scholar]
- Nomura T., Nishikawa N., Yokota T. Intracellular HRP study of nociceptive neurons within the ventrobasal complex of the cat thalamus. Brain Res. 1992 Jan 20;570(1-2):323–332. doi: 10.1016/0006-8993(92)90597-3. [DOI] [PubMed] [Google Scholar]
- Nuñez A., Amzica F., Steriade M. Intrinsic and synaptically generated delta (1-4 Hz) rhythms in dorsal lateral geniculate neurons and their modulation by light-induced fast (30-70 Hz) events. Neuroscience. 1992 Nov;51(2):269–284. doi: 10.1016/0306-4522(92)90314-r. [DOI] [PubMed] [Google Scholar]
- Ohara P. T., Ralston H. J., 3rd, Havton L. A. Architecture of individual dendrites from intracellularly labeled thalamocortical projection neurons in the ventral posterolateral and ventral posteromedial nuclei of cat. J Comp Neurol. 1995 Aug 7;358(4):563–572. doi: 10.1002/cne.903580409. [DOI] [PubMed] [Google Scholar]
- Pape H. C., Budde T., Mager R., Kisvárday Z. F. Prevention of Ca(2+)-mediated action potentials in GABAergic local circuit neurones of rat thalamus by a transient K+ current. J Physiol. 1994 Aug 1;478(Pt 3):403–422. doi: 10.1113/jphysiol.1994.sp020261. [DOI] [PMC free article] [PubMed] [Google Scholar]
- Pape H. C., McCormick D. A. Electrophysiological and pharmacological properties of interneurons in the cat dorsal lateral geniculate nucleus. Neuroscience. 1995 Oct;68(4):1105–1125. doi: 10.1016/0306-4522(95)00205-w. [DOI] [PubMed] [Google Scholar]
- Penny G. R., Itoh K., Diamond I. T. Cells of different sizes in the ventral nuclei project to different layers of the somatic cortex in the cat. Brain Res. 1982 Jun 17;242(1):55–65. doi: 10.1016/0006-8993(82)90495-4. [DOI] [PubMed] [Google Scholar]
- Ralston H. J., 3rd Local circuitry of the somatosensory thalamus in the processing of sensory information. Prog Brain Res. 1991;87:13–28. doi: 10.1016/s0079-6123(08)63045-9. [DOI] [PubMed] [Google Scholar]
- Rausell E., Avendaño C. Thalamocortical neurons projecting to superficial and to deep layers in parietal, frontal and prefrontal regions in the cat. Brain Res. 1985 Nov 11;347(1):159–165. doi: 10.1016/0006-8993(85)90905-9. [DOI] [PubMed] [Google Scholar]
- SHOLL D. A. Dendritic organization in the neurons of the visual and motor cortices of the cat. J Anat. 1953 Oct;87(4):387–406. [PMC free article] [PubMed] [Google Scholar]
- Salt T. E. Mediation of thalamic sensory input by both NMDA receptors and non-NMDA receptors. Nature. 1986 Jul 17;322(6076):263–265. doi: 10.1038/322263a0. [DOI] [PubMed] [Google Scholar]
- Steriade M., Contreras D. Relations between cortical and thalamic cellular events during transition from sleep patterns to paroxysmal activity. J Neurosci. 1995 Jan;15(1 Pt 2):623–642. doi: 10.1523/JNEUROSCI.15-01-00623.1995. [DOI] [PMC free article] [PubMed] [Google Scholar]
- Vergnes M., Marescaux C., Depaulis A. Mapping of spontaneous spike and wave discharges in Wistar rats with genetic generalized non-convulsive epilepsy. Brain Res. 1990 Jul 16;523(1):87–91. doi: 10.1016/0006-8993(90)91638-w. [DOI] [PubMed] [Google Scholar]
- Williams S. R., Tóth T. I., Turner J. P., Hughes S. W., Crunelli V. The 'window' component of the low threshold Ca2+ current produces input signal amplification and bistability in cat and rat thalamocortical neurones. J Physiol. 1997 Dec 15;505(Pt 3):689–705. doi: 10.1111/j.1469-7793.1997.689ba.x. [DOI] [PMC free article] [PubMed] [Google Scholar]
- Yen C. T., Conley M., Jones E. G. Morphological and functional types of neurons in cat ventral posterior thalamic nucleus. J Neurosci. 1985 May;5(5):1316–1338. doi: 10.1523/JNEUROSCI.05-05-01316.1985. [DOI] [PMC free article] [PubMed] [Google Scholar]