Abstract
1. Muscle birefringence, the difference between the refractive indices of light polarized parallel and perpendicular to the muscle fibre axis, was measured at 3 degrees C in intact single fibres isolated from frog muscle. Resting birefringence was 2.20 +/- 0.02 x 10(-3) (mean +/- S.E.M., n = 44) at sarcomere length 2.4-2.7 microns and 2.35 +/- 0.03 x 10(-3) (n = 19) at 3.5-3.8 microns. 2. Birefringence decreased during isometric twitch or tetanic contractions. The peak change in a twitch at sarcomere length 2.6 microns, determined by two independent methods, was 0.150 +/- 0.017 x 10(-3) (mean +/- S.E.M., n = 6). The corresponding value after 0.4 s of tetanic stimulation was 0.167 +/- 0.012 x 10(-3) (n = 6). 3. The birefringence change had a shorter latency than tension and reached its half-maximum value earlier than tension. The difference in time to half-maximum in tetani was 11.5 +/- 1.3 ms (mean +/- S.E.M., n = 6) at 3 degrees C. After stimulation birefringence recovered to its pre-stimulus baseline more slowly than tension. 4. The birefringence decrease after 0.4 s of tetanic stimulation was linearly related to the expected degree of overlap between actin and myosin filaments in the sarcomere length range 2.6-3.6 microns. The amplitude of the birefringence decrease at full filament overlap (sarcomere length 2.2 microns) was estimated to be 0.235 +/- 0.015 x 10(-3). 5. Birefringence changes associated with shortening steps of 0.9% fibre length at sarcomere length 2.6 microns exhibited four phases corresponding to those of the tension transient. There was no consistent birefringence change during the length step itself. During the rapid tension recovery birefringence increased by 0.014 +/- 0.001 x 10(-3) (n = 3), measured from the end of the length step to 2 ms later. Birefringence continued to increase as tension recovery slowed, reaching a peak about 10 ms after the step, then recovered with a rate similar to that of the final tension recovery. 6. These birefringence changes are likely to be caused by axial rotation of the head domain of the myosin cross-bridge. During isometric contraction heads bind to actin with their long axes more perpendicular to the fibre axis than in resting muscle, although there is likely to be a wide range of head orientations during contraction.(ABSTRACT TRUNCATED AT 400 WORDS)
Full text
PDF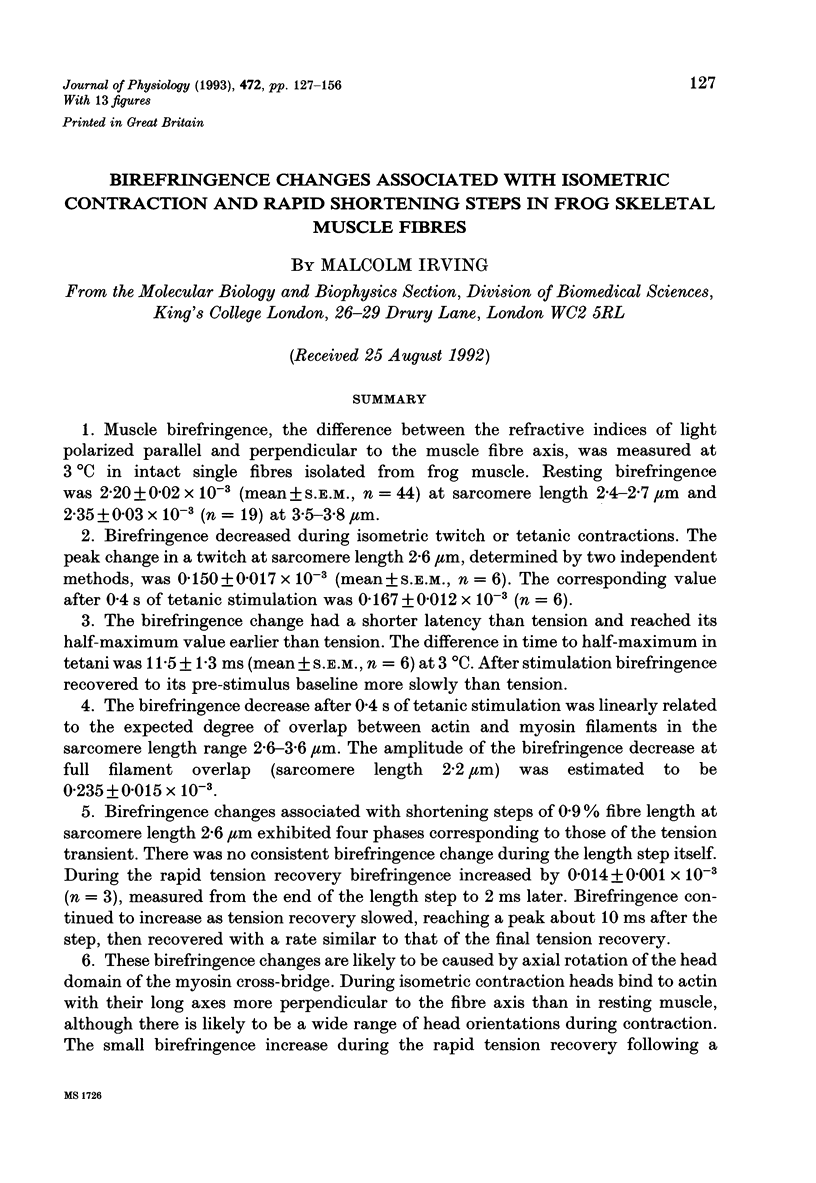
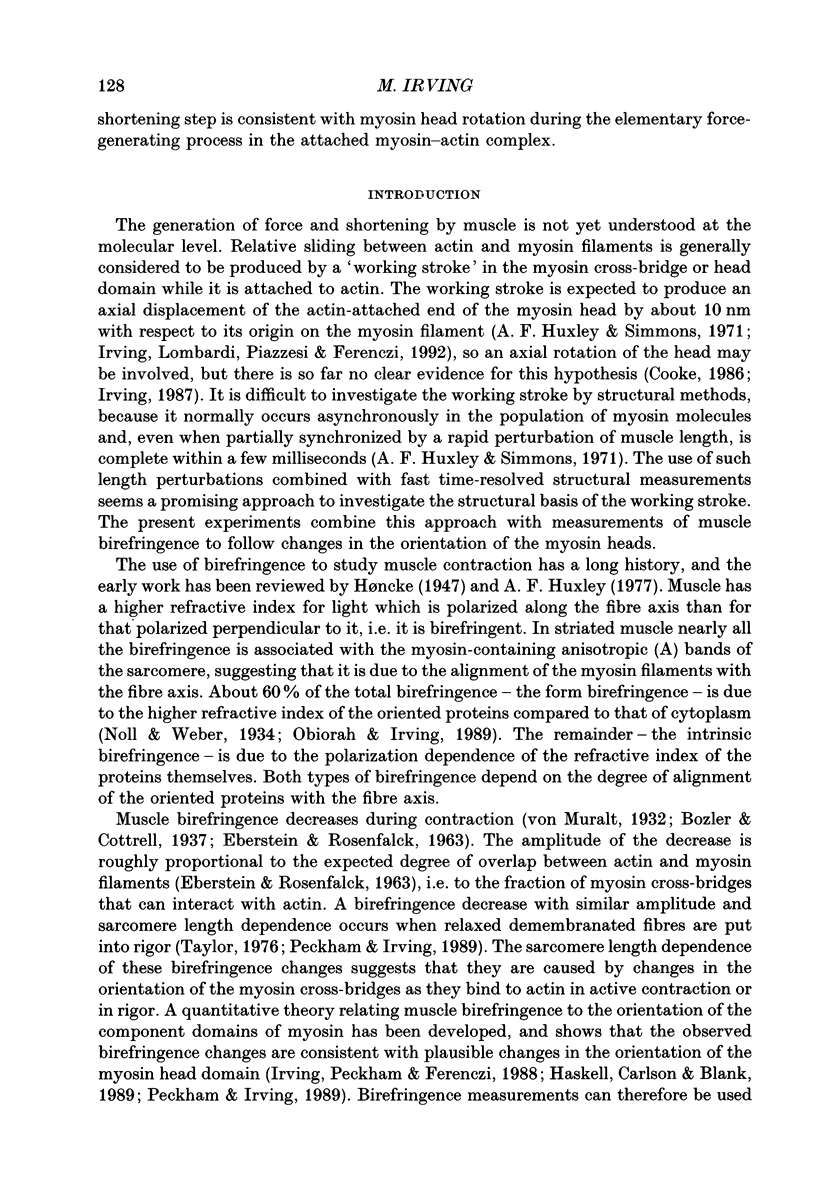
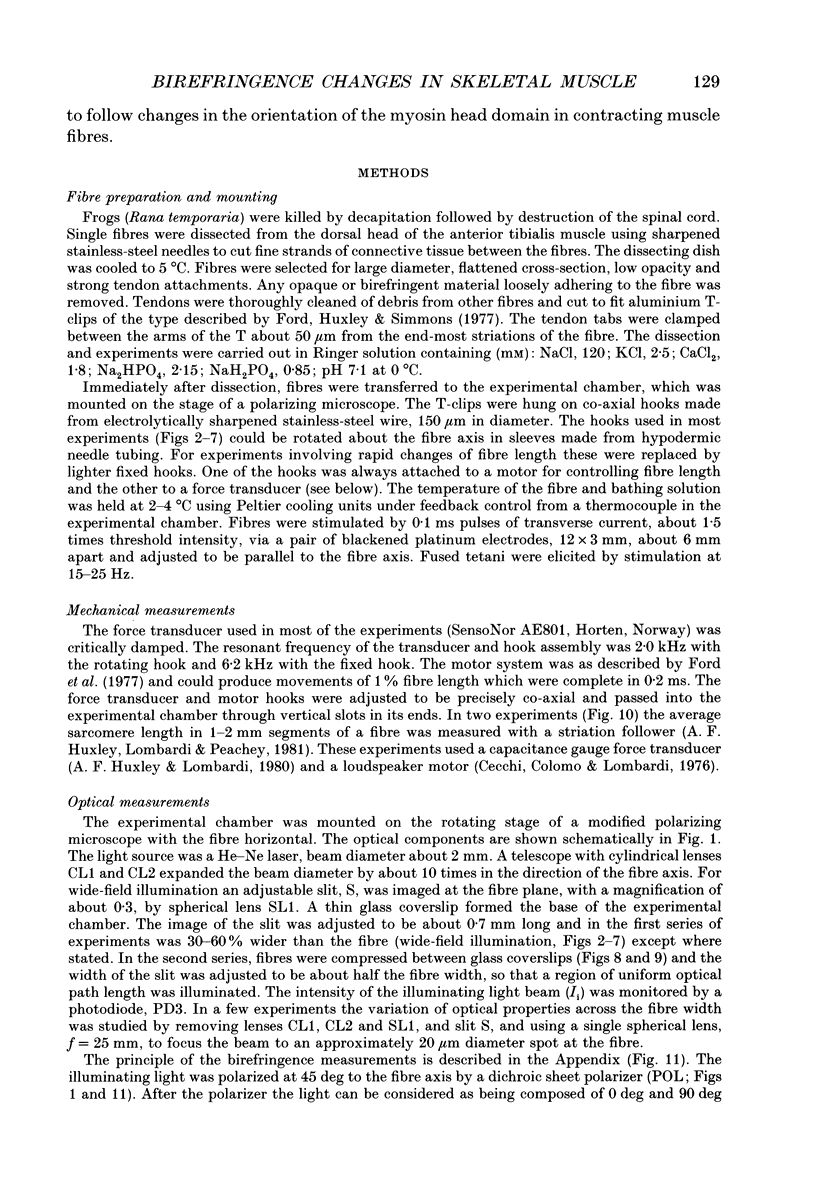
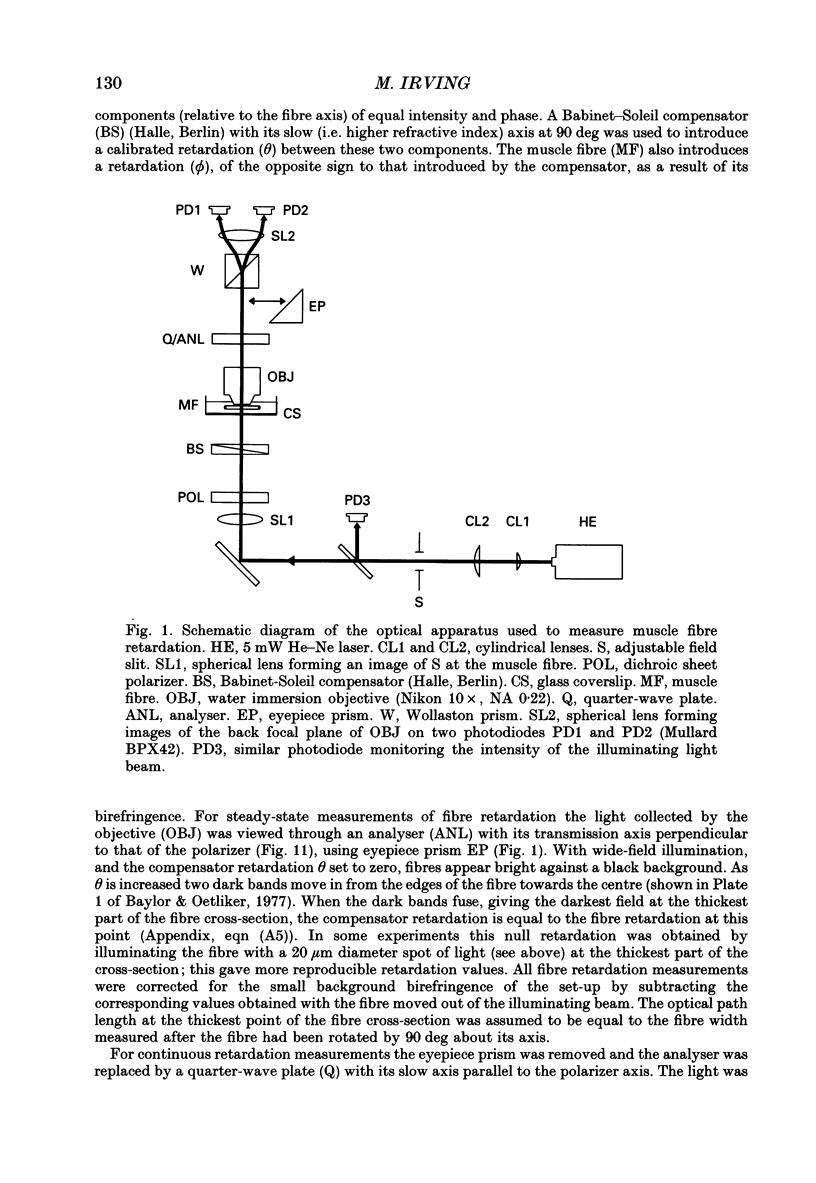
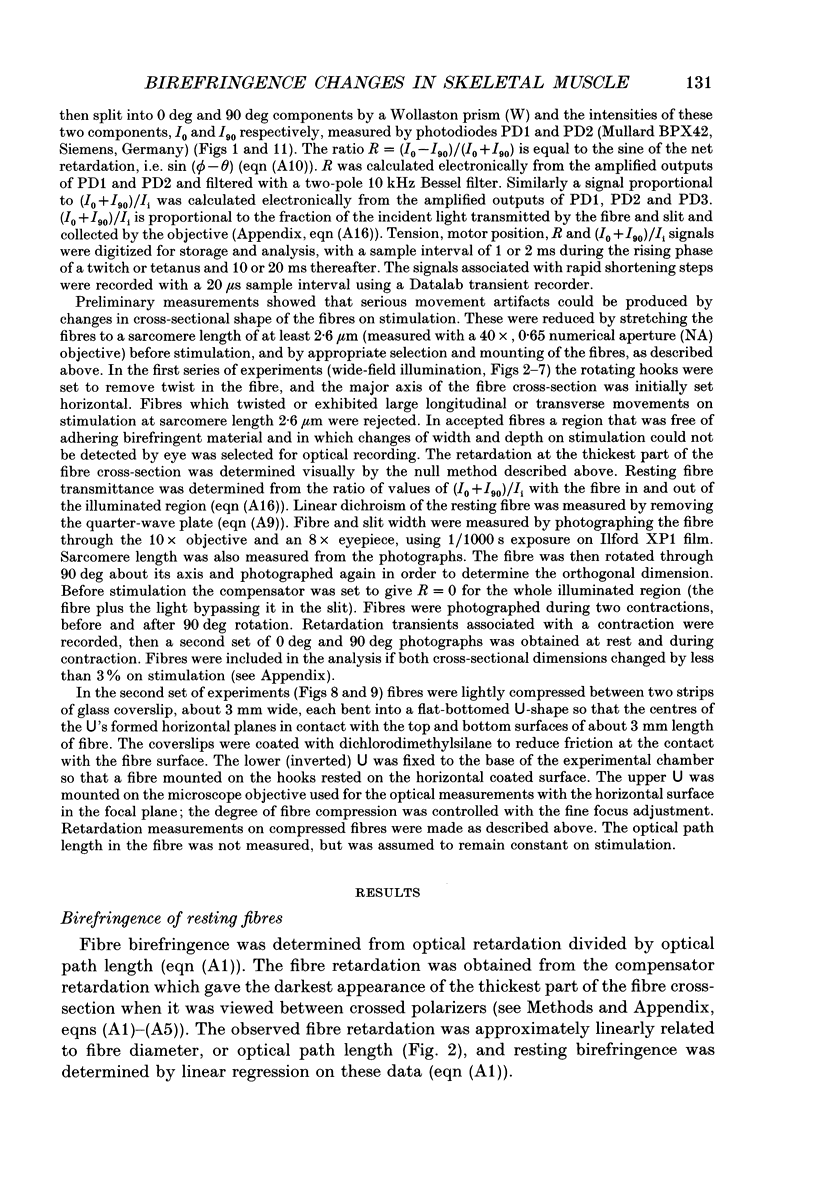
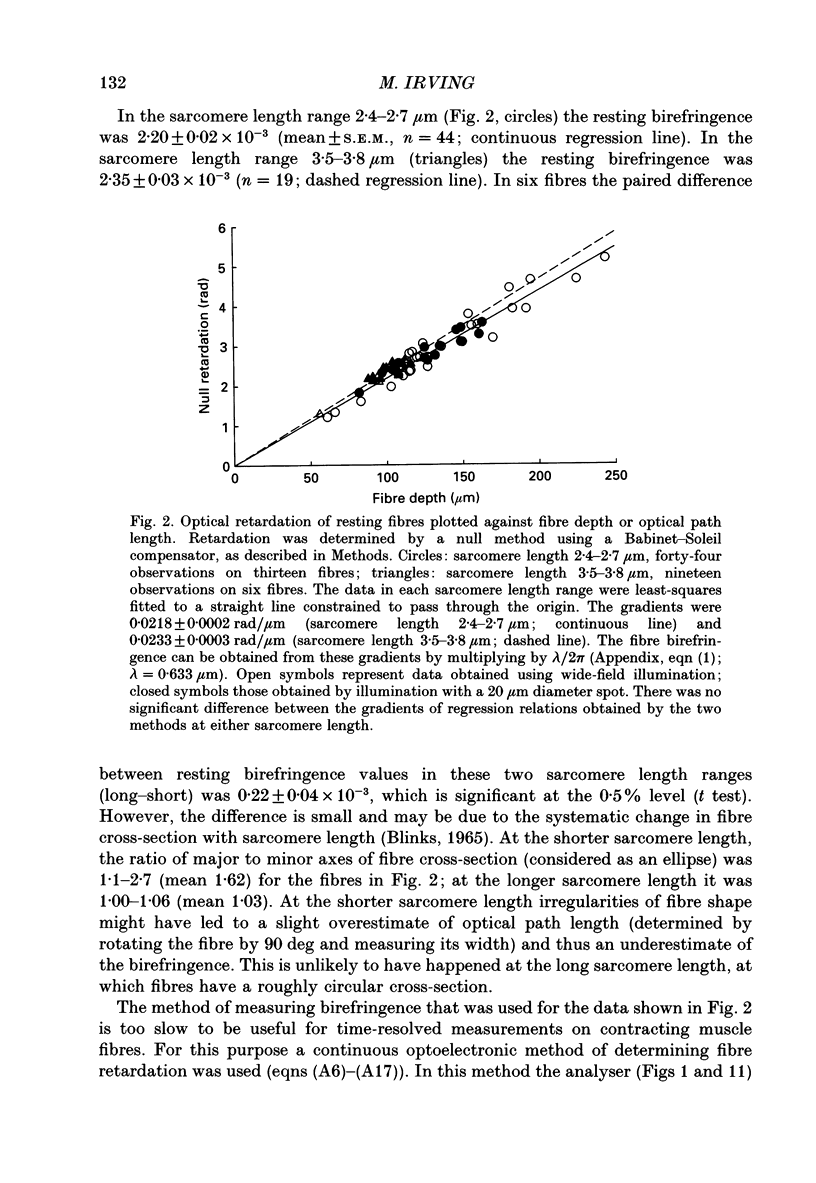
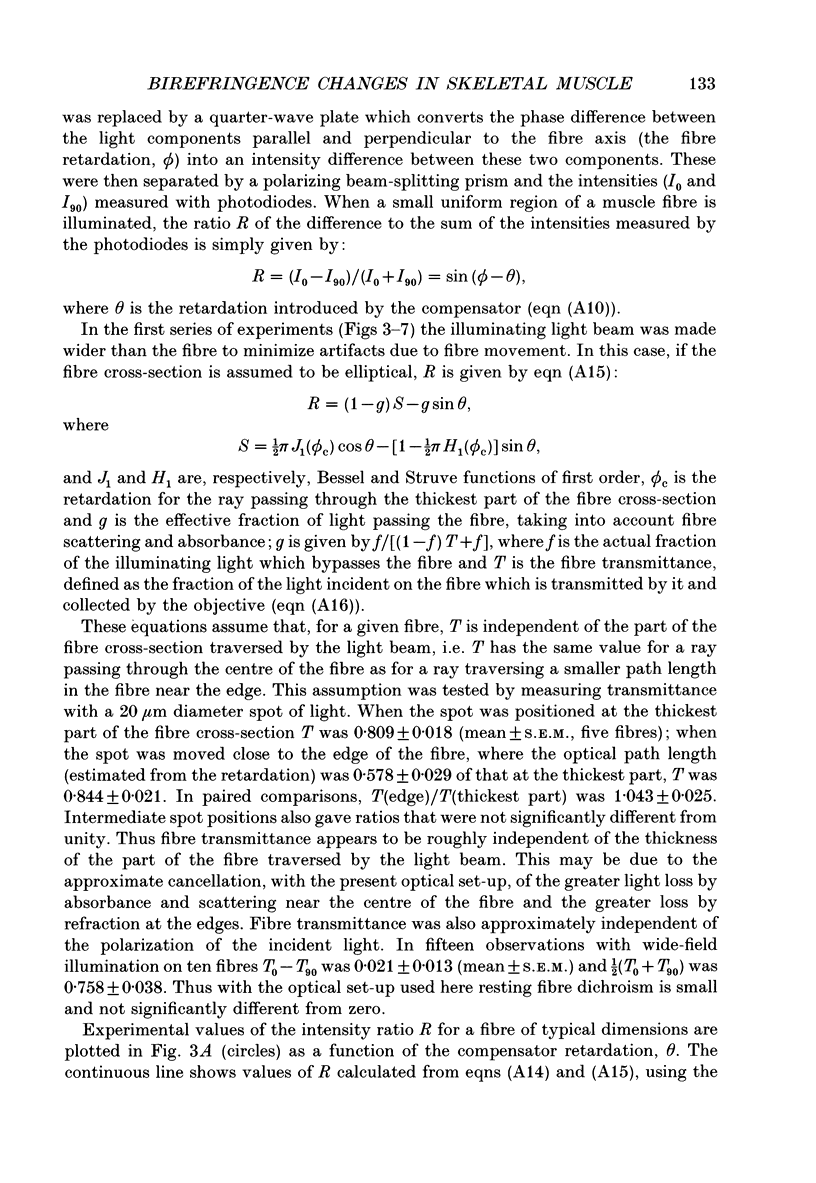
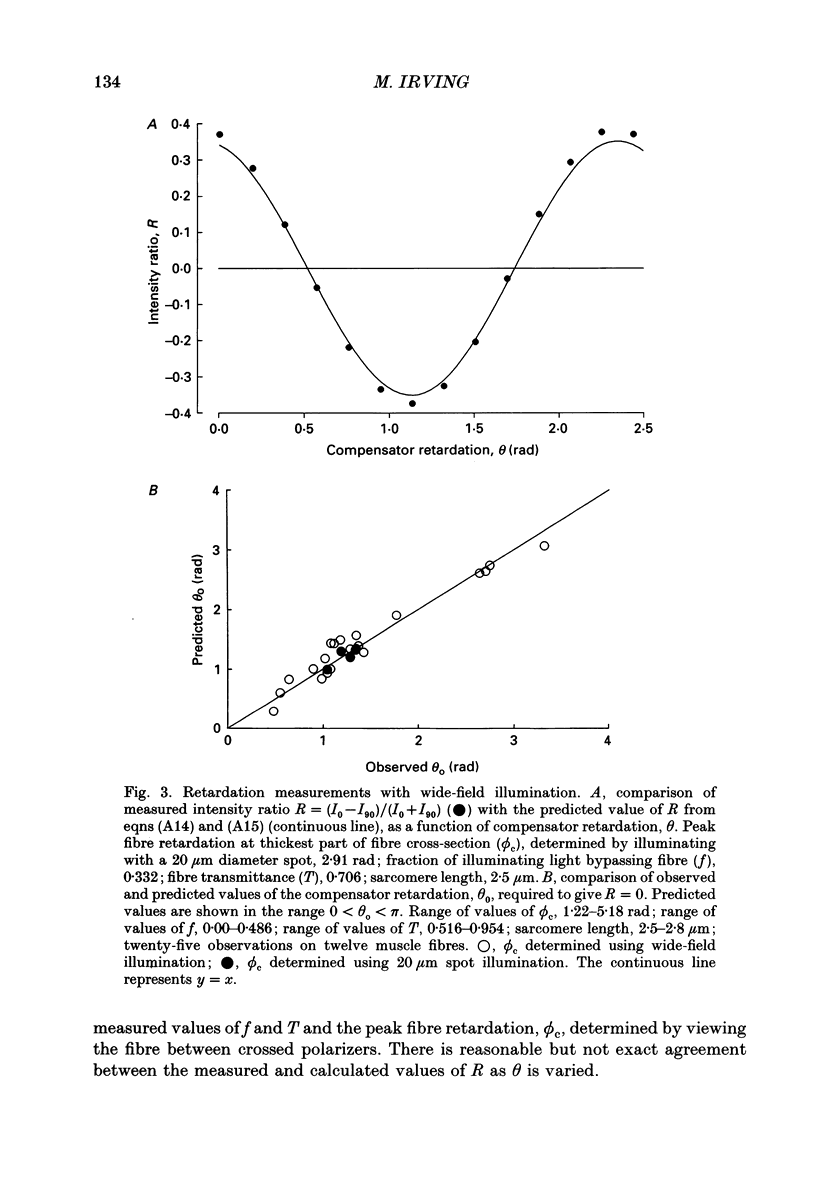
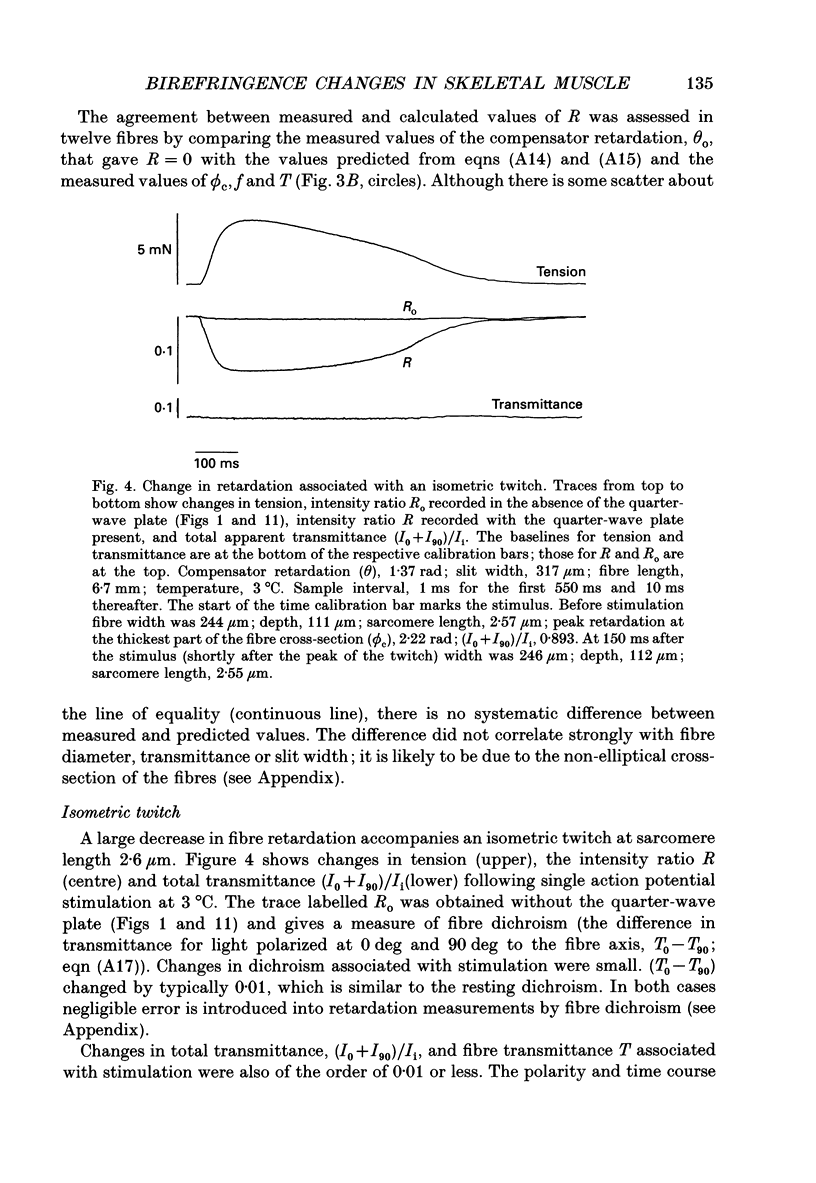
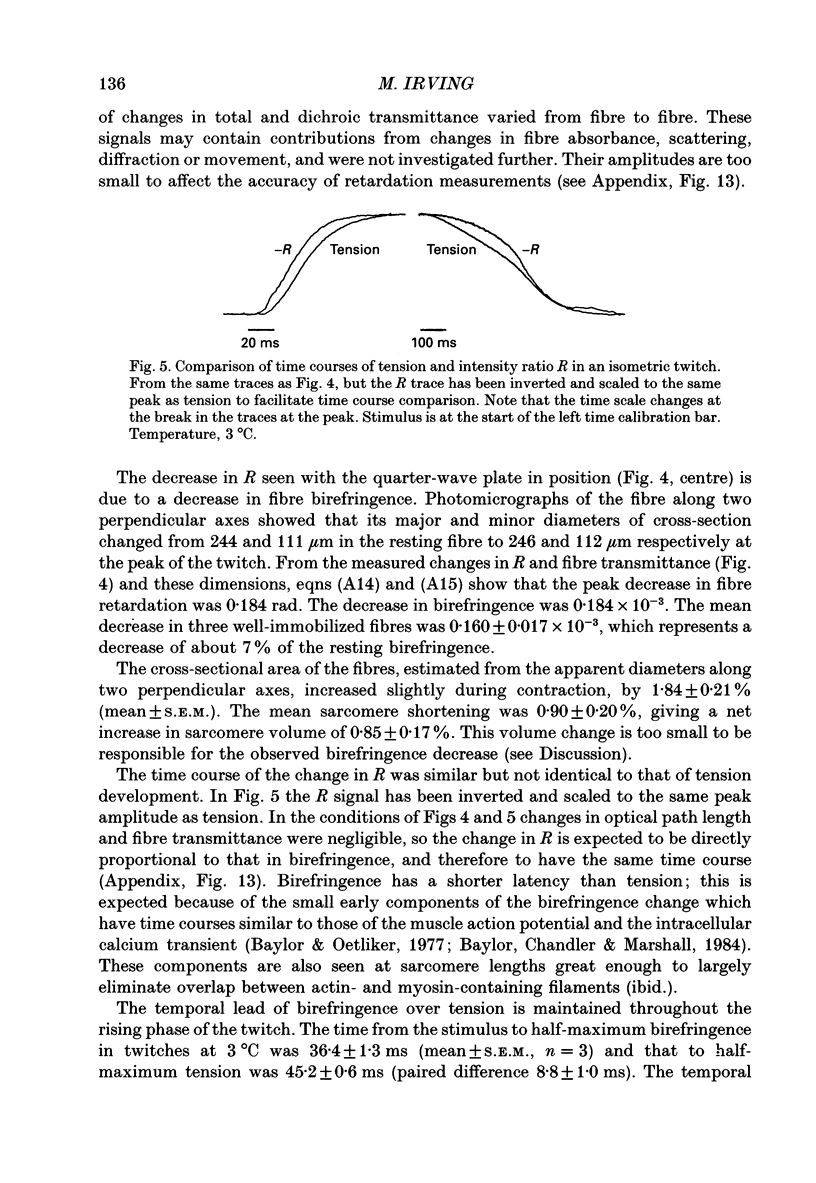
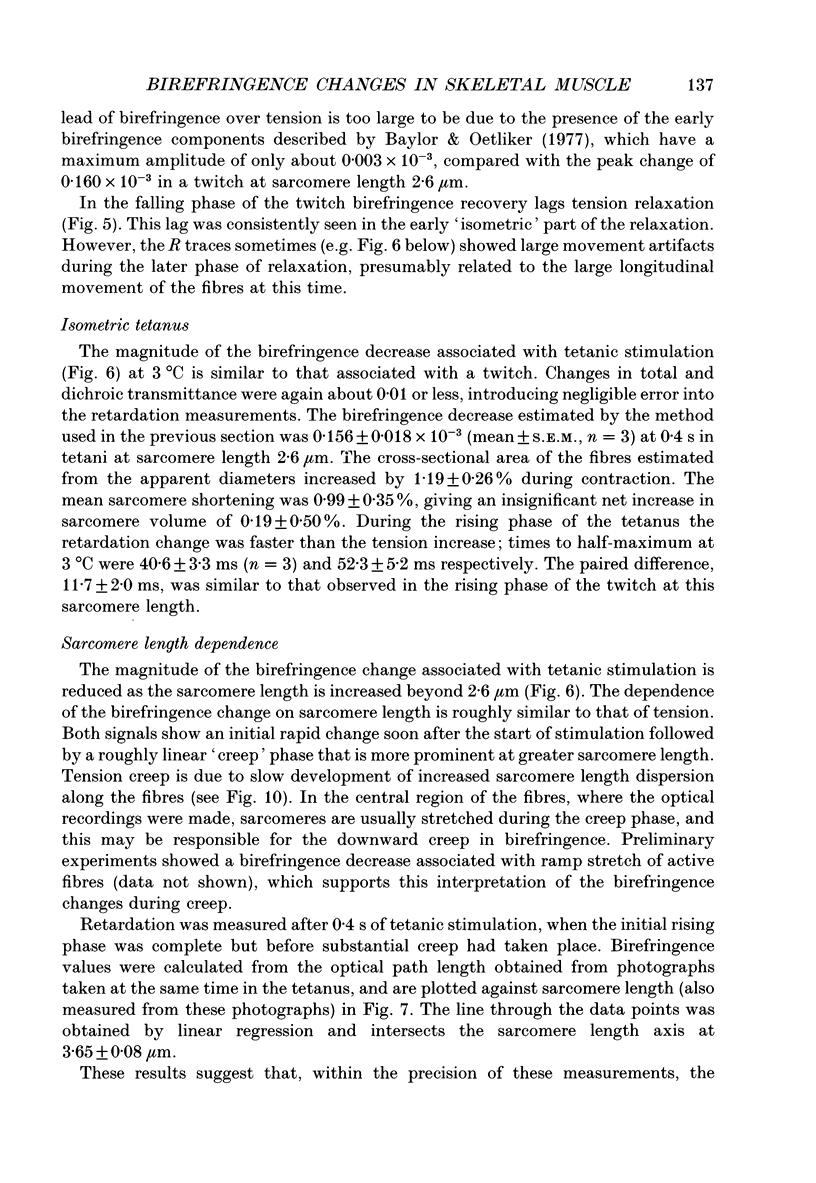
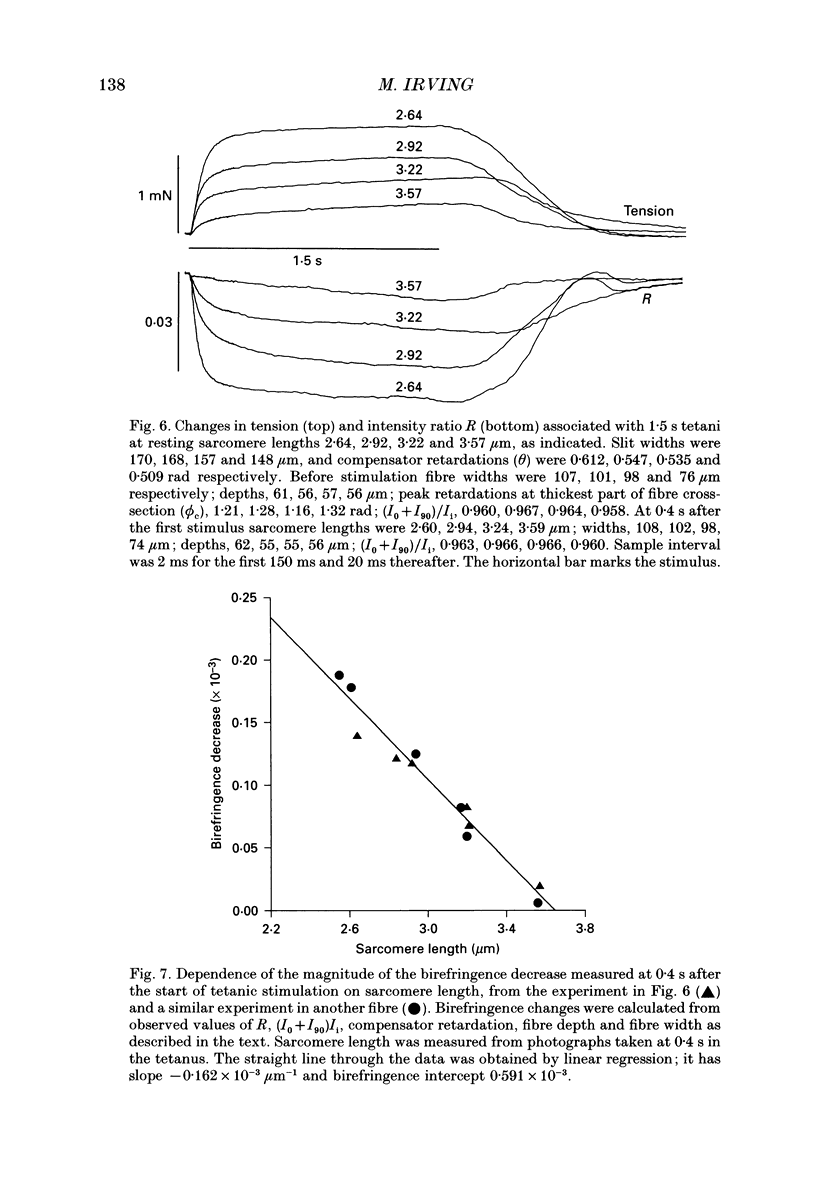
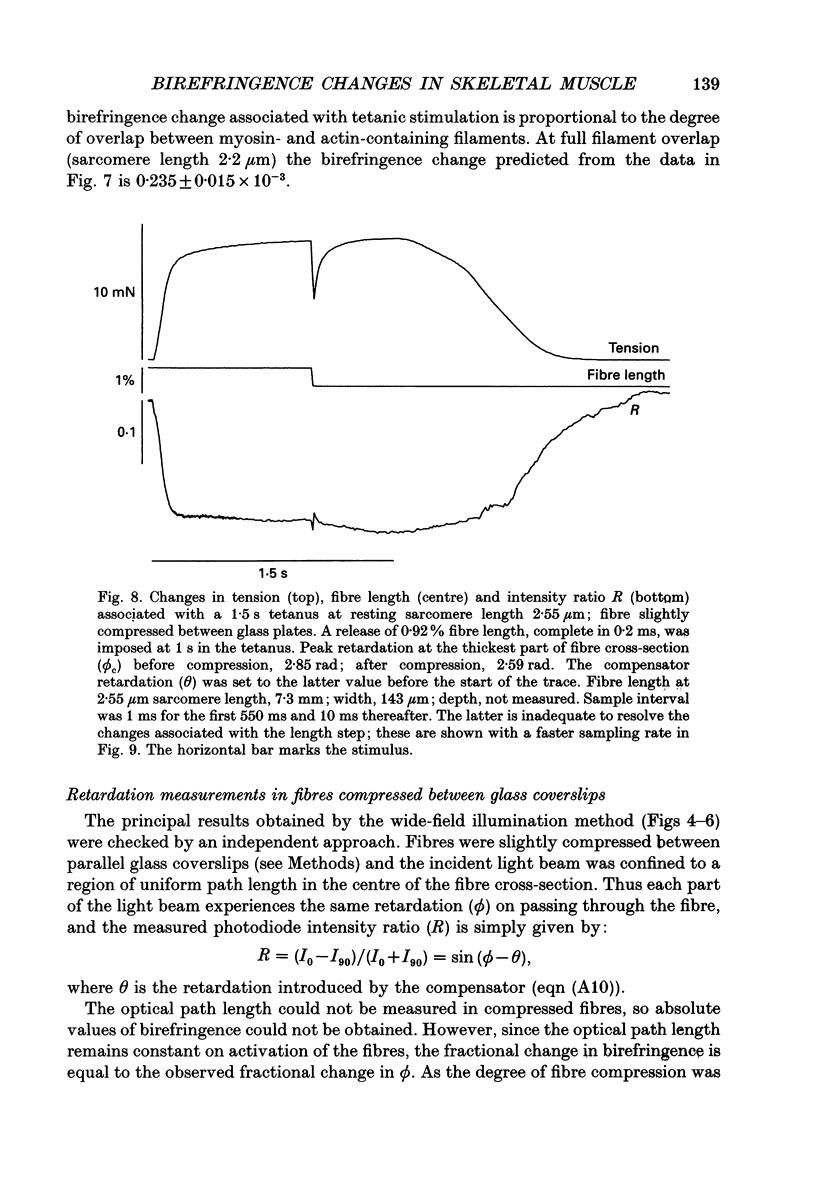
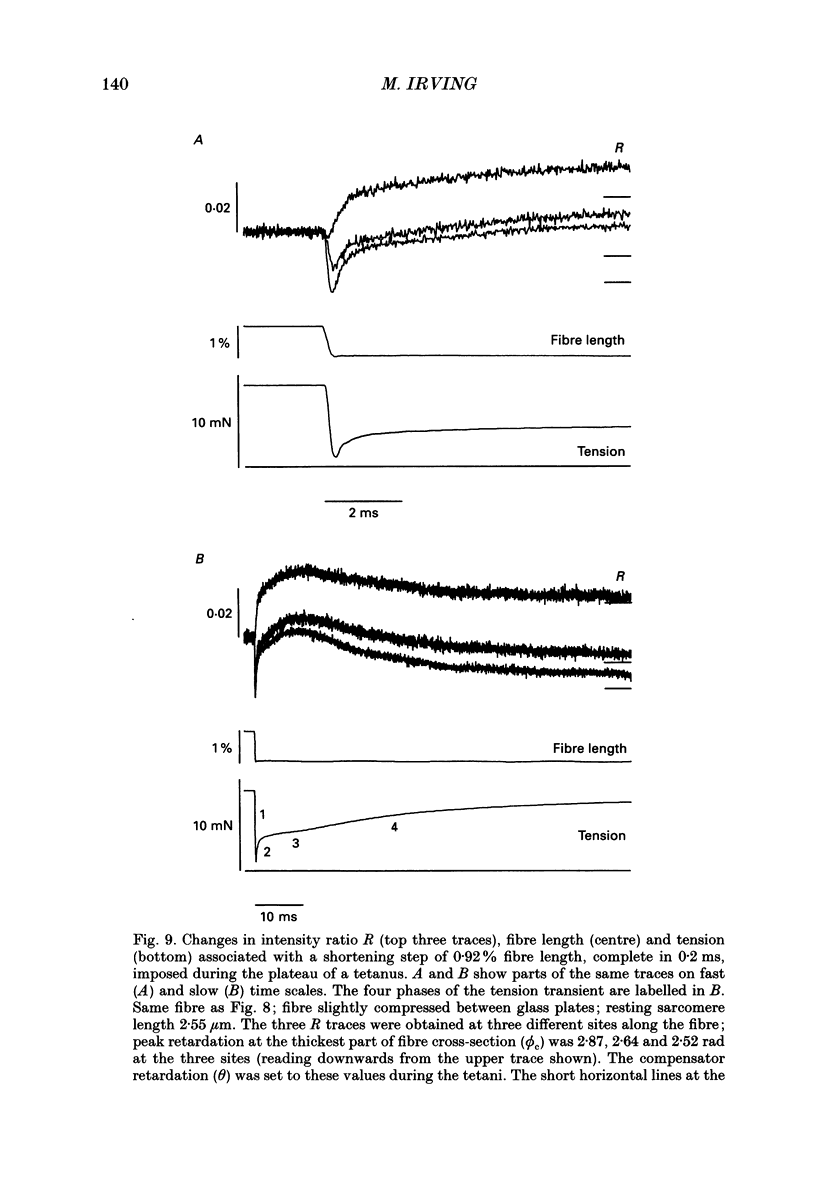
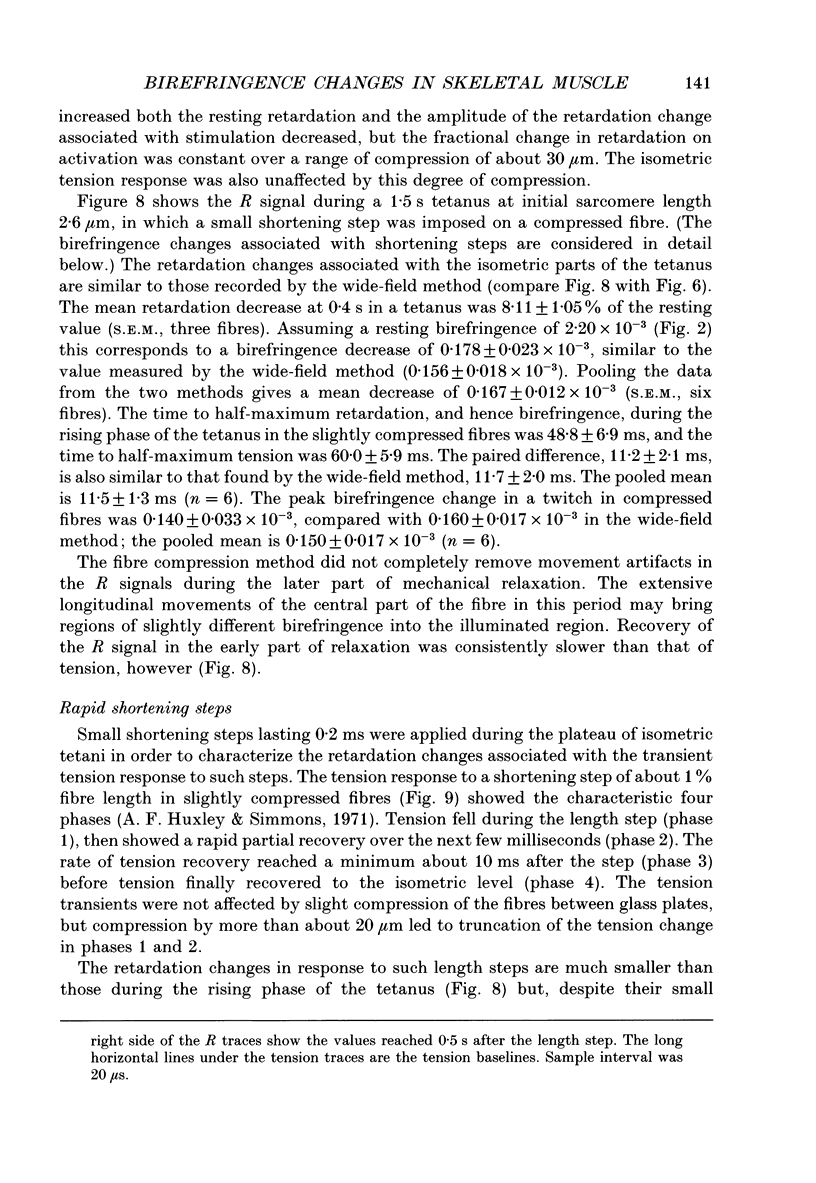
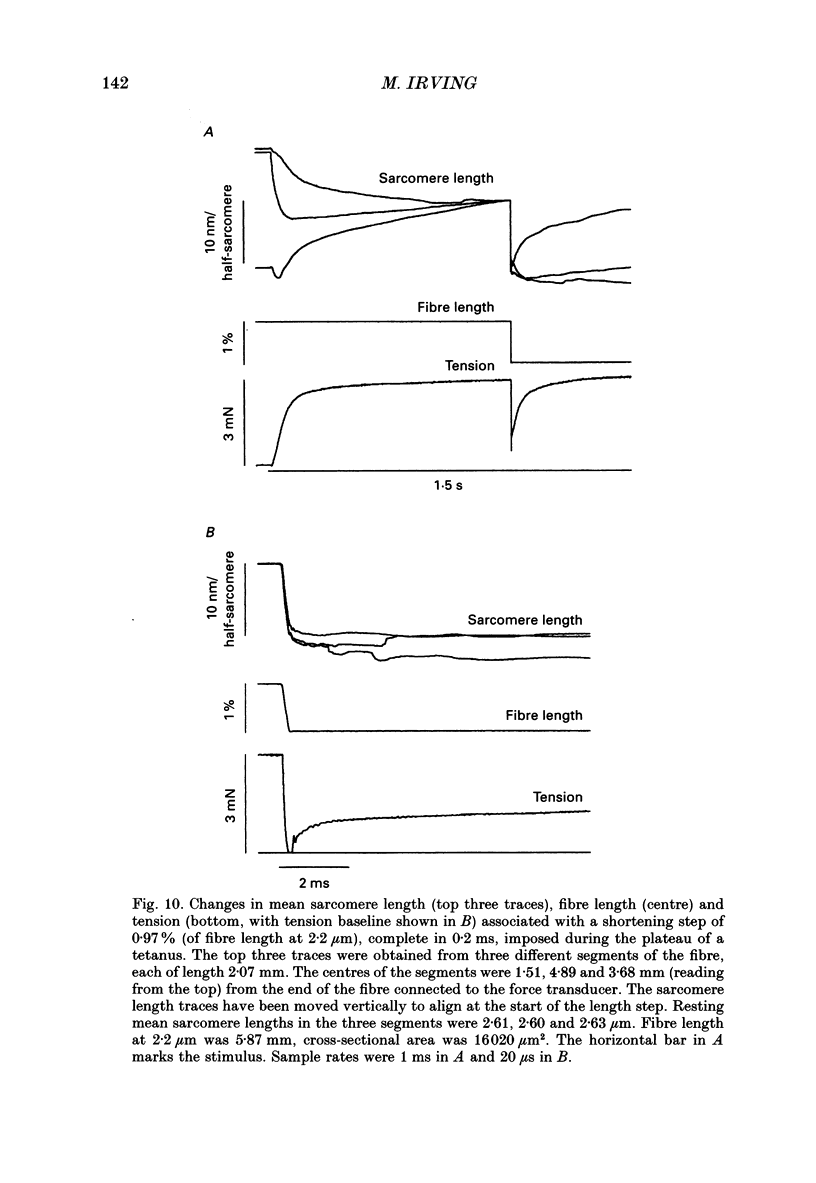
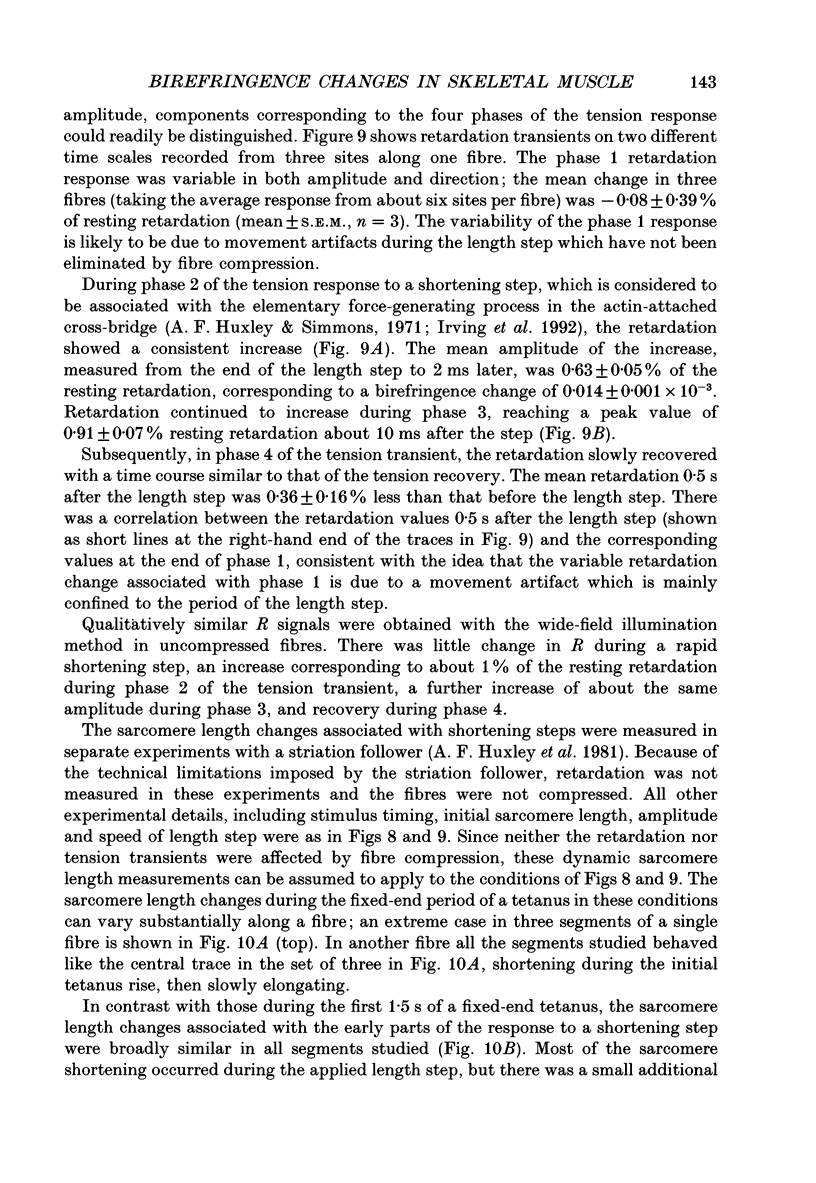
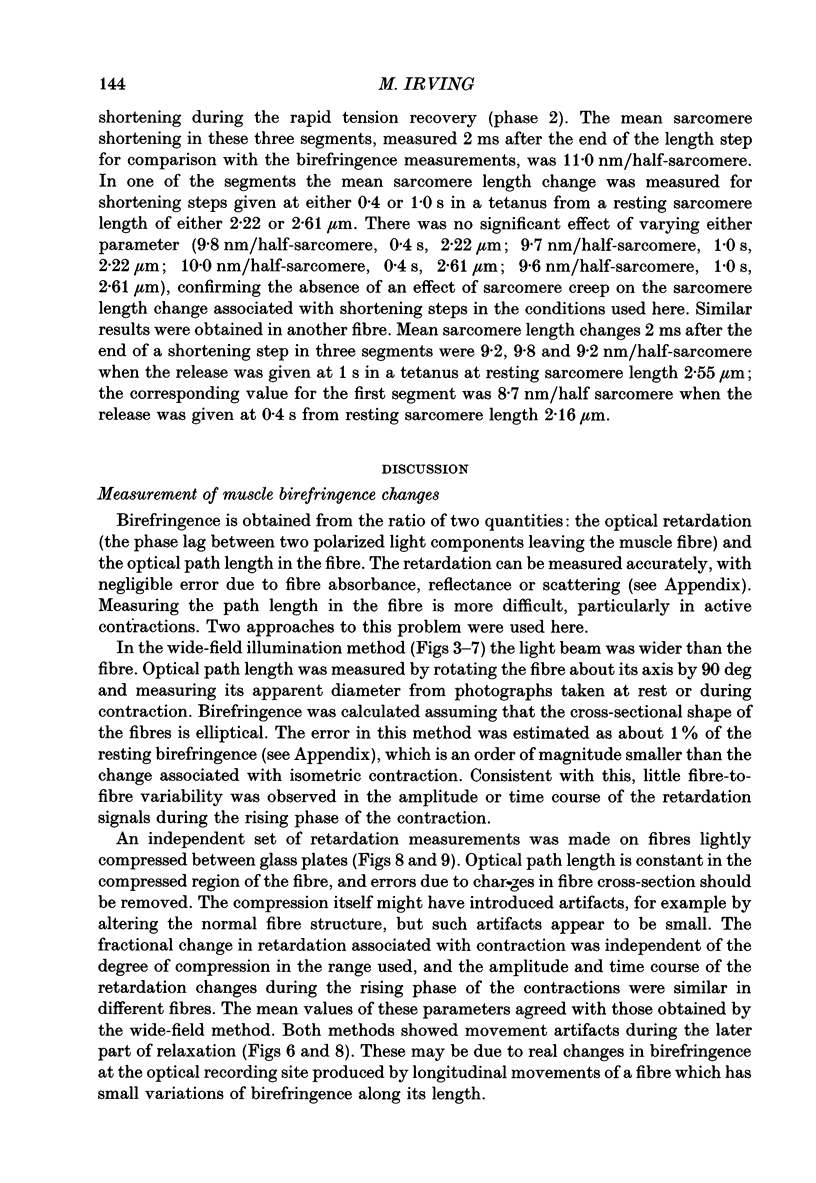
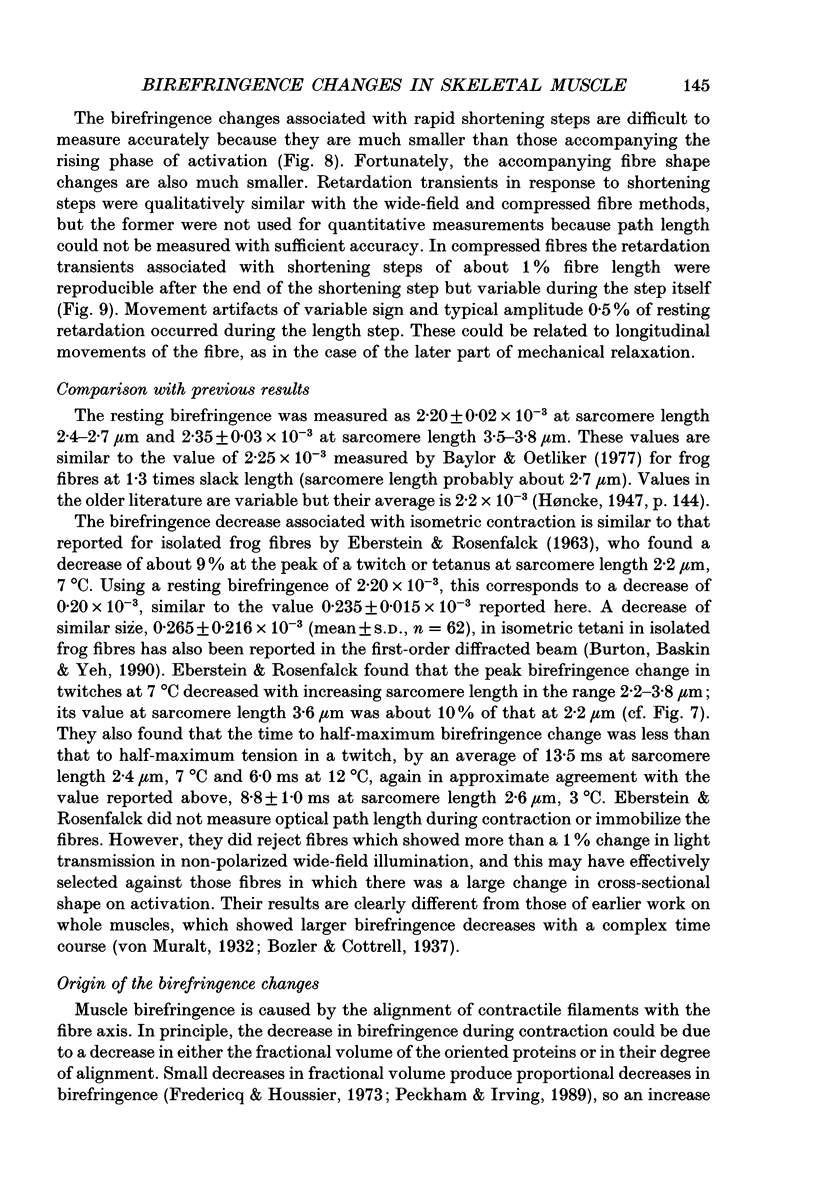
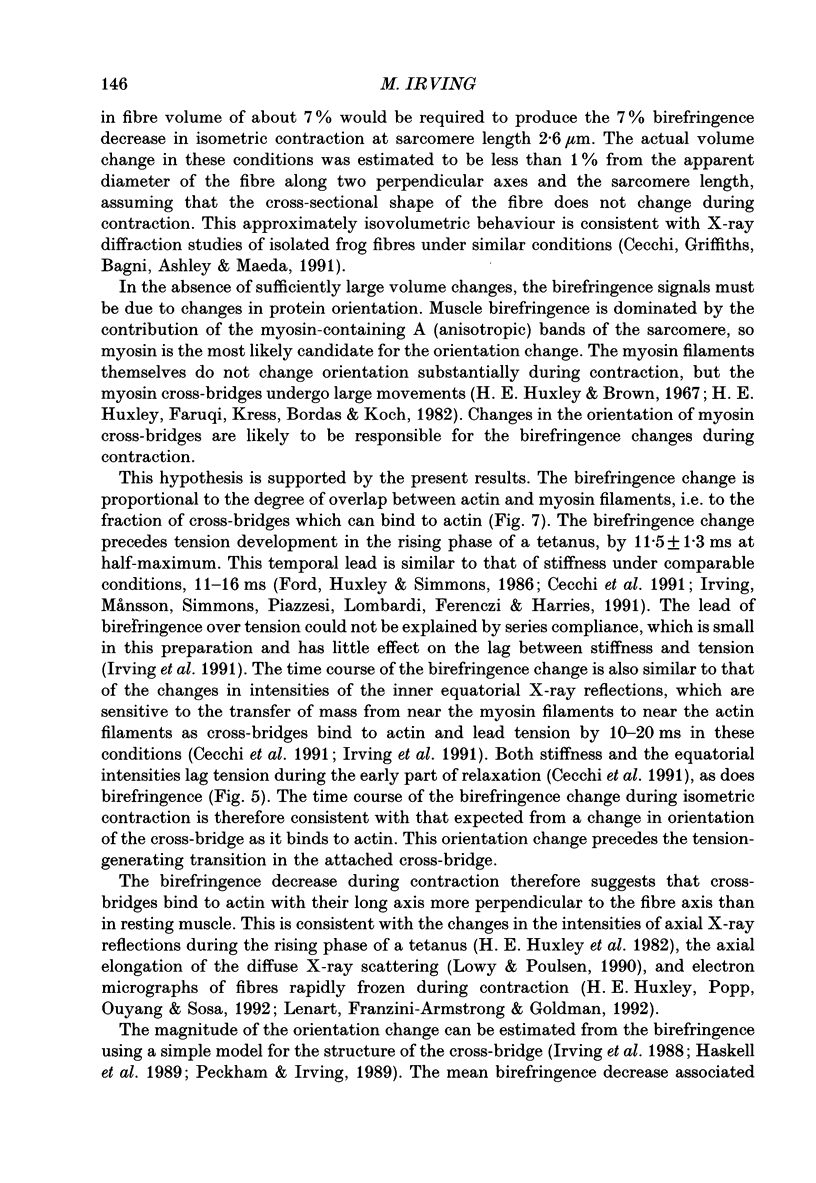
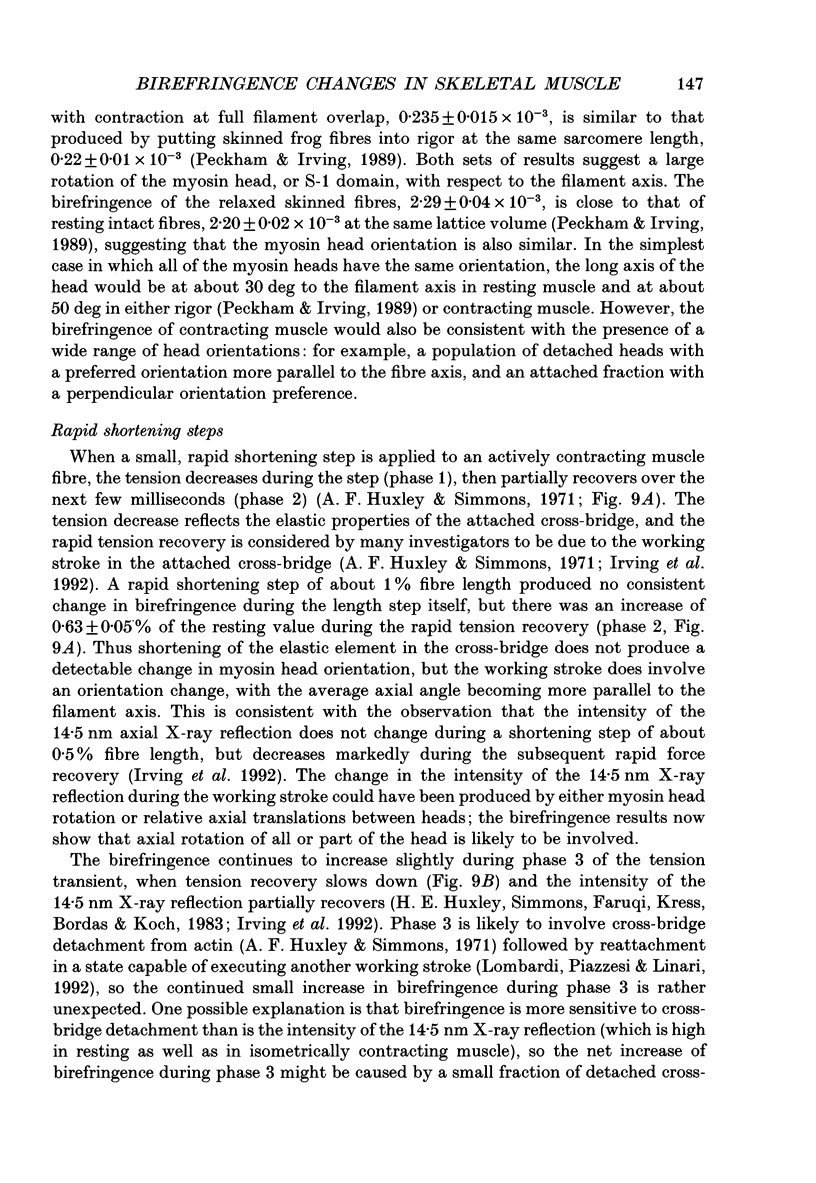
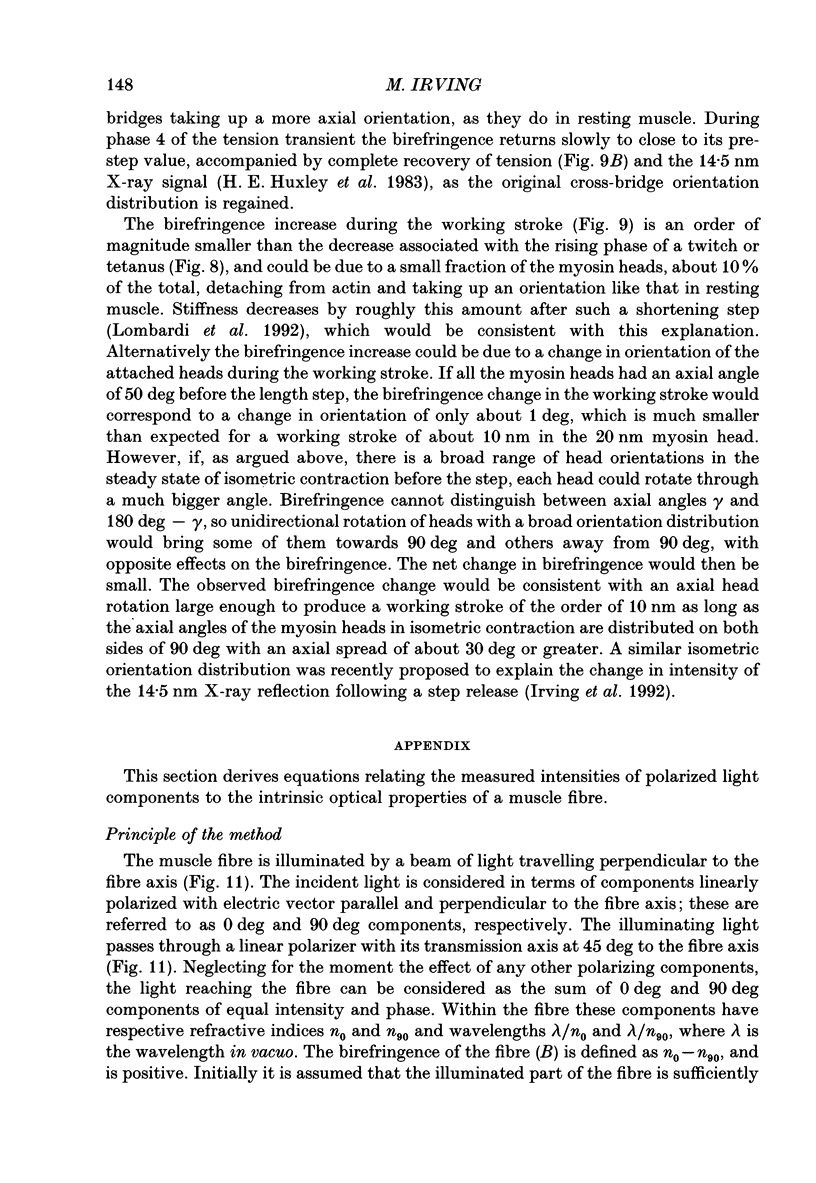
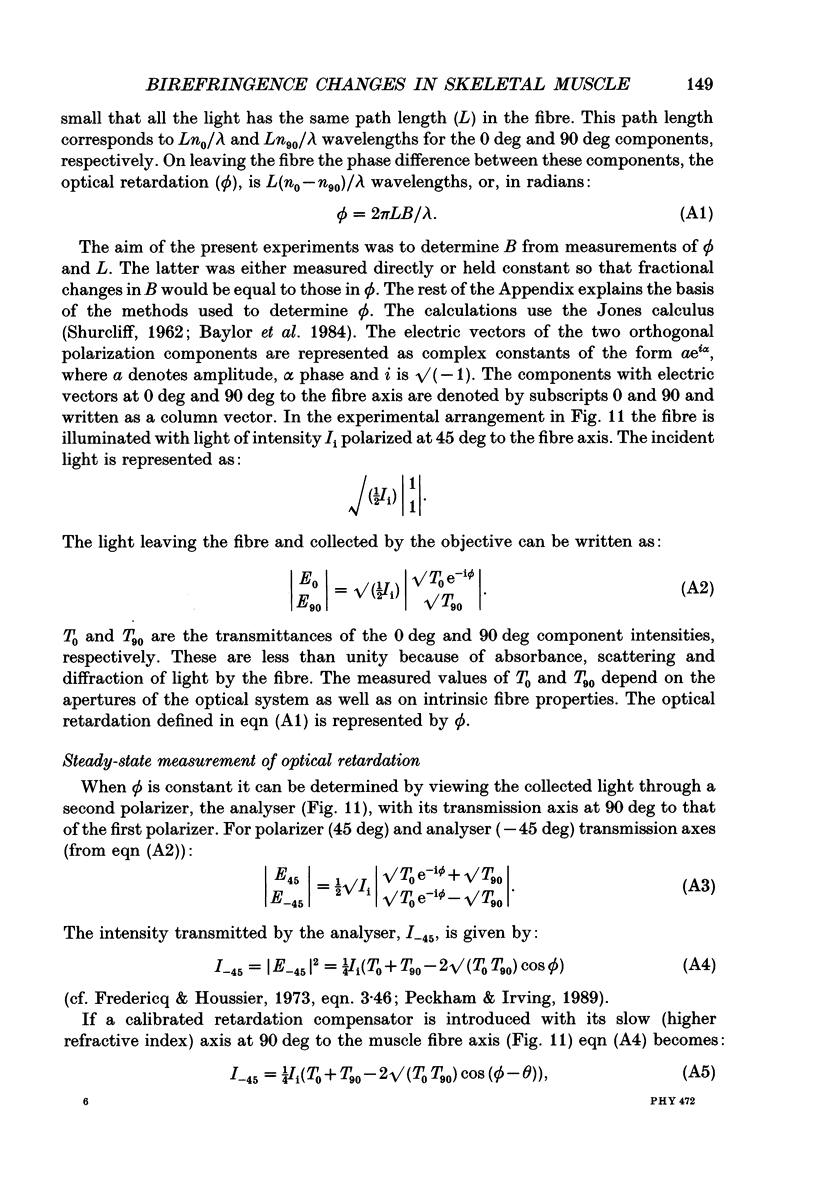
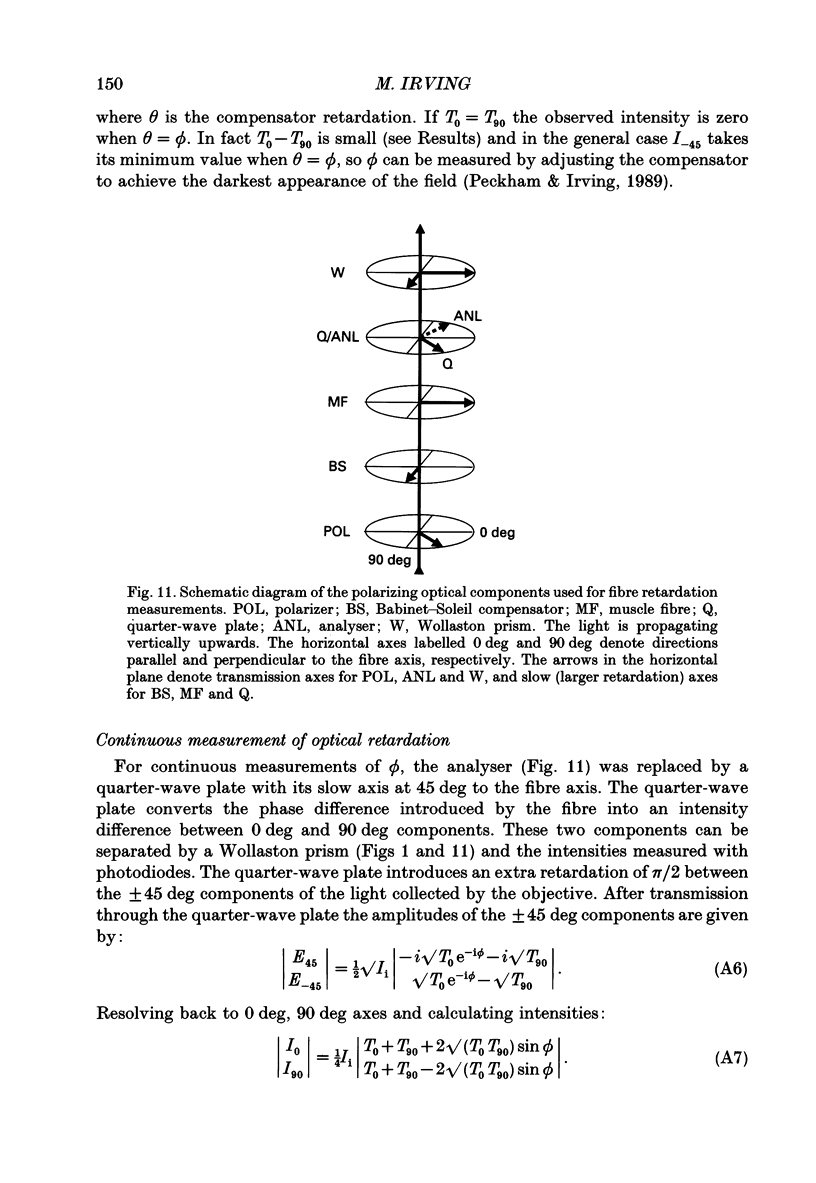
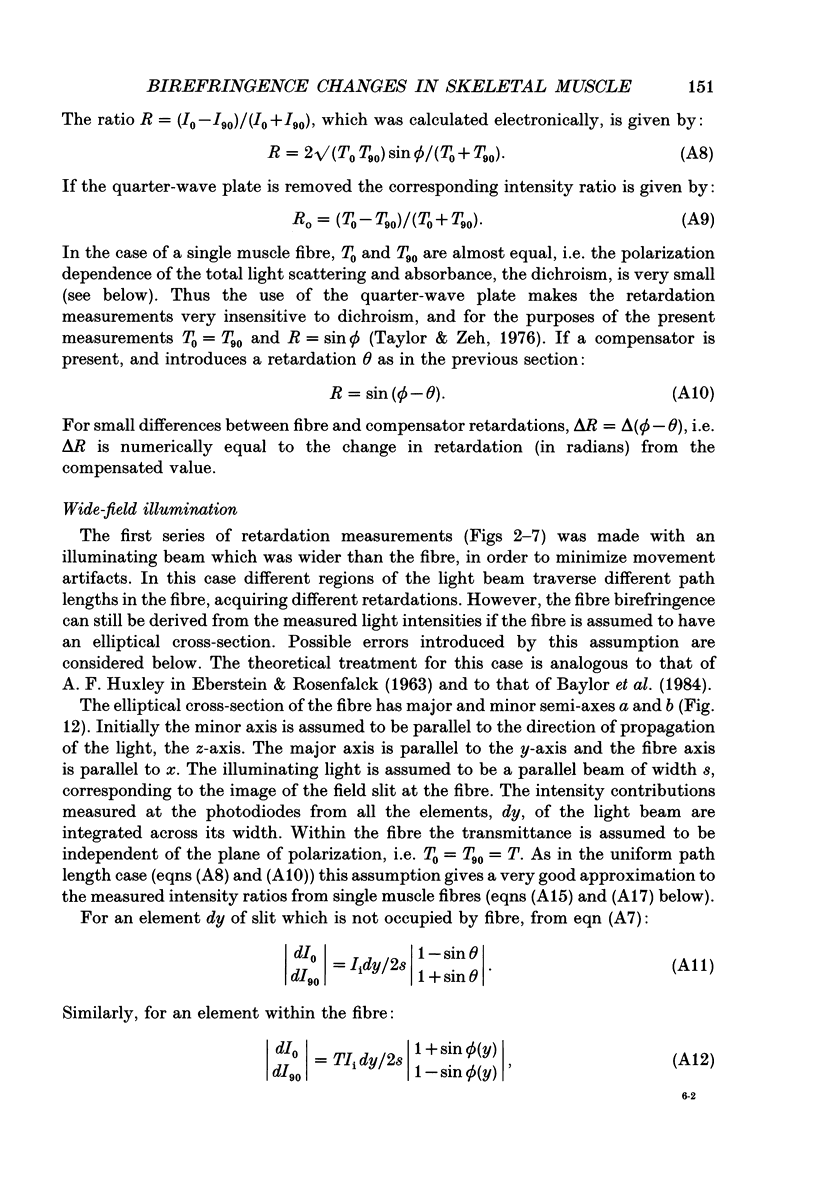
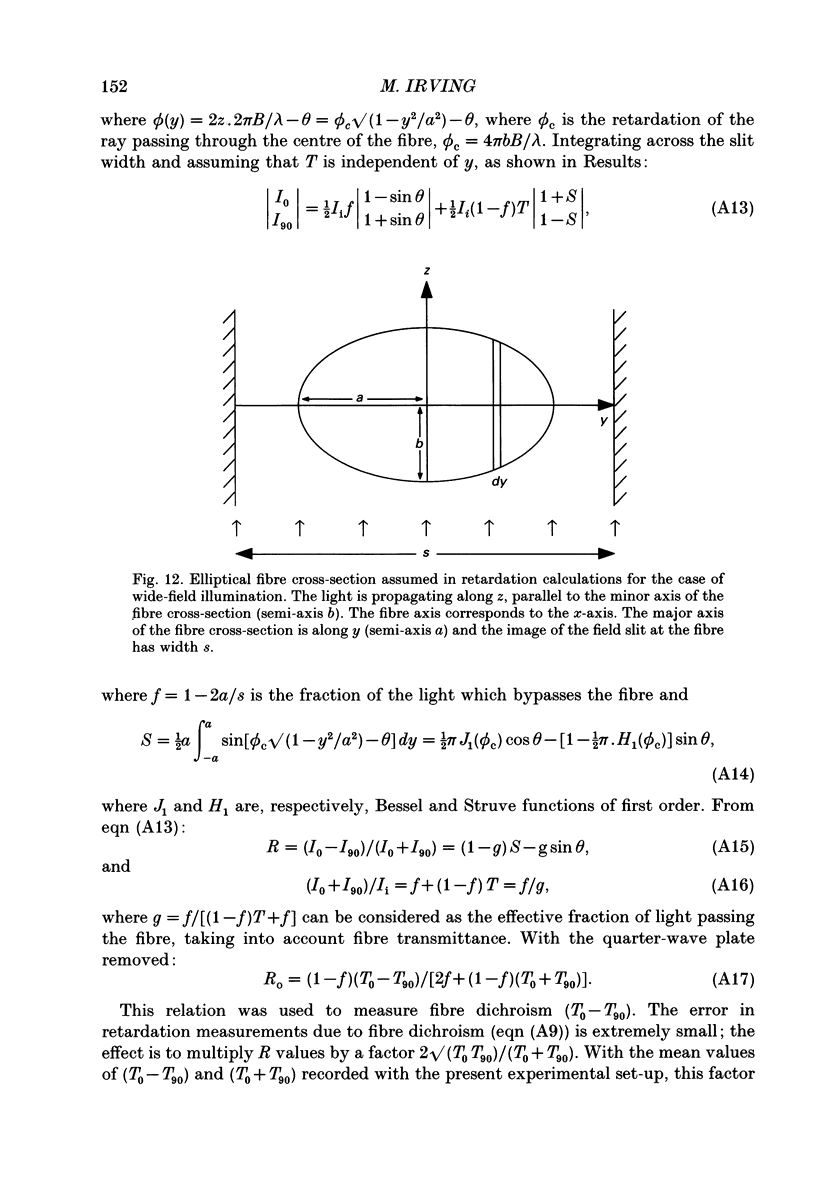
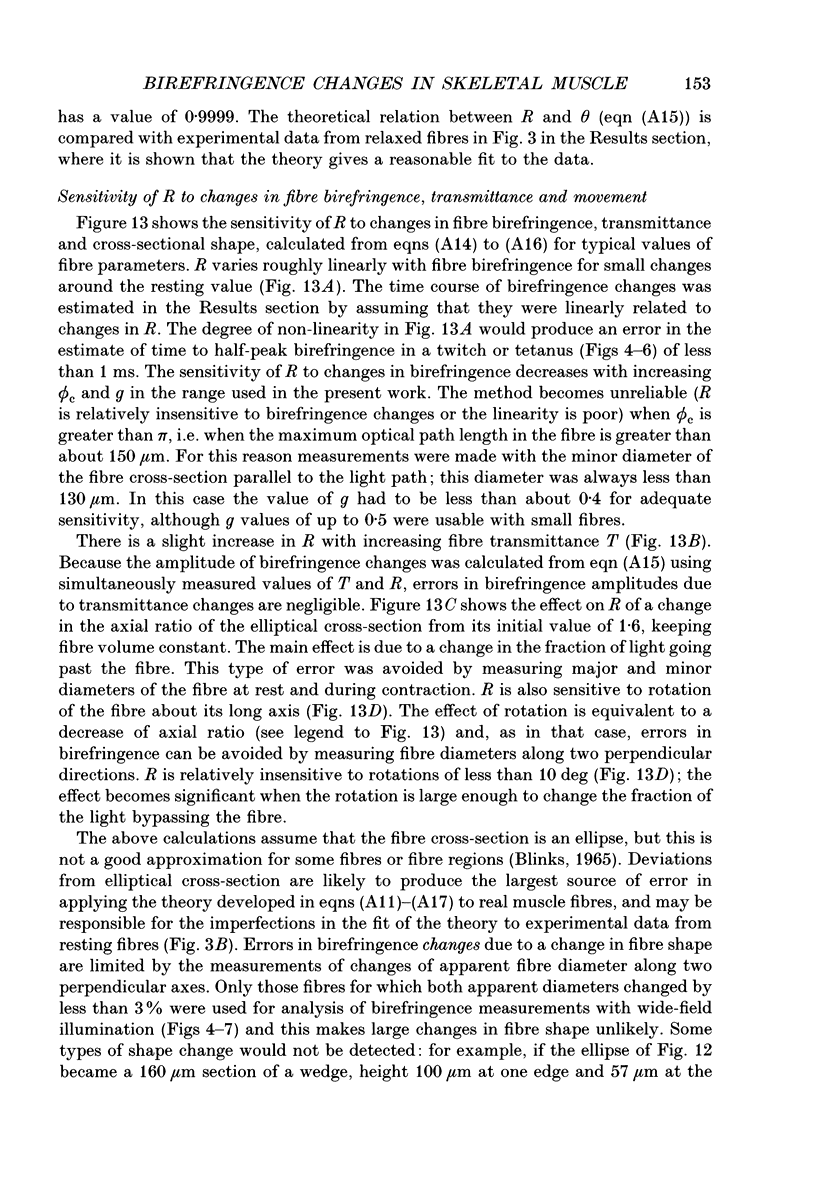
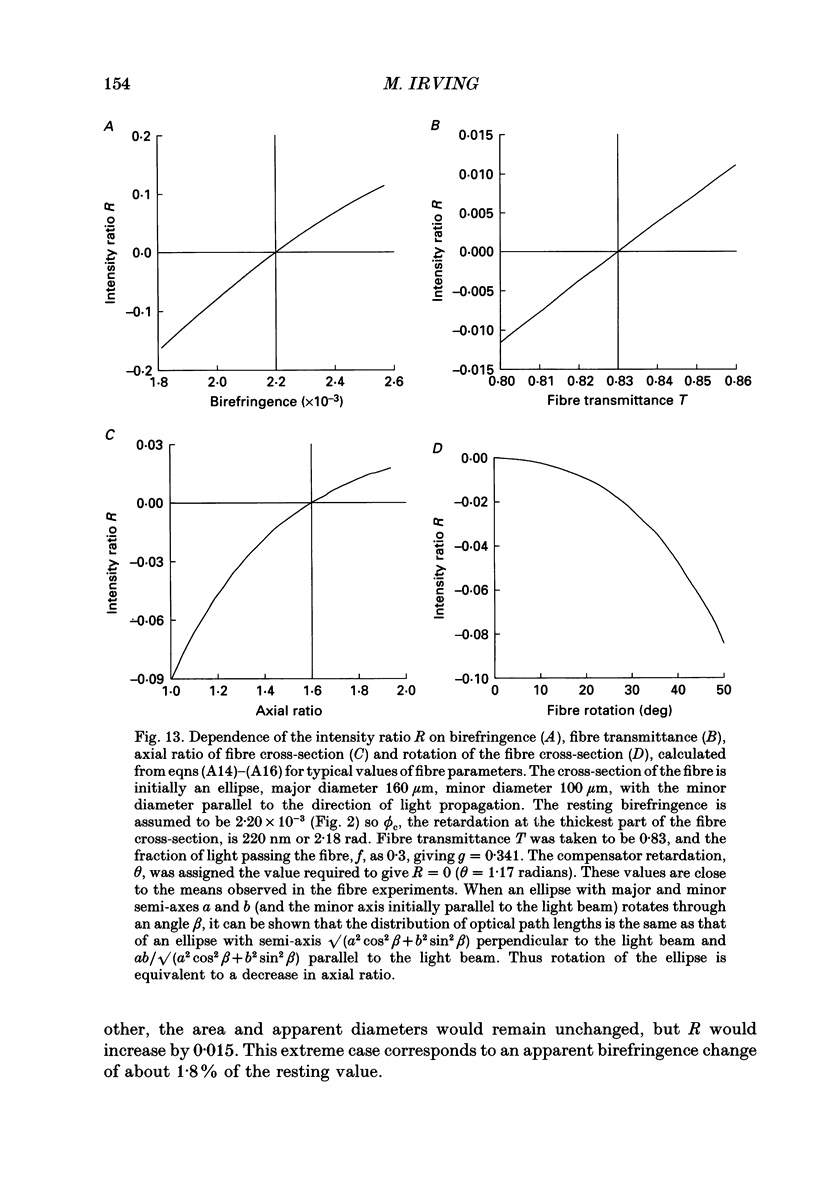
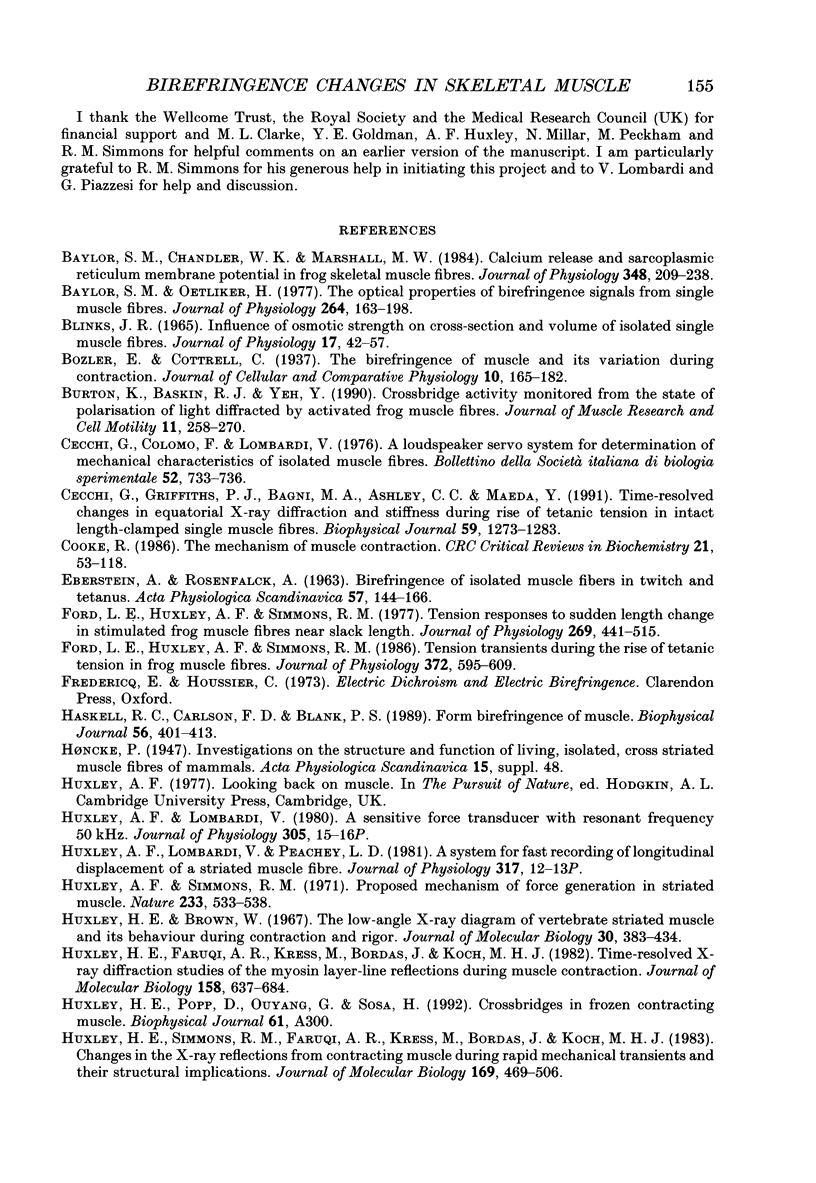
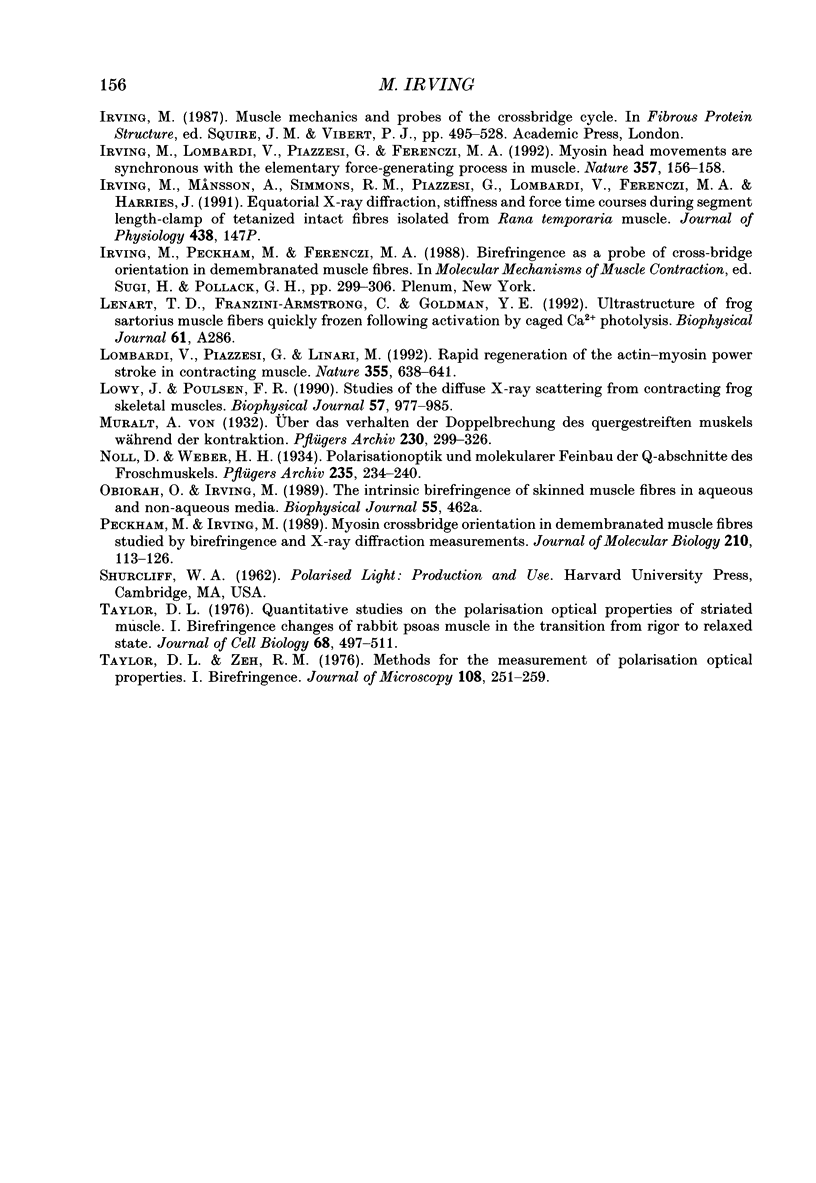
Selected References
These references are in PubMed. This may not be the complete list of references from this article.
- BLINKS J. R. INFLUENCE OF OSMOTIC STRENGTH ON CROSS-SECTION AND VOLUME OF ISOLATED SINGLE MUSCLE FIBRES. J Physiol. 1965 Mar;177:42–57. doi: 10.1113/jphysiol.1965.sp007574. [DOI] [PMC free article] [PubMed] [Google Scholar]
- Baylor S. M., Chandler W. K., Marshall M. W. Calcium release and sarcoplasmic reticulum membrane potential in frog skeletal muscle fibres. J Physiol. 1984 Mar;348:209–238. doi: 10.1113/jphysiol.1984.sp015106. [DOI] [PMC free article] [PubMed] [Google Scholar]
- Baylor S. M., Oetliker H. The optical properties of birefringence signals from single muscle fibres. J Physiol. 1977 Jan;264(1):163–198. doi: 10.1113/jphysiol.1977.sp011662. [DOI] [PMC free article] [PubMed] [Google Scholar]
- Burton K., Baskin R. J., Yeh Y. Crossbridge activity monitored from the state of polarization of light diffracted by activated frog muscle fibres. J Muscle Res Cell Motil. 1990 Jun;11(3):258–270. doi: 10.1007/BF01843579. [DOI] [PubMed] [Google Scholar]
- Cecchi G., Colomo F., Lombardi V. A loudspeaker servo system for determination of mechanical characteristics of isolated muscle fibres. Boll Soc Ital Biol Sper. 1976 May 30;52(10):733–736. [PubMed] [Google Scholar]
- Cecchi G., Griffiths P. J., Bagni M. A., Ashley C. C., Maeda Y. Time-resolved changes in equatorial x-ray diffraction and stiffness during rise of tetanic tension in intact length-clamped single muscle fibers. Biophys J. 1991 Jun;59(6):1273–1283. doi: 10.1016/S0006-3495(91)82342-6. [DOI] [PMC free article] [PubMed] [Google Scholar]
- Cooke R. The mechanism of muscle contraction. CRC Crit Rev Biochem. 1986;21(1):53–118. doi: 10.3109/10409238609113609. [DOI] [PubMed] [Google Scholar]
- Ford L. E., Huxley A. F., Simmons R. M. Tension responses to sudden length change in stimulated frog muscle fibres near slack length. J Physiol. 1977 Jul;269(2):441–515. doi: 10.1113/jphysiol.1977.sp011911. [DOI] [PMC free article] [PubMed] [Google Scholar]
- Ford L. E., Huxley A. F., Simmons R. M. Tension transients during the rise of tetanic tension in frog muscle fibres. J Physiol. 1986 Mar;372:595–609. doi: 10.1113/jphysiol.1986.sp016027. [DOI] [PMC free article] [PubMed] [Google Scholar]
- Haskell R. C., Carlson F. D., Blank P. S. Form birefringence of muscle. Biophys J. 1989 Aug;56(2):401–413. doi: 10.1016/S0006-3495(89)82686-4. [DOI] [PMC free article] [PubMed] [Google Scholar]
- Huxley A. F., Simmons R. M. Proposed mechanism of force generation in striated muscle. Nature. 1971 Oct 22;233(5321):533–538. doi: 10.1038/233533a0. [DOI] [PubMed] [Google Scholar]
- Huxley H. E., Brown W. The low-angle x-ray diagram of vertebrate striated muscle and its behaviour during contraction and rigor. J Mol Biol. 1967 Dec 14;30(2):383–434. doi: 10.1016/s0022-2836(67)80046-9. [DOI] [PubMed] [Google Scholar]
- Huxley H. E., Faruqi A. R., Kress M., Bordas J., Koch M. H. Time-resolved X-ray diffraction studies of the myosin layer-line reflections during muscle contraction. J Mol Biol. 1982 Jul 15;158(4):637–684. doi: 10.1016/0022-2836(82)90253-4. [DOI] [PubMed] [Google Scholar]
- Huxley H. E., Simmons R. M., Faruqi A. R., Kress M., Bordas J., Koch M. H. Changes in the X-ray reflections from contracting muscle during rapid mechanical transients and their structural implications. J Mol Biol. 1983 Sep 15;169(2):469–506. doi: 10.1016/s0022-2836(83)80062-x. [DOI] [PubMed] [Google Scholar]
- Irving M., Lombardi V., Piazzesi G., Ferenczi M. A. Myosin head movements are synchronous with the elementary force-generating process in muscle. Nature. 1992 May 14;357(6374):156–158. doi: 10.1038/357156a0. [DOI] [PubMed] [Google Scholar]
- Irving M., Peckham M., Ferenczi M. A. Birefringence as a probe of crossbridge orientation in demembranated muscle fibres. Adv Exp Med Biol. 1988;226:299–306. [PubMed] [Google Scholar]
- Lombardi V., Piazzesi G., Linari M. Rapid regeneration of the actin-myosin power stroke in contracting muscle. Nature. 1992 Feb 13;355(6361):638–641. doi: 10.1038/355638a0. [DOI] [PubMed] [Google Scholar]
- Lowy J., Poulsen F. R. Studies of the diffuse x-ray scattering from contracting frog skeletal muscles. Biophys J. 1990 May;57(5):977–985. doi: 10.1016/S0006-3495(90)82617-5. [DOI] [PMC free article] [PubMed] [Google Scholar]
- Peckham M., Irving M. Myosin crossbridge orientation in demembranated muscle fibres studied by birefringence and X-ray diffraction measurements. J Mol Biol. 1989 Nov 5;210(1):113–126. doi: 10.1016/0022-2836(89)90294-5. [DOI] [PubMed] [Google Scholar]
- Toylor D. L. Quantitative studies on the polarization optical properties of striated muscle. I. Birefringence changes of rabbit psoas muscle in the transition from rigor to relaxed state. J Cell Biol. 1976 Mar;68(3):497–511. doi: 10.1083/jcb.68.3.497. [DOI] [PMC free article] [PubMed] [Google Scholar]