Abstract
1. Voltage-dependent currents of untreated (proliferating) and lipopolysaccharide (LPS)-treated rat microglial cells in culture were recorded using the whole-cell patch-clamp technique. 2. Membrane potentials showed prominent peaks at -35 mV and -70 mV. Membrane potentials of LPS-treated cells alternated between the two values. This may be due to a negative slope region of the I-V relation resulting in two zero current potentials. 3. From a holding potential of -70 mV, hyperpolarizing steps evoked an inwardly rectifying current both in proliferating and in LPS-treated cells, while depolarizing steps below -50 mV evoked an outwardly rectifying current only in LPS-treated microglia. The currents were K+ selective, as indicated by their reversal potential of approximately 0 mV in symmetric K+ concentrations (150 mM both intra- and extracellularly) and the reversal potential of the outward tail currents of approximately -90 mV at a normal extracellular K+ concentration (4.5 mM). 4. The activation of the outward current could be fitted by Hodgkin-Huxley-type n4 kinetics. The time constant of activation depended on voltage. 5. The inactivation of the inward and outward currents could be fitted by a single exponential. The time constant of the inward current inactivation was dependent on voltage, whereas the time constant of the outward current inactivation was virtually independent of voltage, except near the threshold of activation. Recovery of the outward from inactivation was slow and could be fitted by two exponentials. Responses to depolarizing steps were stable at 0.125 Hz, but greatly decreased from the first to the second pulse at 1 Hz. 6. The inactivation of the inward, but not of the outward, current disappeared in a low Na(+)-containing medium (5 mM). The inward current was selectively inhibited by extracellular Cs+ and Ba2+. The outward current was selectively inhibited by Cd2+, 4-aminopyridine and charybdotoxin. Replacement of intracellular K+ by an equimolar concentration of Cs+, and the extracellular application of tetraethylammonium and quinine inhibited both currents. 7. An increase of extracellular Ca2+ from 2 to 20 mM resulted in outwardly rectifying K+ channels activating at more positive potentials. Omission of Ca2+ from the extracellular medium had the opposite effect. When the intracellular free Ca2+ was increased from 0.01 to 1 microM, the outward current amplitudes were depressed. The Ca2+ ionophore A23187 had a similar effect. 8. LPS-treated microglial cells possess inwardly and outwardly rectifying K+ channels. The physiological and pharmacological characteristics of these two channel populations are markedly different.(ABSTRACT TRUNCATED AT 400 WORDS)
Full text
PDF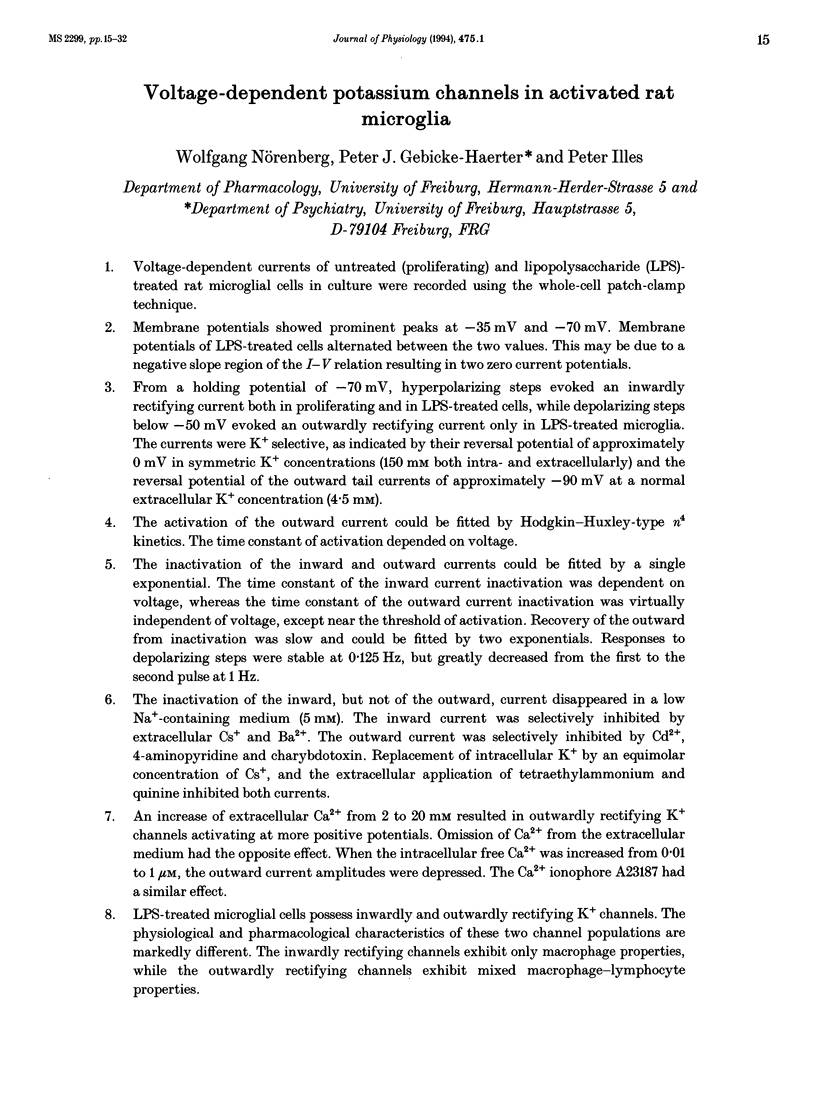
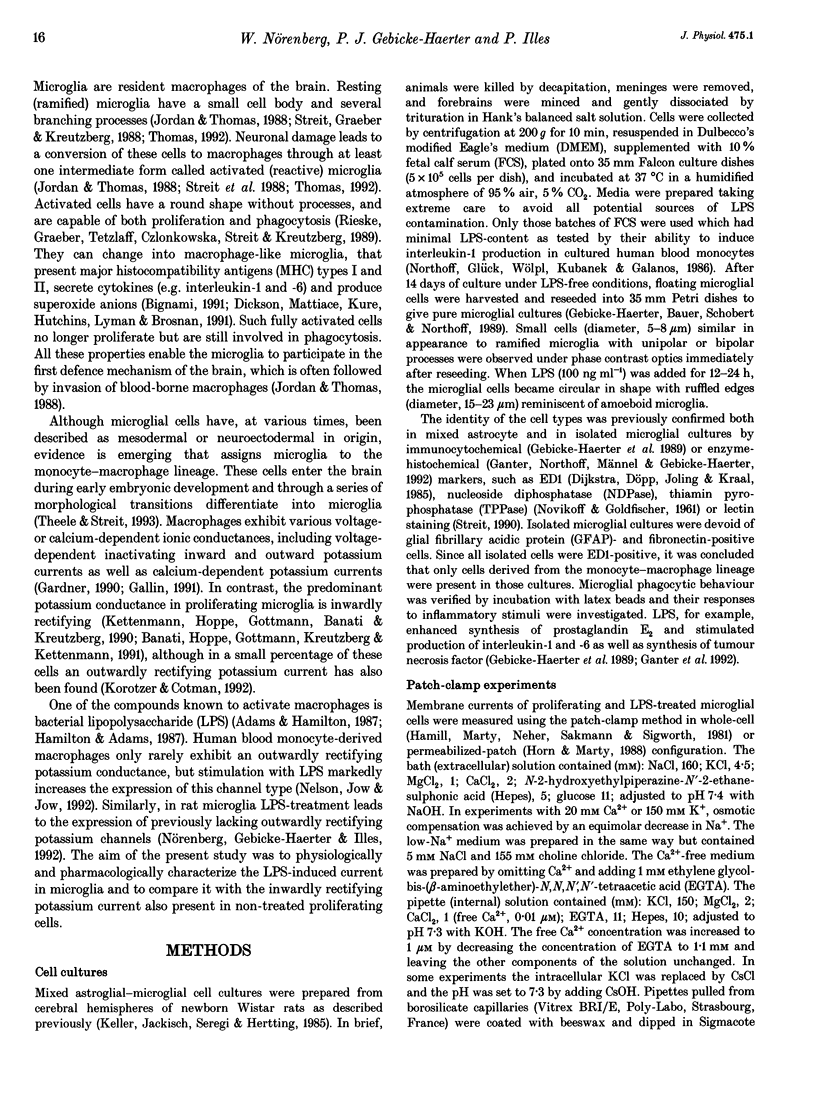
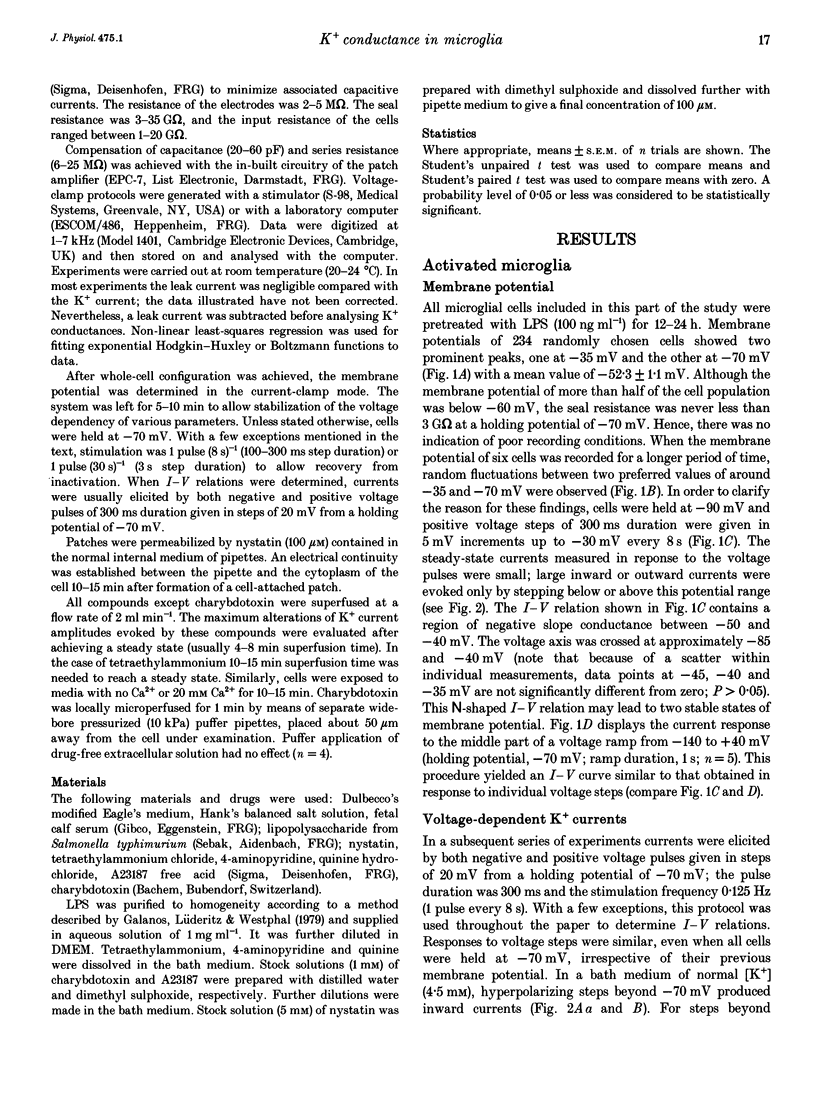
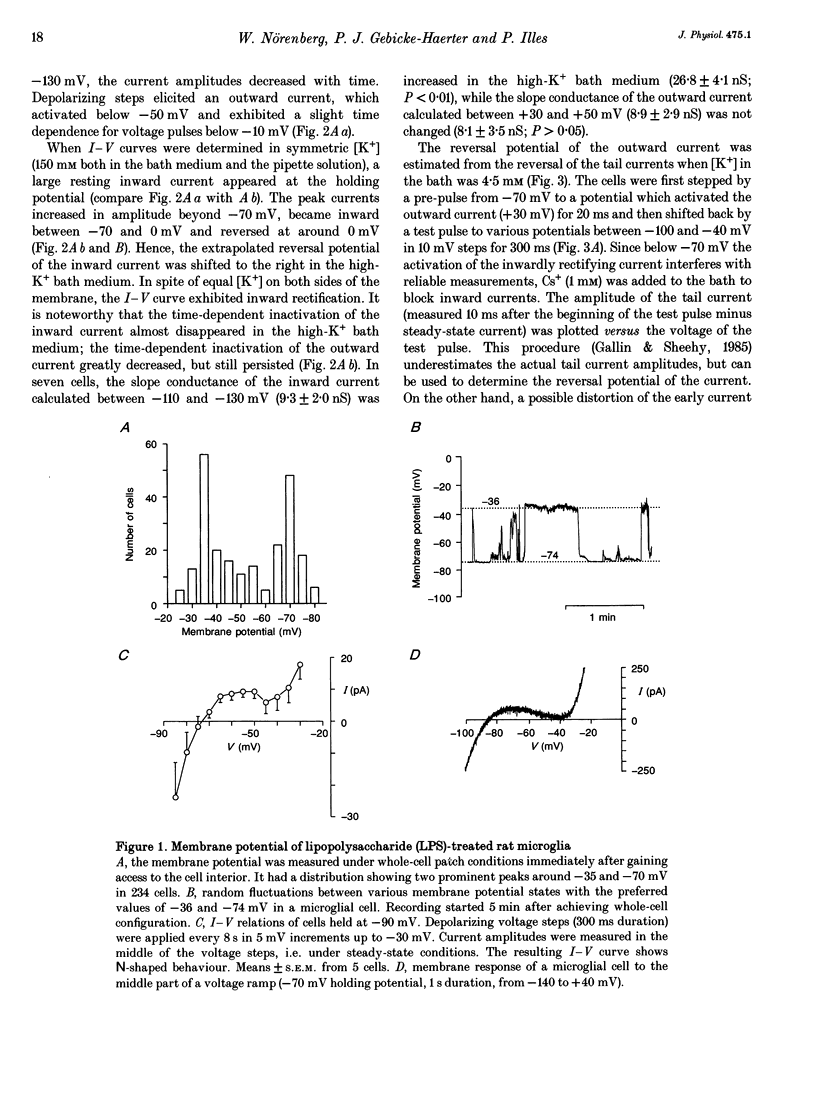
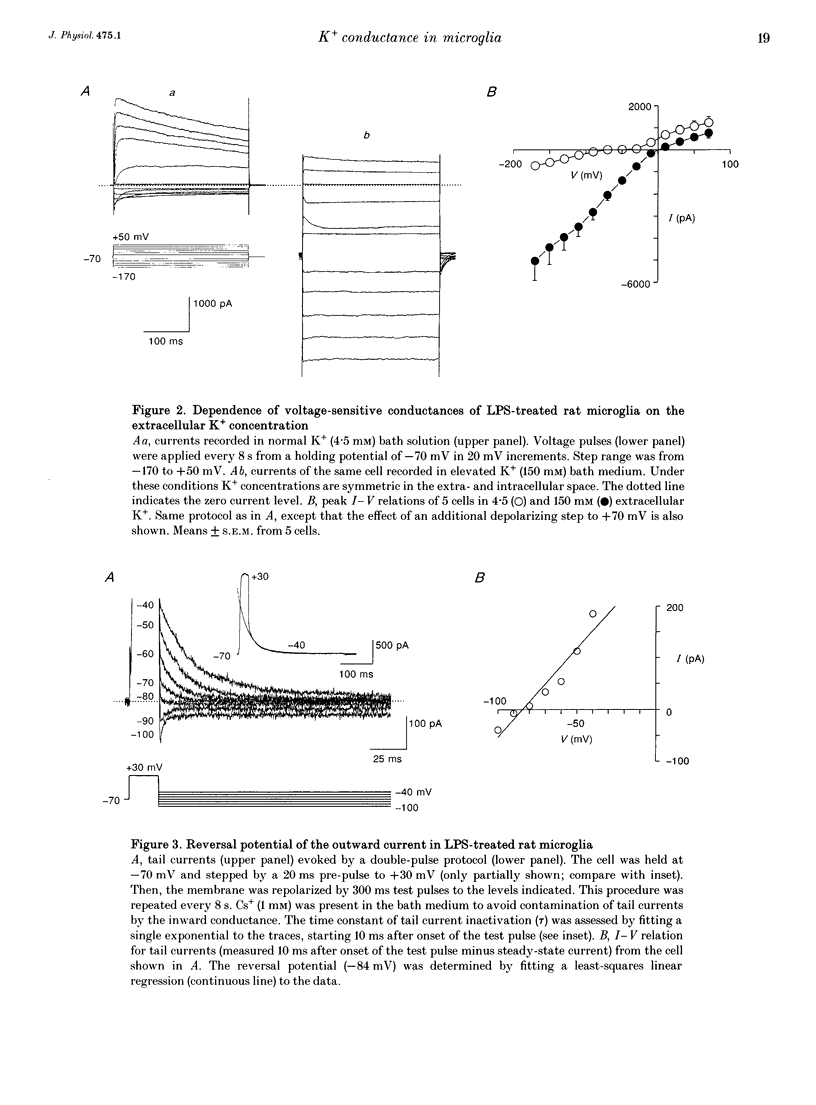
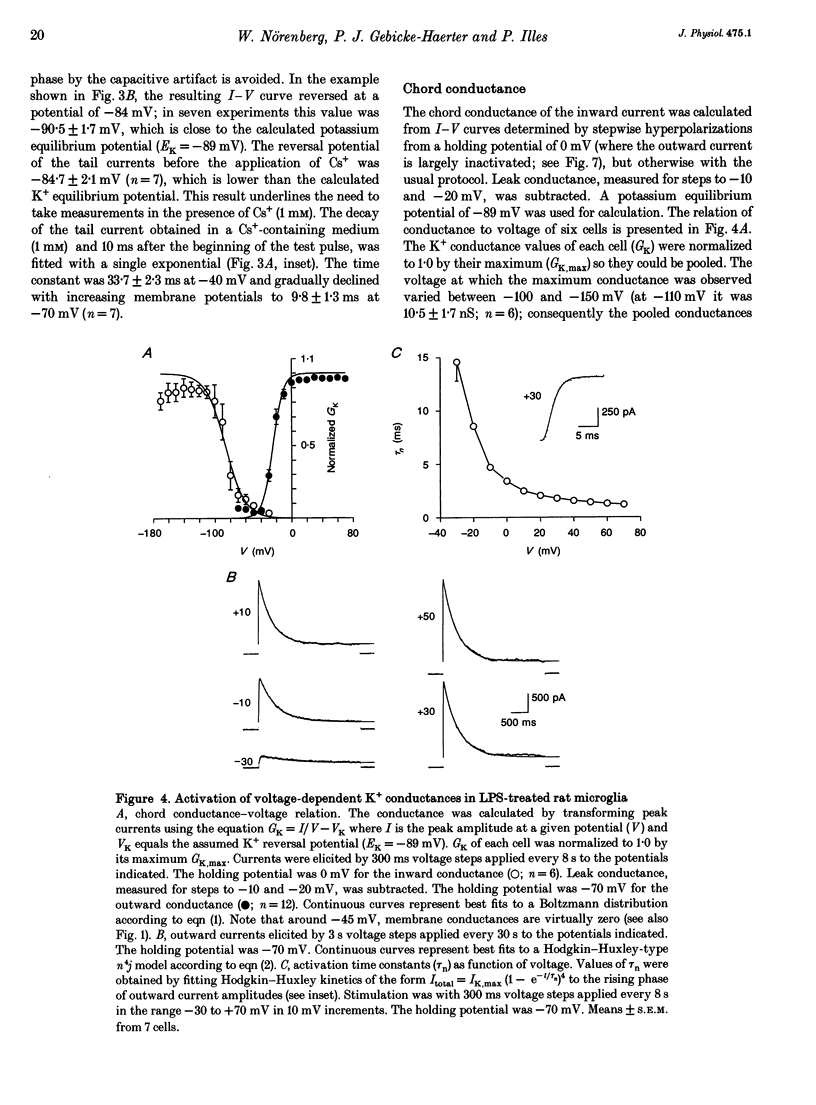
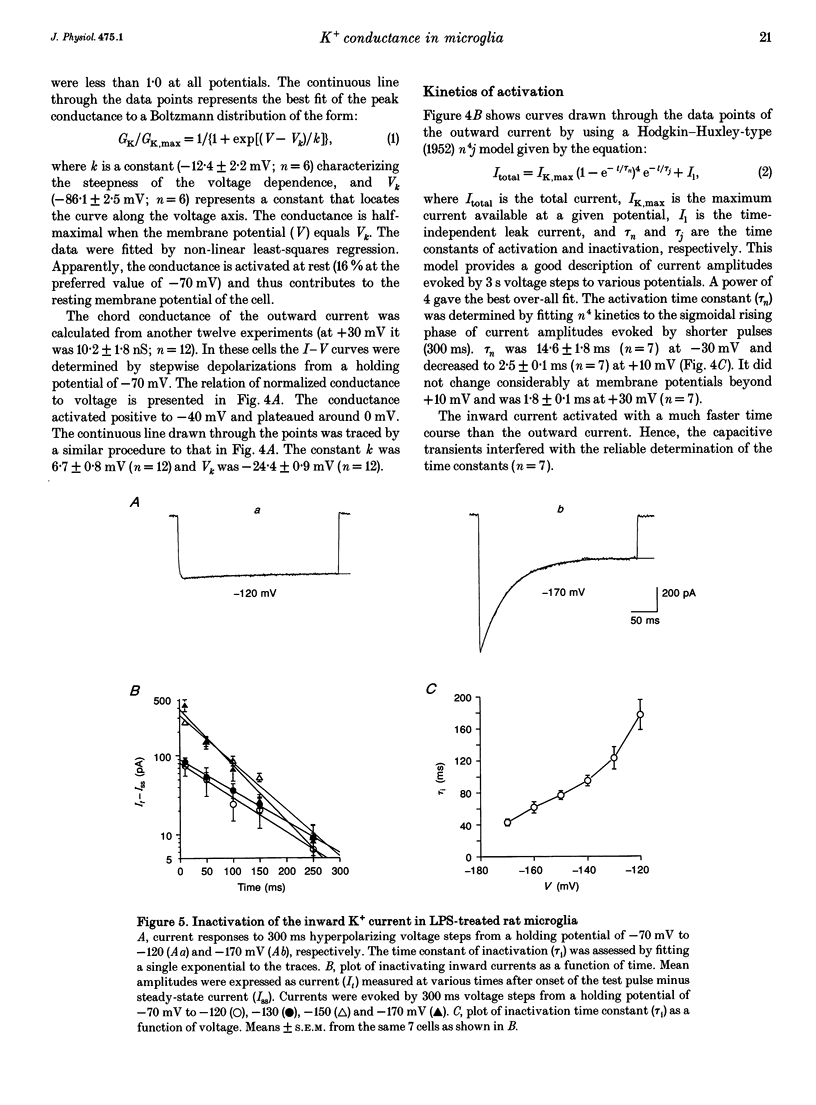
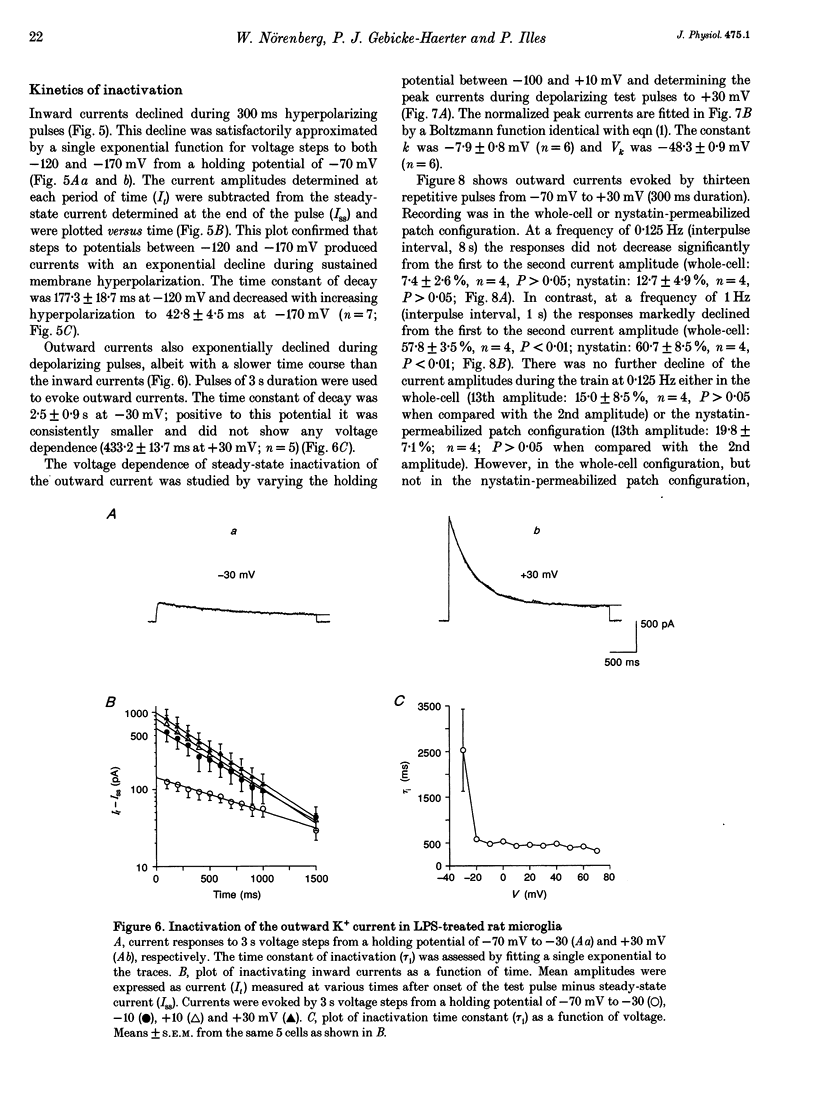
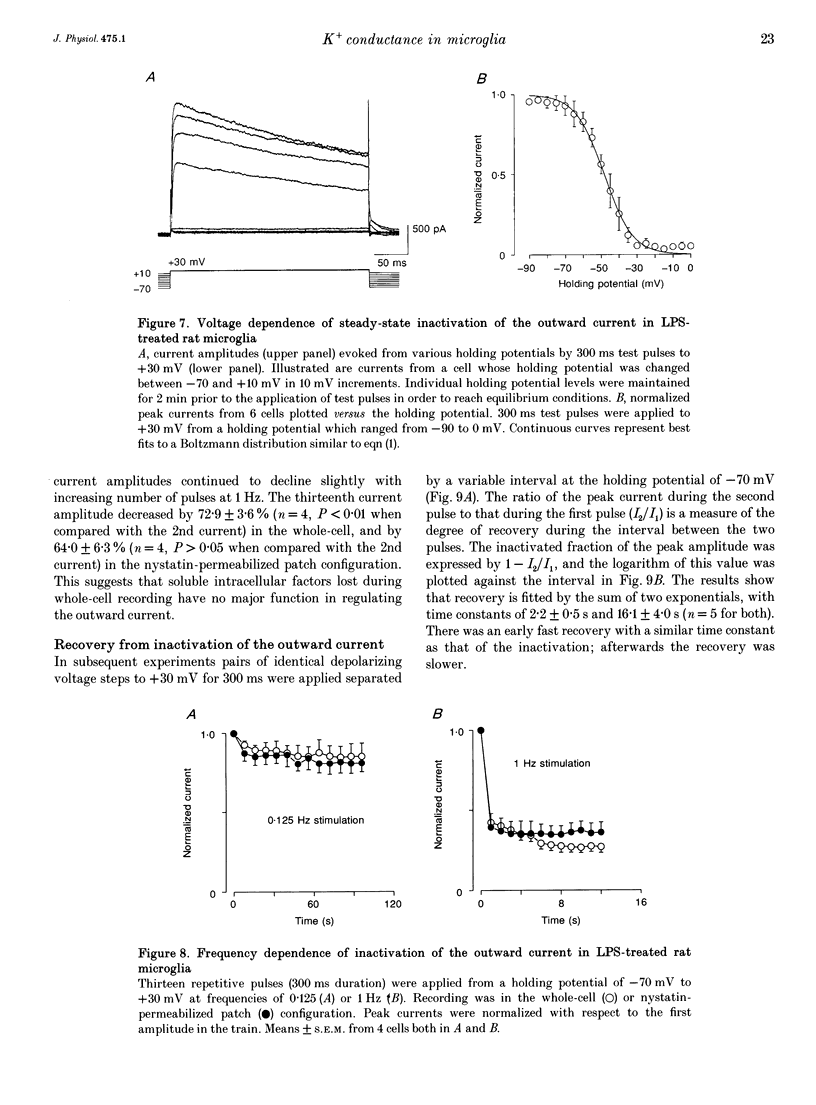
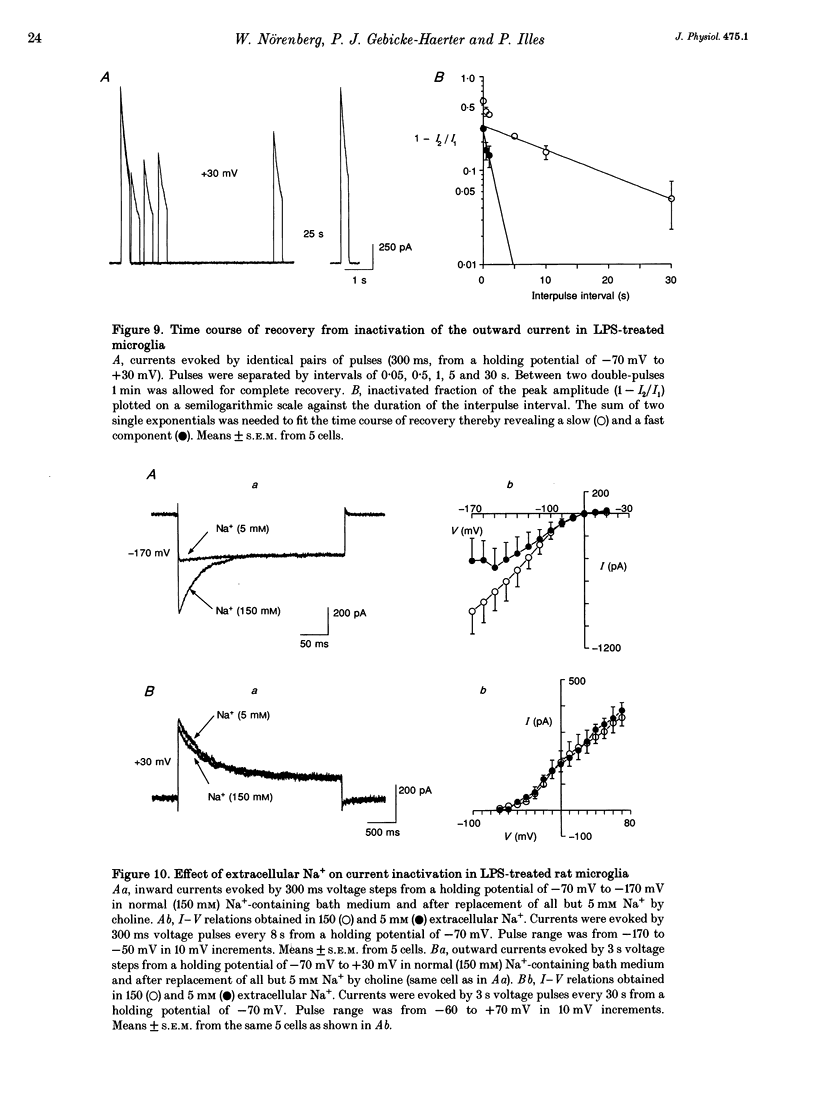
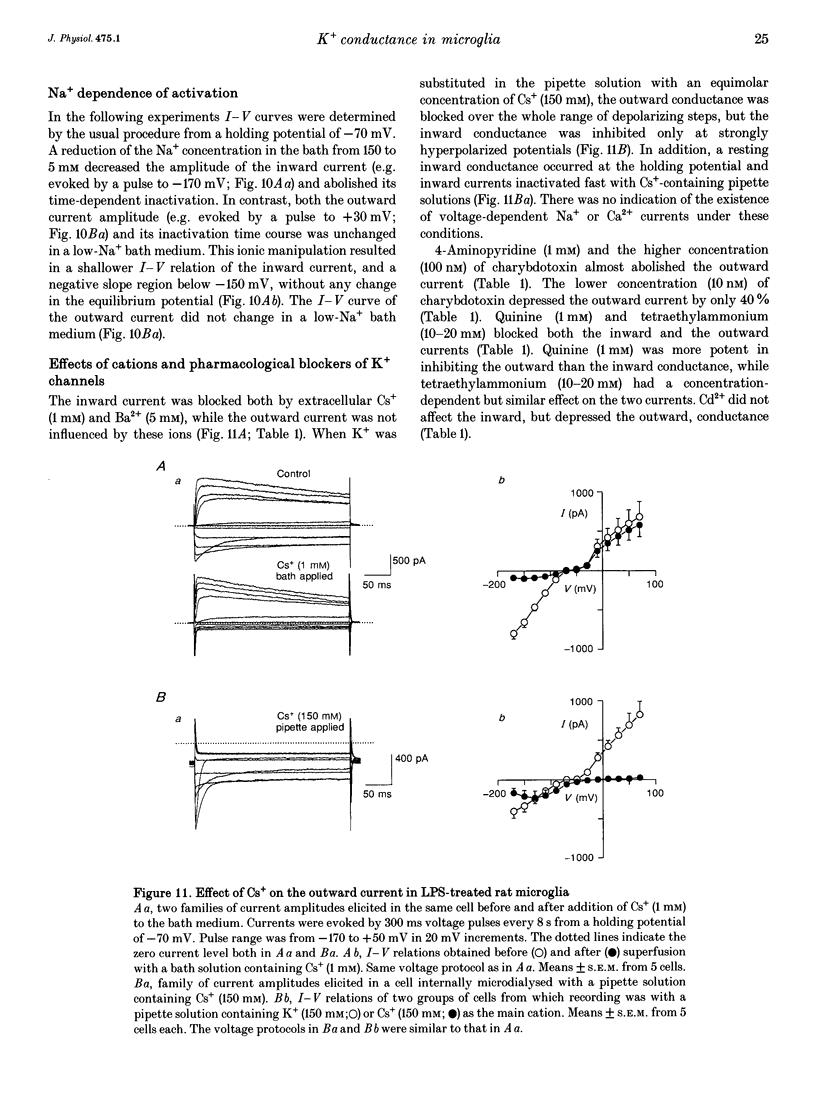
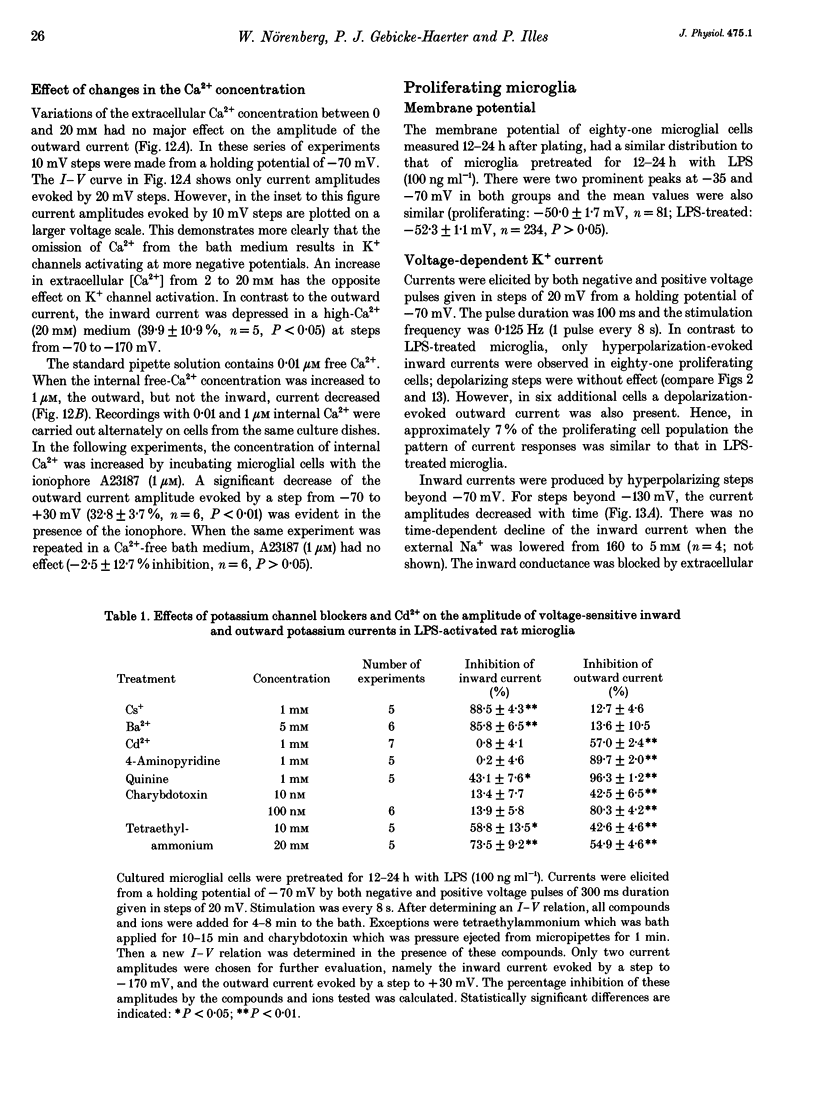
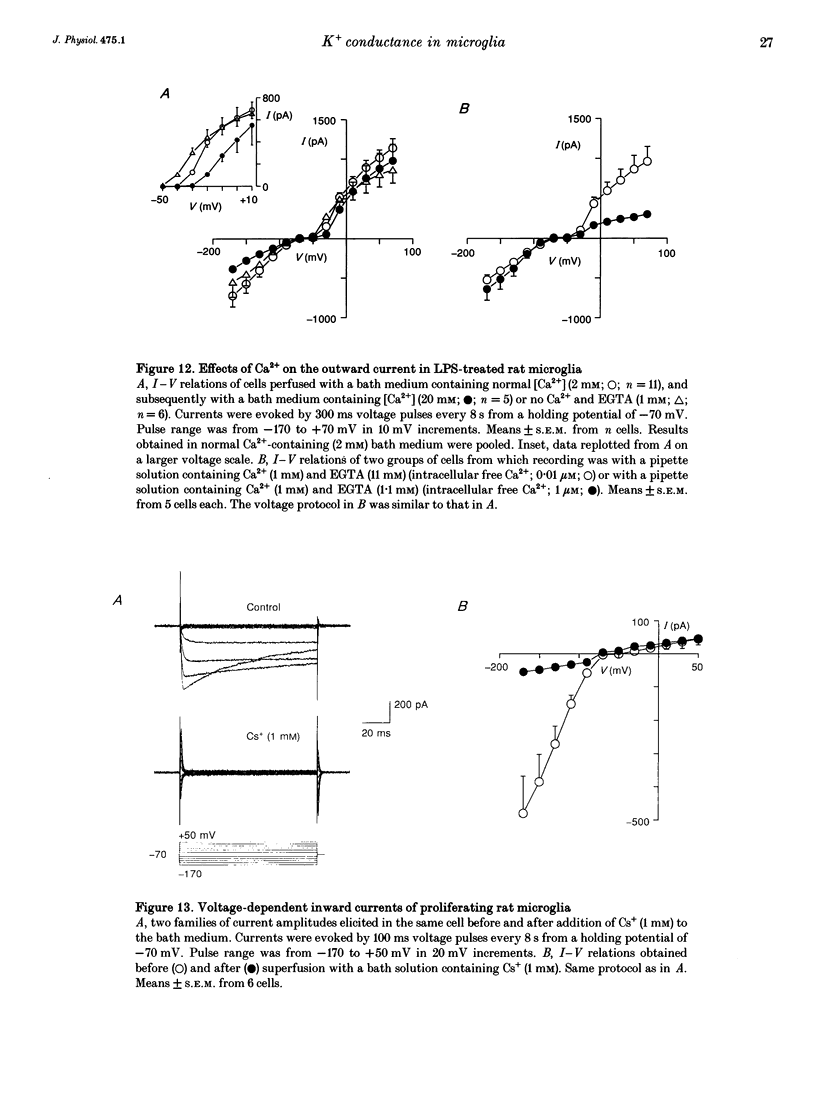
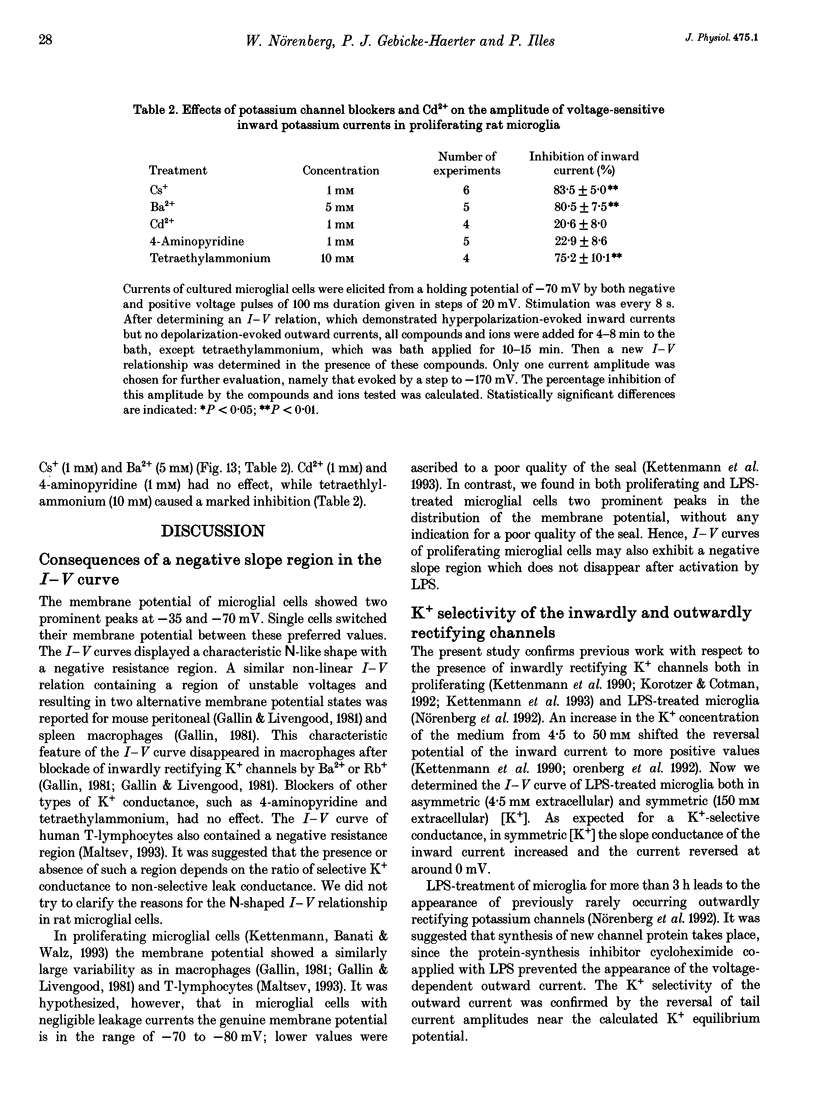
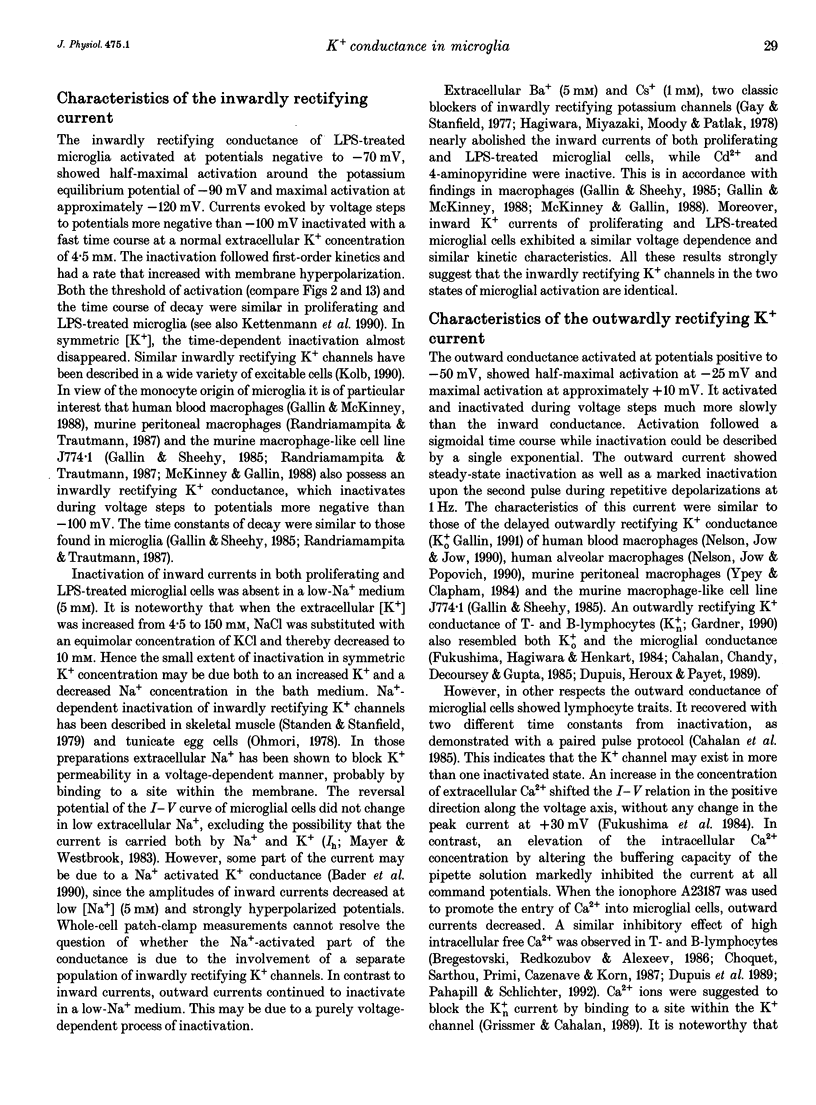
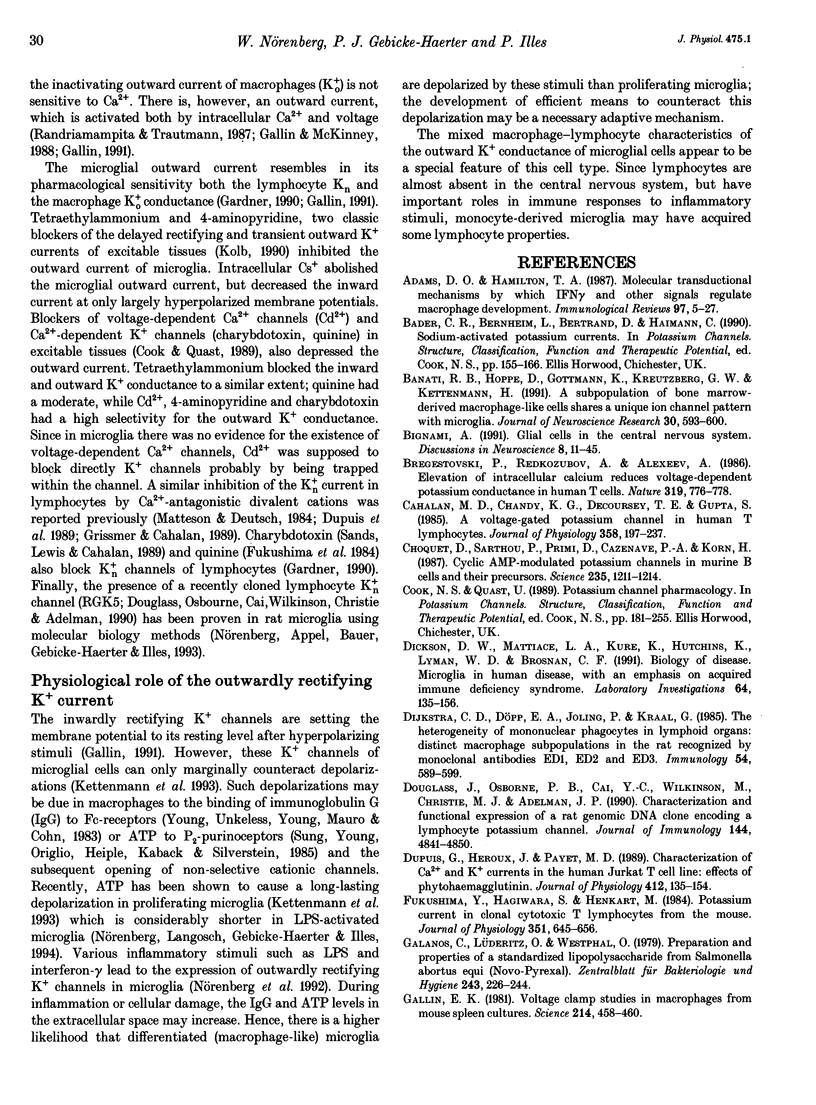
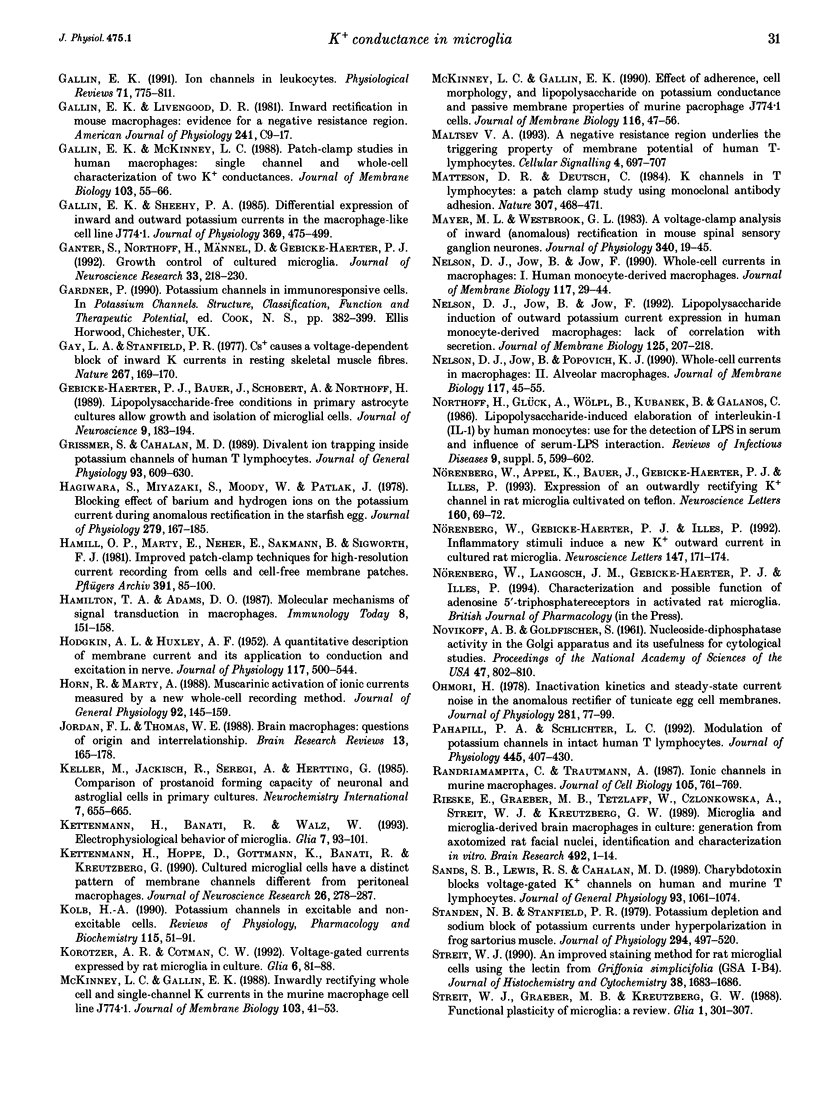
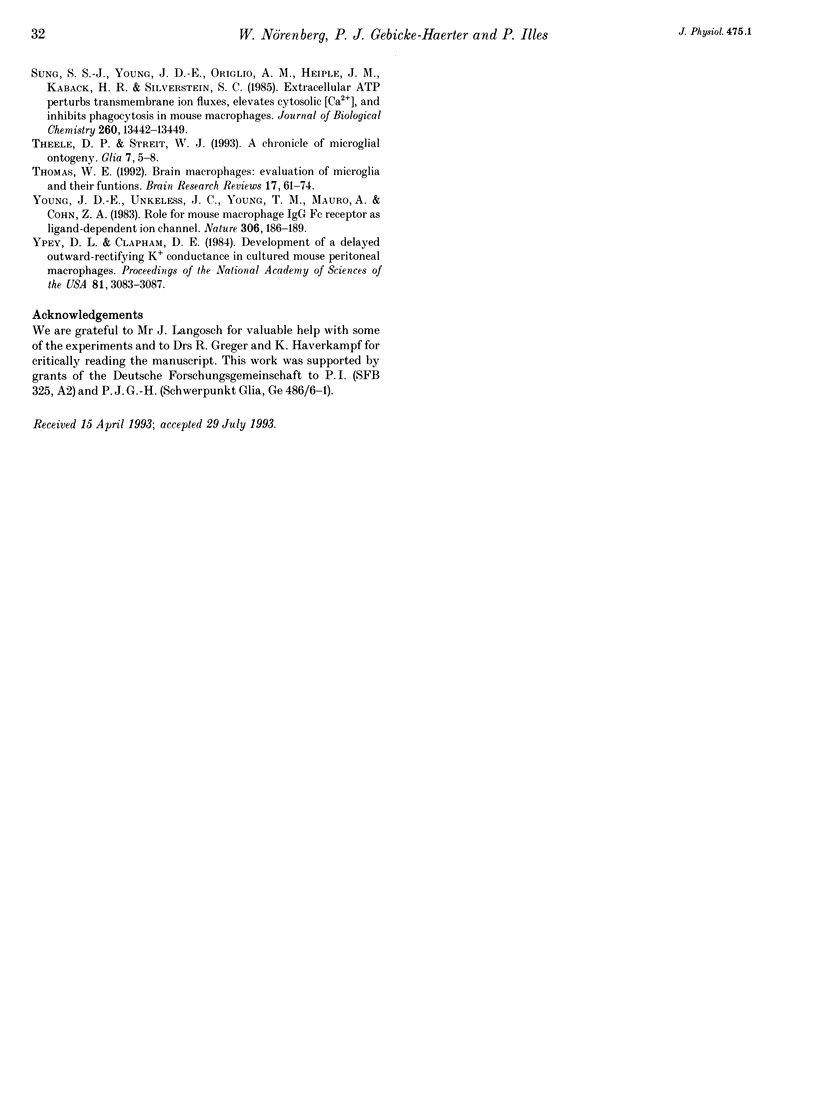
Selected References
These references are in PubMed. This may not be the complete list of references from this article.
- Adams D. O., Hamilton T. A. Molecular transductional mechanisms by which IFN gamma and other signals regulate macrophage development. Immunol Rev. 1987 Jun;97:5–27. doi: 10.1111/j.1600-065x.1987.tb00514.x. [DOI] [PubMed] [Google Scholar]
- Banati R. B., Hoppe D., Gottmann K., Kreutzberg G. W., Kettenmann H. A subpopulation of bone marrow-derived macrophage-like cells shares a unique ion channel pattern with microglia. J Neurosci Res. 1991 Dec;30(4):593–600. doi: 10.1002/jnr.490300402. [DOI] [PubMed] [Google Scholar]
- Bregestovski P., Redkozubov A., Alexeev A. Elevation of intracellular calcium reduces voltage-dependent potassium conductance in human T cells. 1986 Feb 27-Mar 5Nature. 319(6056):776–778. doi: 10.1038/319776a0. [DOI] [PubMed] [Google Scholar]
- Cahalan M. D., Chandy K. G., DeCoursey T. E., Gupta S. A voltage-gated potassium channel in human T lymphocytes. J Physiol. 1985 Jan;358:197–237. doi: 10.1113/jphysiol.1985.sp015548. [DOI] [PMC free article] [PubMed] [Google Scholar]
- Choquet D., Sarthou P., Primi D., Cazenave P. A., Korn H. Cyclic AMP-modulated potassium channels in murine B cells and their precursors. Science. 1987 Mar 6;235(4793):1211–1214. doi: 10.1126/science.2434998. [DOI] [PubMed] [Google Scholar]
- Dickson D. W., Mattiace L. A., Kure K., Hutchins K., Lyman W. D., Brosnan C. F. Microglia in human disease, with an emphasis on acquired immune deficiency syndrome. Lab Invest. 1991 Feb;64(2):135–156. [PubMed] [Google Scholar]
- Dijkstra C. D., Döpp E. A., Joling P., Kraal G. The heterogeneity of mononuclear phagocytes in lymphoid organs: distinct macrophage subpopulations in the rat recognized by monoclonal antibodies ED1, ED2 and ED3. Immunology. 1985 Mar;54(3):589–599. [PMC free article] [PubMed] [Google Scholar]
- Douglass J., Osborne P. B., Cai Y. C., Wilkinson M., Christie M. J., Adelman J. P. Characterization and functional expression of a rat genomic DNA clone encoding a lymphocyte potassium channel. J Immunol. 1990 Jun 15;144(12):4841–4850. [PubMed] [Google Scholar]
- Dupuis G., Héroux J., Payet M. D. Characterization of Ca2+ and K+ currents in the human Jurkat T cell line: effects of phytohaemagglutinin. J Physiol. 1989 May;412:135–154. doi: 10.1113/jphysiol.1989.sp017608. [DOI] [PMC free article] [PubMed] [Google Scholar]
- Fukushima Y., Hagiwara S., Henkart M. Potassium current in clonal cytotoxic T lymphocytes from the mouse. J Physiol. 1984 Jun;351:645–656. doi: 10.1113/jphysiol.1984.sp015268. [DOI] [PMC free article] [PubMed] [Google Scholar]
- Galanos C., Lüderitz O., Westphal O. Preparation and properties of a standardized lipopolysaccharide from salmonella abortus equi (Novo-Pyrexal). Zentralbl Bakteriol Orig A. 1979 Apr;243(2-3):226–244. [PubMed] [Google Scholar]
- Gallin E. K. Ion channels in leukocytes. Physiol Rev. 1991 Jul;71(3):775–811. doi: 10.1152/physrev.1991.71.3.775. [DOI] [PubMed] [Google Scholar]
- Gallin E. K., Livengood D. R. Inward rectification in mouse macrophages: evidence for a negative resistance region. Am J Physiol. 1981 Jul;241(1):C9–17. doi: 10.1152/ajpcell.1981.241.1.C9. [DOI] [PubMed] [Google Scholar]
- Gallin E. K., McKinney L. C. Patch-clamp studies in human macrophages: single-channel and whole-cell characterization of two K+ conductances. J Membr Biol. 1988 Jul;103(1):55–66. doi: 10.1007/BF01871932. [DOI] [PubMed] [Google Scholar]
- Gallin E. K., Sheehy P. A. Differential expression of inward and outward potassium currents in the macrophage-like cell line J774.1. J Physiol. 1985 Dec;369:475–499. doi: 10.1113/jphysiol.1985.sp015911. [DOI] [PMC free article] [PubMed] [Google Scholar]
- Gallin E. K. Voltage clamp studies in macrophages from mouse spleen cultures. Science. 1981 Oct 23;214(4519):458–460. doi: 10.1126/science.7291986. [DOI] [PubMed] [Google Scholar]
- Ganter S., Northoff H., Männel D., Gebicke-Härter P. J. Growth control of cultured microglia. J Neurosci Res. 1992 Oct;33(2):218–230. doi: 10.1002/jnr.490330205. [DOI] [PubMed] [Google Scholar]
- Gay L. A., Stanfield P. R. Cs(+) causes a voltage-dependent block of inward K currents in resting skeletal muscle fibres. Nature. 1977 May 12;267(5607):169–170. doi: 10.1038/267169a0. [DOI] [PubMed] [Google Scholar]
- Gebicke-Haerter P. J., Bauer J., Schobert A., Northoff H. Lipopolysaccharide-free conditions in primary astrocyte cultures allow growth and isolation of microglial cells. J Neurosci. 1989 Jan;9(1):183–194. doi: 10.1523/JNEUROSCI.09-01-00183.1989. [DOI] [PMC free article] [PubMed] [Google Scholar]
- Grissmer S., Cahalan M. D. Divalent ion trapping inside potassium channels of human T lymphocytes. J Gen Physiol. 1989 Apr;93(4):609–630. doi: 10.1085/jgp.93.4.609. [DOI] [PMC free article] [PubMed] [Google Scholar]
- HODGKIN A. L., HUXLEY A. F. A quantitative description of membrane current and its application to conduction and excitation in nerve. J Physiol. 1952 Aug;117(4):500–544. doi: 10.1113/jphysiol.1952.sp004764. [DOI] [PMC free article] [PubMed] [Google Scholar]
- Hagiwara S., Miyazaki S., Moody W., Patlak J. Blocking effects of barium and hydrogen ions on the potassium current during anomalous rectification in the starfish egg. J Physiol. 1978 Jun;279:167–185. doi: 10.1113/jphysiol.1978.sp012338. [DOI] [PMC free article] [PubMed] [Google Scholar]
- Hamill O. P., Marty A., Neher E., Sakmann B., Sigworth F. J. Improved patch-clamp techniques for high-resolution current recording from cells and cell-free membrane patches. Pflugers Arch. 1981 Aug;391(2):85–100. doi: 10.1007/BF00656997. [DOI] [PubMed] [Google Scholar]
- Horn R., Marty A. Muscarinic activation of ionic currents measured by a new whole-cell recording method. J Gen Physiol. 1988 Aug;92(2):145–159. doi: 10.1085/jgp.92.2.145. [DOI] [PMC free article] [PubMed] [Google Scholar]
- Jordan F. L., Thomas W. E. Brain macrophages: questions of origin and interrelationship. Brain Res. 1988 Apr-Jun;472(2):165–178. doi: 10.1016/0165-0173(88)90019-7. [DOI] [PubMed] [Google Scholar]
- Kettenmann H., Banati R., Walz W. Electrophysiological behavior of microglia. Glia. 1993 Jan;7(1):93–101. doi: 10.1002/glia.440070115. [DOI] [PubMed] [Google Scholar]
- Kettenmann H., Hoppe D., Gottmann K., Banati R., Kreutzberg G. Cultured microglial cells have a distinct pattern of membrane channels different from peritoneal macrophages. J Neurosci Res. 1990 Jul;26(3):278–287. doi: 10.1002/jnr.490260303. [DOI] [PubMed] [Google Scholar]
- Korotzer A. R., Cotman C. W. Voltage-gated currents expressed by rat microglia in culture. Glia. 1992;6(2):81–88. doi: 10.1002/glia.440060202. [DOI] [PubMed] [Google Scholar]
- Maltsev V. A. A negative resistance region underlies the triggering property of membrane potential in human T-lymphocytes. Cell Signal. 1992 Nov;4(6):697–707. doi: 10.1016/0898-6568(92)90050-i. [DOI] [PubMed] [Google Scholar]
- Matteson D. R., Deutsch C. K channels in T lymphocytes: a patch clamp study using monoclonal antibody adhesion. Nature. 1984 Feb 2;307(5950):468–471. doi: 10.1038/307468a0. [DOI] [PubMed] [Google Scholar]
- Mayer M. L., Westbrook G. L. A voltage-clamp analysis of inward (anomalous) rectification in mouse spinal sensory ganglion neurones. J Physiol. 1983 Jul;340:19–45. doi: 10.1113/jphysiol.1983.sp014747. [DOI] [PMC free article] [PubMed] [Google Scholar]
- McKinney L. C., Gallin E. K. Effect of adherence, cell morphology, and lipopolysaccharide on potassium conductance and passive membrane properties of murine macrophage J774.1 cells. J Membr Biol. 1990 Jun;116(1):47–56. doi: 10.1007/BF01871671. [DOI] [PubMed] [Google Scholar]
- McKinney L. C., Gallin E. K. Inwardly rectifying whole-cell and single-channel K currents in the murine macrophage cell line J774.1. J Membr Biol. 1988 Jul;103(1):41–53. doi: 10.1007/BF01871931. [DOI] [PubMed] [Google Scholar]
- NOVIKOFF A. B., GOLDFISCHER S. Nucleosidediphosphatase activity in the Golgi apparatus and its usefulness for cytological studies. Proc Natl Acad Sci U S A. 1961 Jun 15;47:802–810. doi: 10.1073/pnas.47.6.802. [DOI] [PMC free article] [PubMed] [Google Scholar]
- Nelson D. J., Jow B., Jow F. Lipopolysaccharide induction of outward potassium current expression in human monocyte-derived macrophages: lack of correlation with secretion. J Membr Biol. 1992 Feb;125(3):207–218. doi: 10.1007/BF00236434. [DOI] [PubMed] [Google Scholar]
- Nelson D. J., Jow B., Jow F. Whole-cell currents in macrophages: I. Human monocyte-derived macrophages. J Membr Biol. 1990 Jul;117(1):29–44. doi: 10.1007/BF01871563. [DOI] [PubMed] [Google Scholar]
- Nelson D. J., Jow B., Popovich K. J. Whole-cell currents in macrophages: II. Alveolar macrophages. J Membr Biol. 1990 Jul;117(1):45–55. doi: 10.1007/BF01871564. [DOI] [PubMed] [Google Scholar]
- Nörenberg W., Appel K., Bauer J., Gebicke-Haerter P. J., Illes P. Expression of an outwardly rectifying K+ channel in rat microglia cultivated on teflon. Neurosci Lett. 1993 Sep 17;160(1):69–72. doi: 10.1016/0304-3940(93)9001-0. [DOI] [PubMed] [Google Scholar]
- Nörenberg W., Gebicke-Haerter P. J., Illes P. Inflammatory stimuli induce a new K+ outward current in cultured rat microglia. Neurosci Lett. 1992 Dec 7;147(2):171–174. doi: 10.1016/0304-3940(92)90587-w. [DOI] [PubMed] [Google Scholar]
- Ohmori H. Inactivation kinetics and steady-state current noise in the anomalous rectifier of tunicate egg cell membranes. J Physiol. 1978 Aug;281:77–99. doi: 10.1113/jphysiol.1978.sp012410. [DOI] [PMC free article] [PubMed] [Google Scholar]
- Pahapill P. A., Schlichter L. C. Modulation of potassium channels in intact human T lymphocytes. J Physiol. 1992 Jan;445:407–430. doi: 10.1113/jphysiol.1992.sp018931. [DOI] [PMC free article] [PubMed] [Google Scholar]
- Randriamampita C., Trautmann A. Ionic channels in murine macrophages. J Cell Biol. 1987 Aug;105(2):761–769. doi: 10.1083/jcb.105.2.761. [DOI] [PMC free article] [PubMed] [Google Scholar]
- Rieske E., Graeber M. B., Tetzlaff W., Czlonkowska A., Streit W. J., Kreutzberg G. W. Microglia and microglia-derived brain macrophages in culture: generation from axotomized rat facial nuclei, identification and characterization in vitro. Brain Res. 1989 Jul 17;492(1-2):1–14. doi: 10.1016/0006-8993(89)90883-4. [DOI] [PubMed] [Google Scholar]
- Sands S. B., Lewis R. S., Cahalan M. D. Charybdotoxin blocks voltage-gated K+ channels in human and murine T lymphocytes. J Gen Physiol. 1989 Jun;93(6):1061–1074. doi: 10.1085/jgp.93.6.1061. [DOI] [PMC free article] [PubMed] [Google Scholar]
- Standen N. B., Stanfield P. R. Potassium depletion and sodium block of potassium currents under hyperpolarization in frog sartorius muscle. J Physiol. 1979 Sep;294:497–520. doi: 10.1113/jphysiol.1979.sp012943. [DOI] [PMC free article] [PubMed] [Google Scholar]
- Streit W. J. An improved staining method for rat microglial cells using the lectin from Griffonia simplicifolia (GSA I-B4). J Histochem Cytochem. 1990 Nov;38(11):1683–1686. doi: 10.1177/38.11.2212623. [DOI] [PubMed] [Google Scholar]
- Streit W. J., Graeber M. B., Kreutzberg G. W. Functional plasticity of microglia: a review. Glia. 1988;1(5):301–307. doi: 10.1002/glia.440010502. [DOI] [PubMed] [Google Scholar]
- Sung S. S., Young J. D., Origlio A. M., Heiple J. M., Kaback H. R., Silverstein S. C. Extracellular ATP perturbs transmembrane ion fluxes, elevates cytosolic [Ca2+], and inhibits phagocytosis in mouse macrophages. J Biol Chem. 1985 Nov 5;260(25):13442–13449. [PubMed] [Google Scholar]
- Theele D. P., Streit W. J. A chronicle of microglial ontogeny. Glia. 1993 Jan;7(1):5–8. doi: 10.1002/glia.440070104. [DOI] [PubMed] [Google Scholar]
- Thomas W. E. Brain macrophages: evaluation of microglia and their functions. Brain Res Brain Res Rev. 1992 Jan-Apr;17(1):61–74. doi: 10.1016/0165-0173(92)90007-9. [DOI] [PubMed] [Google Scholar]
- Young J. D., Unkeless J. C., Young T. M., Mauro A., Cohn Z. A. Role for mouse macrophage IgG Fc receptor as ligand-dependent ion channel. Nature. 1983 Nov 10;306(5939):186–189. doi: 10.1038/306186a0. [DOI] [PubMed] [Google Scholar]
- Ypey D. L., Clapham D. E. Development of a delayed outward-rectifying K+ conductance in cultured mouse peritoneal macrophages. Proc Natl Acad Sci U S A. 1984 May;81(10):3083–3087. doi: 10.1073/pnas.81.10.3083. [DOI] [PMC free article] [PubMed] [Google Scholar]