Abstract
1. The fall of maximum Ca(2+)-activated force of cardiac myofibrils at short muscle lengths could be due to a reduction of cross-bridge cycling or to development of an opposing (restoring) force. To try to distinguish between these possibilities, we measured simultaneously myofibrillar force development and MgATPase activity (a measure of cross-bridge cycling) in rat skinned trabeculae at different muscle lengths. ATPase activity was measured photometrically from the utilization of NADH in a coupled enzyme assay. Muscle length was varied to give estimated 0.2 micron changes in sarcomere length (SL) over the range 1.4-2.4 microns. 2. Both Ca(2+)-activated force development and ATPase activity were optimal at a muscle length (Lo) where the resting SL was 2.2 microns. At Lo the maximum ATPase activity at 21 degrees C was 0.56 +/- 0.05 mM s-1 (mean +/- S.E.M., n = 6), which was equivalent to an ATP turnover per myosin S1 head of 3.3 s-1. 3. The relationship between ATPase activity and SL was curved, with rather little change in ATPase activity over the SL range 2.0-2.4 microns, but significant falls at 1.8 microns and below. At 65% of Lo (corresponding to a mean active SL of approximately 1.4 microns), the ATPase activity was only 50% of its value at 2.2 microns SL. 4. Force development decreased linearly as SL was reduced below 2.2 microns. Force fell by more than ATPase activity, particularly at SL 1.6 and 1.8 microns. 5. The fall of ATPase activity indicates that some of the decline of force production at short SL results from a fall in the net rate of cross-bridge cycling. This is probably the result of double overlap of thin filaments. However, the differential effect on force and ATPase reveals that, in the intermediate range of SL, decreased cross-bridge cycling can account for only part of the fall of force; the remainder is probably due to an increase in a restoring force, which may arise from deformation of the connective tissue in the muscle preparations used.
Full text
PDF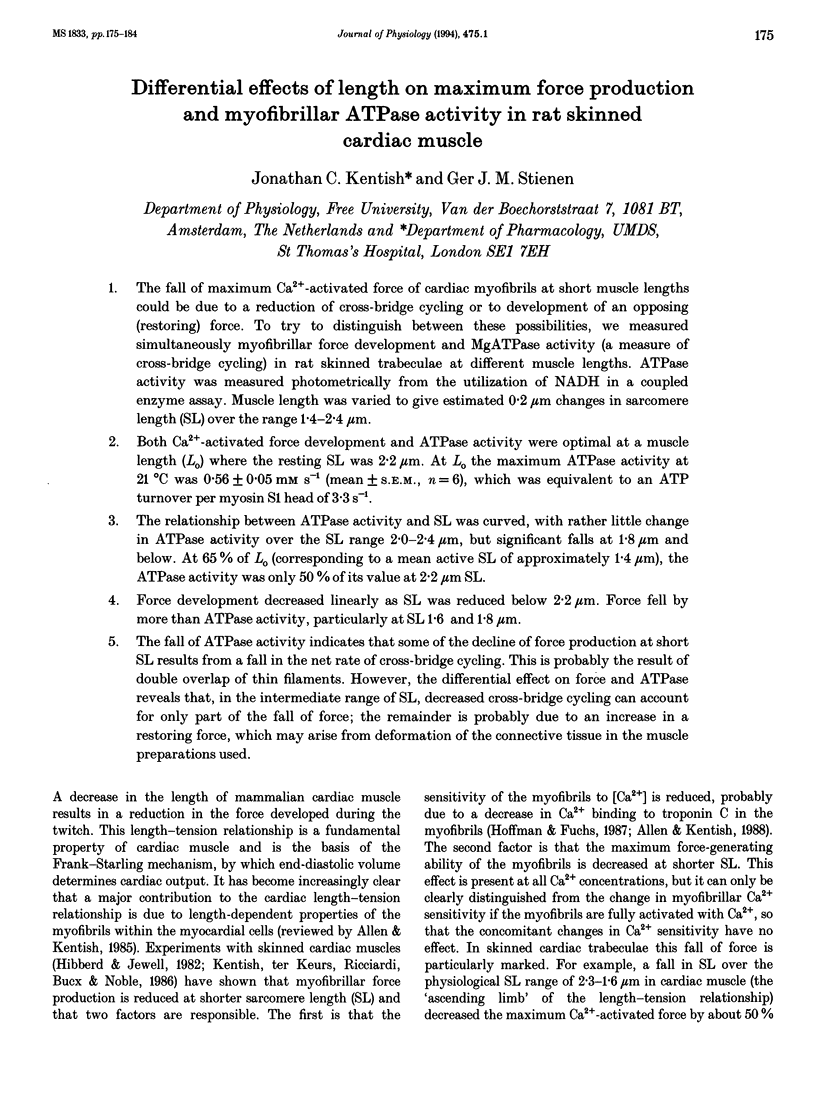
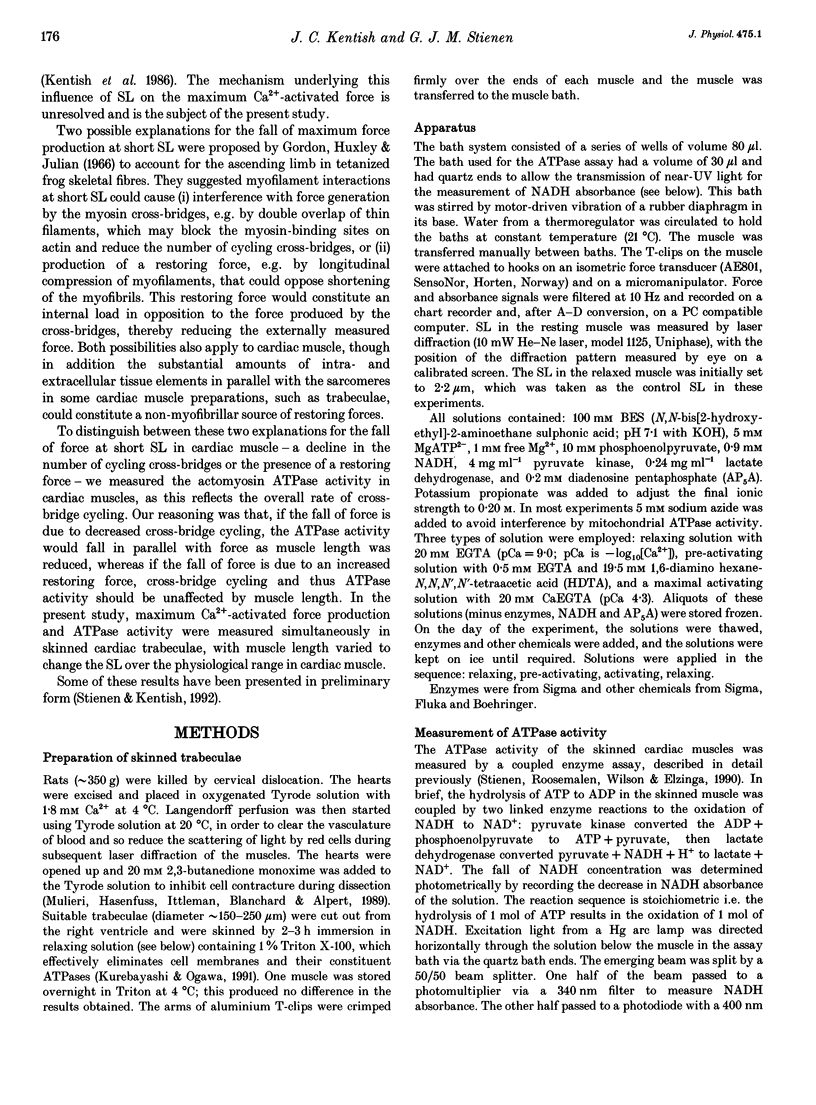
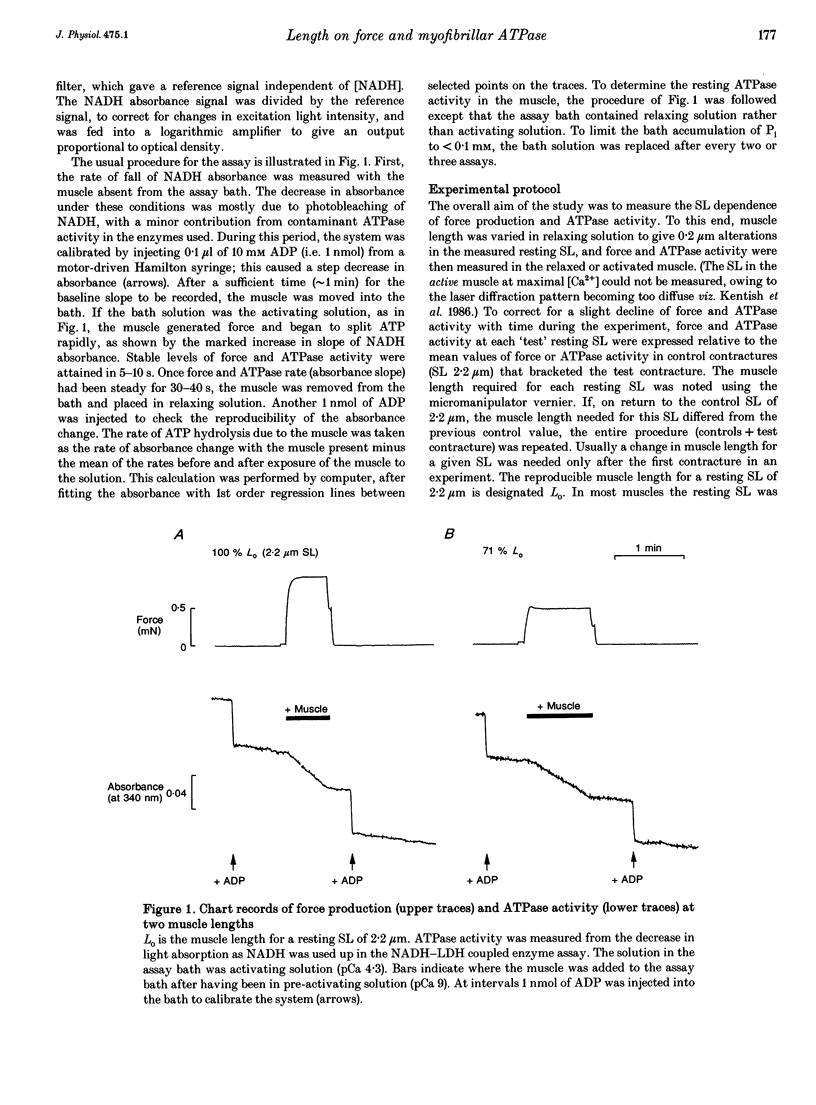
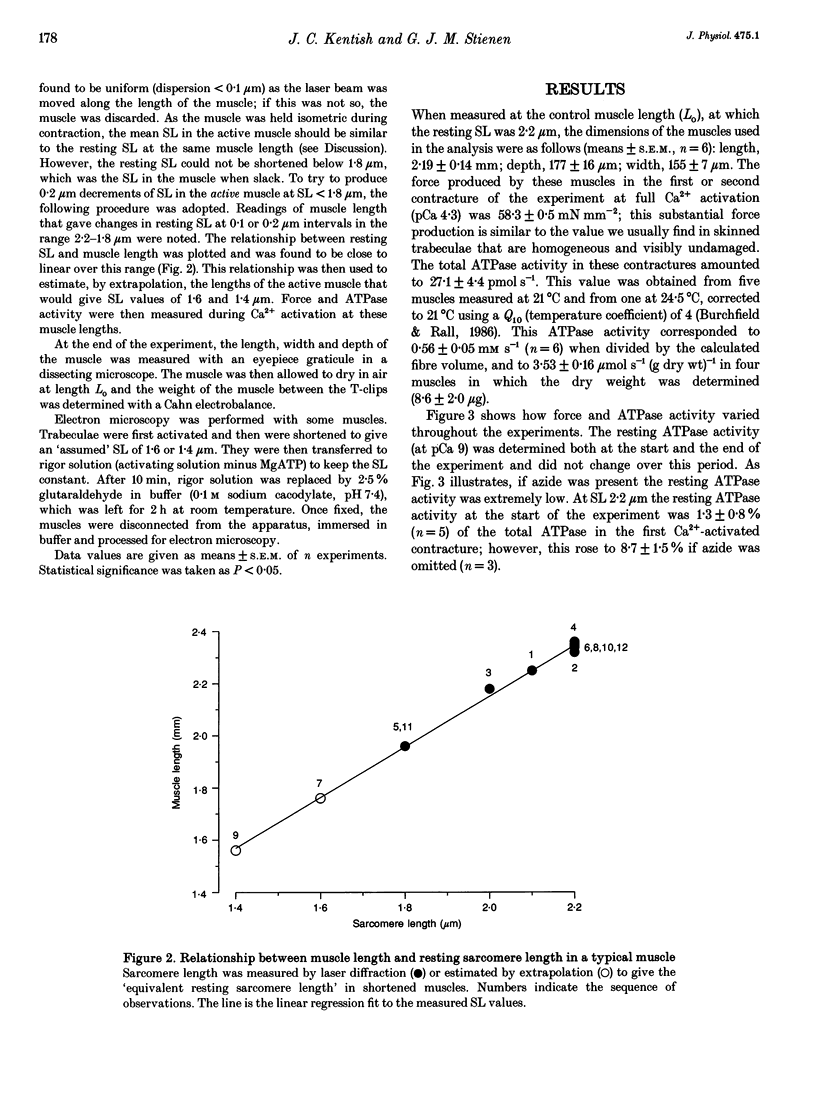
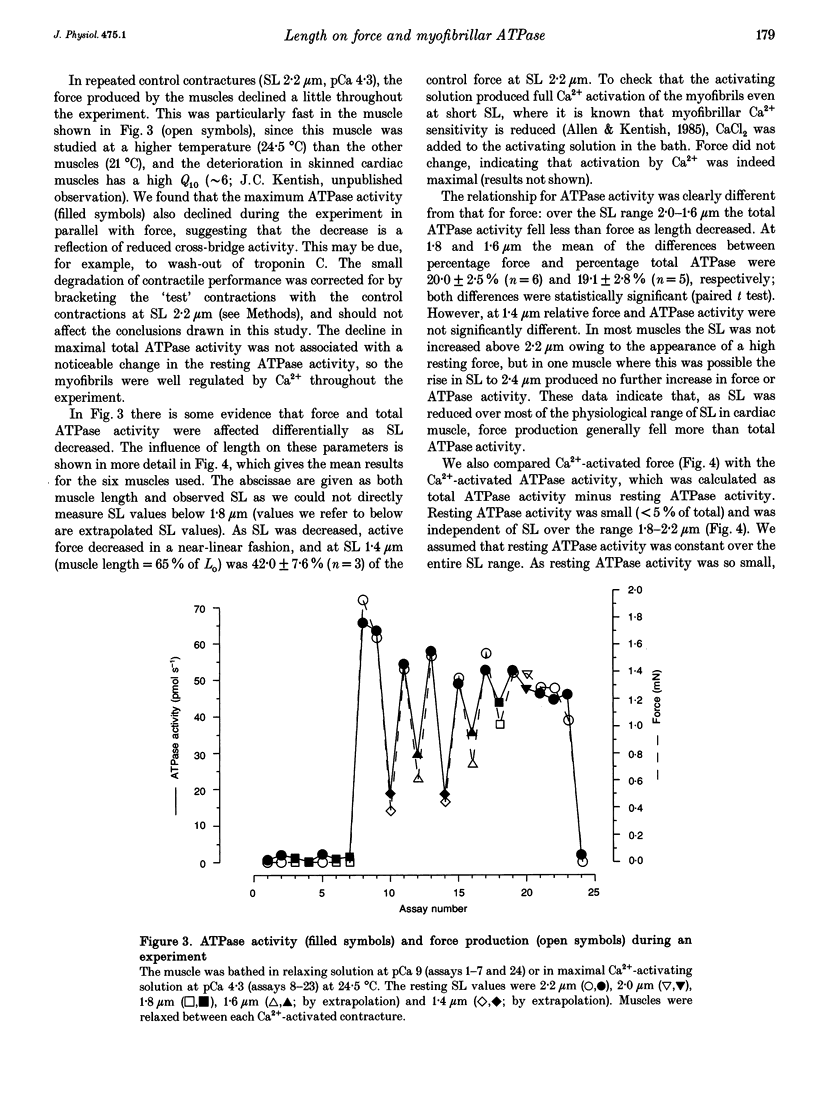
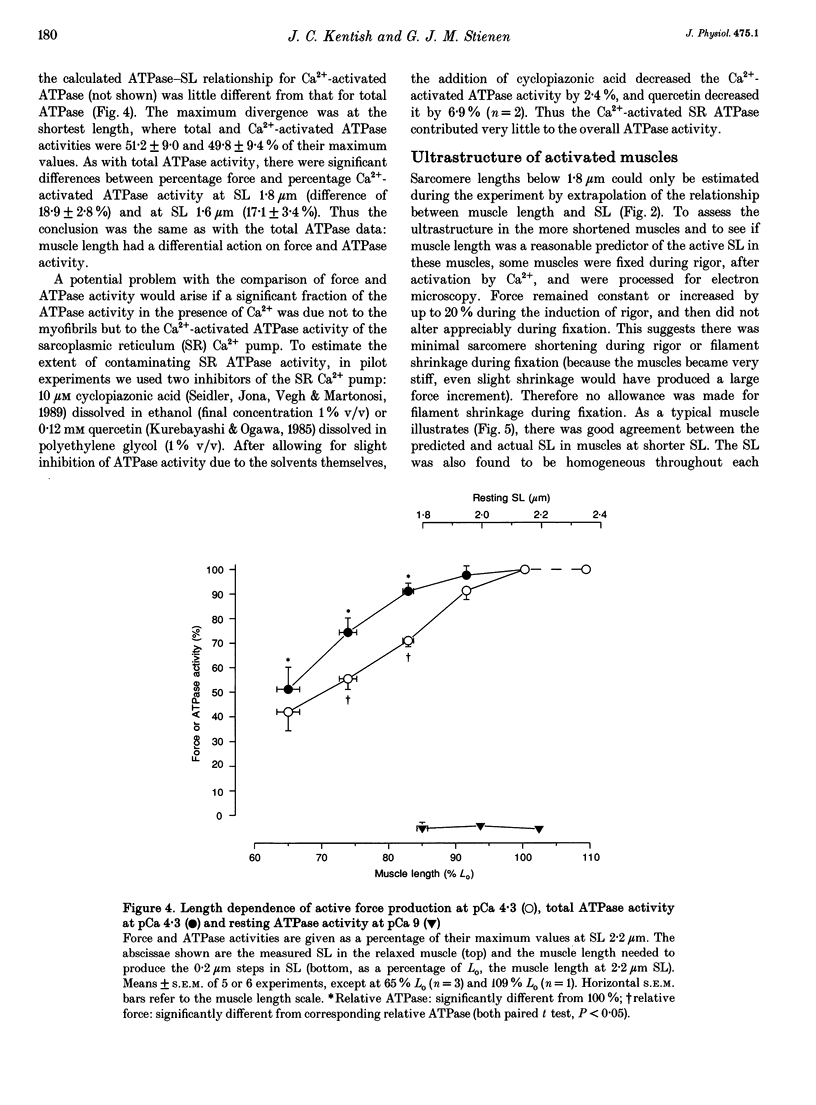
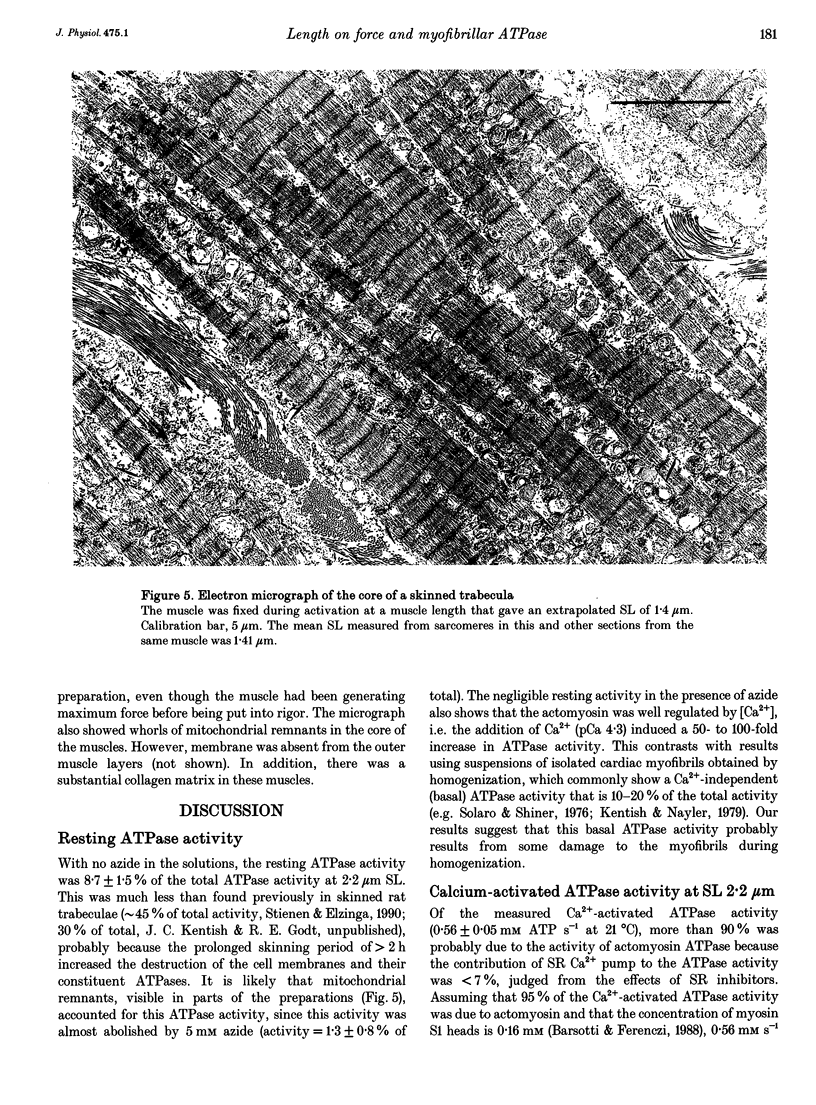
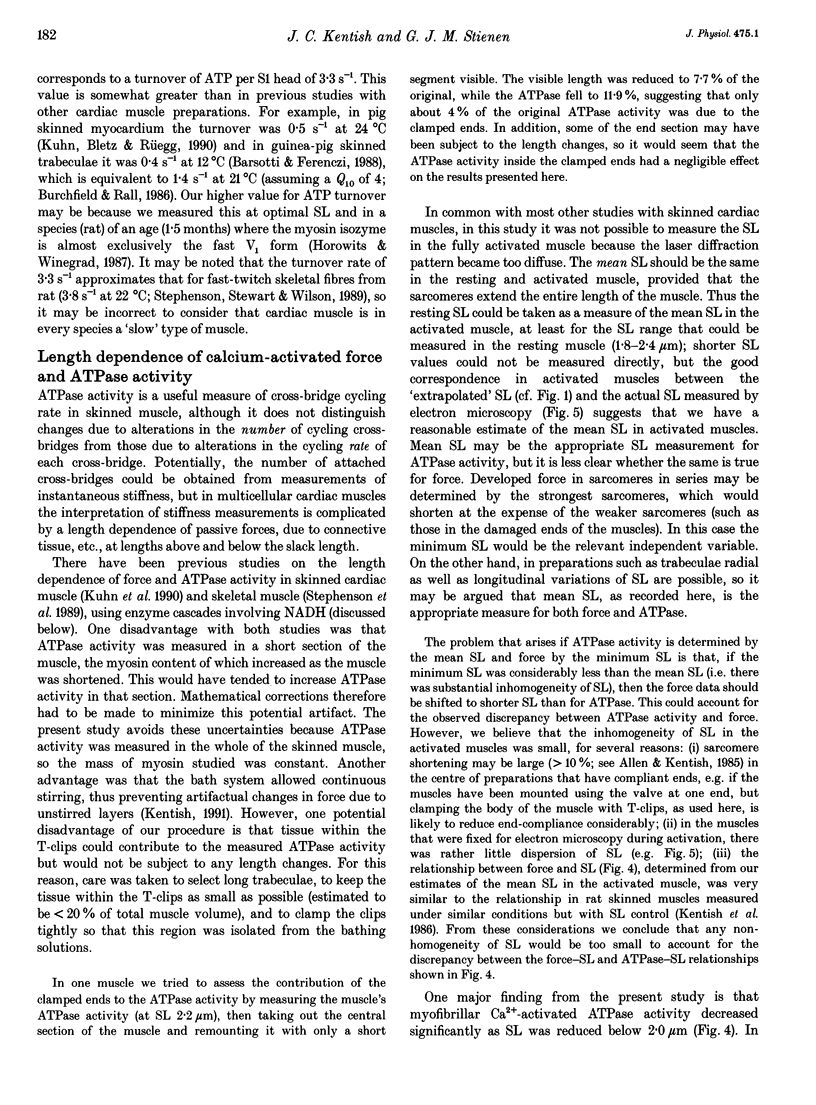
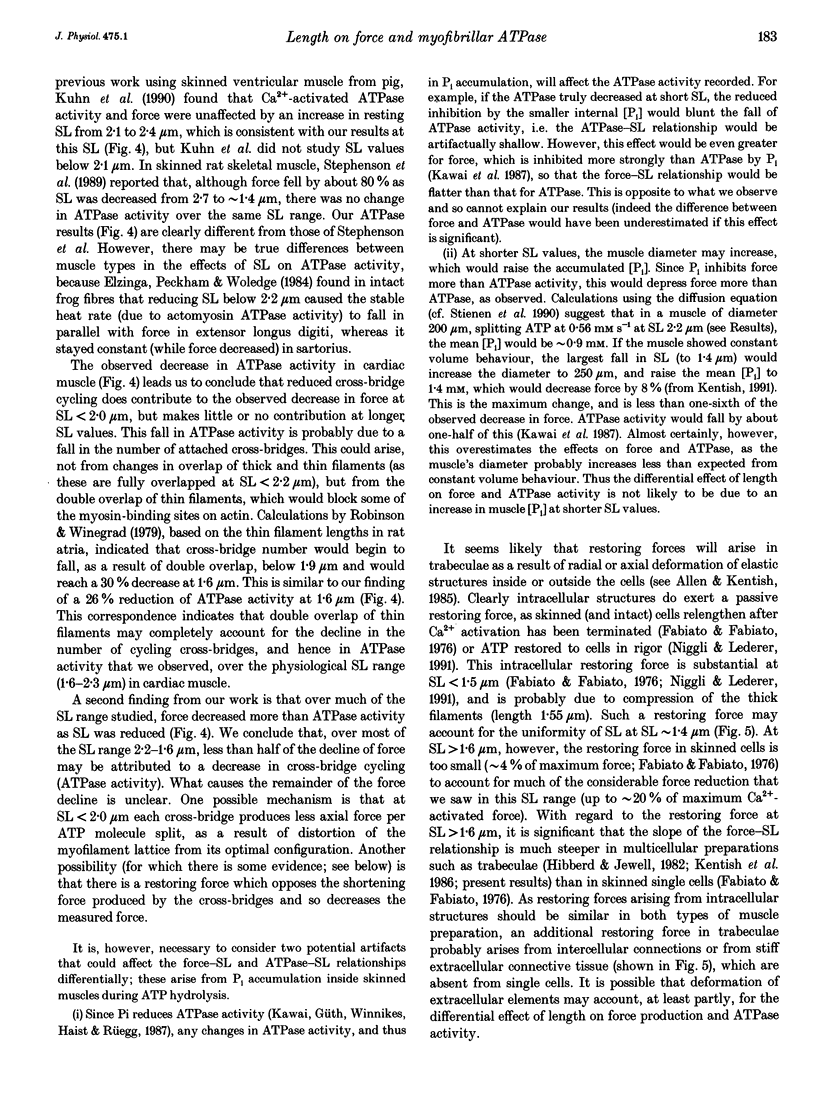
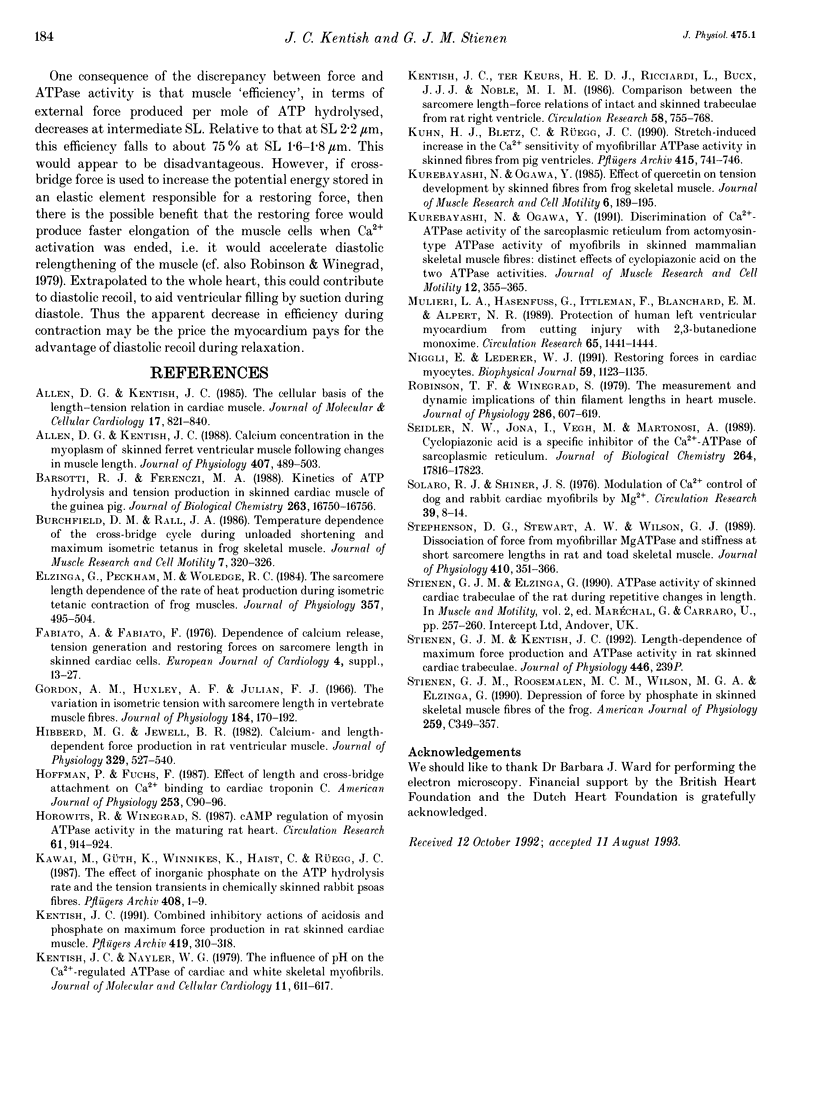
Images in this article
Selected References
These references are in PubMed. This may not be the complete list of references from this article.
- Allen D. G., Kentish J. C. Calcium concentration in the myoplasm of skinned ferret ventricular muscle following changes in muscle length. J Physiol. 1988 Dec;407:489–503. doi: 10.1113/jphysiol.1988.sp017427. [DOI] [PMC free article] [PubMed] [Google Scholar]
- Allen D. G., Kentish J. C. The cellular basis of the length-tension relation in cardiac muscle. J Mol Cell Cardiol. 1985 Sep;17(9):821–840. doi: 10.1016/s0022-2828(85)80097-3. [DOI] [PubMed] [Google Scholar]
- Barsotti R. J., Ferenczi M. A. Kinetics of ATP hydrolysis and tension production in skinned cardiac muscle of the guinea pig. J Biol Chem. 1988 Nov 15;263(32):16750–16756. [PubMed] [Google Scholar]
- Burchfield D. M., Rall J. A. Temperature dependence of the crossbridge cycle during unloaded shortening and maximum isometric tetanus in frog skeletal muscle. J Muscle Res Cell Motil. 1986 Aug;7(4):320–326. doi: 10.1007/BF01753652. [DOI] [PubMed] [Google Scholar]
- Elzinga G., Peckham M., Woledge R. C. The sarcomere length dependence of the rate of heat production during isometric tetanic contraction of frog muscles. J Physiol. 1984 Dec;357:495–504. doi: 10.1113/jphysiol.1984.sp015513. [DOI] [PMC free article] [PubMed] [Google Scholar]
- Fabiato A., Fabiato F. Dependence of calcium release, tension generation and restoring forces on sarcomere length in skinned cardiac cells. Eur J Cardiol. 1976 May;4 (Suppl):13–27. [PubMed] [Google Scholar]
- Gordon A. M., Huxley A. F., Julian F. J. The variation in isometric tension with sarcomere length in vertebrate muscle fibres. J Physiol. 1966 May;184(1):170–192. doi: 10.1113/jphysiol.1966.sp007909. [DOI] [PMC free article] [PubMed] [Google Scholar]
- Hibberd M. G., Jewell B. R. Calcium- and length-dependent force production in rat ventricular muscle. J Physiol. 1982 Aug;329:527–540. doi: 10.1113/jphysiol.1982.sp014317. [DOI] [PMC free article] [PubMed] [Google Scholar]
- Hofmann P. A., Fuchs F. Effect of length and cross-bridge attachment on Ca2+ binding to cardiac troponin C. Am J Physiol. 1987 Jul;253(1 Pt 1):C90–C96. doi: 10.1152/ajpcell.1987.253.1.C90. [DOI] [PubMed] [Google Scholar]
- Horowits R., Winegrad S. cAMP regulation of myosin ATPase activity in the maturing rat heart. Circ Res. 1987 Dec;61(6):914–924. doi: 10.1161/01.res.61.6.914. [DOI] [PubMed] [Google Scholar]
- Kawai M., Güth K., Winnikes K., Haist C., Rüegg J. C. The effect of inorganic phosphate on the ATP hydrolysis rate and the tension transients in chemically skinned rabbit psoas fibers. Pflugers Arch. 1987 Jan;408(1):1–9. doi: 10.1007/BF00581833. [DOI] [PubMed] [Google Scholar]
- Kentish J. C. Combined inhibitory actions of acidosis and phosphate on maximum force production in rat skinned cardiac muscle. Pflugers Arch. 1991 Oct;419(3-4):310–318. doi: 10.1007/BF00371112. [DOI] [PubMed] [Google Scholar]
- Kentish J. C., Nayler W. G. The influence of pH on the Ca2+-regulated ATPase of cardiac and white skeletal myofibrils. J Mol Cell Cardiol. 1979 Jun;11(6):611–617. doi: 10.1016/0022-2828(79)90435-8. [DOI] [PubMed] [Google Scholar]
- Kentish J. C., ter Keurs H. E., Ricciardi L., Bucx J. J., Noble M. I. Comparison between the sarcomere length-force relations of intact and skinned trabeculae from rat right ventricle. Influence of calcium concentrations on these relations. Circ Res. 1986 Jun;58(6):755–768. doi: 10.1161/01.res.58.6.755. [DOI] [PubMed] [Google Scholar]
- Kuhn H. J., Bletz C., Rüegg J. C. Stretch-induced increase in the Ca2+ sensitivity of myofibrillar ATPase activity in skinned fibres from pig ventricles. Pflugers Arch. 1990 Mar;415(6):741–746. doi: 10.1007/BF02584014. [DOI] [PubMed] [Google Scholar]
- Kurebayashi N., Ogawa Y. Discrimination of Ca(2+)-ATPase activity of the sarcoplasmic reticulum from actomyosin-type ATPase activity of myofibrils in skinned mammalian skeletal muscle fibres: distinct effects of cyclopiazonic acid on the two ATPase activities. J Muscle Res Cell Motil. 1991 Aug;12(4):355–365. doi: 10.1007/BF01738590. [DOI] [PubMed] [Google Scholar]
- Kurebayashi N., Ogawa Y. Effect of quercetin on tension development by skinned fibres from frog skeletal muscle. J Muscle Res Cell Motil. 1985 Apr;6(2):189–195. doi: 10.1007/BF00713060. [DOI] [PubMed] [Google Scholar]
- Mulieri L. A., Hasenfuss G., Ittleman F., Blanchard E. M., Alpert N. R. Protection of human left ventricular myocardium from cutting injury with 2,3-butanedione monoxime. Circ Res. 1989 Nov;65(5):1441–1449. doi: 10.1161/01.res.65.5.1441. [DOI] [PubMed] [Google Scholar]
- Niggli E., Lederer W. J. Restoring forces in cardiac myocytes. Insight from relaxations induced by photolysis of caged ATP. Biophys J. 1991 May;59(5):1123–1135. doi: 10.1016/S0006-3495(91)82327-X. [DOI] [PMC free article] [PubMed] [Google Scholar]
- Robinson T. F., Winegrad S. The measurement and dynamic implications of thin filament lengths in heart muscle. J Physiol. 1979 Jan;286:607–619. doi: 10.1113/jphysiol.1979.sp012640. [DOI] [PMC free article] [PubMed] [Google Scholar]
- Seidler N. W., Jona I., Vegh M., Martonosi A. Cyclopiazonic acid is a specific inhibitor of the Ca2+-ATPase of sarcoplasmic reticulum. J Biol Chem. 1989 Oct 25;264(30):17816–17823. [PubMed] [Google Scholar]
- Solaro R. J., Shiner J. S. Modulation of Ca2+ control of dog and rabbit cardiac myofibrils by Mg2+. Comparison with rabbit skeletal myofibrils. Circ Res. 1976 Jul;39(1):8–14. doi: 10.1161/01.res.39.1.8. [DOI] [PubMed] [Google Scholar]
- Stephenson D. G., Stewart A. W., Wilson G. J. Dissociation of force from myofibrillar MgATPase and stiffness at short sarcomere lengths in rat and toad skeletal muscle. J Physiol. 1989 Mar;410:351–366. doi: 10.1113/jphysiol.1989.sp017537. [DOI] [PMC free article] [PubMed] [Google Scholar]
- Stienen G. J., Roosemalen M. C., Wilson M. G., Elzinga G. Depression of force by phosphate in skinned skeletal muscle fibers of the frog. Am J Physiol. 1990 Aug;259(2 Pt 1):C349–C357. doi: 10.1152/ajpcell.1990.259.2.C349. [DOI] [PubMed] [Google Scholar]