Abstract
1. We have measured intracellular pH (pHi) in freshly isolated pyramidal neurones from the CA1 region of the rat hippocampus using the fluorescent indicator 2',7'-bis(carboxy-ethyl)-5-(and-6)-carboxyfluorescein (BCECF). 2. The neurones selected by our isolation procedure, when studied in the nominal absence of CO2-HCO3-, had a mean steady-state pHi of 6.81 +/- 0.02 (n = 163). The recovery of pHi from acid loads was very slow. The rate of recovery from acid loads was reduced by Na+ removal, but only very slightly inhibited by 1 mM amiloride. 3. The addition of 5% CO2-25 mM HCO3- caused steady-state pHi to increase from 6.74 +/- 0.05 to 7.03 +/- 0.03 (n = 28). In the presence of 5% CO2-25 mM HCO3-, the rate of pHi recovery from acid loads was much faster than in its absence. 4. The HCO(3-)-induced alkalinization was reversible, and did not occur in the absence of extracellular Na+ or in the presence of DIDS (4,4'-diisothiocyanatostilbene- 2,2'-disulphonic acid). 5. In the absence of external Cl-, successive exposures to CO2-HCO3- elicited alkalinizations that were progressively reduced in rate and amplitude. This effect, presumably due to gradual depletion of internal Cl-, was rapidly reversed by returning Cl- to the external medium. 6. We conclude that the major acid-extrusion mechanism in pyramidal CA1 neurones is the Na(+)-dependent Cl(-)-HCO3- exchanger. The Na(+)-dependent mechanism that operates in the nominal absence of HCO3- is far less active.
Full text
PDF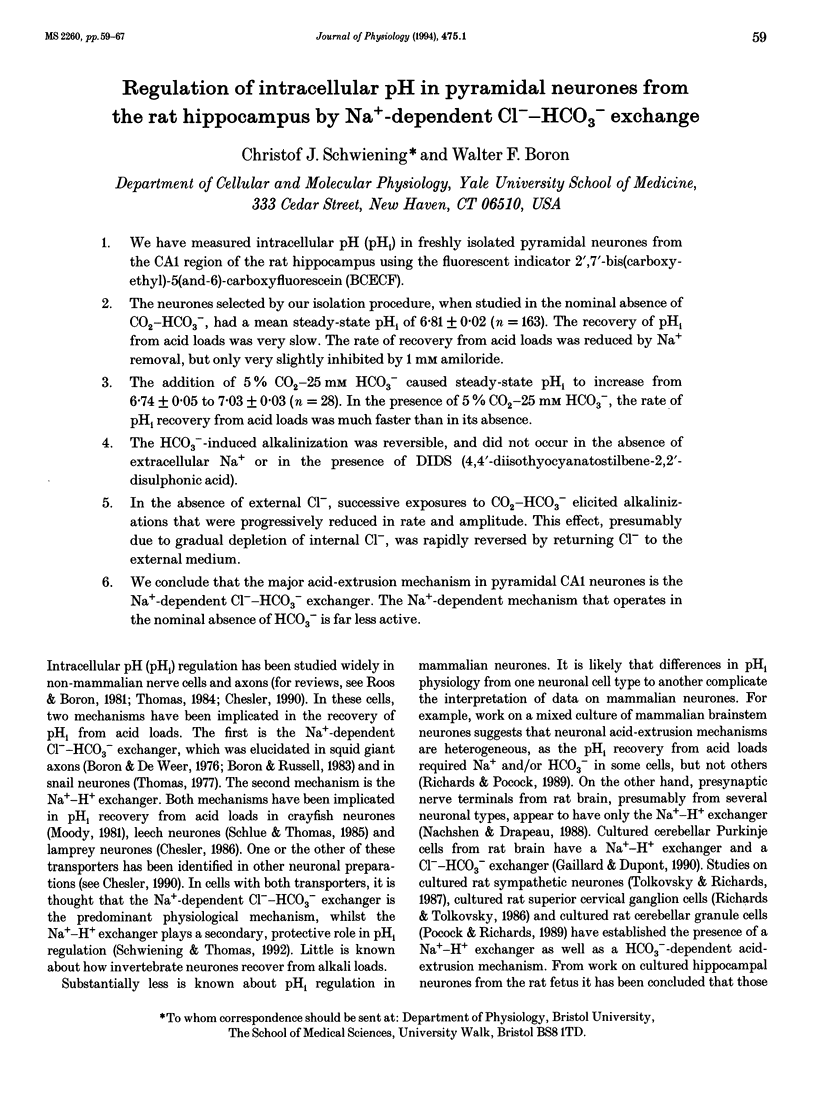
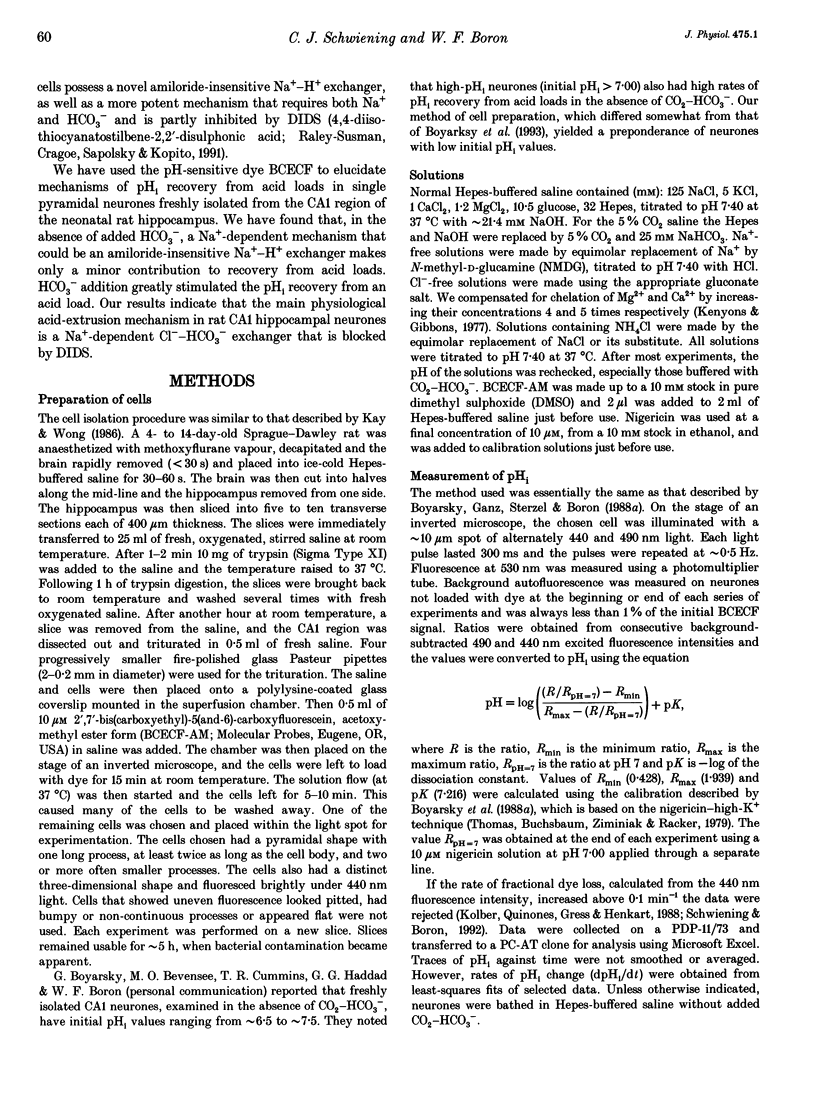
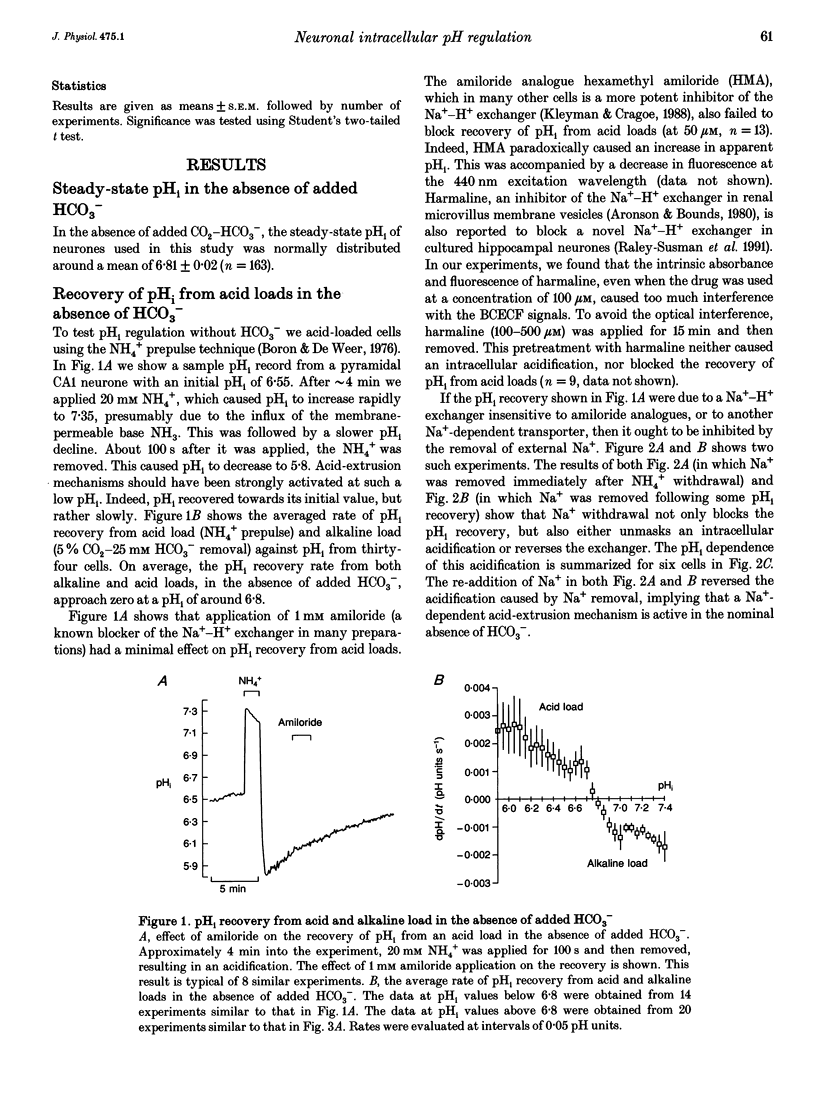
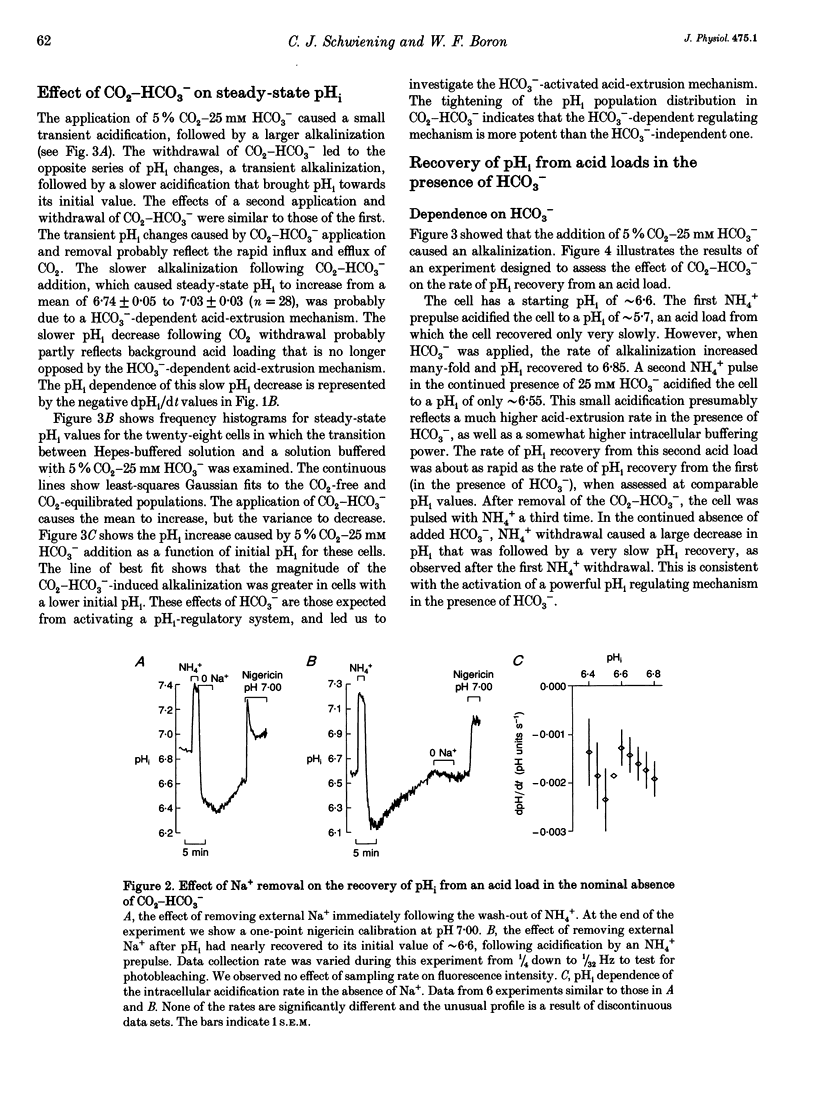
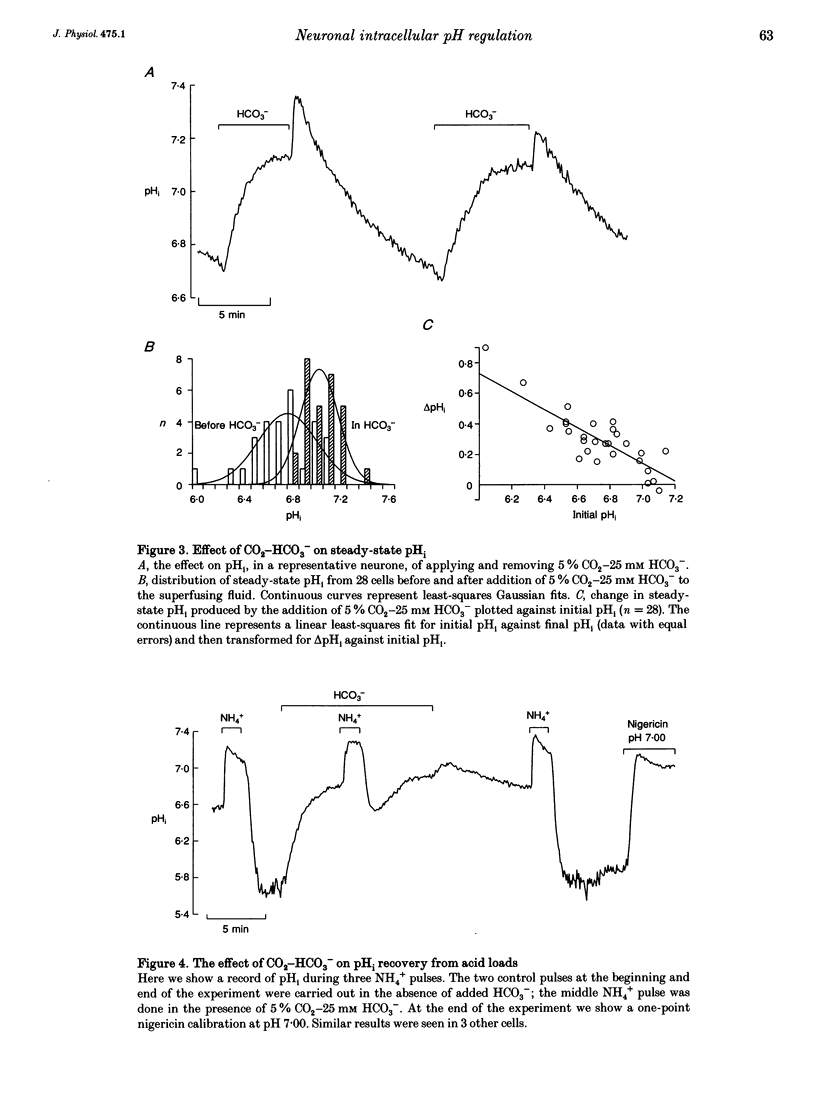
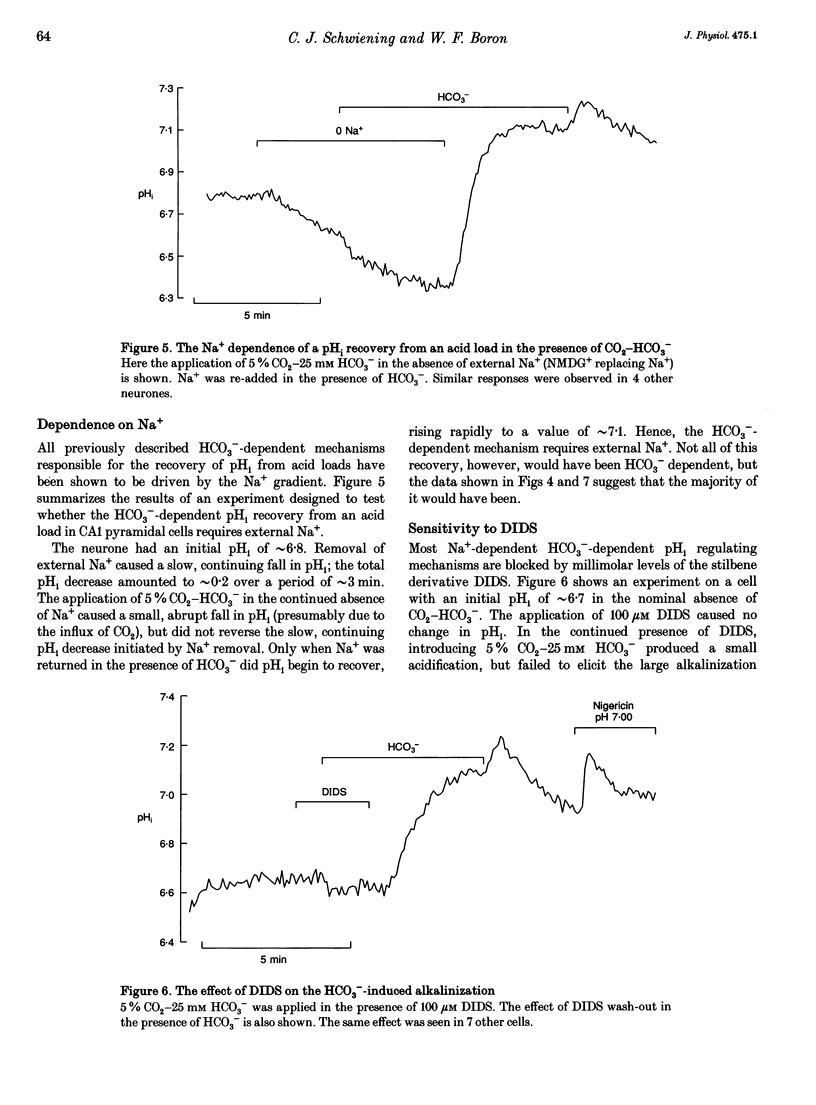
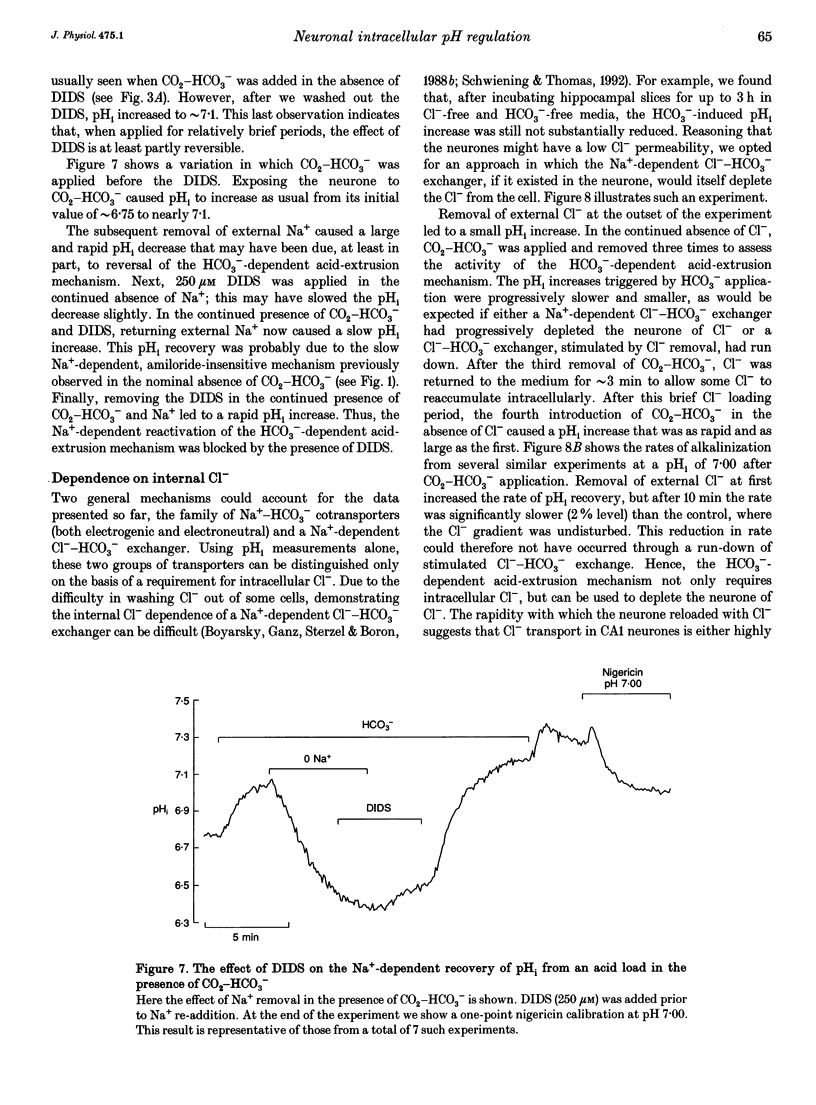
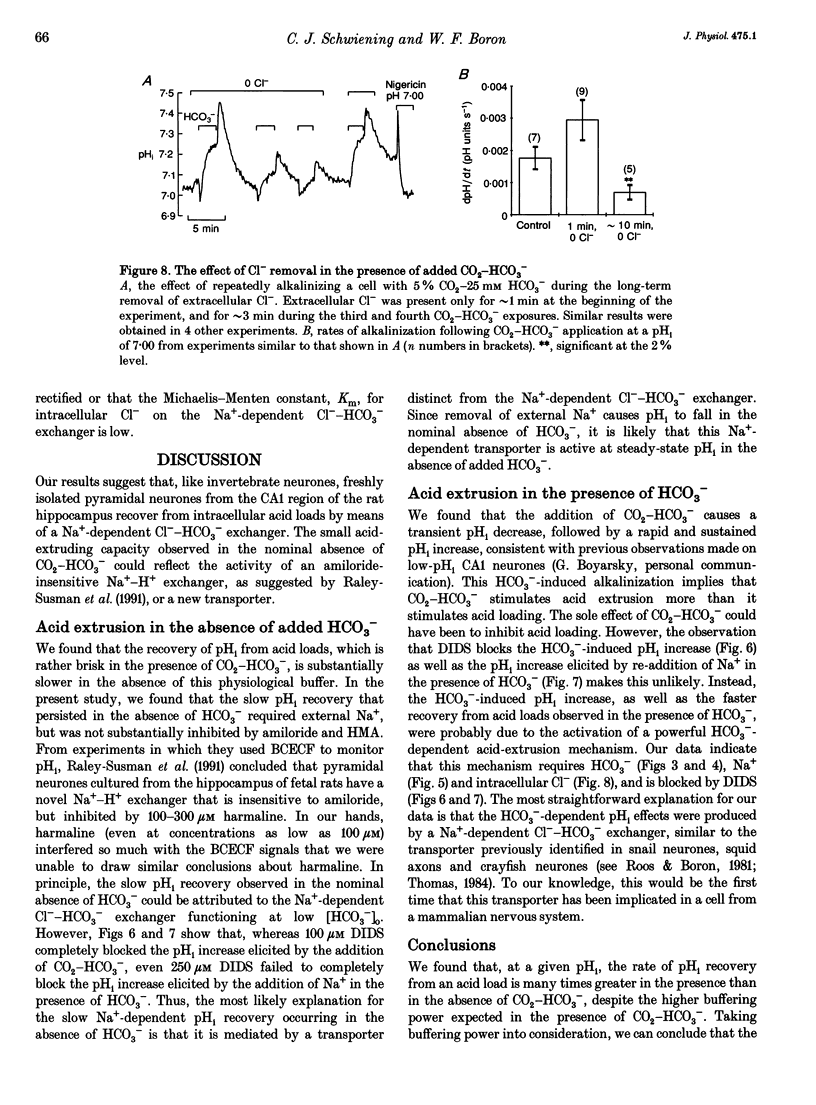
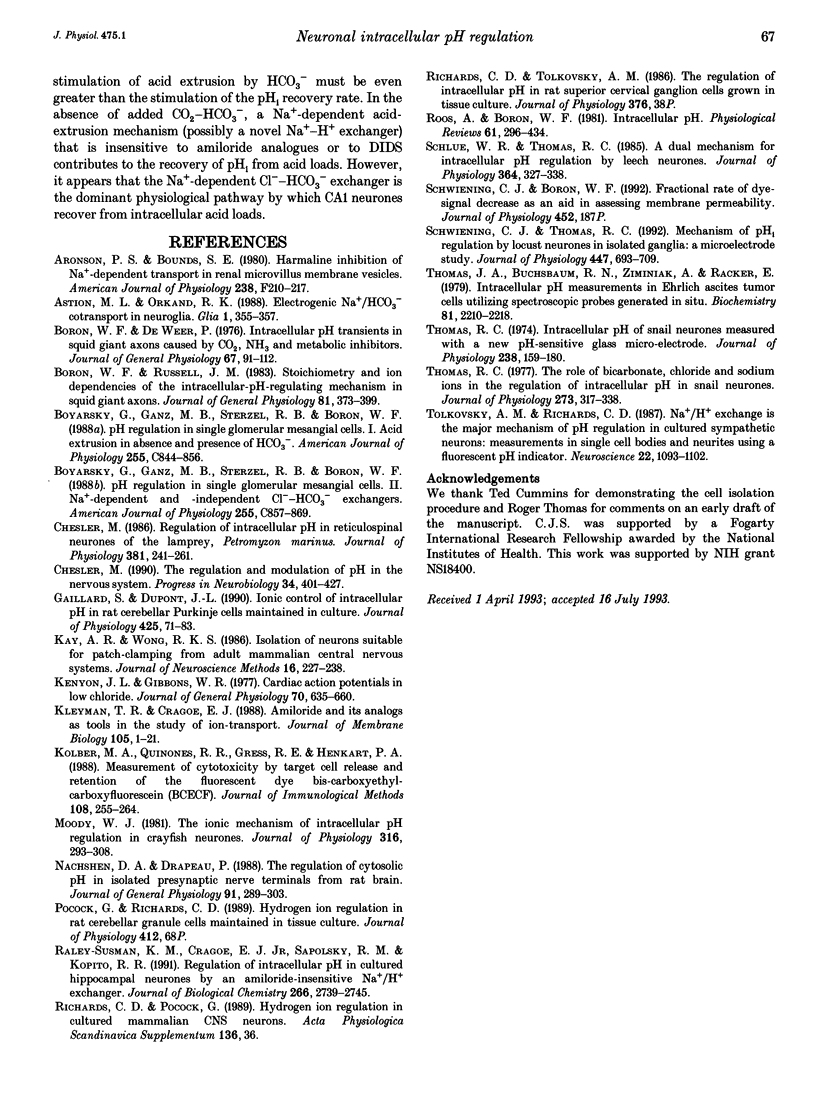
Selected References
These references are in PubMed. This may not be the complete list of references from this article.
- Aronson P. S., Bounds S. E. Harmaline inhibition of Na-dependent transport in renal microvillus membrane vesicles. Am J Physiol. 1980 Mar;238(3):F210–F217. doi: 10.1152/ajprenal.1980.238.3.F210. [DOI] [PubMed] [Google Scholar]
- Astion M. L., Orkand R. K. Electrogenic Na+/HCO3- cotransport in neuroglia. Glia. 1988;1(5):355–357. doi: 10.1002/glia.440010508. [DOI] [PubMed] [Google Scholar]
- Boron W. F., De Weer P. Intracellular pH transients in squid giant axons caused by CO2, NH3, and metabolic inhibitors. J Gen Physiol. 1976 Jan;67(1):91–112. doi: 10.1085/jgp.67.1.91. [DOI] [PMC free article] [PubMed] [Google Scholar]
- Boron W. F., Russell J. M. Stoichiometry and ion dependencies of the intracellular-pH-regulating mechanism in squid giant axons. J Gen Physiol. 1983 Mar;81(3):373–399. doi: 10.1085/jgp.81.3.373. [DOI] [PMC free article] [PubMed] [Google Scholar]
- Boyarsky G., Ganz M. B., Sterzel R. B., Boron W. F. pH regulation in single glomerular mesangial cells. I. Acid extrusion in absence and presence of HCO3-. Am J Physiol. 1988 Dec;255(6 Pt 1):C844–C856. doi: 10.1152/ajpcell.1988.255.6.C844. [DOI] [PubMed] [Google Scholar]
- Boyarsky G., Ganz M. B., Sterzel R. B., Boron W. F. pH regulation in single glomerular mesangial cells. II. Na+-dependent and -independent Cl(-)-HCO3- exchangers. Am J Physiol. 1988 Dec;255(6 Pt 1):C857–C869. doi: 10.1152/ajpcell.1988.255.6.C857. [DOI] [PubMed] [Google Scholar]
- Chesler M. Regulation of intracellular pH in reticulospinal neurones of the lamprey, Petromyzon marinus. J Physiol. 1986 Dec;381:241–261. doi: 10.1113/jphysiol.1986.sp016325. [DOI] [PMC free article] [PubMed] [Google Scholar]
- Chesler M. The regulation and modulation of pH in the nervous system. Prog Neurobiol. 1990;34(5):401–427. doi: 10.1016/0301-0082(90)90034-e. [DOI] [PubMed] [Google Scholar]
- Gaillard S., Dupont J. L. Ionic control of intracellular pH in rat cerebellar Purkinje cells maintained in culture. J Physiol. 1990 Jun;425:71–83. doi: 10.1113/jphysiol.1990.sp018093. [DOI] [PMC free article] [PubMed] [Google Scholar]
- Kay A. R., Wong R. K. Isolation of neurons suitable for patch-clamping from adult mammalian central nervous systems. J Neurosci Methods. 1986 May;16(3):227–238. doi: 10.1016/0165-0270(86)90040-3. [DOI] [PubMed] [Google Scholar]
- Kenyon J. L., Gibbons W. R. Effects of low-chloride solutions on action potentials of sheep cardiac Purkinje fibers. J Gen Physiol. 1977 Nov;70(5):635–660. doi: 10.1085/jgp.70.5.635. [DOI] [PMC free article] [PubMed] [Google Scholar]
- Kleyman T. R., Cragoe E. J., Jr Amiloride and its analogs as tools in the study of ion transport. J Membr Biol. 1988 Oct;105(1):1–21. doi: 10.1007/BF01871102. [DOI] [PubMed] [Google Scholar]
- Kolber M. A., Quinones R. R., Gress R. E., Henkart P. A. Measurement of cytotoxicity by target cell release and retention of the fluorescent dye bis-carboxyethyl-carboxyfluorescein (BCECF). J Immunol Methods. 1988 Apr 6;108(1-2):255–264. doi: 10.1016/0022-1759(88)90427-9. [DOI] [PubMed] [Google Scholar]
- Moody W. J., Jr The ionic mechanism of intracellular pH regulation in crayfish neurones. J Physiol. 1981 Jul;316:293–308. doi: 10.1113/jphysiol.1981.sp013788. [DOI] [PMC free article] [PubMed] [Google Scholar]
- Nachshen D. A., Drapeau P. The regulation of cytosolic pH in isolated presynaptic nerve terminals from rat brain. J Gen Physiol. 1988 Feb;91(2):289–303. doi: 10.1085/jgp.91.2.289. [DOI] [PMC free article] [PubMed] [Google Scholar]
- Raley-Susman K. M., Cragoe E. J., Jr, Sapolsky R. M., Kopito R. R. Regulation of intracellular pH in cultured hippocampal neurons by an amiloride-insensitive Na+/H+ exchanger. J Biol Chem. 1991 Feb 15;266(5):2739–2745. [PubMed] [Google Scholar]
- Richards C. D., Pocock G. Hydrogen ion regulation in cultured mammalian CNS neurones. Acta Physiol Scand Suppl. 1989;582:36–36. [PubMed] [Google Scholar]
- Roos A., Boron W. F. Intracellular pH. Physiol Rev. 1981 Apr;61(2):296–434. doi: 10.1152/physrev.1981.61.2.296. [DOI] [PubMed] [Google Scholar]
- Schlue W. R., Thomas R. C. A dual mechanism for intracellular pH regulation by leech neurones. J Physiol. 1985 Jul;364:327–338. doi: 10.1113/jphysiol.1985.sp015748. [DOI] [PMC free article] [PubMed] [Google Scholar]
- Schwiening C. J., Thomas R. C. Mechanism of pHi regulation by locust neurones in isolated ganglia: a microelectrode study. J Physiol. 1992 Feb;447:693–709. doi: 10.1113/jphysiol.1992.sp019024. [DOI] [PMC free article] [PubMed] [Google Scholar]
- Thomas J. A., Buchsbaum R. N., Zimniak A., Racker E. Intracellular pH measurements in Ehrlich ascites tumor cells utilizing spectroscopic probes generated in situ. Biochemistry. 1979 May 29;18(11):2210–2218. doi: 10.1021/bi00578a012. [DOI] [PubMed] [Google Scholar]
- Thomas R. C. Intracellular pH of snail neurones measured with a new pH-sensitive glass mirco-electrode. J Physiol. 1974 Apr;238(1):159–180. doi: 10.1113/jphysiol.1974.sp010516. [DOI] [PMC free article] [PubMed] [Google Scholar]
- Thomas R. C. The role of bicarbonate, chloride and sodium ions in the regulation of intracellular pH in snail neurones. J Physiol. 1977 Dec;273(1):317–338. doi: 10.1113/jphysiol.1977.sp012096. [DOI] [PMC free article] [PubMed] [Google Scholar]
- Tolkovsky A. M., Richards C. D. Na+/H+ exchange is the major mechanism of pH regulation in cultured sympathetic neurons: measurements in single cell bodies and neurites using a fluorescent pH indicator. Neuroscience. 1987 Sep;22(3):1093–1102. doi: 10.1016/0306-4522(87)92984-8. [DOI] [PubMed] [Google Scholar]