Abstract
1. Single muscle fibres were dissociated enzymatically from the extensor digitorum longus and communis muscles of rats and guinea-pigs. The fibres were mounted into a double Vaseline gap experimental chamber and the events in excitation-contraction coupling were studied under voltage clamp conditions. 2. The voltage dependence of intramembrane charge movement followed a two-state Boltzmann distribution with maximal available charge of 26.1 +/- 1.5 and 26.1 +/- 1.3 nC microF-1, mid-point voltage of -35.1 +/- 5.0 and -42.2 +/- 1.2 mV and steepness of 16.7 +/- 2.2 and 17.0 +/- 1.9 mV (means +/- S.E.M., n = 7 and 4) in rats and guinea-pigs, respectively. 3. Intracellular calcium concentration ([Ca2+]i) was monitored using the calcium-sensitive dyes antipyrylazo III, fura-2 and mag-fura-5. Resting [Ca2+]i was similar in rats and guinea-pigs with 125 +/- 18 and 115 +/- 8 nM (n = 10 and 9), respectively, while the maximal increase for a 100 ms depolarization to 0 mV was larger in rats (6.3 +/- 1.0 microM; n = 7), than in guinea-pigs (2.8 +/- 0.3; n = 4). 4. The rate of calcium release (Rrel) from the sarcoplasmic reticulum (SR) displayed an early peak followed by a fast and a slow decline to a quasi maintained steady level. After normalizing Rrel to the estimated SR calcium content (1.2 +/- 0.1 and 0.9 +/- 0.1 mM in rats and guinea-pigs, respectively) and correcting for depletion of calcium in the SR the peak and steady levels at 0 mV, respectively, were found to be 2.50 +/- 0.08 and 0.81 +/- 0.06% ms-1 in rats and 2.43 +/- 0.25 and 0.88 +/- 0.01% ms-1 in guinea-pigs. The voltage dependence was essentially the same in both species, but different from that in amphibians. 5. These experiments show that enzymatic isolation yields functionally intact mammalian skeletal muscle fibres for Vaseline gap experiments. The data also suggest a close connection in the regulation of the different kinetic components of SR calcium release in mammalian skeletal muscle.
Full text
PDF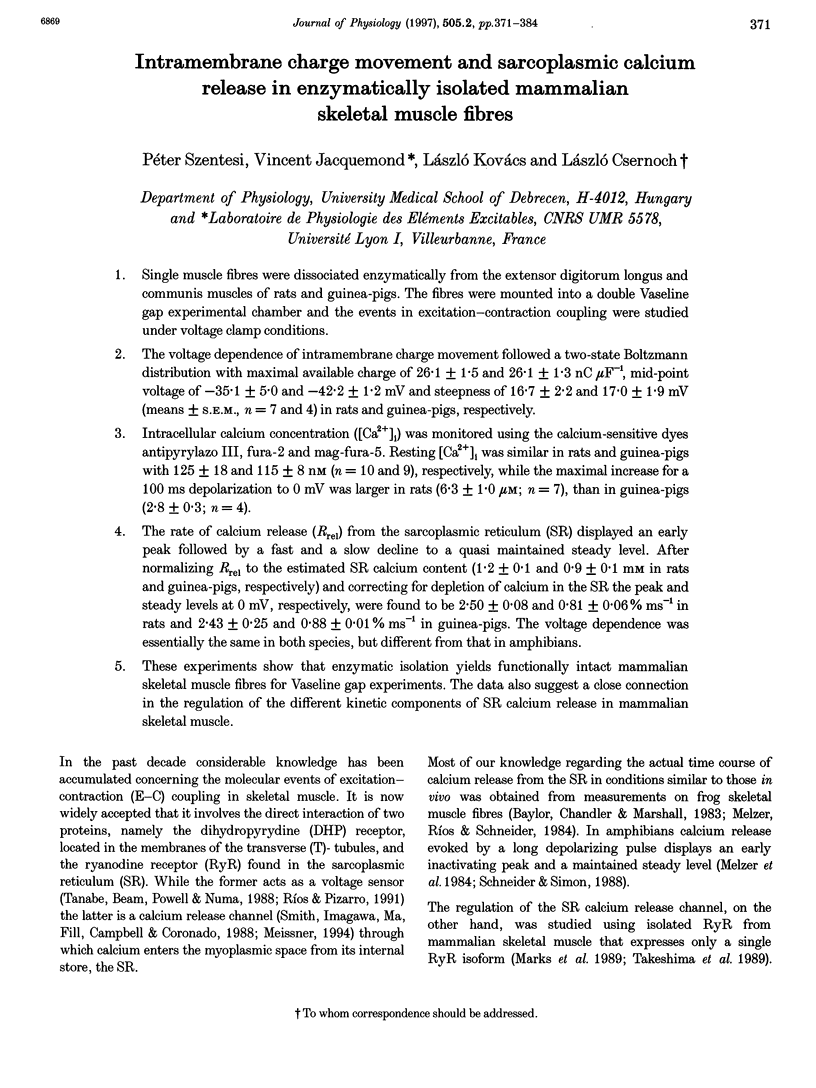
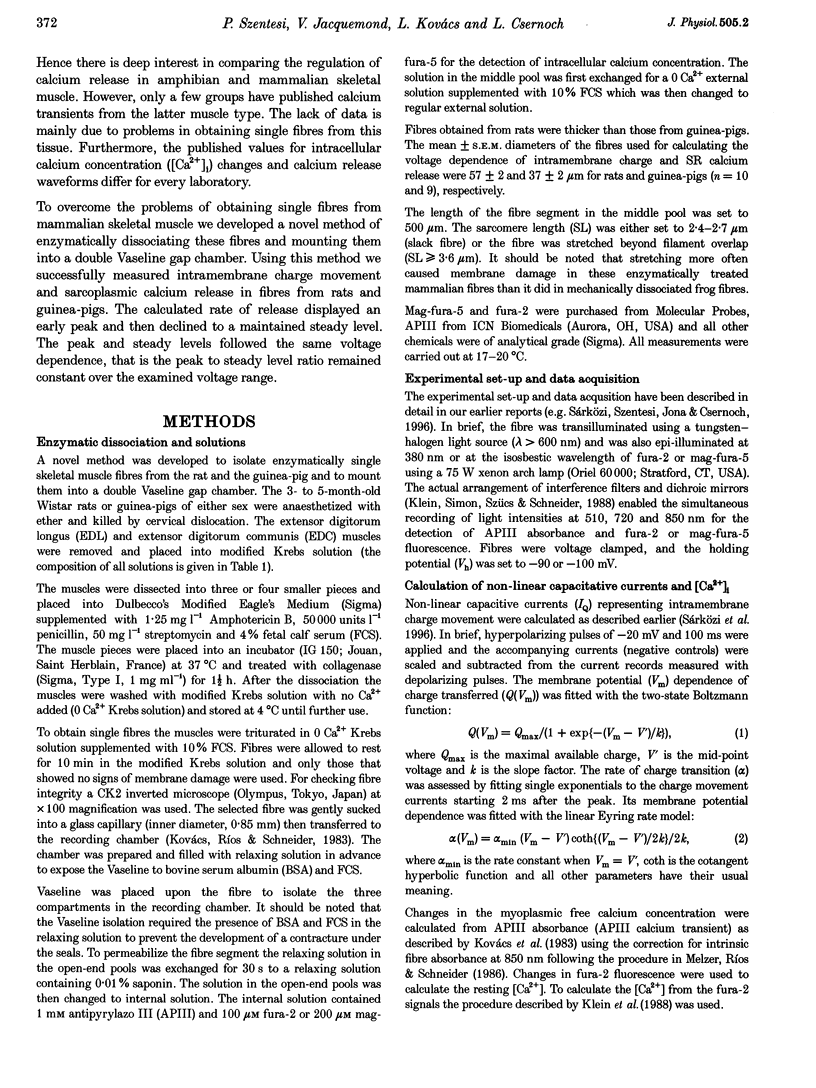
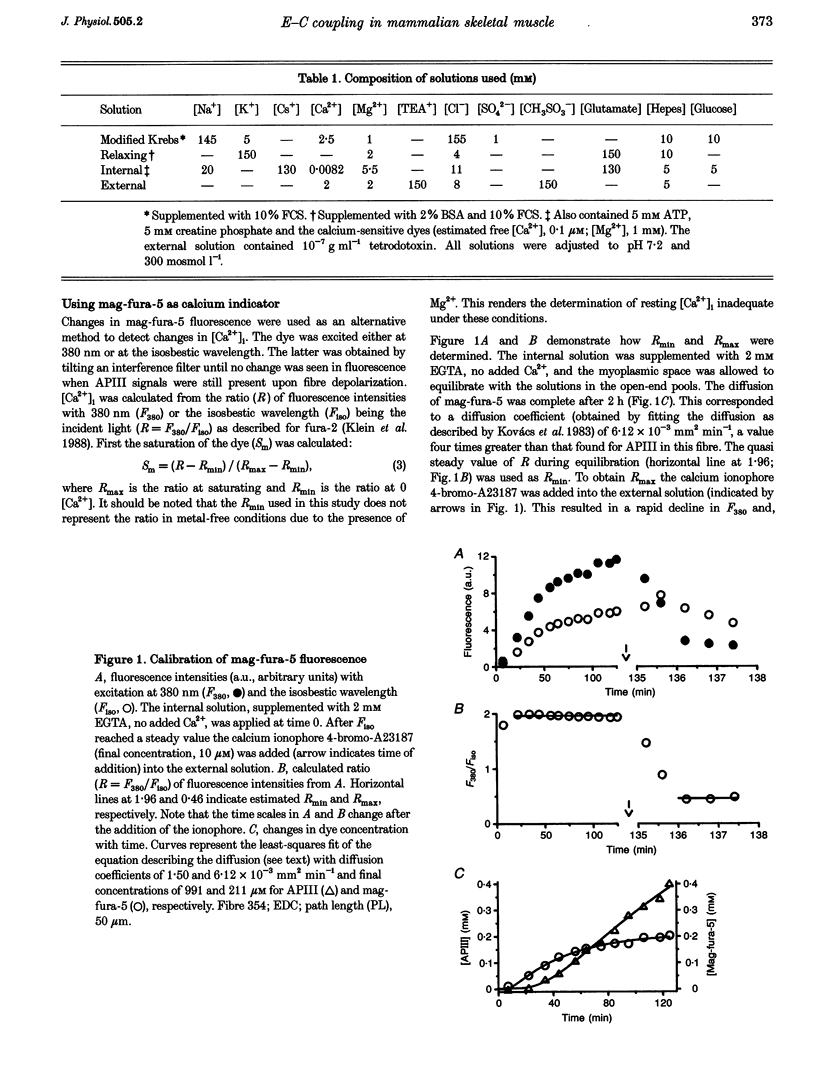
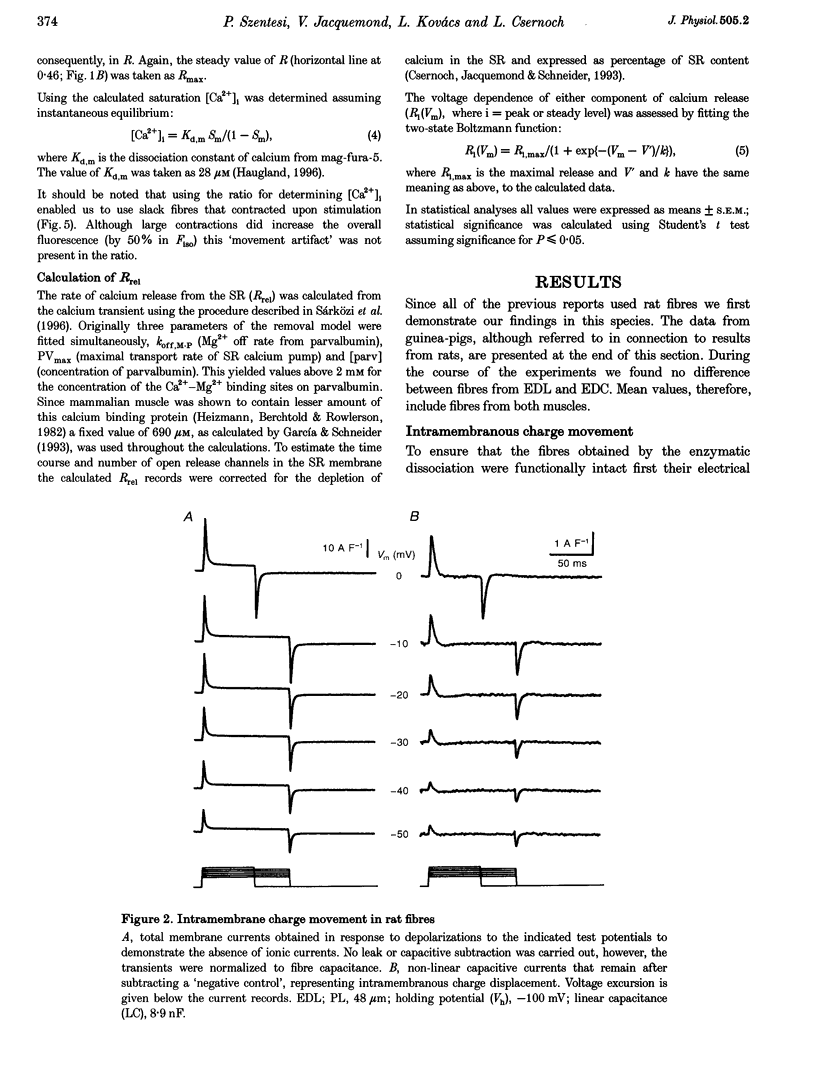
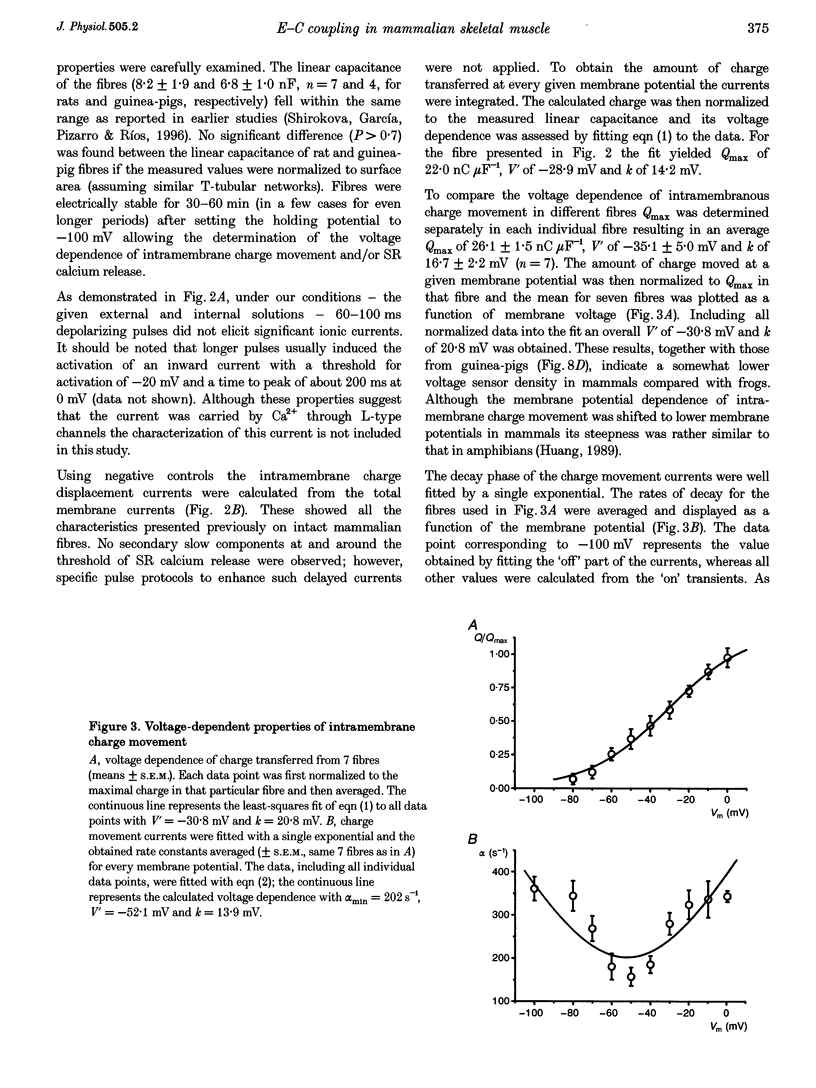
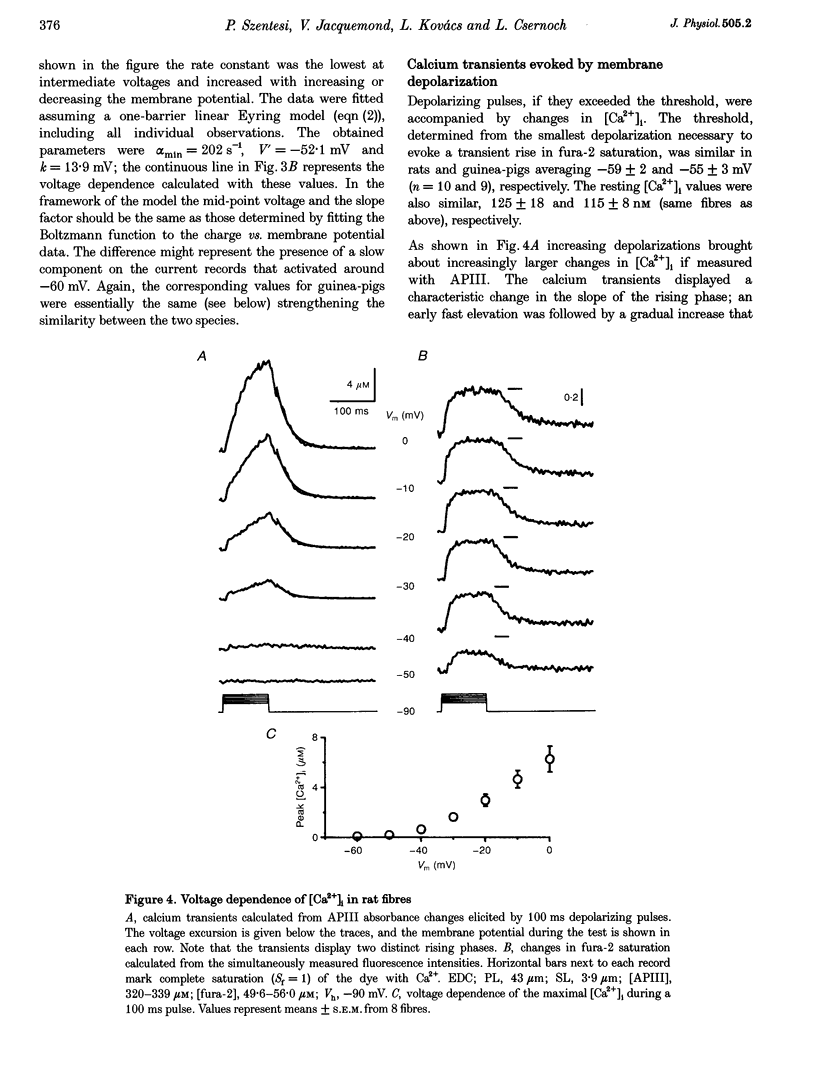
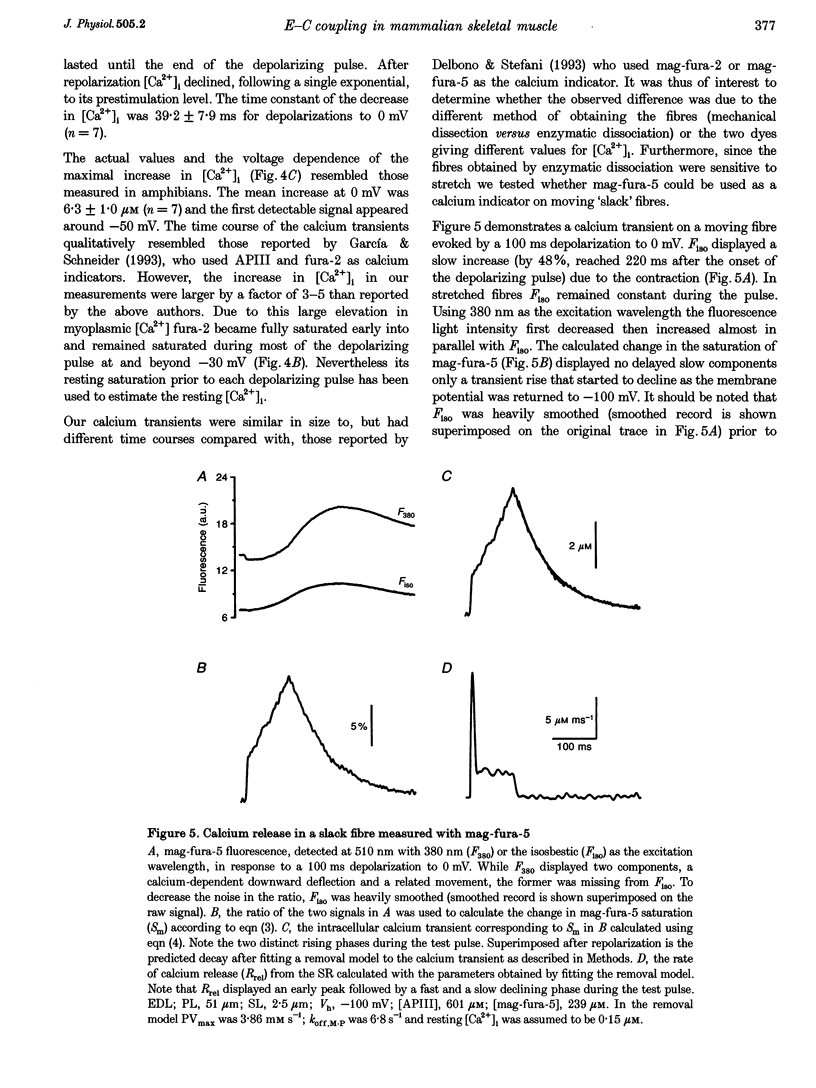
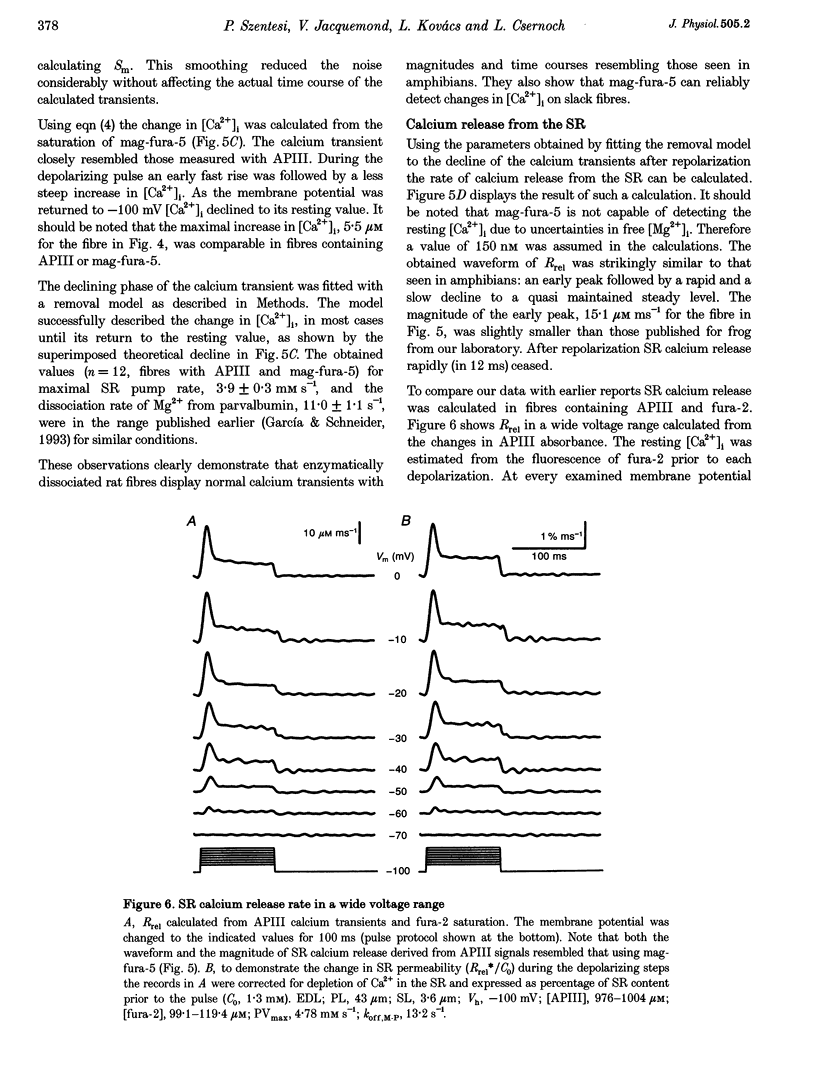
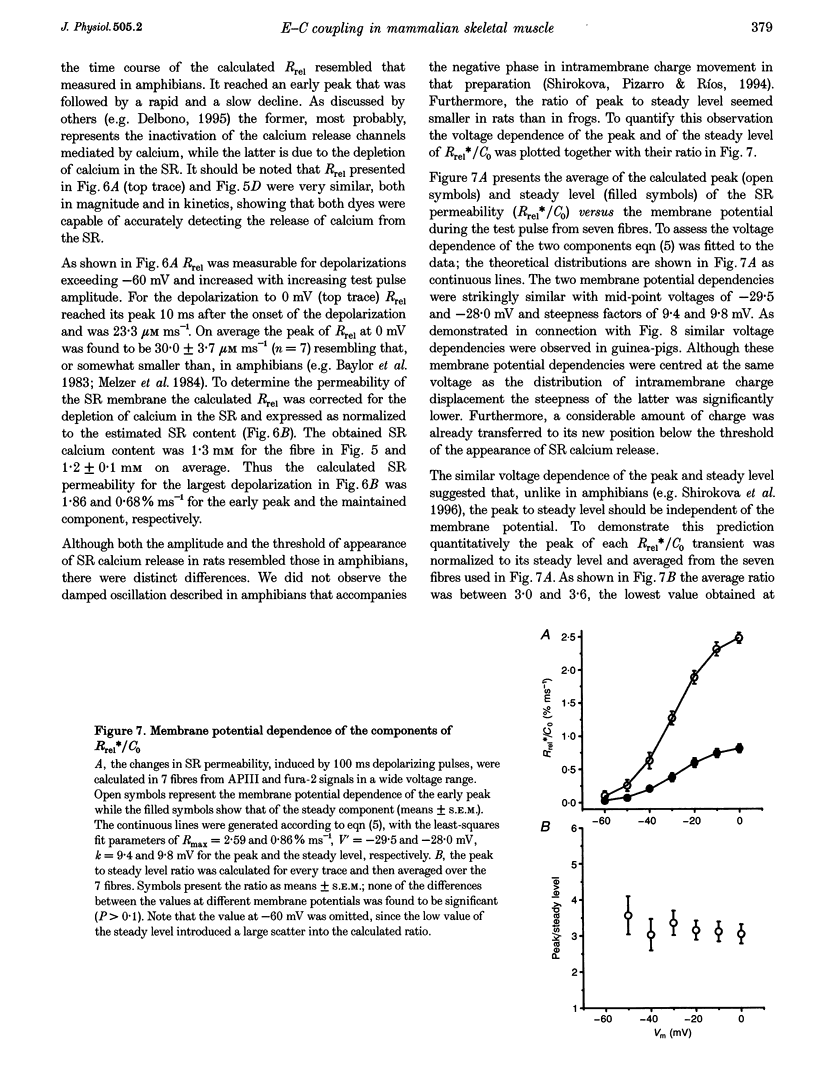
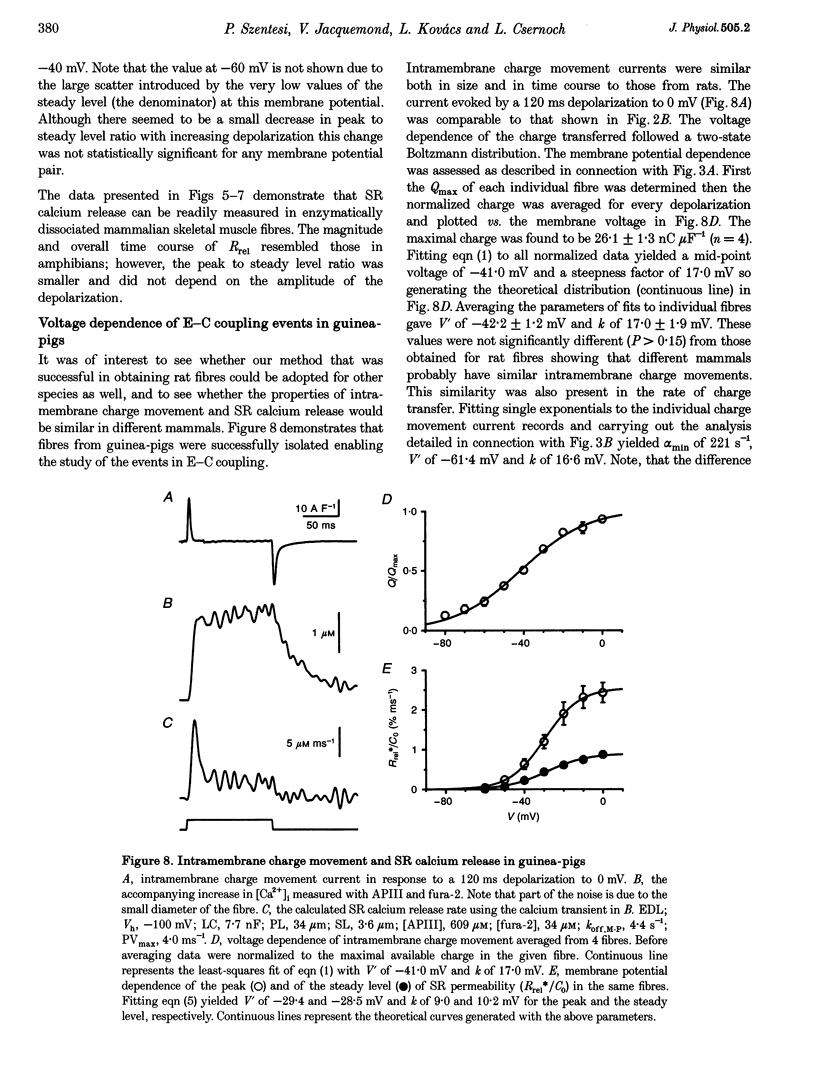
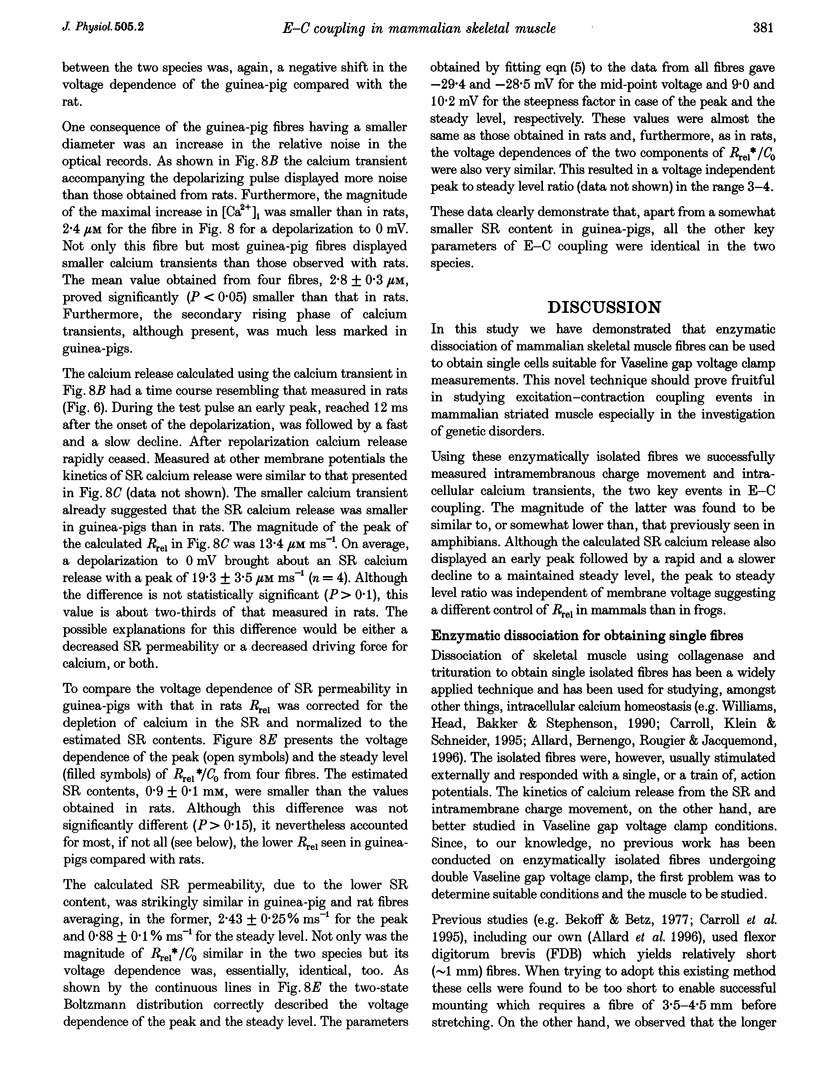
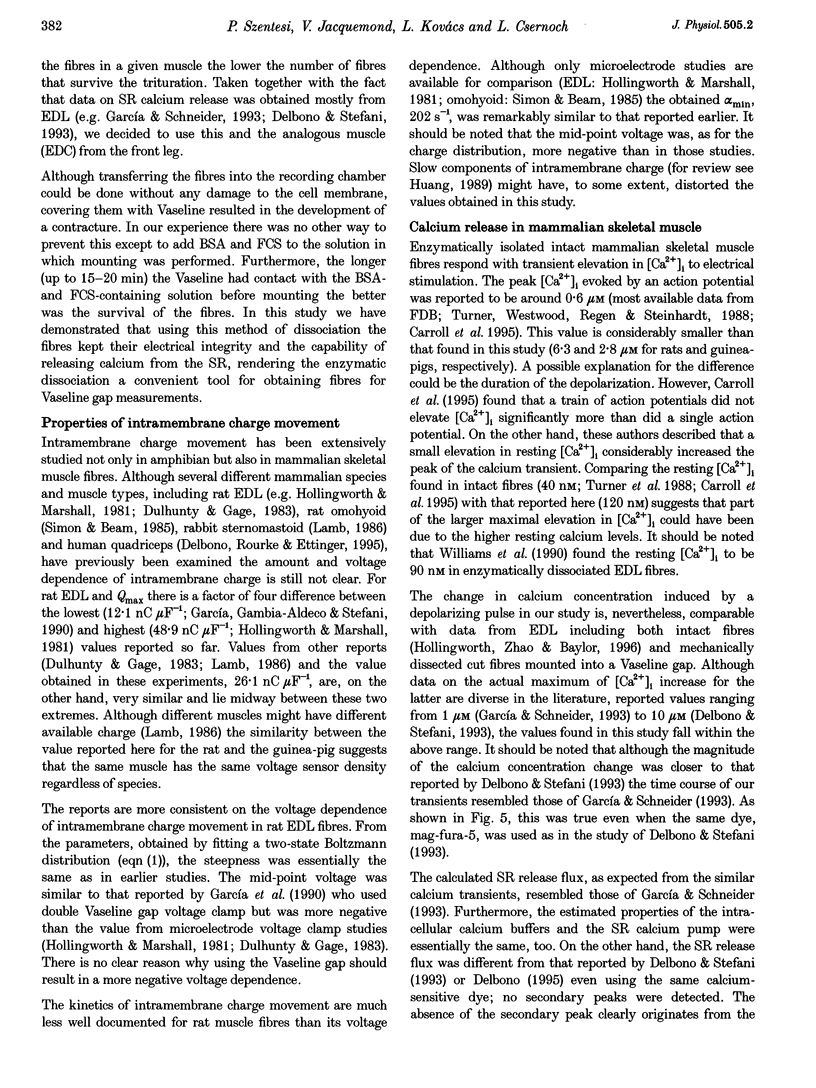
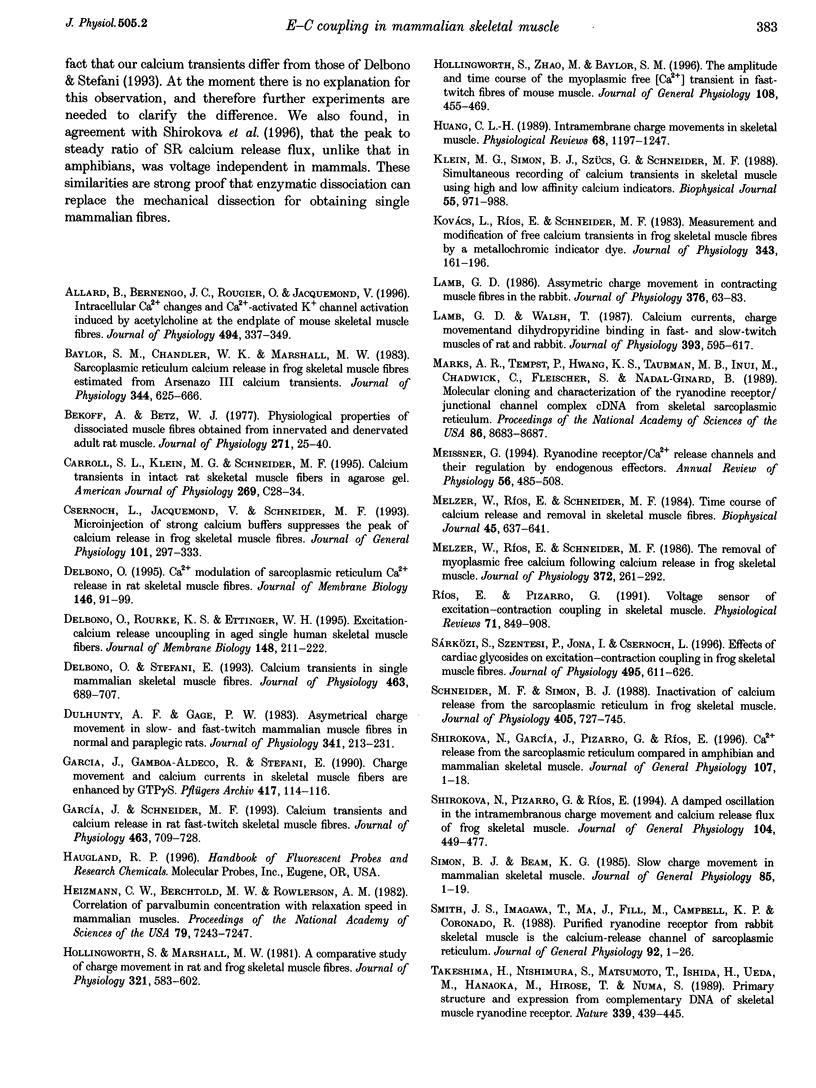
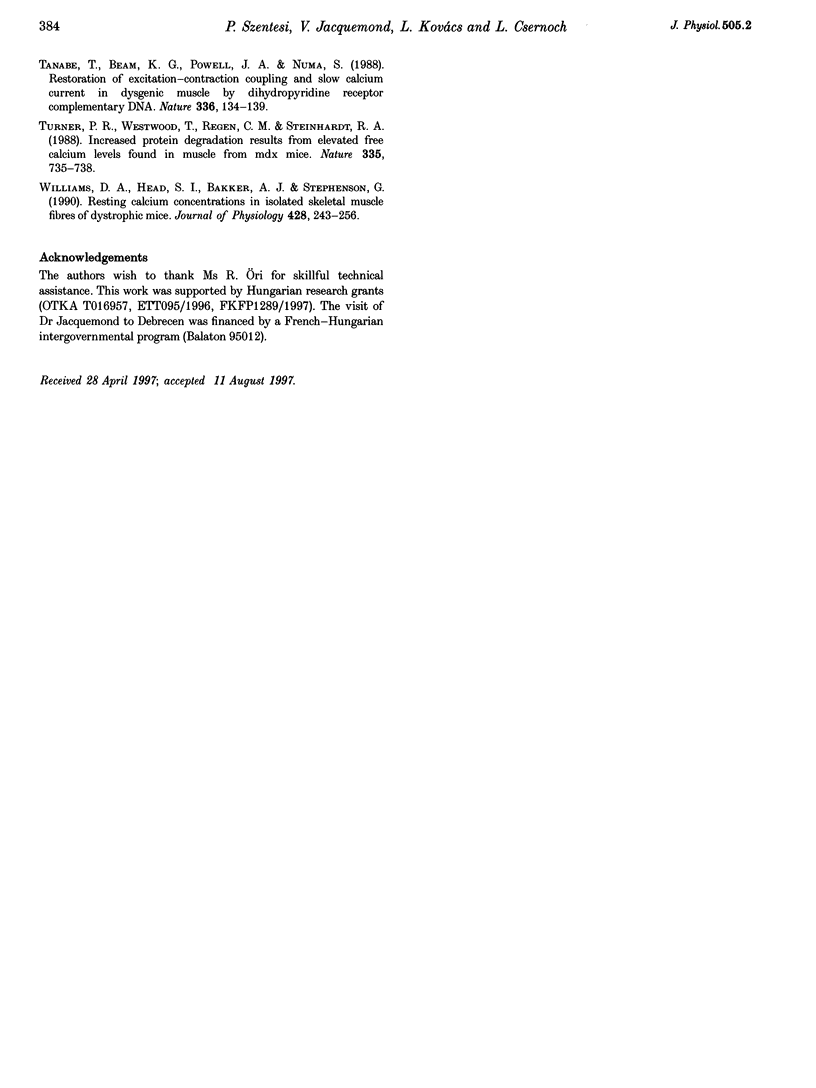
Selected References
These references are in PubMed. This may not be the complete list of references from this article.
- Allard B., Bernengo J. C., Rougier O., Jacquemond V. Intracellular Ca2+ changes and Ca2+-activated K+ channel activation induced by acetylcholine at the endplate of mouse skeletal muscle fibres. J Physiol. 1996 Jul 15;494(Pt 2):337–349. doi: 10.1113/jphysiol.1996.sp021496. [DOI] [PMC free article] [PubMed] [Google Scholar]
- Baylor S. M., Chandler W. K., Marshall M. W. Sarcoplasmic reticulum calcium release in frog skeletal muscle fibres estimated from Arsenazo III calcium transients. J Physiol. 1983 Nov;344:625–666. doi: 10.1113/jphysiol.1983.sp014959. [DOI] [PMC free article] [PubMed] [Google Scholar]
- Bekoff A., Betz W. J. Physiological properties of dissociated muscle fibres obtained from innervated and denervated adult rat muscle. J Physiol. 1977 Sep;271(1):25–40. doi: 10.1113/jphysiol.1977.sp011988. [DOI] [PMC free article] [PubMed] [Google Scholar]
- Carroll S. L., Klein M. G., Schneider M. F. Calcium transients in intact rat skeletal muscle fibers in agarose gel. Am J Physiol. 1995 Jul;269(1 Pt 1):C28–C34. doi: 10.1152/ajpcell.1995.269.1.C28. [DOI] [PubMed] [Google Scholar]
- Csernoch L., Jacquemond V., Schneider M. F. Microinjection of strong calcium buffers suppresses the peak of calcium release during depolarization in frog skeletal muscle fibers. J Gen Physiol. 1993 Feb;101(2):297–333. doi: 10.1085/jgp.101.2.297. [DOI] [PMC free article] [PubMed] [Google Scholar]
- Delbono O. Ca2+ modulation of sarcoplasmic reticulum Ca2+ release in rat skeletal muscle fibers. J Membr Biol. 1995 Jul;146(1):91–99. [PubMed] [Google Scholar]
- Delbono O., O'Rourke K. S., Ettinger W. H. Excitation-calcium release uncoupling in aged single human skeletal muscle fibers. J Membr Biol. 1995 Dec;148(3):211–222. doi: 10.1007/BF00235039. [DOI] [PubMed] [Google Scholar]
- Delbono O., Stefani E. Calcium transients in single mammalian skeletal muscle fibres. J Physiol. 1993 Apr;463:689–707. doi: 10.1113/jphysiol.1993.sp019617. [DOI] [PMC free article] [PubMed] [Google Scholar]
- Dulhunty A. F., Gage P. W. Asymmetrical charge movement in slow- and fast-twitch mammalian muscle fibres in normal and paraplegic rats. J Physiol. 1983 Aug;341:213–231. doi: 10.1113/jphysiol.1983.sp014802. [DOI] [PMC free article] [PubMed] [Google Scholar]
- Garcia J., Schneider M. F. Calcium transients and calcium release in rat fast-twitch skeletal muscle fibres. J Physiol. 1993 Apr;463:709–728. doi: 10.1113/jphysiol.1993.sp019618. [DOI] [PMC free article] [PubMed] [Google Scholar]
- García J., Gamboa-Aldeco R., Stefani E. Charge movement and calcium currents in skeletal muscle fibers are enhanced by GTP gamma S. Pflugers Arch. 1990 Sep;417(1):114–116. doi: 10.1007/BF00370779. [DOI] [PubMed] [Google Scholar]
- Heizmann C. W., Berchtold M. W., Rowlerson A. M. Correlation of parvalbumin concentration with relaxation speed in mammalian muscles. Proc Natl Acad Sci U S A. 1982 Dec;79(23):7243–7247. doi: 10.1073/pnas.79.23.7243. [DOI] [PMC free article] [PubMed] [Google Scholar]
- Hollingworth S., Marshall M. W. A comparative study of charge movement in rat and frog skeletal muscle fibres. J Physiol. 1981 Dec;321:583–602. doi: 10.1113/jphysiol.1981.sp014004. [DOI] [PMC free article] [PubMed] [Google Scholar]
- Hollingworth S., Zhao M., Baylor S. M. The amplitude and time course of the myoplasmic free [Ca2+] transient in fast-twitch fibers of mouse muscle. J Gen Physiol. 1996 Nov;108(5):455–469. doi: 10.1085/jgp.108.5.455. [DOI] [PMC free article] [PubMed] [Google Scholar]
- Huang C. L. Intramembrane charge movements in skeletal muscle. Physiol Rev. 1988 Oct;68(4):1197–1147. doi: 10.1152/physrev.1988.68.4.1197. [DOI] [PubMed] [Google Scholar]
- Klein M. G., Simon B. J., Szucs G., Schneider M. F. Simultaneous recording of calcium transients in skeletal muscle using high- and low-affinity calcium indicators. Biophys J. 1988 Jun;53(6):971–988. doi: 10.1016/S0006-3495(88)83178-3. [DOI] [PMC free article] [PubMed] [Google Scholar]
- Kovacs L., Rios E., Schneider M. F. Measurement and modification of free calcium transients in frog skeletal muscle fibres by a metallochromic indicator dye. J Physiol. 1983 Oct;343:161–196. doi: 10.1113/jphysiol.1983.sp014887. [DOI] [PMC free article] [PubMed] [Google Scholar]
- Lamb G. D. Asymmetric charge movement in contracting muscle fibres in the rabbit. J Physiol. 1986 Jul;376:63–83. doi: 10.1113/jphysiol.1986.sp016142. [DOI] [PMC free article] [PubMed] [Google Scholar]
- Lamb G. D., Walsh T. Calcium currents, charge movement and dihydropyridine binding in fast- and slow-twitch muscles of rat and rabbit. J Physiol. 1987 Dec;393:595–617. doi: 10.1113/jphysiol.1987.sp016843. [DOI] [PMC free article] [PubMed] [Google Scholar]
- Marks A. R., Tempst P., Hwang K. S., Taubman M. B., Inui M., Chadwick C., Fleischer S., Nadal-Ginard B. Molecular cloning and characterization of the ryanodine receptor/junctional channel complex cDNA from skeletal muscle sarcoplasmic reticulum. Proc Natl Acad Sci U S A. 1989 Nov;86(22):8683–8687. doi: 10.1073/pnas.86.22.8683. [DOI] [PMC free article] [PubMed] [Google Scholar]
- Meissner G. Ryanodine receptor/Ca2+ release channels and their regulation by endogenous effectors. Annu Rev Physiol. 1994;56:485–508. doi: 10.1146/annurev.ph.56.030194.002413. [DOI] [PubMed] [Google Scholar]
- Melzer W., Rios E., Schneider M. F. Time course of calcium release and removal in skeletal muscle fibers. Biophys J. 1984 Mar;45(3):637–641. doi: 10.1016/S0006-3495(84)84203-4. [DOI] [PMC free article] [PubMed] [Google Scholar]
- Melzer W., Ríos E., Schneider M. F. The removal of myoplasmic free calcium following calcium release in frog skeletal muscle. J Physiol. 1986 Mar;372:261–292. doi: 10.1113/jphysiol.1986.sp016008. [DOI] [PMC free article] [PubMed] [Google Scholar]
- Ríos E., Pizarro G. Voltage sensor of excitation-contraction coupling in skeletal muscle. Physiol Rev. 1991 Jul;71(3):849–908. doi: 10.1152/physrev.1991.71.3.849. [DOI] [PubMed] [Google Scholar]
- Schneider M. F., Simon B. J. Inactivation of calcium release from the sarcoplasmic reticulum in frog skeletal muscle. J Physiol. 1988 Nov;405:727–745. doi: 10.1113/jphysiol.1988.sp017358. [DOI] [PMC free article] [PubMed] [Google Scholar]
- Shirokova N., García J., Pizarro G., Ríos E. Ca2+ release from the sarcoplasmic reticulum compared in amphibian and mammalian skeletal muscle. J Gen Physiol. 1996 Jan;107(1):1–18. doi: 10.1085/jgp.107.1.1. [DOI] [PMC free article] [PubMed] [Google Scholar]
- Shirokova N., Pizarro G., Ríos E. A damped oscillation in the intramembranous charge movement and calcium release flux of frog skeletal muscle fibers. J Gen Physiol. 1994 Sep;104(3):449–476. doi: 10.1085/jgp.104.3.449. [DOI] [PMC free article] [PubMed] [Google Scholar]
- Simon B. J., Beam K. G. Slow charge movement in mammalian skeletal muscle. J Gen Physiol. 1985 Jan;85(1):1–19. doi: 10.1085/jgp.85.1.1. [DOI] [PMC free article] [PubMed] [Google Scholar]
- Smith J. S., Imagawa T., Ma J., Fill M., Campbell K. P., Coronado R. Purified ryanodine receptor from rabbit skeletal muscle is the calcium-release channel of sarcoplasmic reticulum. J Gen Physiol. 1988 Jul;92(1):1–26. doi: 10.1085/jgp.92.1.1. [DOI] [PMC free article] [PubMed] [Google Scholar]
- Sárközi S., Szentesi P., Jona I., Csernoch L. Effects of cardiac glycosides on excitation-contraction coupling in frog skeletal muscle fibres. J Physiol. 1996 Sep 15;495(Pt 3):611–626. doi: 10.1113/jphysiol.1996.sp021620. [DOI] [PMC free article] [PubMed] [Google Scholar]
- Takeshima H., Nishimura S., Matsumoto T., Ishida H., Kangawa K., Minamino N., Matsuo H., Ueda M., Hanaoka M., Hirose T. Primary structure and expression from complementary DNA of skeletal muscle ryanodine receptor. Nature. 1989 Jun 8;339(6224):439–445. doi: 10.1038/339439a0. [DOI] [PubMed] [Google Scholar]
- Tanabe T., Beam K. G., Powell J. A., Numa S. Restoration of excitation-contraction coupling and slow calcium current in dysgenic muscle by dihydropyridine receptor complementary DNA. Nature. 1988 Nov 10;336(6195):134–139. doi: 10.1038/336134a0. [DOI] [PubMed] [Google Scholar]
- Turner P. R., Westwood T., Regen C. M., Steinhardt R. A. Increased protein degradation results from elevated free calcium levels found in muscle from mdx mice. Nature. 1988 Oct 20;335(6192):735–738. doi: 10.1038/335735a0. [DOI] [PubMed] [Google Scholar]
- Williams D. A., Head S. I., Bakker A. J., Stephenson D. G. Resting calcium concentrations in isolated skeletal muscle fibres of dystrophic mice. J Physiol. 1990 Sep;428:243–256. doi: 10.1113/jphysiol.1990.sp018210. [DOI] [PMC free article] [PubMed] [Google Scholar]