Abstract
1. The effects of inorganic phosphate (Pi) and pH on maximal calcium-activated isometric force and MgATPase activity were studied in chemically skinned cardiac trabeculae from rat. ATP hydrolysis was coupled enzymatically to the breakdown of NADH, and its concentration was determined photometrically. Measurements were performed at 2.1 microns sarcomere length and 20 degrees C. ATPase activity and force were also determined when square-wave-shaped length changes were applied, with a frequency of 23 Hz and an amplitude of 2.5%. 2. At pH 7.0 without added Pi, the average isometric force (+/- S.E.M.) was 51 +/- 3 kN m-2 (n = 23). The average isometric ATPase activity was 0.43 +/- 0.02 mM s-1 (n = 23). During the changes in length ATPase activity increased to 152 +/- 3% of the isometric value, while the average force level decreased to 48 +/- 2%. 3. Isometric force gradually decreased to 31 +/- 2% of the control value when the Pi concentration was increased to 30 mM. Isometric ATPase activity, however, remained constant for Pi concentrations up to 5 mM and decreased to 87 +/- 3% at 30 mM Pi. When Pi accumulation inside the preparation due to ATP hydrolysis was taken into account, a linear relationship was found between isometric force and log [Pi]. The decrease in relative force was found to be 44 +/- 4% per decade. 4. During the length changes, ATPase activity and average force showed, apart from the increase in ATPase activity and decrease in average force, the same dependence on Pi as the isometric values. Stiffness, estimated from the amplitude of the force responses during the length changes, decreased in proportion to isometric force when the Pi concentration was increased. The changes in the shape of the force responses due to the repetitive changes in length as a function of the Pi concentration were relatively small. These results suggest that the effect of Pi on the transitions which influence ATP turnover is rather insensitive to changes in cross-bridge strain. 5. Isometric force, normalized to the control value at pH 7.0, increased gradually from 54 +/- 1% at pH 6.2 to 143 +/- 10% at pH 7.5. ATPase activity remained practically constant for pH values from 6.8 to 7.2 but decreased to 80 +/- 1% at pH 6.2 and to 83 +/- 5% at pH 7.5. ATPase activity during the length changes was reduced more than the isometric ATPase activity when pH was lowered.(ABSTRACT TRUNCATED AT 400 WORDS)
Full text
PDF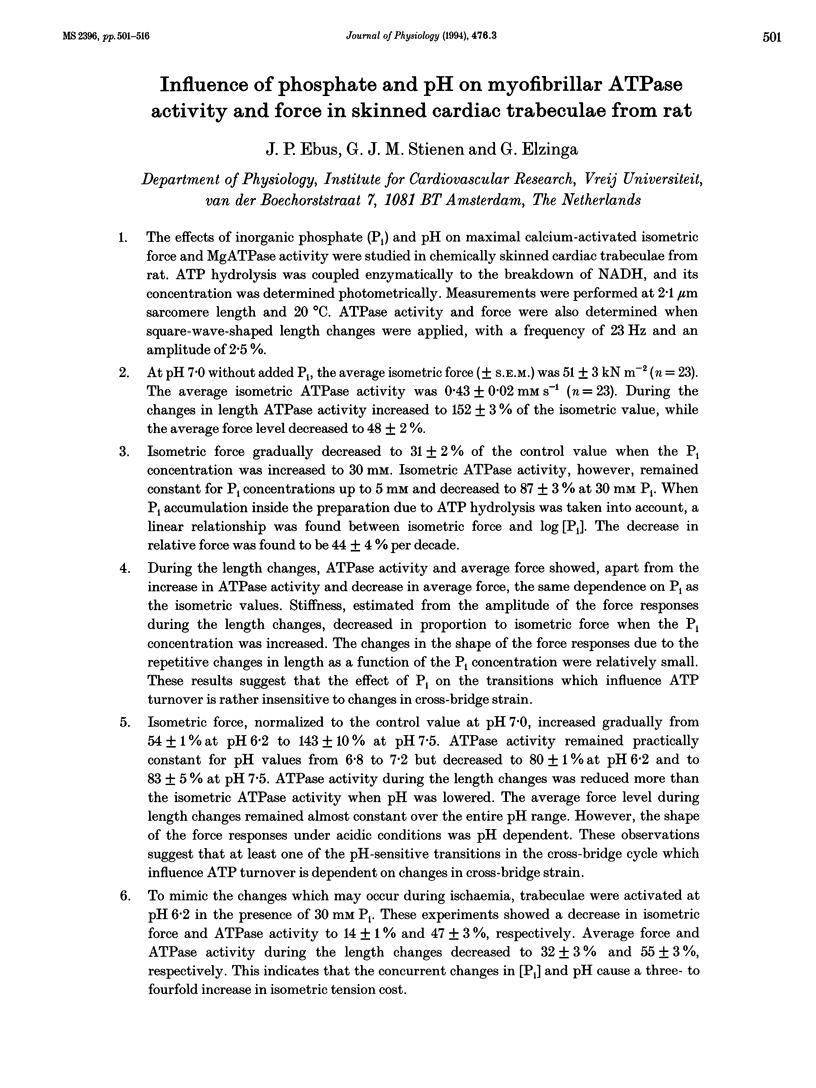
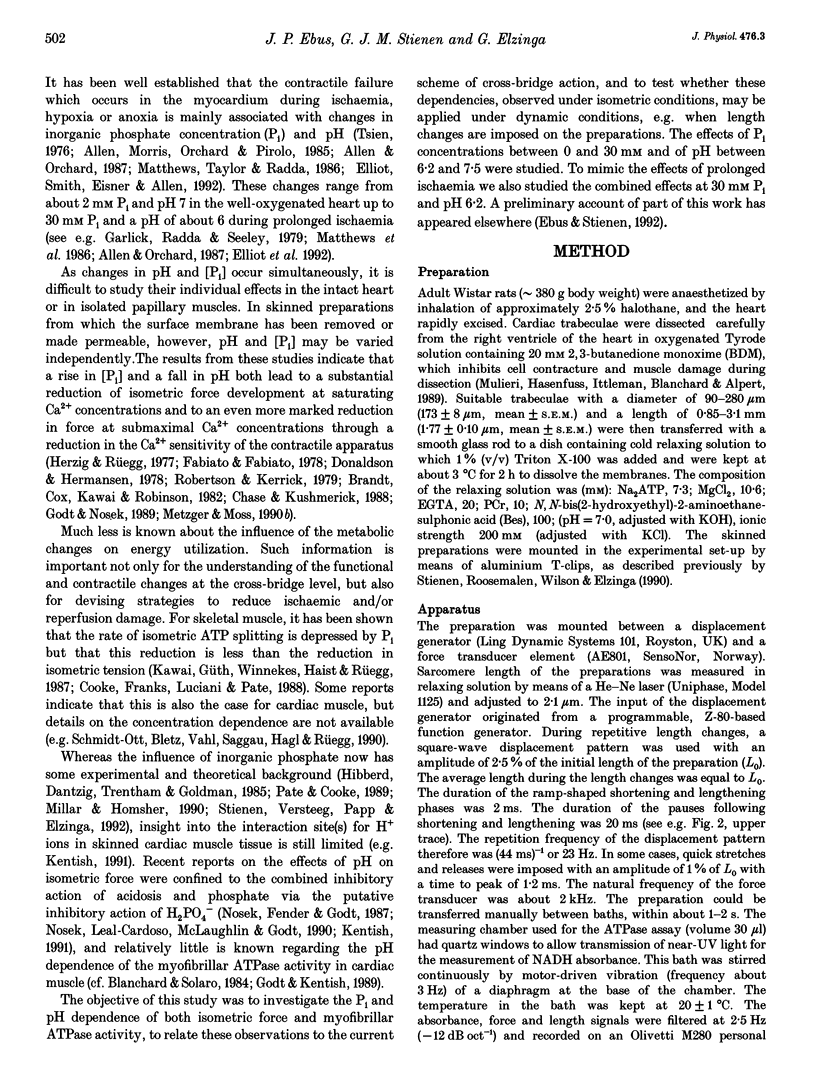
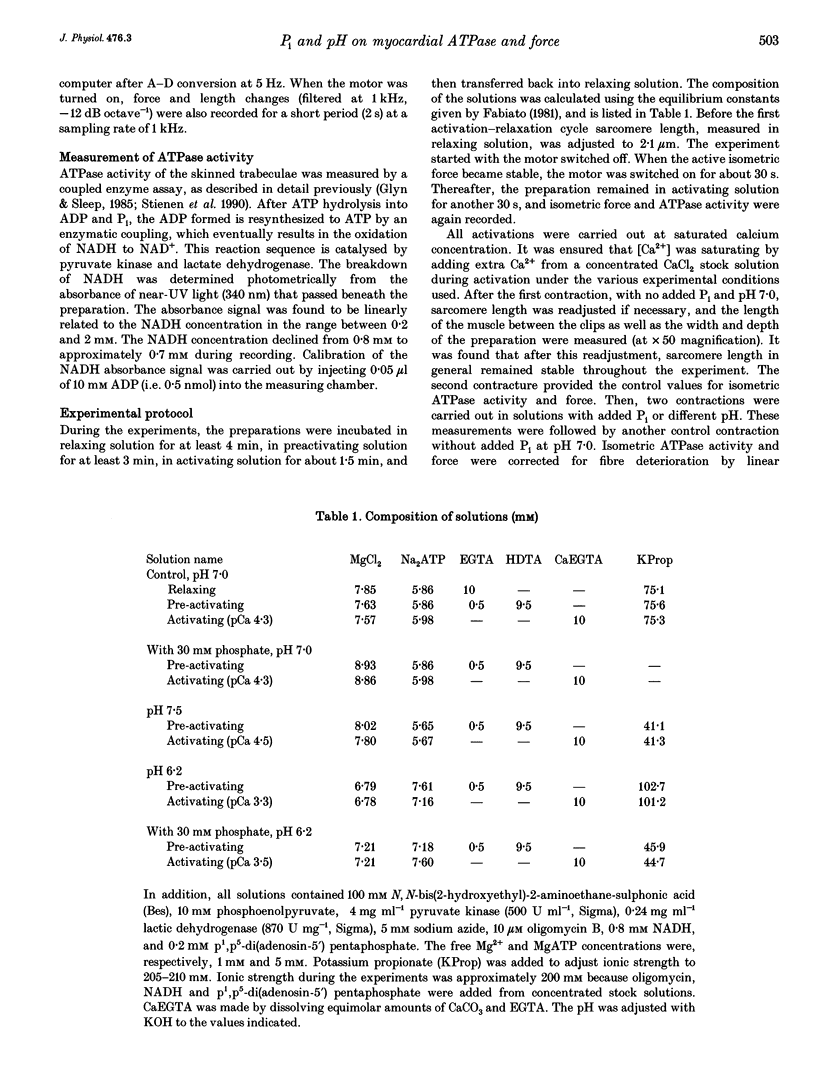
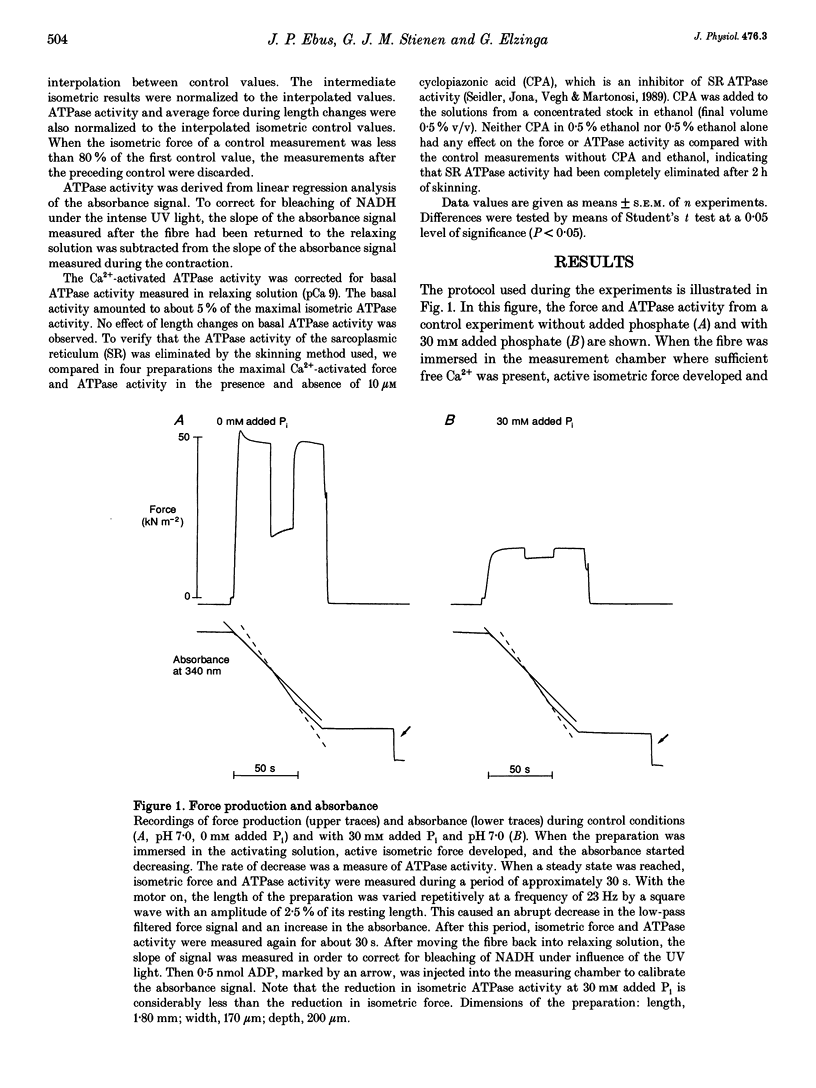
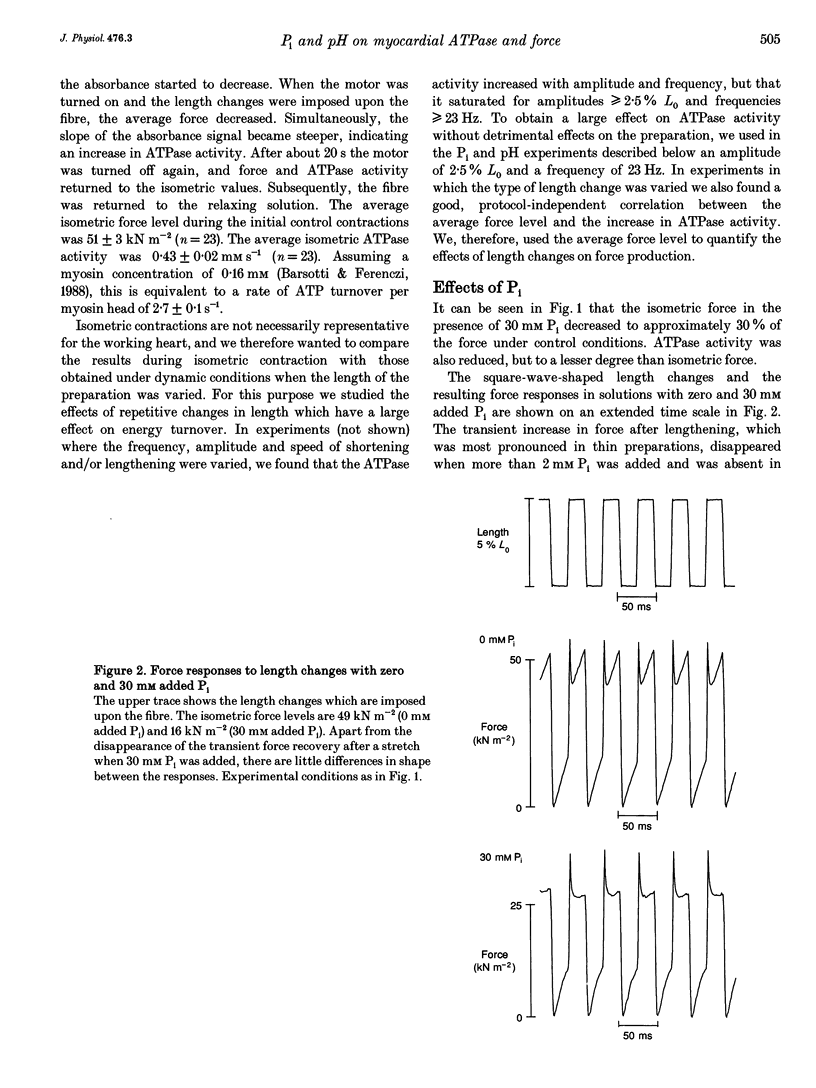
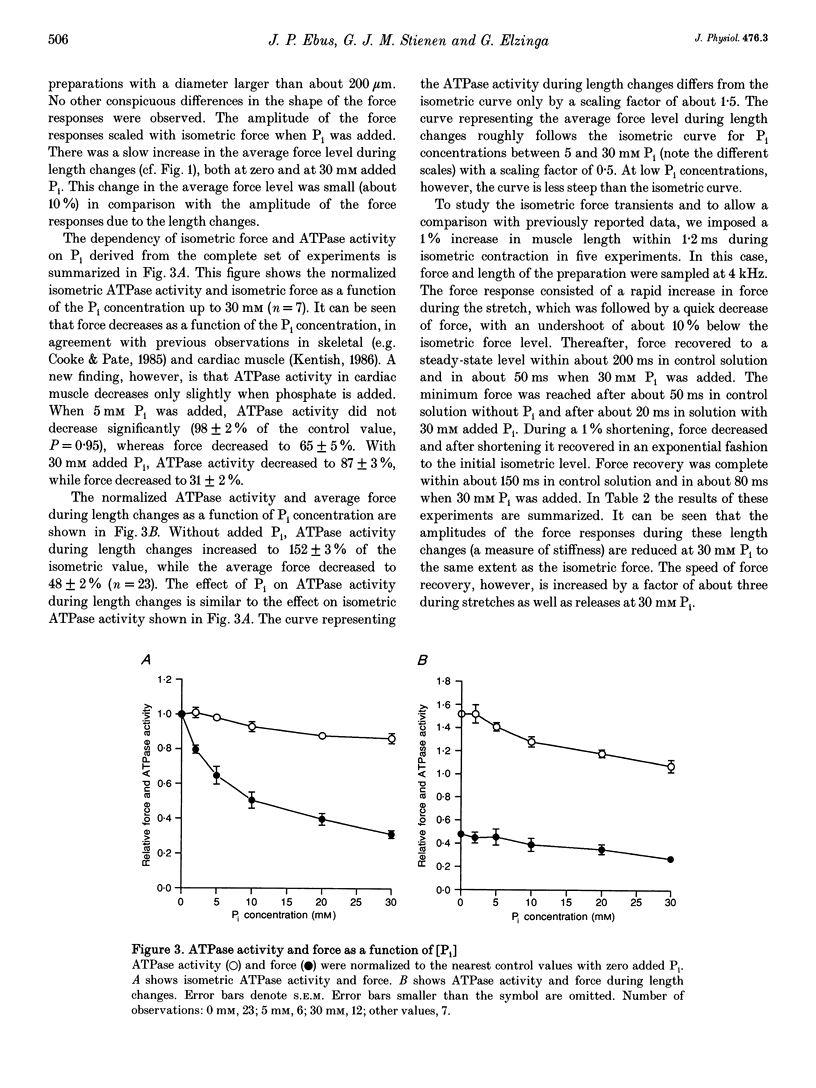
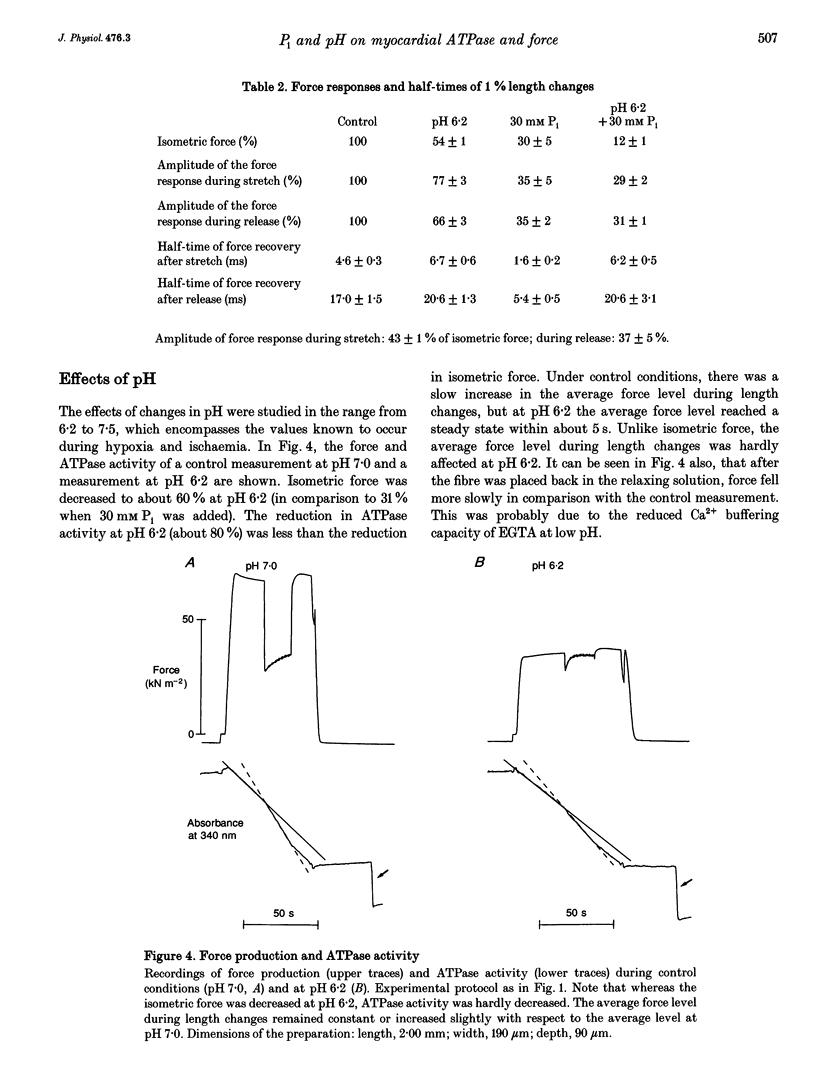
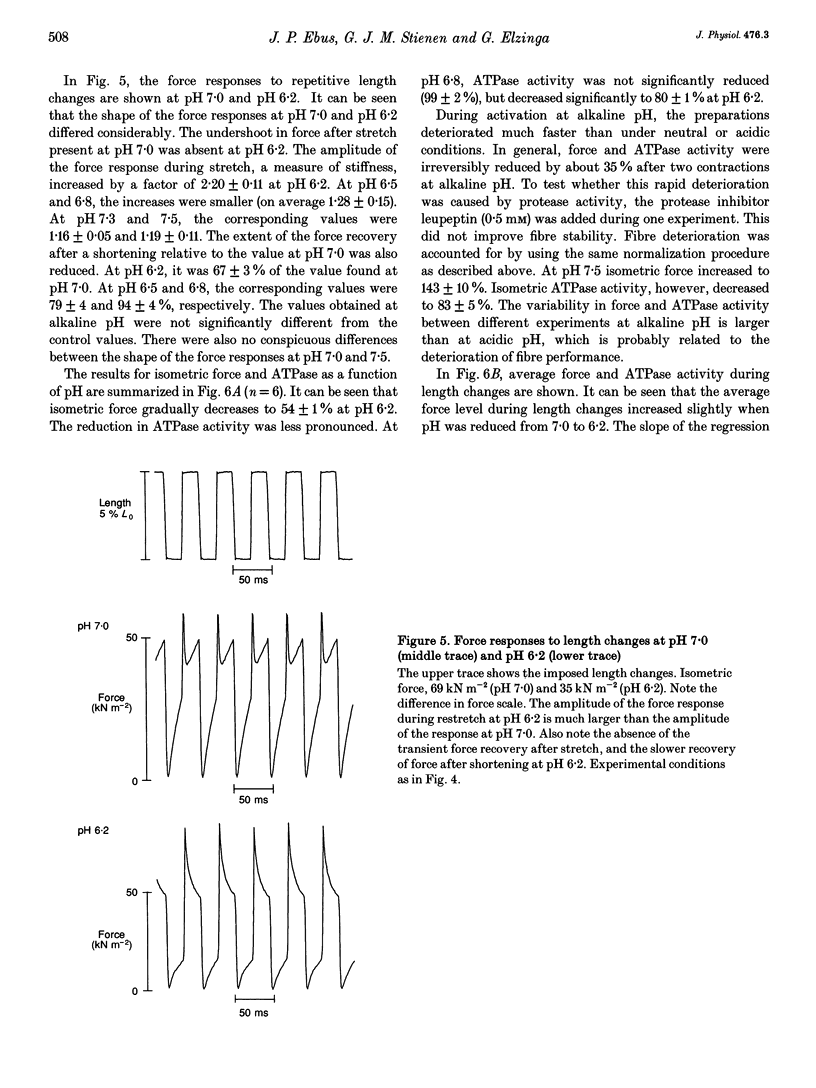
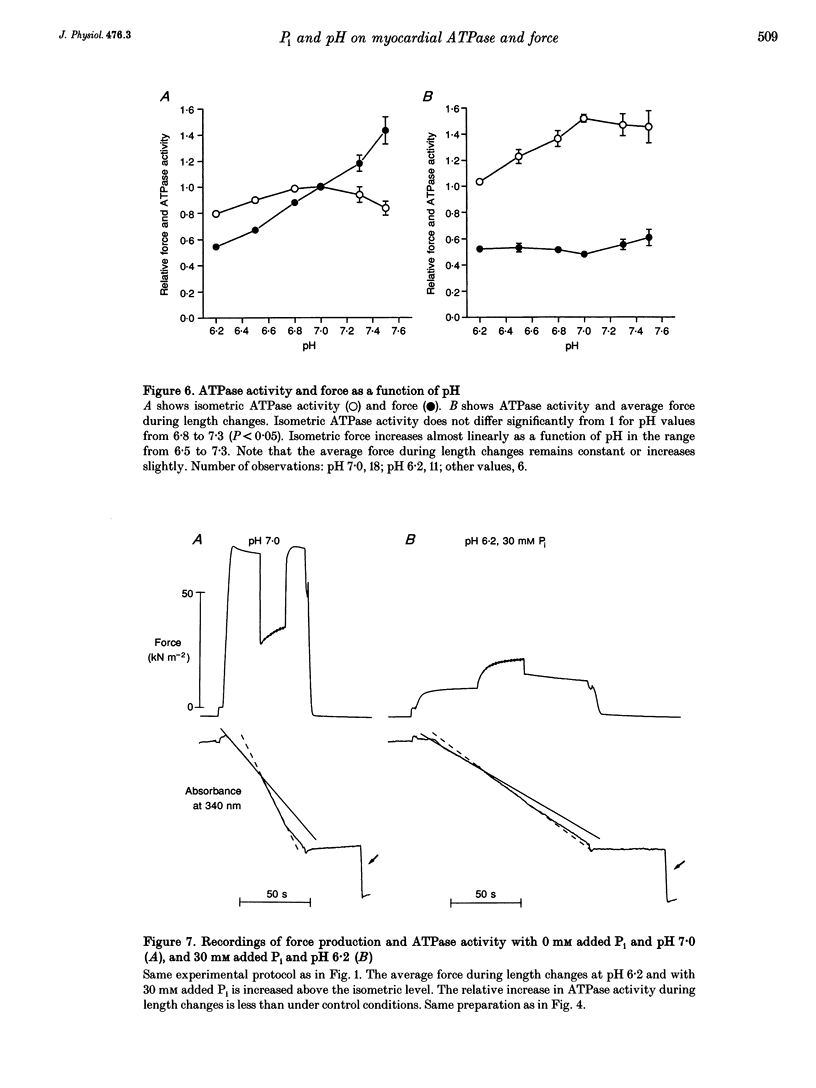
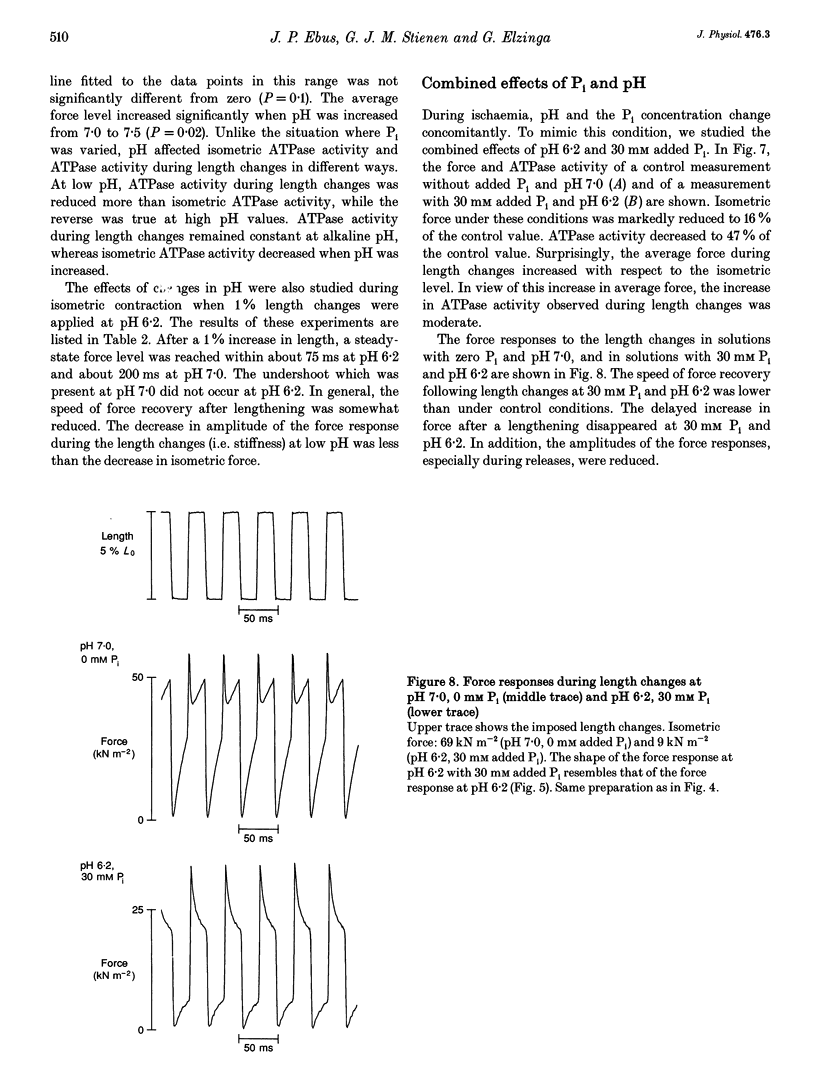
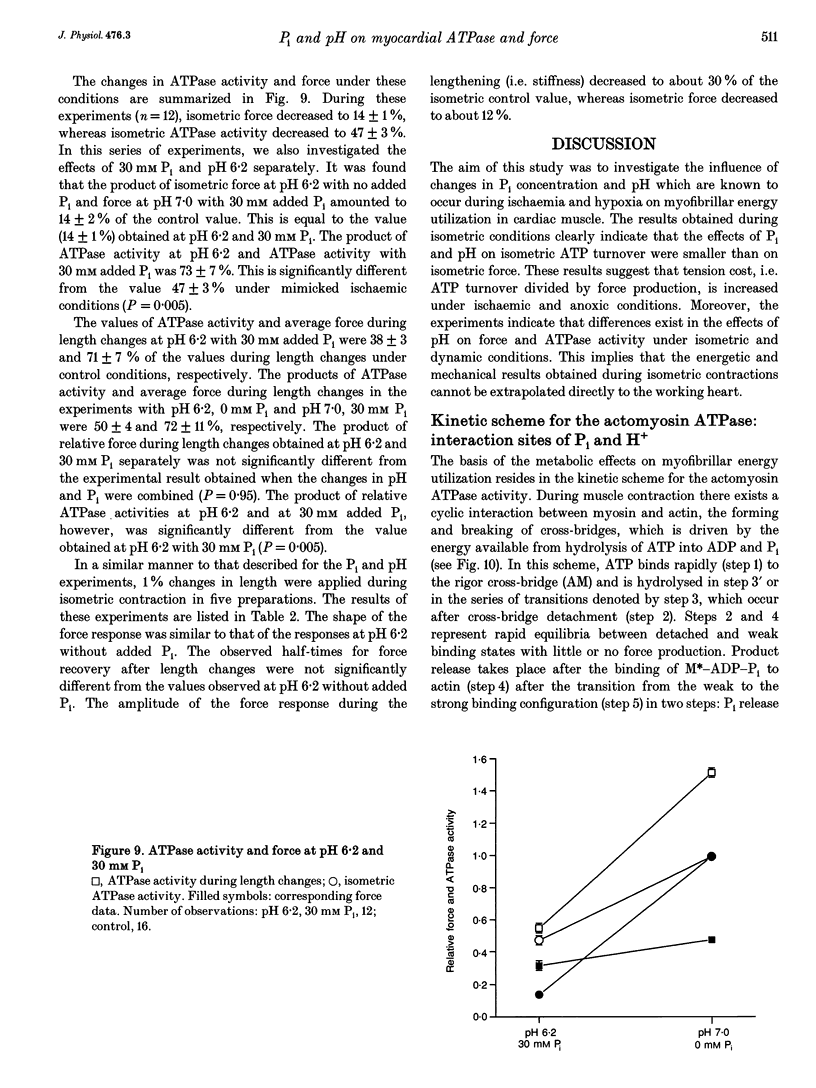
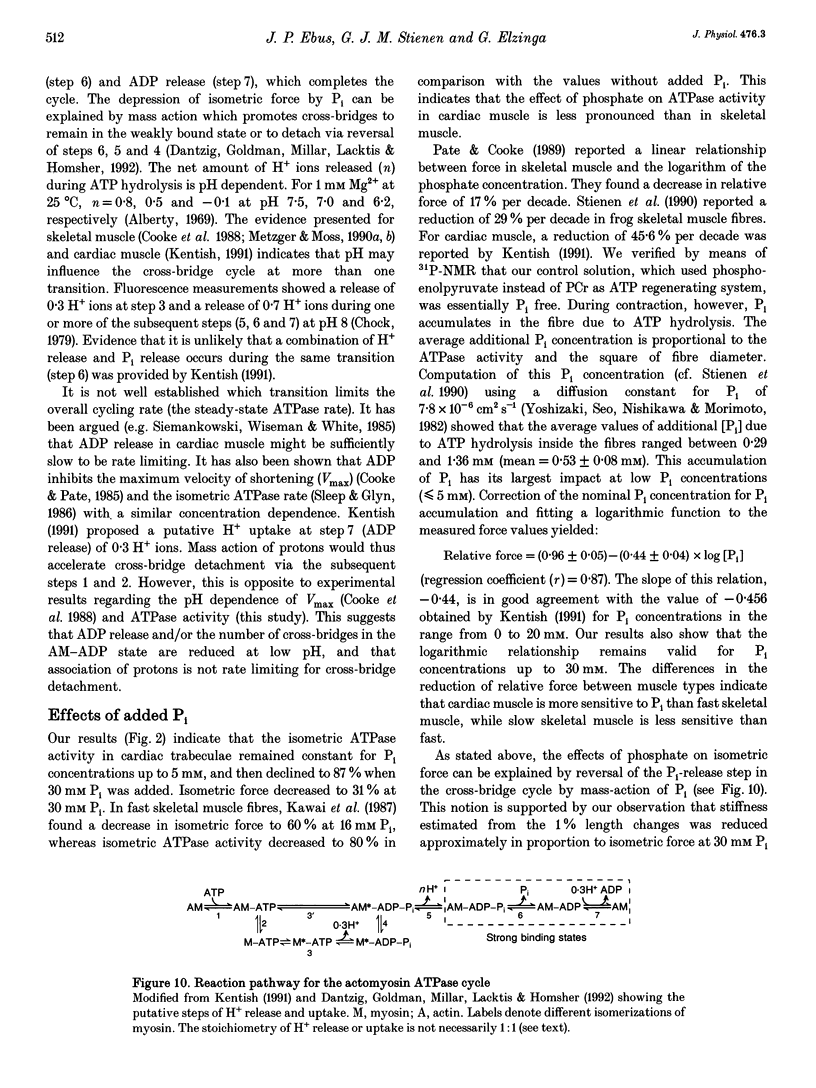
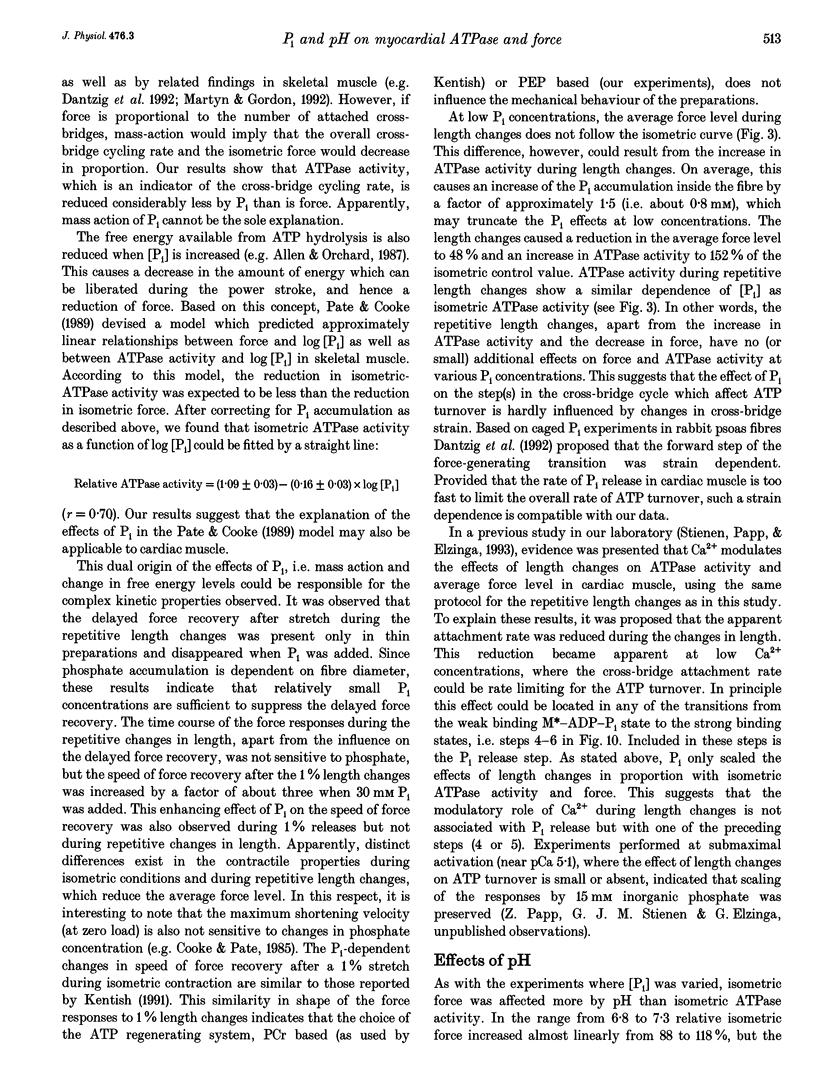
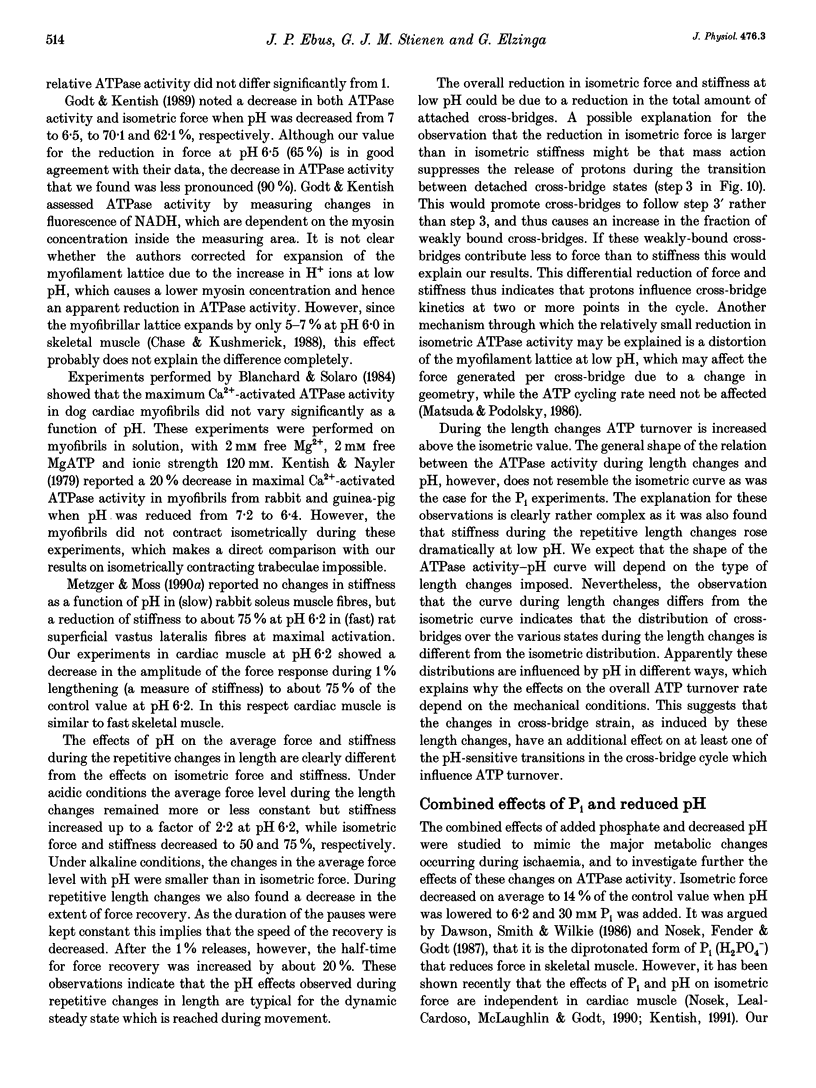
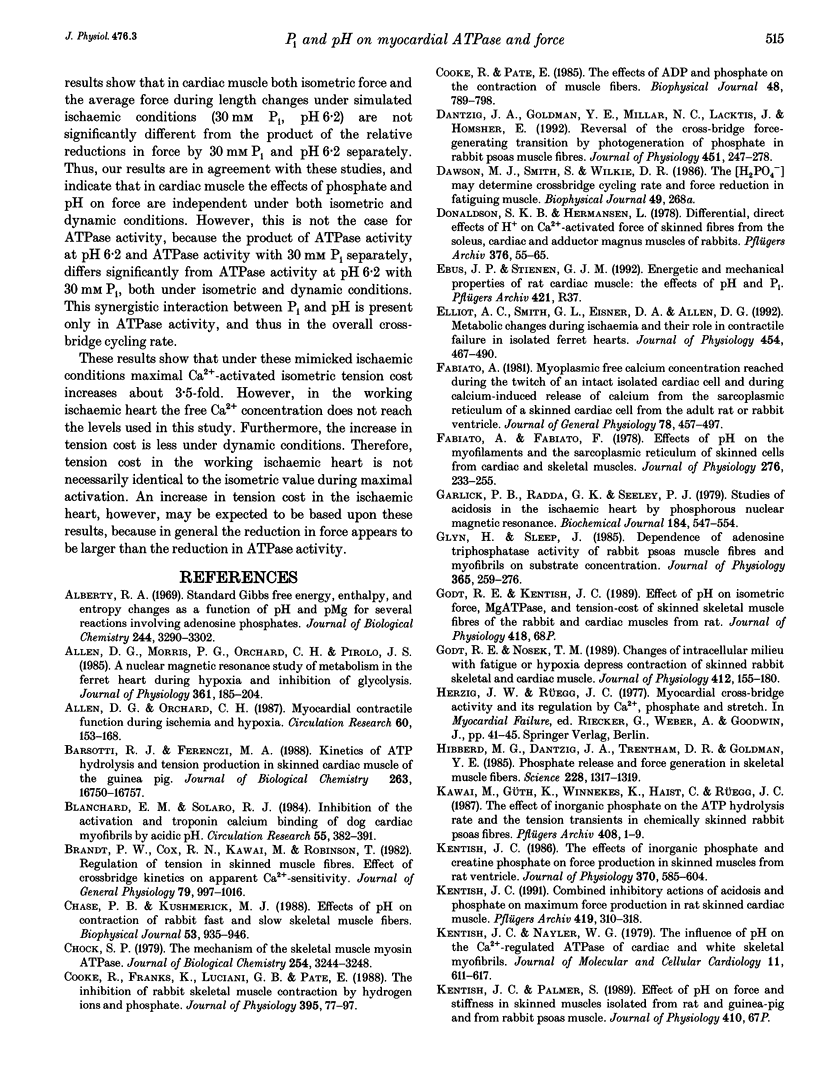
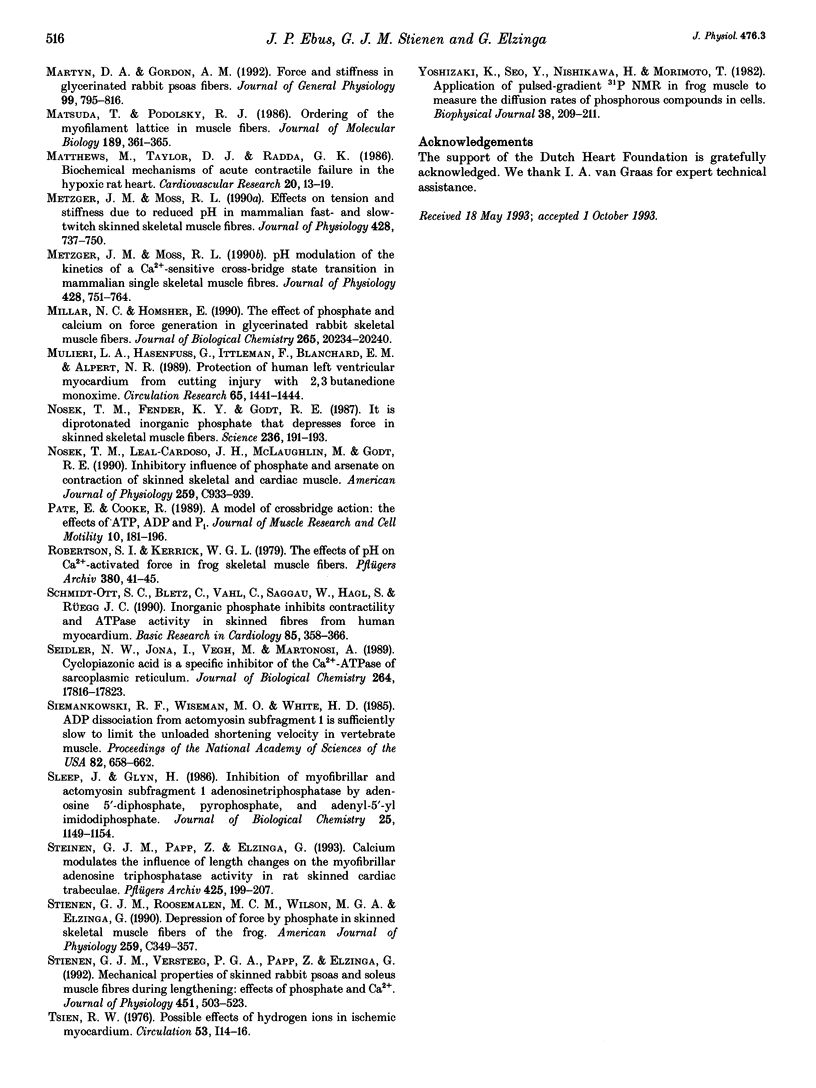
Selected References
These references are in PubMed. This may not be the complete list of references from this article.
- Alberty R. A. Standard Gibbs free energy, enthalpy, and entropy changes as a function of pH and pMg for several reactions involving adenosine phosphates. J Biol Chem. 1969 Jun 25;244(12):3290–3302. [PubMed] [Google Scholar]
- Allen D. G., Morris P. G., Orchard C. H., Pirolo J. S. A nuclear magnetic resonance study of metabolism in the ferret heart during hypoxia and inhibition of glycolysis. J Physiol. 1985 Apr;361:185–204. doi: 10.1113/jphysiol.1985.sp015640. [DOI] [PMC free article] [PubMed] [Google Scholar]
- Allen D. G., Orchard C. H. Myocardial contractile function during ischemia and hypoxia. Circ Res. 1987 Feb;60(2):153–168. doi: 10.1161/01.res.60.2.153. [DOI] [PubMed] [Google Scholar]
- Barsotti R. J., Ferenczi M. A. Kinetics of ATP hydrolysis and tension production in skinned cardiac muscle of the guinea pig. J Biol Chem. 1988 Nov 15;263(32):16750–16756. [PubMed] [Google Scholar]
- Blanchard E. M., Solaro R. J. Inhibition of the activation and troponin calcium binding of dog cardiac myofibrils by acidic pH. Circ Res. 1984 Sep;55(3):382–391. doi: 10.1161/01.res.55.3.382. [DOI] [PubMed] [Google Scholar]
- Bosma M. M. Anion channels with multiple conductance levels in a mouse B lymphocyte cell line. J Physiol. 1989 Mar;410:67–90. doi: 10.1113/jphysiol.1989.sp017521. [DOI] [PMC free article] [PubMed] [Google Scholar]
- Brandt P. W., Cox R. N., Kawai M., Robinson T. Effect of cross-bridge kinetics on apparent Ca2+ sensitivity. J Gen Physiol. 1982 Jun;79(6):997–1016. doi: 10.1085/jgp.79.6.997. [DOI] [PMC free article] [PubMed] [Google Scholar]
- Chase P. B., Kushmerick M. J. Effects of pH on contraction of rabbit fast and slow skeletal muscle fibers. Biophys J. 1988 Jun;53(6):935–946. doi: 10.1016/S0006-3495(88)83174-6. [DOI] [PMC free article] [PubMed] [Google Scholar]
- Chock S. P. The mechanism of the skeletal muscle myosin ATPase. III. Relationship of the H+ release and the protein absorbance change induced by ATP to the initial Pi burst. J Biol Chem. 1979 May 10;254(9):3244–3248. [PubMed] [Google Scholar]
- Cooke R., Franks K., Luciani G. B., Pate E. The inhibition of rabbit skeletal muscle contraction by hydrogen ions and phosphate. J Physiol. 1988 Jan;395:77–97. doi: 10.1113/jphysiol.1988.sp016909. [DOI] [PMC free article] [PubMed] [Google Scholar]
- Cooke R., Pate E. The effects of ADP and phosphate on the contraction of muscle fibers. Biophys J. 1985 Nov;48(5):789–798. doi: 10.1016/S0006-3495(85)83837-6. [DOI] [PMC free article] [PubMed] [Google Scholar]
- Dantzig J. A., Goldman Y. E., Millar N. C., Lacktis J., Homsher E. Reversal of the cross-bridge force-generating transition by photogeneration of phosphate in rabbit psoas muscle fibres. J Physiol. 1992;451:247–278. doi: 10.1113/jphysiol.1992.sp019163. [DOI] [PMC free article] [PubMed] [Google Scholar]
- Donaldson S. K., Hermansen L., Bolles L. Differential, direct effects of H+ on Ca2+ -activated force of skinned fibers from the soleus, cardiac and adductor magnus muscles of rabbits. Pflugers Arch. 1978 Aug 25;376(1):55–65. doi: 10.1007/BF00585248. [DOI] [PubMed] [Google Scholar]
- Elliott A. C., Smith G. L., Eisner D. A., Allen D. G. Metabolic changes during ischaemia and their role in contractile failure in isolated ferret hearts. J Physiol. 1992 Aug;454:467–490. doi: 10.1113/jphysiol.1992.sp019274. [DOI] [PMC free article] [PubMed] [Google Scholar]
- Fabiato A., Fabiato F. Effects of pH on the myofilaments and the sarcoplasmic reticulum of skinned cells from cardiace and skeletal muscles. J Physiol. 1978 Mar;276:233–255. doi: 10.1113/jphysiol.1978.sp012231. [DOI] [PMC free article] [PubMed] [Google Scholar]
- Fabiato A. Myoplasmic free calcium concentration reached during the twitch of an intact isolated cardiac cell and during calcium-induced release of calcium from the sarcoplasmic reticulum of a skinned cardiac cell from the adult rat or rabbit ventricle. J Gen Physiol. 1981 Nov;78(5):457–497. doi: 10.1085/jgp.78.5.457. [DOI] [PMC free article] [PubMed] [Google Scholar]
- Garlick P. B., Radda G. K., Seeley P. J. Studies of acidosis in the ischaemic heart by phosphorus nuclear magnetic resonance. Biochem J. 1979 Dec 15;184(3):547–554. doi: 10.1042/bj1840547. [DOI] [PMC free article] [PubMed] [Google Scholar]
- Glyn H., Sleep J. Dependence of adenosine triphosphatase activity of rabbit psoas muscle fibres and myofibrils on substrate concentration. J Physiol. 1985 Aug;365:259–276. doi: 10.1113/jphysiol.1985.sp015770. [DOI] [PMC free article] [PubMed] [Google Scholar]
- Godt R. E., Nosek T. M. Changes of intracellular milieu with fatigue or hypoxia depress contraction of skinned rabbit skeletal and cardiac muscle. J Physiol. 1989 May;412:155–180. doi: 10.1113/jphysiol.1989.sp017609. [DOI] [PMC free article] [PubMed] [Google Scholar]
- Hibberd M. G., Dantzig J. A., Trentham D. R., Goldman Y. E. Phosphate release and force generation in skeletal muscle fibers. Science. 1985 Jun 14;228(4705):1317–1319. doi: 10.1126/science.3159090. [DOI] [PubMed] [Google Scholar]
- Kawai M., Güth K., Winnikes K., Haist C., Rüegg J. C. The effect of inorganic phosphate on the ATP hydrolysis rate and the tension transients in chemically skinned rabbit psoas fibers. Pflugers Arch. 1987 Jan;408(1):1–9. doi: 10.1007/BF00581833. [DOI] [PubMed] [Google Scholar]
- Kentish J. C. Combined inhibitory actions of acidosis and phosphate on maximum force production in rat skinned cardiac muscle. Pflugers Arch. 1991 Oct;419(3-4):310–318. doi: 10.1007/BF00371112. [DOI] [PubMed] [Google Scholar]
- Kentish J. C., Nayler W. G. The influence of pH on the Ca2+-regulated ATPase of cardiac and white skeletal myofibrils. J Mol Cell Cardiol. 1979 Jun;11(6):611–617. doi: 10.1016/0022-2828(79)90435-8. [DOI] [PubMed] [Google Scholar]
- Kentish J. C. The effects of inorganic phosphate and creatine phosphate on force production in skinned muscles from rat ventricle. J Physiol. 1986 Jan;370:585–604. doi: 10.1113/jphysiol.1986.sp015952. [DOI] [PMC free article] [PubMed] [Google Scholar]
- Martyn D. A., Gordon A. M. Force and stiffness in glycerinated rabbit psoas fibers. Effects of calcium and elevated phosphate. J Gen Physiol. 1992 May;99(5):795–816. doi: 10.1085/jgp.99.5.795. [DOI] [PMC free article] [PubMed] [Google Scholar]
- Matsuda T., Podolsky R. J. Ordering of the myofilament lattice in muscle fibers. J Mol Biol. 1986 May 20;189(2):361–365. doi: 10.1016/0022-2836(86)90516-4. [DOI] [PubMed] [Google Scholar]
- Matthews P. M., Taylor D. J., Radda G. K. Biochemical mechanisms of acute contractile failure in the hypoxic rat heart. Cardiovasc Res. 1986 Jan;20(1):13–19. doi: 10.1093/cvr/20.1.13. [DOI] [PubMed] [Google Scholar]
- Metzger J. M., Moss R. L. Effects of tension and stiffness due to reduced pH in mammalian fast- and slow-twitch skinned skeletal muscle fibres. J Physiol. 1990 Sep;428:737–750. doi: 10.1113/jphysiol.1990.sp018238. [DOI] [PMC free article] [PubMed] [Google Scholar]
- Metzger J. M., Moss R. L. pH modulation of the kinetics of a Ca2(+)-sensitive cross-bridge state transition in mammalian single skeletal muscle fibres. J Physiol. 1990 Sep;428:751–764. doi: 10.1113/jphysiol.1990.sp018239. [DOI] [PMC free article] [PubMed] [Google Scholar]
- Millar N. C., Homsher E. The effect of phosphate and calcium on force generation in glycerinated rabbit skeletal muscle fibers. A steady-state and transient kinetic study. J Biol Chem. 1990 Nov 25;265(33):20234–20240. [PubMed] [Google Scholar]
- Mulieri L. A., Hasenfuss G., Ittleman F., Blanchard E. M., Alpert N. R. Protection of human left ventricular myocardium from cutting injury with 2,3-butanedione monoxime. Circ Res. 1989 Nov;65(5):1441–1449. doi: 10.1161/01.res.65.5.1441. [DOI] [PubMed] [Google Scholar]
- Nosek T. M., Fender K. Y., Godt R. E. It is diprotonated inorganic phosphate that depresses force in skinned skeletal muscle fibers. Science. 1987 Apr 10;236(4798):191–193. doi: 10.1126/science.3563496. [DOI] [PubMed] [Google Scholar]
- Nosek T. M., Leal-Cardoso J. H., McLaughlin M., Godt R. E. Inhibitory influence of phosphate and arsenate on contraction of skinned skeletal and cardiac muscle. Am J Physiol. 1990 Dec;259(6 Pt 1):C933–C939. doi: 10.1152/ajpcell.1990.259.6.C933. [DOI] [PubMed] [Google Scholar]
- Pate E., Cooke R. A model of crossbridge action: the effects of ATP, ADP and Pi. J Muscle Res Cell Motil. 1989 Jun;10(3):181–196. doi: 10.1007/BF01739809. [DOI] [PubMed] [Google Scholar]
- Robertson S. P., Kerrick W. G. The effects of pH on Ca2+-activated force in frog skeletal muscle fibers. Pflugers Arch. 1979 May 15;380(1):41–45. doi: 10.1007/BF00582610. [DOI] [PubMed] [Google Scholar]
- Schmidt-Ott S. C., Bletz C., Vahl C., Saggau W., Hagl S., Rüegg J. C. Inorganic phosphate inhibits contractility and ATPase activity in skinned fibers from human myocardium. Basic Res Cardiol. 1990 Jul-Aug;85(4):358–366. doi: 10.1007/BF01907128. [DOI] [PubMed] [Google Scholar]
- Seidler N. W., Jona I., Vegh M., Martonosi A. Cyclopiazonic acid is a specific inhibitor of the Ca2+-ATPase of sarcoplasmic reticulum. J Biol Chem. 1989 Oct 25;264(30):17816–17823. [PubMed] [Google Scholar]
- Siemankowski R. F., Wiseman M. O., White H. D. ADP dissociation from actomyosin subfragment 1 is sufficiently slow to limit the unloaded shortening velocity in vertebrate muscle. Proc Natl Acad Sci U S A. 1985 Feb;82(3):658–662. doi: 10.1073/pnas.82.3.658. [DOI] [PMC free article] [PubMed] [Google Scholar]
- Sleep J., Glyn H. Inhibition of myofibrillar and actomyosin subfragment 1 adenosinetriphosphatase by adenosine 5'-diphosphate, pyrophosphate, and adenyl-5'-yl imidodiphosphate. Biochemistry. 1986 Mar 11;25(5):1149–1154. doi: 10.1021/bi00353a030. [DOI] [PubMed] [Google Scholar]
- Stienen G. J., Papp Z., Elzinga G. Calcium modulates the influence of length changes on the myofibrillar adenosine triphosphatase activity in rat skinned cardiac trabeculae. Pflugers Arch. 1993 Nov;425(3-4):199–207. doi: 10.1007/BF00374167. [DOI] [PubMed] [Google Scholar]
- Stienen G. J., Roosemalen M. C., Wilson M. G., Elzinga G. Depression of force by phosphate in skinned skeletal muscle fibers of the frog. Am J Physiol. 1990 Aug;259(2 Pt 1):C349–C357. doi: 10.1152/ajpcell.1990.259.2.C349. [DOI] [PubMed] [Google Scholar]
- Stienen G. J., Versteeg P. G., Papp Z., Elzinga G. Mechanical properties of skinned rabbit psoas and soleus muscle fibres during lengthening: effects of phosphate and Ca2+. J Physiol. 1992;451:503–523. doi: 10.1113/jphysiol.1992.sp019176. [DOI] [PMC free article] [PubMed] [Google Scholar]
- Yoshizaki K., Seo Y., Nishikawa H., Morimoto T. Application of pulsed-gradient 31P NMR on frog muscle to measure the diffusion rates of phosphorus compounds in cells. Biophys J. 1982 May;38(2):209–211. doi: 10.1016/S0006-3495(82)84549-9. [DOI] [PMC free article] [PubMed] [Google Scholar]