Abstract
1. Intracellularly applied heparin was found to cause a novel, use-dependent block of excitation-contraction (E-C) coupling in skinned skeletal muscle fibres of the toad. After one to four depolarizations in the presence of 100 micrograms ml-1 heparin, no further depolarization-induced responses could be elicited, even though addition of caffeine or lowering [Mg2+] could still induce massive Ca2+ release. This effect could not be reversed by extensive wash-out of the heparin (> 15 min). 2. Heparin (100 micrograms ml-1) did not abolish subsequent depolarization-induced responses if applied while the voltage sensors were in either their resting or inactivated states, that is (a) while a fibre remained fully polarized, (b) when a fibre was already chronically depolarized or (c) after a fibre had been depolarized in the presence of D600 (gallopamil) and then repolarized. 3. When a toad fibre was depolarized in heparin, with the associated Ca2+ release blocked by the presence of 10 mM intracellular Mg2+, subsequent E-C coupling was abolished. Heparin did not interrupt E-C coupling when Ca2+ release was triggered in the absence of any depolarization, by either caffeine or low [Mg2+]. Thus, the opening of the Ca2+ release channels was neither necessary nor sufficient for heparin to abolish E-C coupling. 4. Heparin had direct effects on the contractile apparatus in toad fibres, increasing the Ca2+ sensitivity and decreasing the maximum Ca(2+)-activated force. These effects could only be partly reversed by extensive wash-out of heparin. 5. At 100 micrograms ml-1, both low molecular weight heparin and pentosanpolysulphate, another highly sulphated polysaccharide, were less effective than heparin in blocking the depolarization-induced response and in changing the properties of the contractile apparatus, and these effects could be substantially reversed by wash-out. Two other polyanions, de-N-sulphated heparin (100 micrograms ml-1), which lacked N-sulphate groups, and polyglutamate (500 micrograms ml-1), had no measurable effect on either E-C coupling or the contractile apparatus. 6. In skinned fibres of the extensor digitorum longus muscle of the rat, 100 micrograms ml-1 heparin had little or no effect on E-C coupling and on the Ca2+ sensitivity of the contractile apparatus, but caused a larger reduction of the maximum Ca(2+)-activated force than in skinned fibres of the toad. 7. These results indicate that heparin blocks E-C coupling in toad muscle if, and only if, it is present when the voltage sensors are activated by depolarization.(ABSTRACT TRUNCATED AT 400 WORDS)
Full text
PDF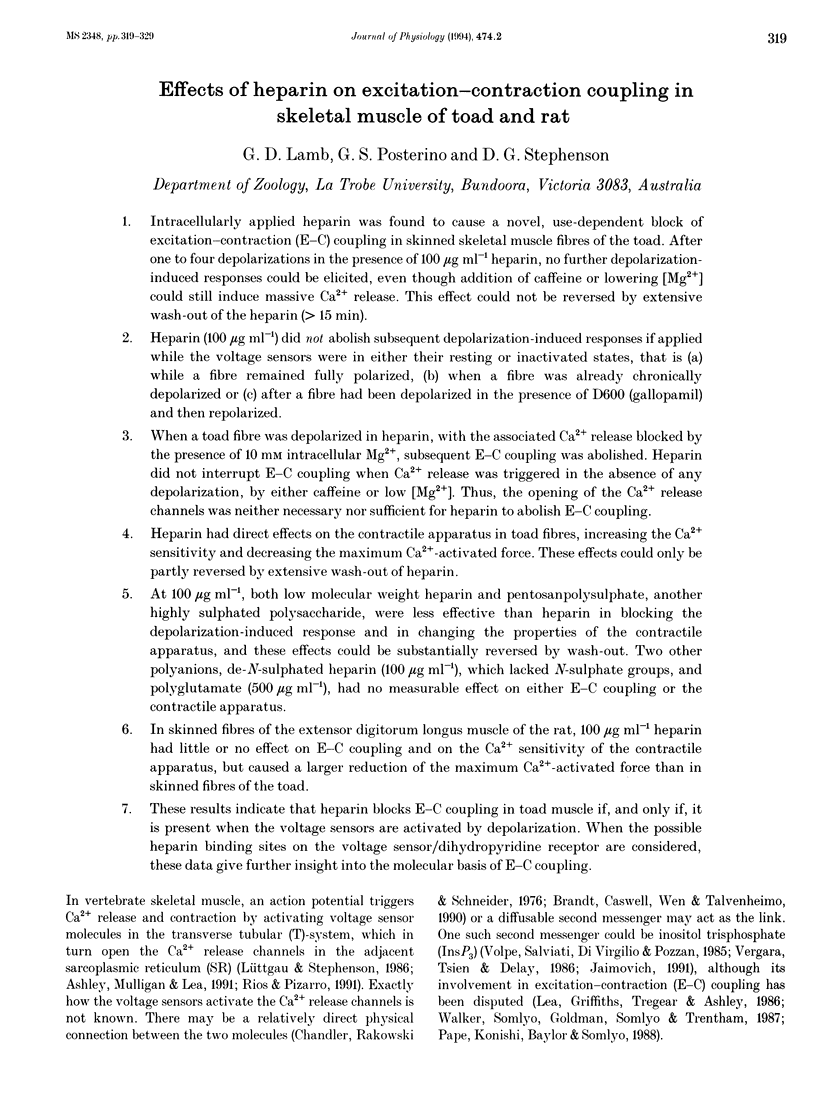
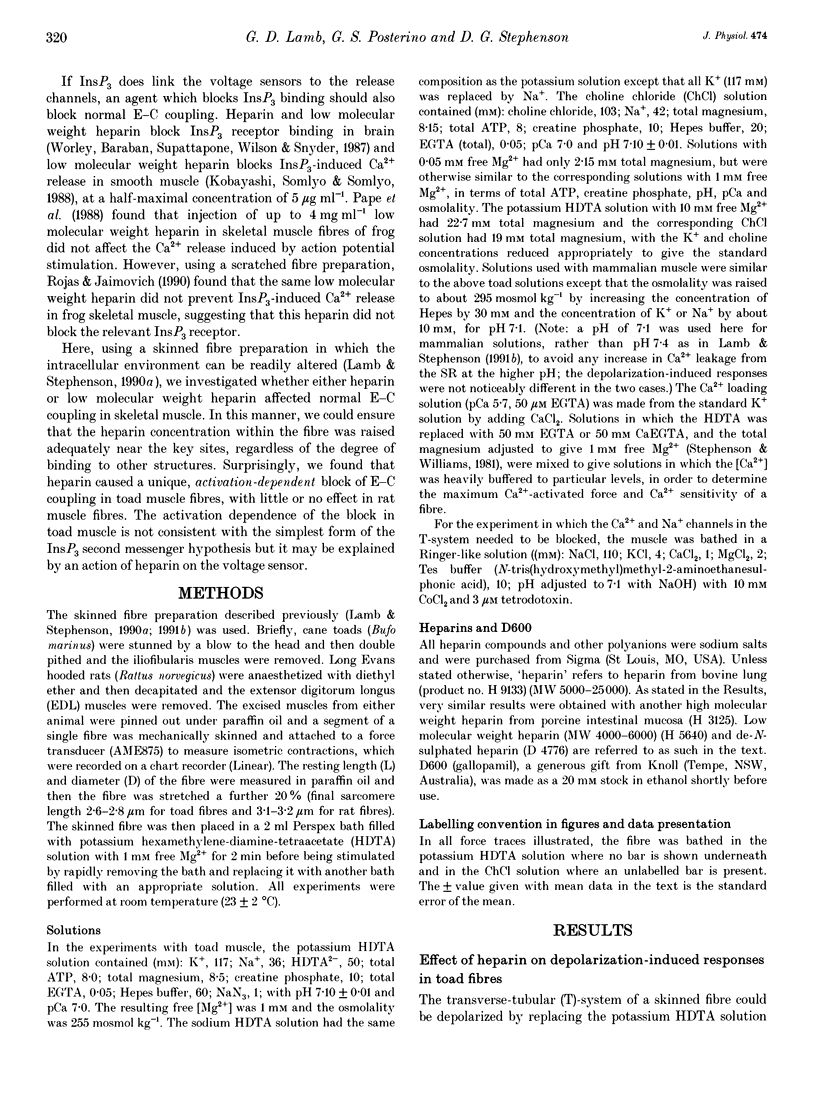
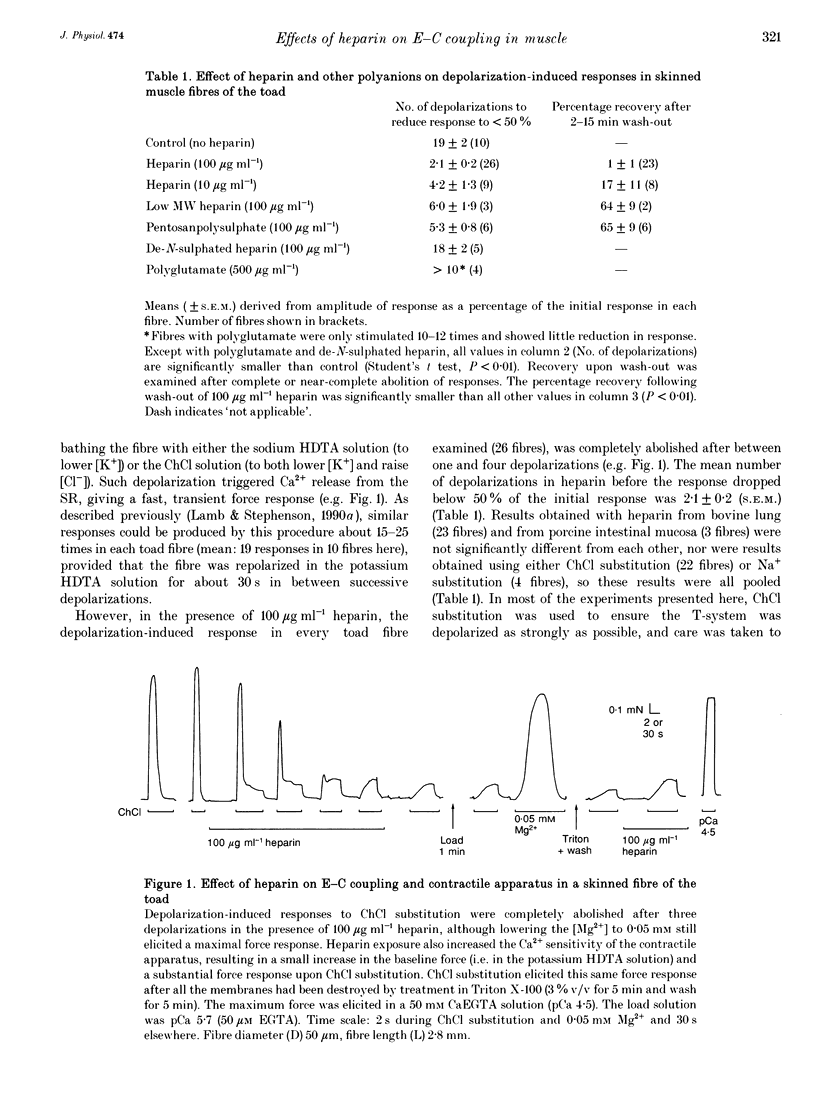
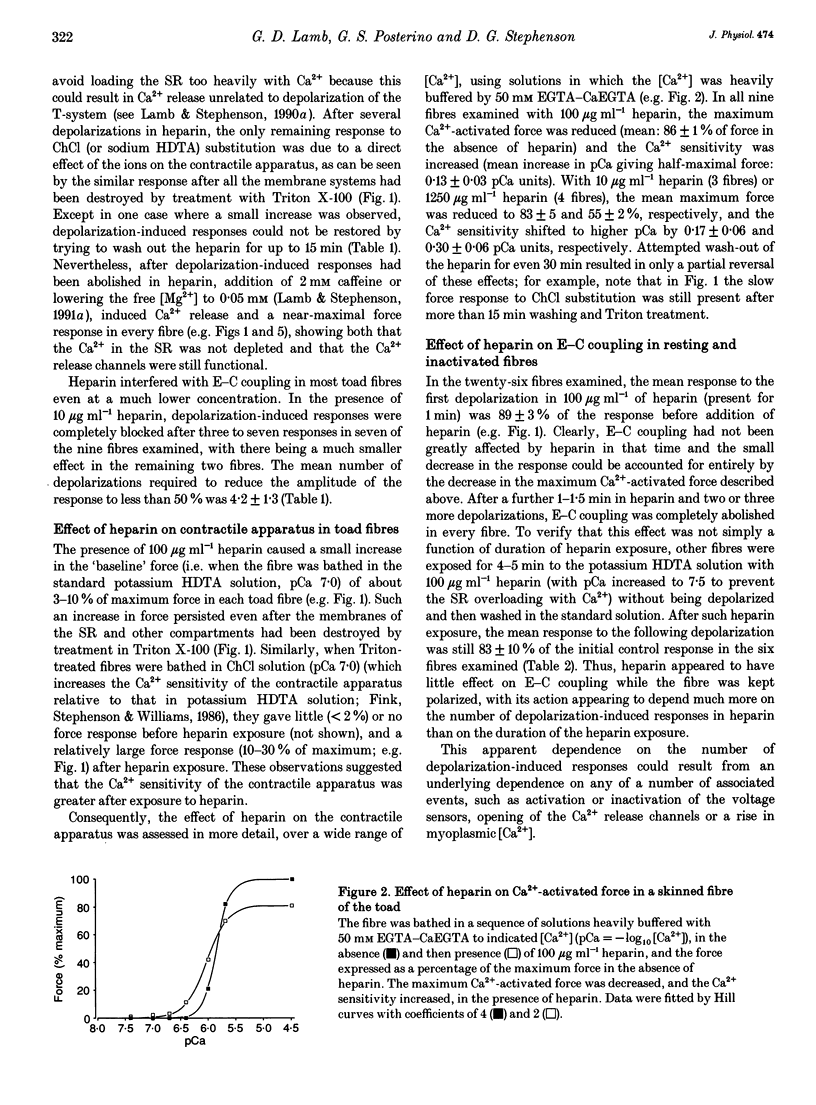
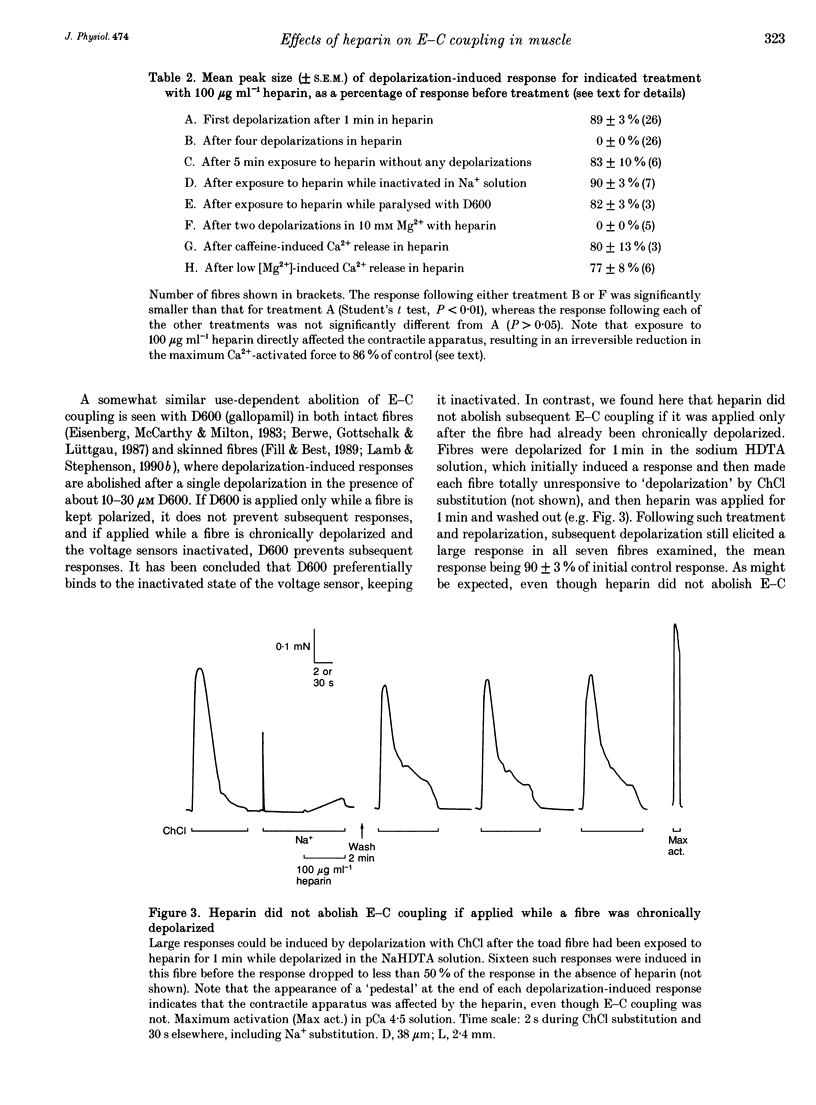
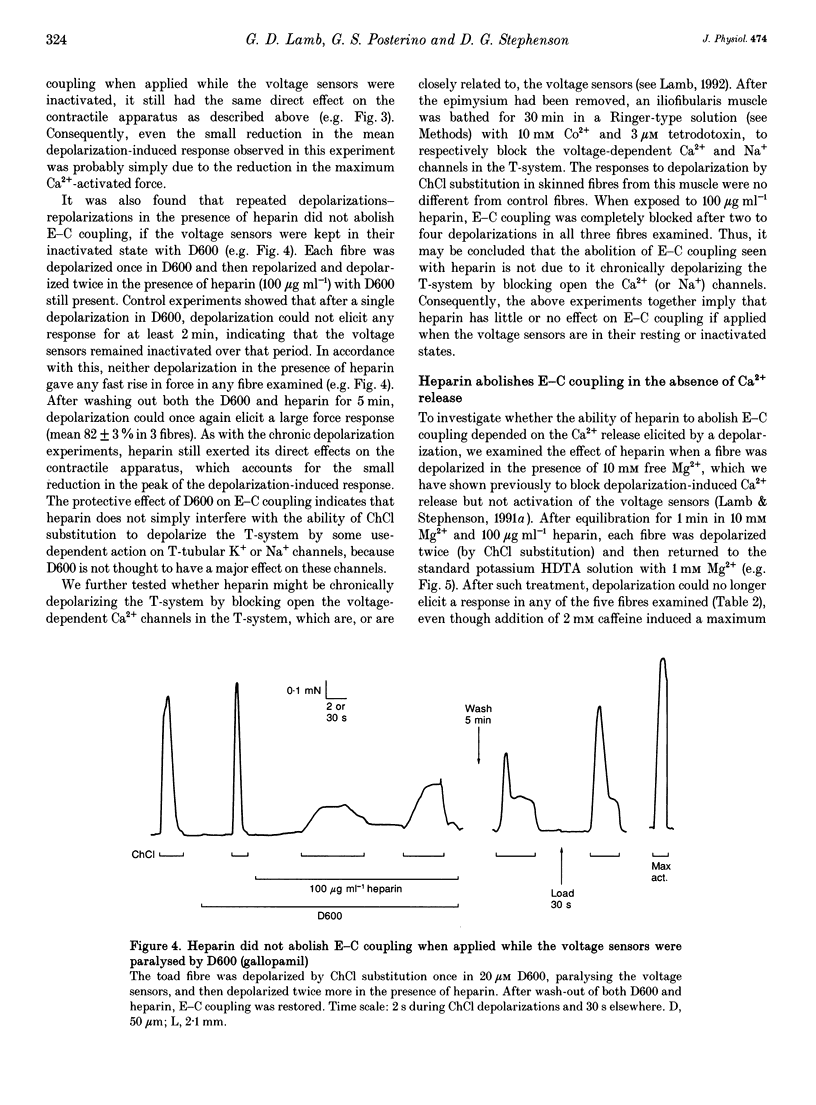
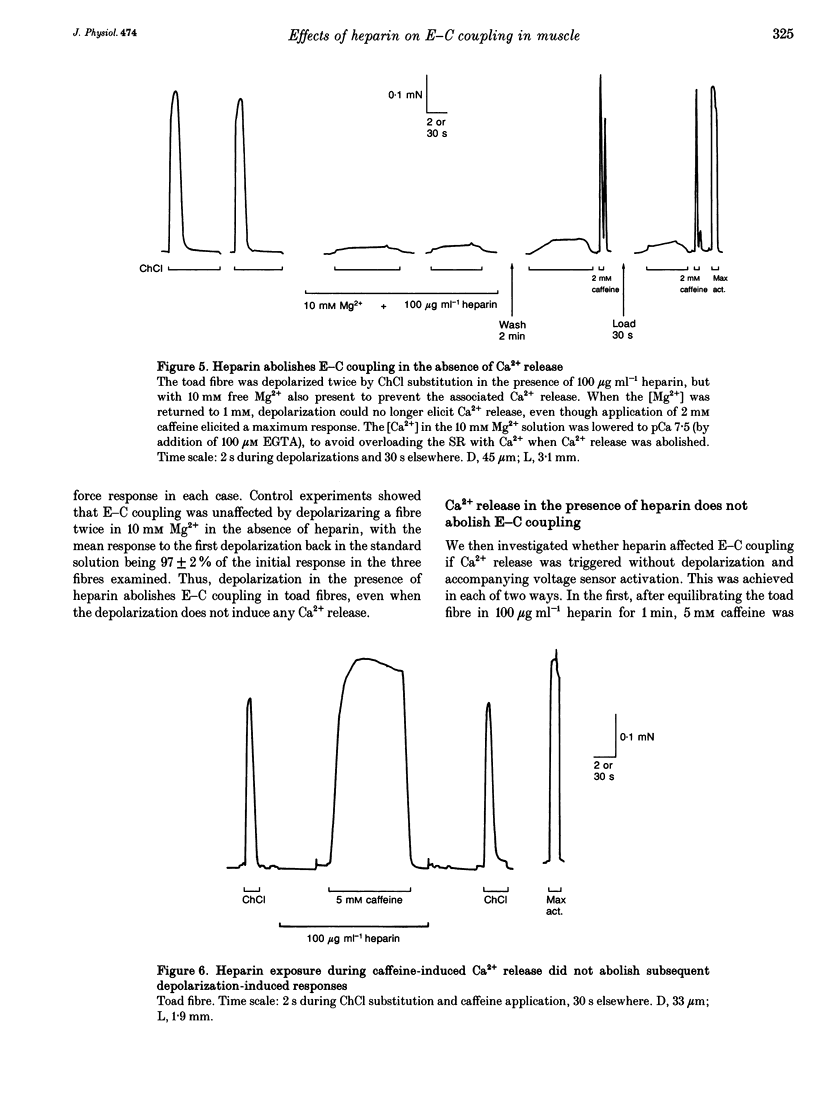
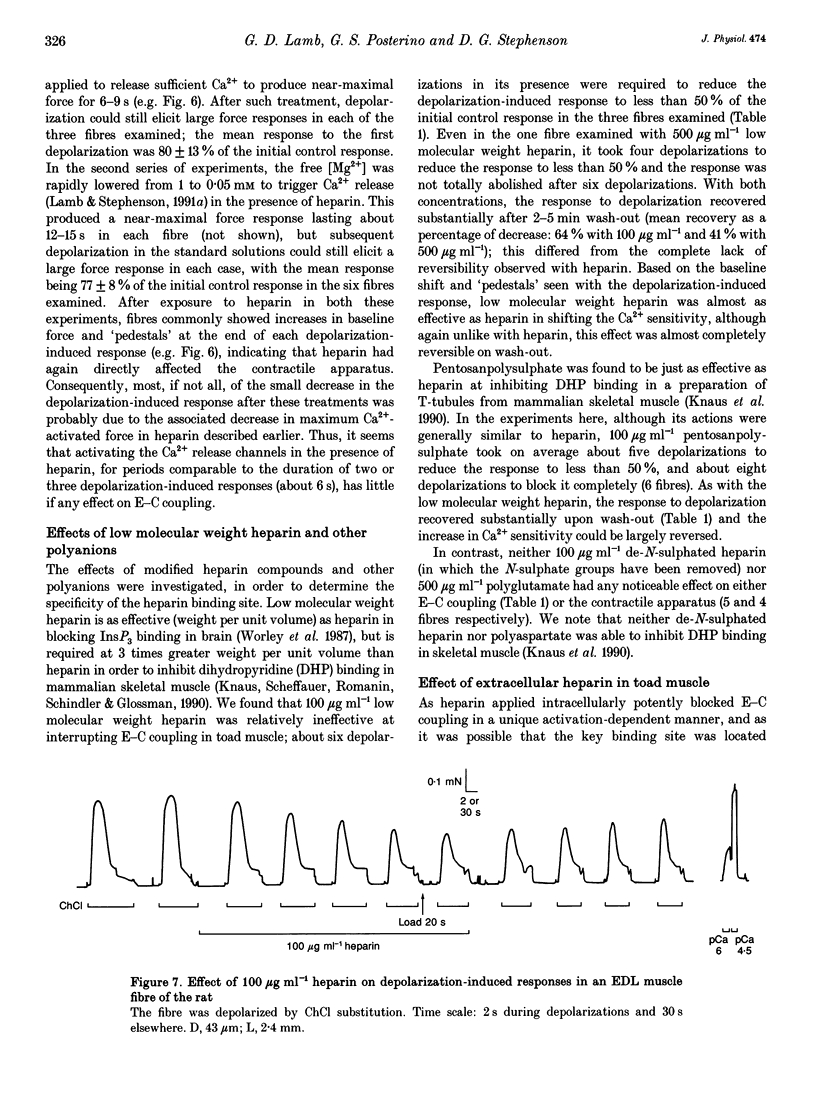
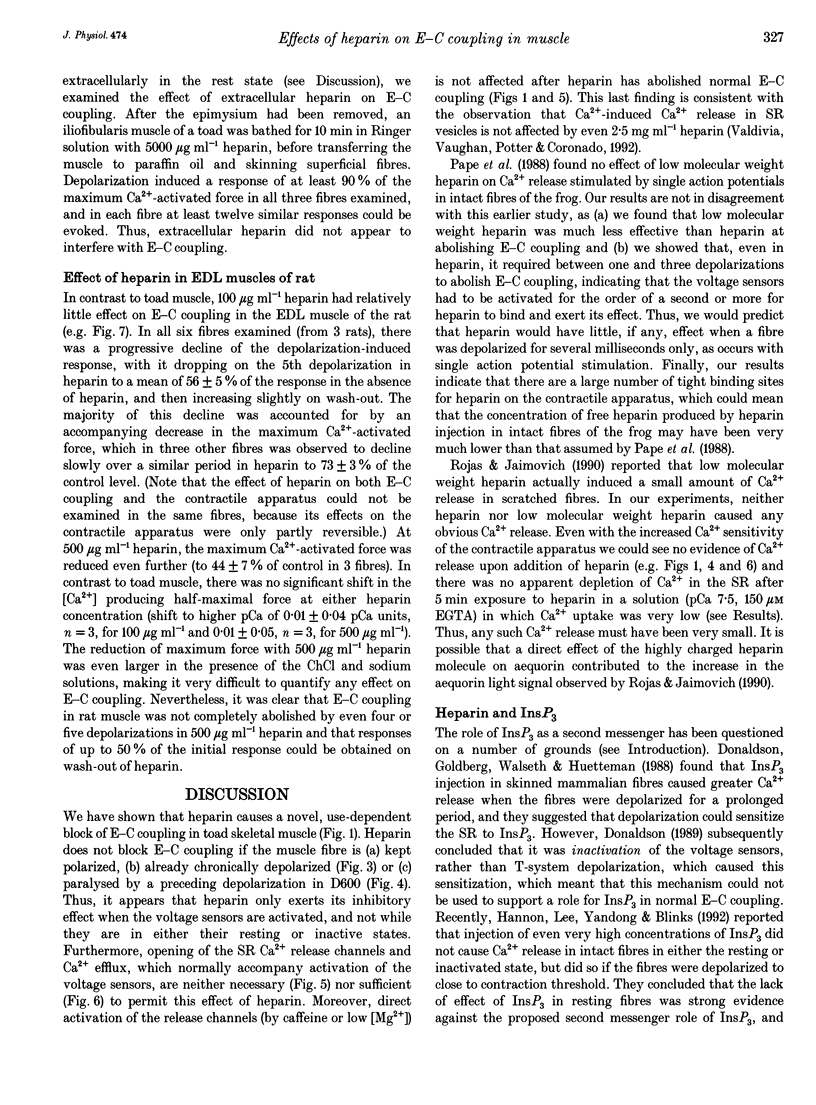
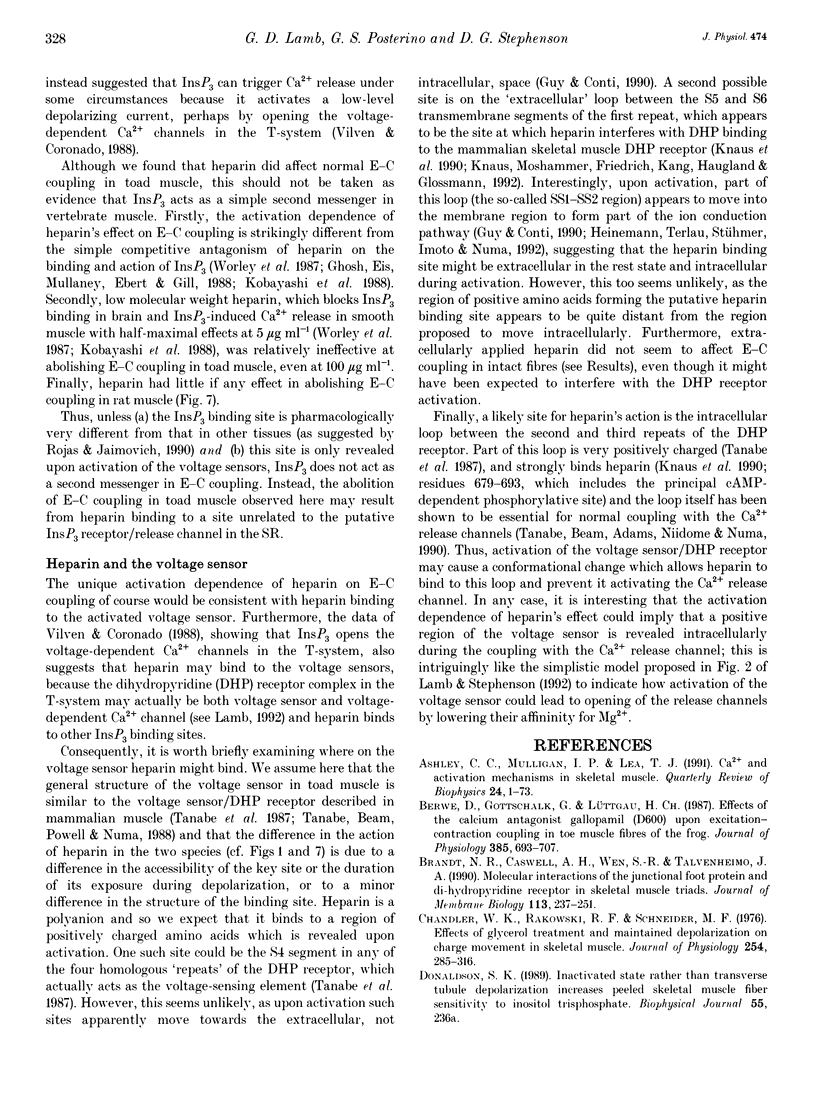
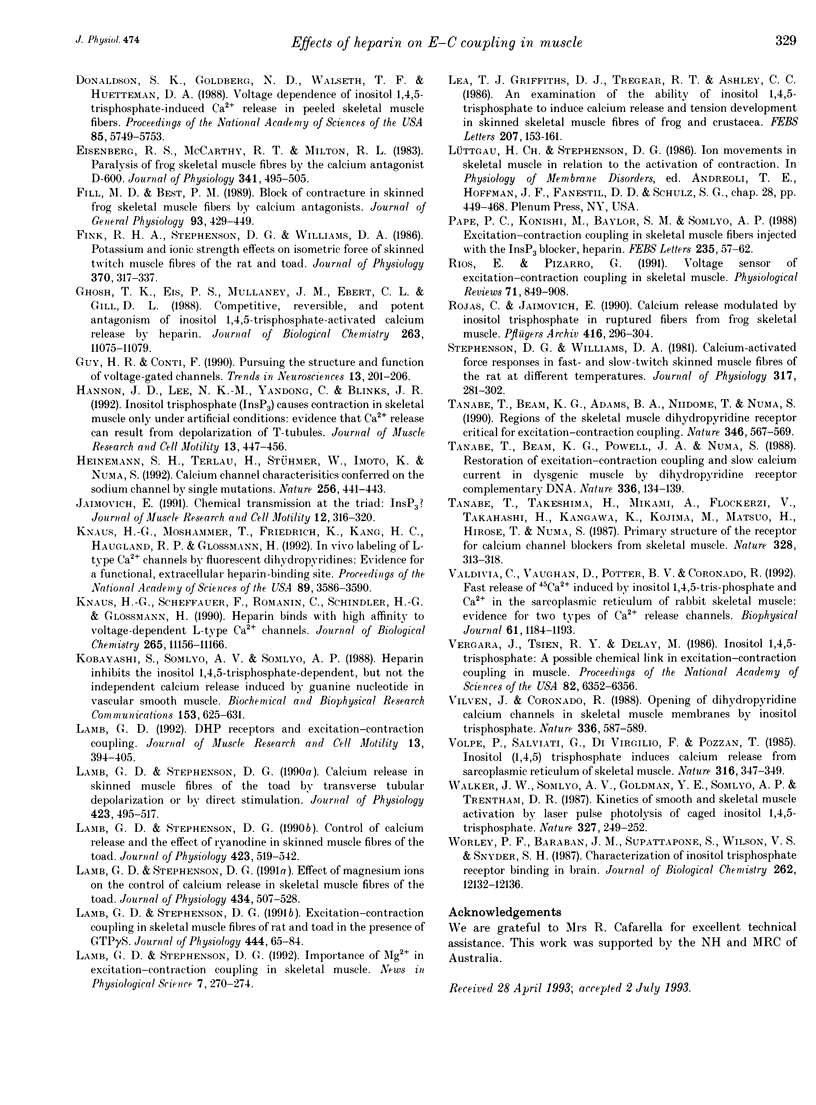
Selected References
These references are in PubMed. This may not be the complete list of references from this article.
- Ashley C. C., Mulligan I. P., Lea T. J. Ca2+ and activation mechanisms in skeletal muscle. Q Rev Biophys. 1991 Feb;24(1):1–73. doi: 10.1017/s0033583500003267. [DOI] [PubMed] [Google Scholar]
- Berwe D., Gottschalk G., Lüttgau H. C. Effects of the calcium antagonist gallopamil (D600) upon excitation-contraction coupling in toe muscle fibres of the frog. J Physiol. 1987 Apr;385:693–707. doi: 10.1113/jphysiol.1987.sp016515. [DOI] [PMC free article] [PubMed] [Google Scholar]
- Brandt N. R., Caswell A. H., Wen S. R., Talvenheimo J. A. Molecular interactions of the junctional foot protein and dihydropyridine receptor in skeletal muscle triads. J Membr Biol. 1990 Feb;113(3):237–251. doi: 10.1007/BF01870075. [DOI] [PubMed] [Google Scholar]
- Chandler W. K., Rakowski R. F., Schneider M. F. Effects of glycerol treatment and maintained depolarization on charge movement in skeletal muscle. J Physiol. 1976 Jan;254(2):285–316. doi: 10.1113/jphysiol.1976.sp011233. [DOI] [PMC free article] [PubMed] [Google Scholar]
- Donaldson S. K., Goldberg N. D., Walseth T. F., Huetteman D. A. Voltage dependence of inositol 1,4,5-trisphosphate-induced Ca2+ release in peeled skeletal muscle fibers. Proc Natl Acad Sci U S A. 1988 Aug;85(15):5749–5753. doi: 10.1073/pnas.85.15.5749. [DOI] [PMC free article] [PubMed] [Google Scholar]
- Eisenberg R. S., McCarthy R. T., Milton R. L. Paralysis of frog skeletal muscle fibres by the calcium antagonist D-600. J Physiol. 1983 Aug;341:495–505. doi: 10.1113/jphysiol.1983.sp014819. [DOI] [PMC free article] [PubMed] [Google Scholar]
- Fill M. D., Best P. M. Block of contracture in skinned frog skeletal muscle fibers by calcium antagonists. J Gen Physiol. 1989 Mar;93(3):429–449. doi: 10.1085/jgp.93.3.429. [DOI] [PMC free article] [PubMed] [Google Scholar]
- Fink R. H., Stephenson D. G., Williams D. A. Potassium and ionic strength effects on the isometric force of skinned twitch muscle fibres of the rat and toad. J Physiol. 1986 Jan;370:317–337. doi: 10.1113/jphysiol.1986.sp015937. [DOI] [PMC free article] [PubMed] [Google Scholar]
- Ghosh T. K., Eis P. S., Mullaney J. M., Ebert C. L., Gill D. L. Competitive, reversible, and potent antagonism of inositol 1,4,5-trisphosphate-activated calcium release by heparin. J Biol Chem. 1988 Aug 15;263(23):11075–11079. [PubMed] [Google Scholar]
- Guy H. R., Conti F. Pursuing the structure and function of voltage-gated channels. Trends Neurosci. 1990 Jun;13(6):201–206. doi: 10.1016/0166-2236(90)90160-c. [DOI] [PubMed] [Google Scholar]
- Hannon J. D., Lee N. K., Yandong C., Blinks J. R. Inositol trisphosphate (InsP3) causes contraction in skeletal muscle only under artificial conditions: evidence that Ca2+ release can result from depolarization of T-tubules. J Muscle Res Cell Motil. 1992 Aug;13(4):447–456. doi: 10.1007/BF01738039. [DOI] [PubMed] [Google Scholar]
- Heinemann S. H., Terlau H., Stühmer W., Imoto K., Numa S. Calcium channel characteristics conferred on the sodium channel by single mutations. Nature. 1992 Apr 2;356(6368):441–443. doi: 10.1038/356441a0. [DOI] [PubMed] [Google Scholar]
- Jaimovich E. Chemical transmission at the triad: InsP3? J Muscle Res Cell Motil. 1991 Aug;12(4):316–320. doi: 10.1007/BF01738586. [DOI] [PubMed] [Google Scholar]
- Knaus H. G., Moshammer T., Friedrich K., Kang H. C., Haugland R. P., Glossman H. In vivo labeling of L-type Ca2+ channels by fluorescent dihydropyridines: evidence for a functional, extracellular heparin-binding site. Proc Natl Acad Sci U S A. 1992 Apr 15;89(8):3586–3590. doi: 10.1073/pnas.89.8.3586. [DOI] [PMC free article] [PubMed] [Google Scholar]
- Knaus H. G., Scheffauer F., Romanin C., Schindler H. G., Glossmann H. Heparin binds with high affinity to voltage-dependent L-type Ca2+ channels. Evidence for an agonistic action. J Biol Chem. 1990 Jul 5;265(19):11156–11166. [PubMed] [Google Scholar]
- Kobayashi S., Somlyo A. V., Somlyo A. P. Heparin inhibits the inositol 1,4,5-trisphosphate-dependent, but not the independent, calcium release induced by guanine nucleotide in vascular smooth muscle. Biochem Biophys Res Commun. 1988 Jun 16;153(2):625–631. doi: 10.1016/s0006-291x(88)81141-0. [DOI] [PubMed] [Google Scholar]
- Lamb G. D., Stephenson D. G. Calcium release in skinned muscle fibres of the toad by transverse tubule depolarization or by direct stimulation. J Physiol. 1990 Apr;423:495–517. doi: 10.1113/jphysiol.1990.sp018036. [DOI] [PMC free article] [PubMed] [Google Scholar]
- Lamb G. D., Stephenson D. G. Control of calcium release and the effect of ryanodine in skinned muscle fibres of the toad. J Physiol. 1990 Apr;423:519–542. doi: 10.1113/jphysiol.1990.sp018037. [DOI] [PMC free article] [PubMed] [Google Scholar]
- Lamb G. D., Stephenson D. G. Effect of Mg2+ on the control of Ca2+ release in skeletal muscle fibres of the toad. J Physiol. 1991 Mar;434:507–528. doi: 10.1113/jphysiol.1991.sp018483. [DOI] [PMC free article] [PubMed] [Google Scholar]
- Lamb G. D., Stephenson D. G. Excitation-contraction coupling in skeletal muscle fibres of rat and toad in the presence of GTP gamma S. J Physiol. 1991 Dec;444:65–84. doi: 10.1113/jphysiol.1991.sp018866. [DOI] [PMC free article] [PubMed] [Google Scholar]
- Lea T. J., Griffiths P. J., Tregear R. T., Ashley C. C. An examination of the ability of inositol 1,4,5-trisphosphate to induce calcium release and tension development in skinned skeletal muscle fibres of frog and crustacea. FEBS Lett. 1986 Oct 20;207(1):153–161. doi: 10.1016/0014-5793(86)80031-x. [DOI] [PubMed] [Google Scholar]
- Pape P. C., Konishi M., Baylor S. M., Somlyo A. P. Excitation-contraction coupling in skeletal muscle fibers injected with the InsP3 blocker, heparin. FEBS Lett. 1988 Aug 1;235(1-2):57–62. doi: 10.1016/0014-5793(88)81233-x. [DOI] [PubMed] [Google Scholar]
- Rojas C., Jaimovich E. Calcium release modulated by inositol trisphosphate in ruptured fibers from frog skeletal muscle. Pflugers Arch. 1990 May;416(3):296–304. doi: 10.1007/BF00392066. [DOI] [PubMed] [Google Scholar]
- Ríos E., Pizarro G. Voltage sensor of excitation-contraction coupling in skeletal muscle. Physiol Rev. 1991 Jul;71(3):849–908. doi: 10.1152/physrev.1991.71.3.849. [DOI] [PubMed] [Google Scholar]
- Stephenson D. G., Williams D. A. Calcium-activated force responses in fast- and slow-twitch skinned muscle fibres of the rat at different temperatures. J Physiol. 1981 Aug;317:281–302. doi: 10.1113/jphysiol.1981.sp013825. [DOI] [PMC free article] [PubMed] [Google Scholar]
- Tanabe T., Beam K. G., Adams B. A., Niidome T., Numa S. Regions of the skeletal muscle dihydropyridine receptor critical for excitation-contraction coupling. Nature. 1990 Aug 9;346(6284):567–569. doi: 10.1038/346567a0. [DOI] [PubMed] [Google Scholar]
- Tanabe T., Beam K. G., Powell J. A., Numa S. Restoration of excitation-contraction coupling and slow calcium current in dysgenic muscle by dihydropyridine receptor complementary DNA. Nature. 1988 Nov 10;336(6195):134–139. doi: 10.1038/336134a0. [DOI] [PubMed] [Google Scholar]
- Tanabe T., Takeshima H., Mikami A., Flockerzi V., Takahashi H., Kangawa K., Kojima M., Matsuo H., Hirose T., Numa S. Primary structure of the receptor for calcium channel blockers from skeletal muscle. Nature. 1987 Jul 23;328(6128):313–318. doi: 10.1038/328313a0. [DOI] [PubMed] [Google Scholar]
- Valdivia C., Vaughan D., Potter B. V., Coronado R. Fast release of 45Ca2+ induced by inositol 1,4,5-trisphosphate and Ca2+ in the sarcoplasmic reticulum of rabbit skeletal muscle: evidence for two types of Ca2+ release channels. Biophys J. 1992 May;61(5):1184–1193. doi: 10.1016/S0006-3495(92)81927-6. [DOI] [PMC free article] [PubMed] [Google Scholar]
- Vergara J., Tsien R. Y., Delay M. Inositol 1,4,5-trisphosphate: a possible chemical link in excitation-contraction coupling in muscle. Proc Natl Acad Sci U S A. 1985 Sep;82(18):6352–6356. doi: 10.1073/pnas.82.18.6352. [DOI] [PMC free article] [PubMed] [Google Scholar]
- Vilven J., Coronado R. Opening of dihydropyridine calcium channels in skeletal muscle membranes by inositol trisphosphate. Nature. 1988 Dec 8;336(6199):587–589. doi: 10.1038/336587a0. [DOI] [PubMed] [Google Scholar]
- Volpe P., Salviati G., Di Virgilio F., Pozzan T. Inositol 1,4,5-trisphosphate induces calcium release from sarcoplasmic reticulum of skeletal muscle. Nature. 1985 Jul 25;316(6026):347–349. doi: 10.1038/316347a0. [DOI] [PubMed] [Google Scholar]
- Walker J. W., Somlyo A. V., Goldman Y. E., Somlyo A. P., Trentham D. R. Kinetics of smooth and skeletal muscle activation by laser pulse photolysis of caged inositol 1,4,5-trisphosphate. Nature. 1987 May 21;327(6119):249–252. doi: 10.1038/327249a0. [DOI] [PubMed] [Google Scholar]
- Worley P. F., Baraban J. M., Supattapone S., Wilson V. S., Snyder S. H. Characterization of inositol trisphosphate receptor binding in brain. Regulation by pH and calcium. J Biol Chem. 1987 Sep 5;262(25):12132–12136. [PubMed] [Google Scholar]