Abstract
1. Excitatory postsynaptic currents (EPSCs) were studied by whole-cell voltage-clamp recording (WCR) from pyramidal cells in the CA3 field of rat hippocampal slices. Input from mossy fibres was evoked by stimuli applied to stratum granulosum ('dentate gyrus stimulation'). This often resulted in complex, multi-component EPSCs with rise times as long as 5.0 ms (mean = 2.5 ms). In contrast, individual EPSC components typically had rise times between 0.3 and 1.0 ms. 2. To isolate monosynaptic, mossy fibre-driven EPSC components, slices were exposed to 'suppressing' media that reduced response amplitudes by 64-88%. In five out of six cases, long EPSC rising phases (> 3 ms) retained the same shape during suppression. This implied that EPSCs were driven by asynchronously active mossy fibre inputs. 3. From latencies of antidromically driven granule cell population spikes (GCPSs) a mean conduction velocity of 0.67 m/s was inferred. Conduction distance had practically no correlation with GCPS duration, implying that velocity dispersion was small and did not desynchronize mossy fibre impulses. EPSC components exhibited 'surplus' latency; they occurred 0.9-4.8 ms after latencies expected on the basis of direct conduction distances. 4. Mossy fibre volleys (MFVs) were evoked by dentate gyrus stimulation and studied with neurotransmission disabled. MFV negative phases lasted from 2.5 to 4.5 ms and had multiple components. By comparison, negative phases of Schaffer collateral fibre volleys (SCFVs) were always simple in shape and lasted 1.5 ms or less. MFV components had surplus latencies similar to those of EPSC components. Late MFV components did not require high stimulus intensities. 5. Widespread activation of granule cells occurred when stimuli were applied to single loci in the stratum granulosum. This implies that such stimuli elicit antidromic impulses in hilar collaterals of mossy fibres, which could result in activation of orthodromic impulses in mossy fibre trunks that had not been stimulated directly. After anti-, then orthodromic conduction, impulses would arrive in the CA3 subfield with 'surplus' latency. 6. When cuts were made in the hilus to prevent anti-/orthodromic conduction, MFV durations were reduced, but only to a small extent. This implies that surplus latency and asynchrony arise in part by anti-/orthodromic conduction, and partly by a mechanism that is intrinsic to mossy fibres or their 'giant' boutons. 7. Because of desynchronization of mossy fibre inputs, there probably are significant differences between kinetic properties of averaged, compound mossy fibre EPSCs and those of unitary mossy fibre EPSCs (i.e. currents driven by input from single presynaptic axons).(ABSTRACT TRUNCATED AT 400 WORDS)
Full text
PDF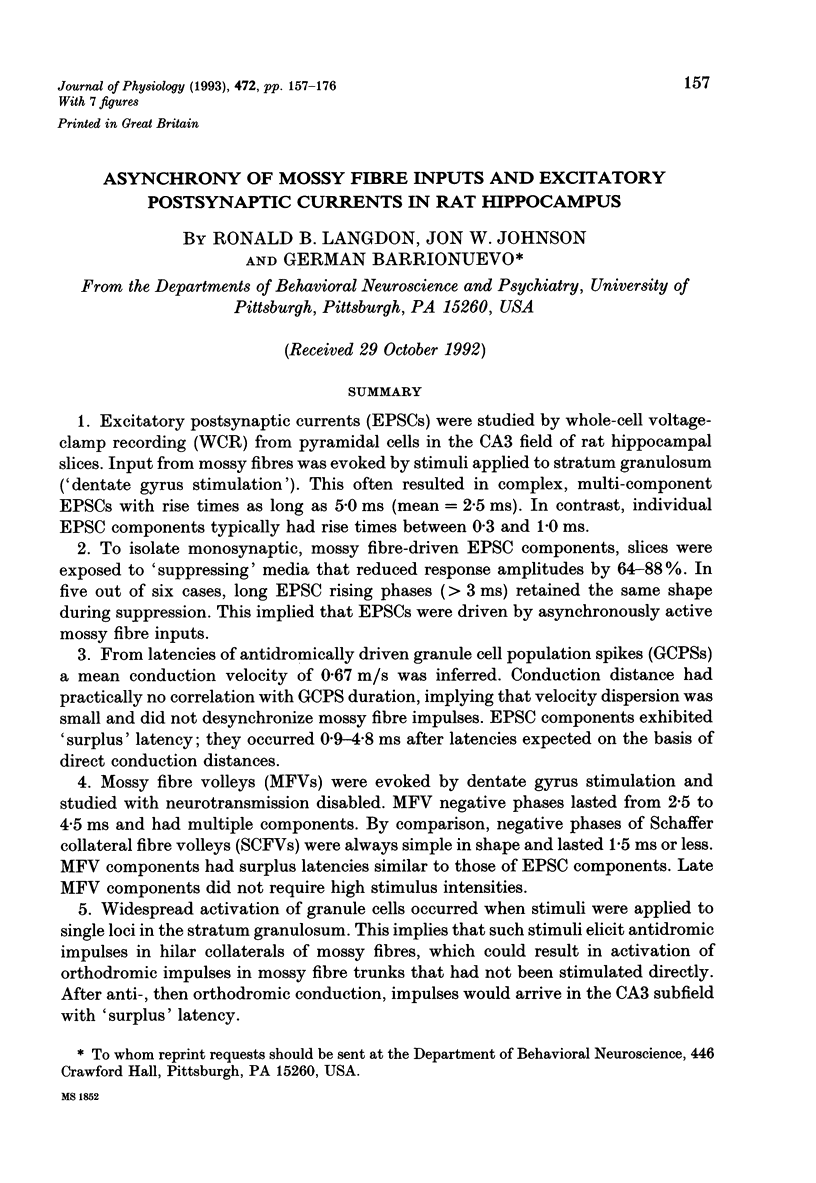
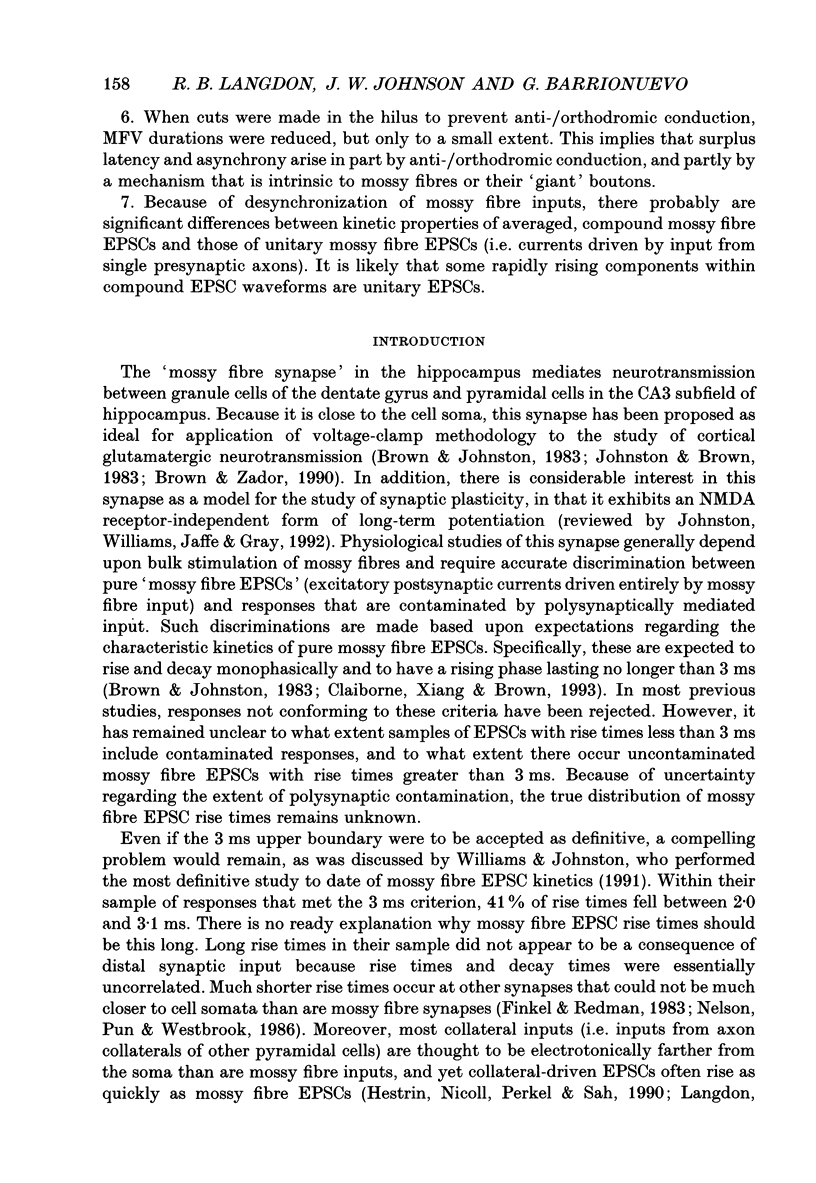
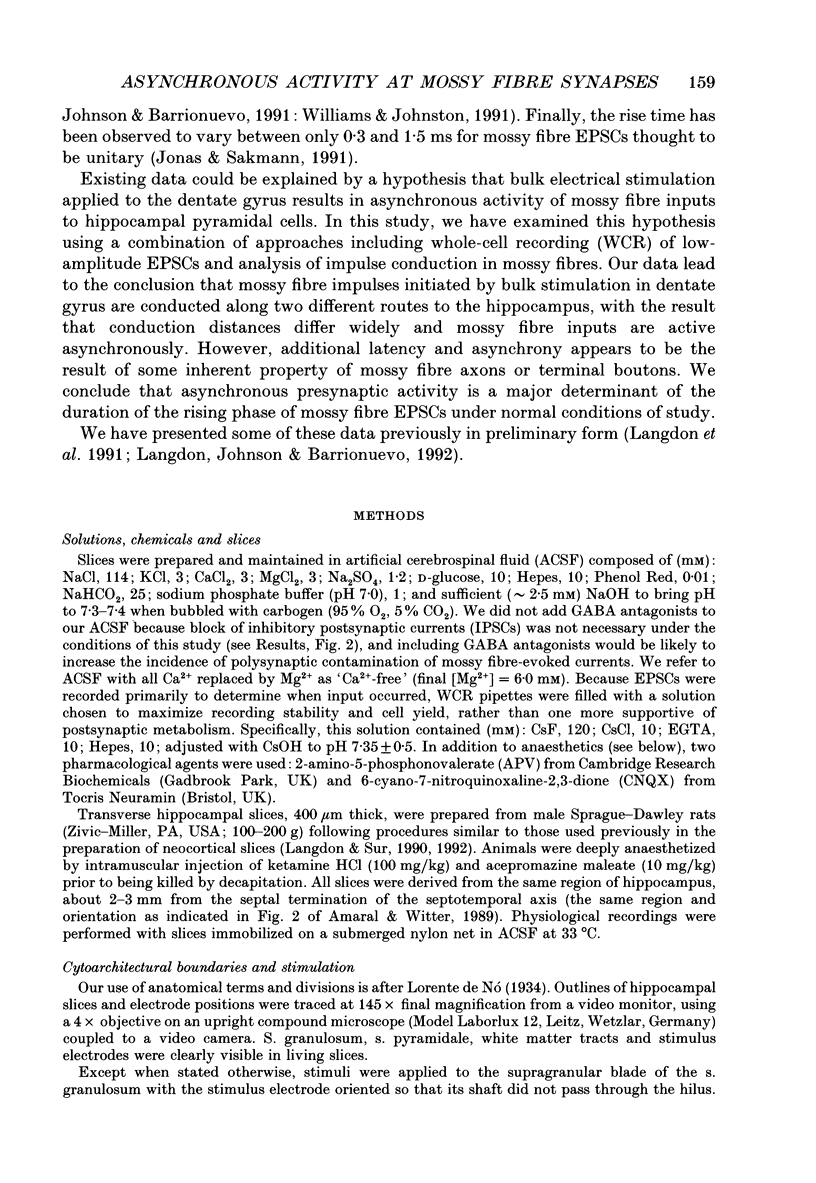
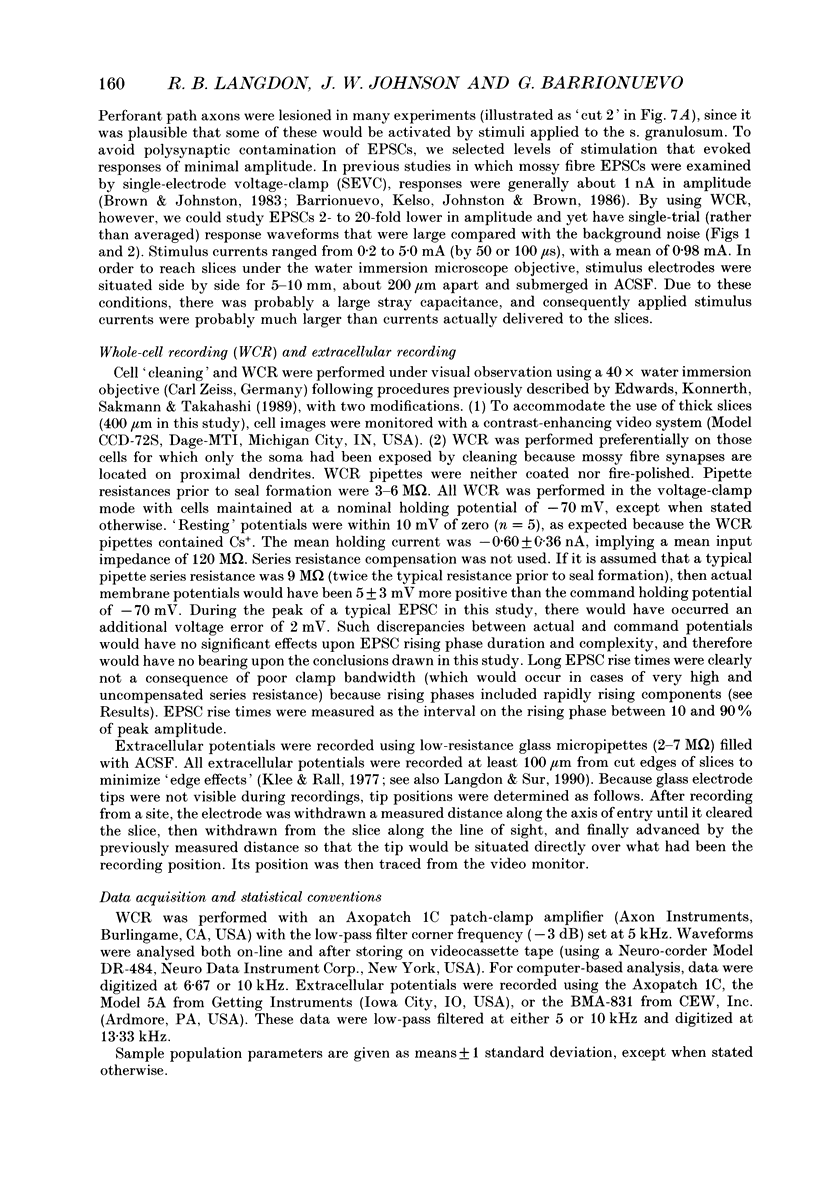
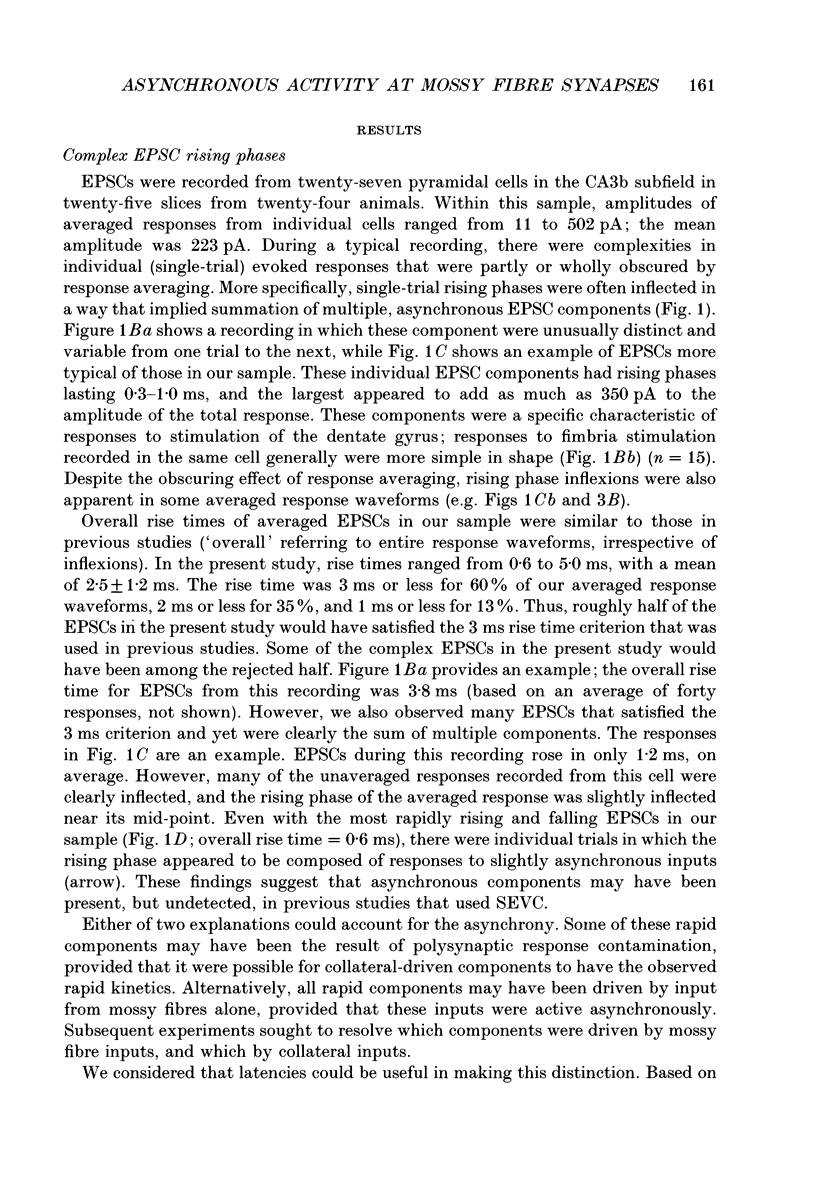
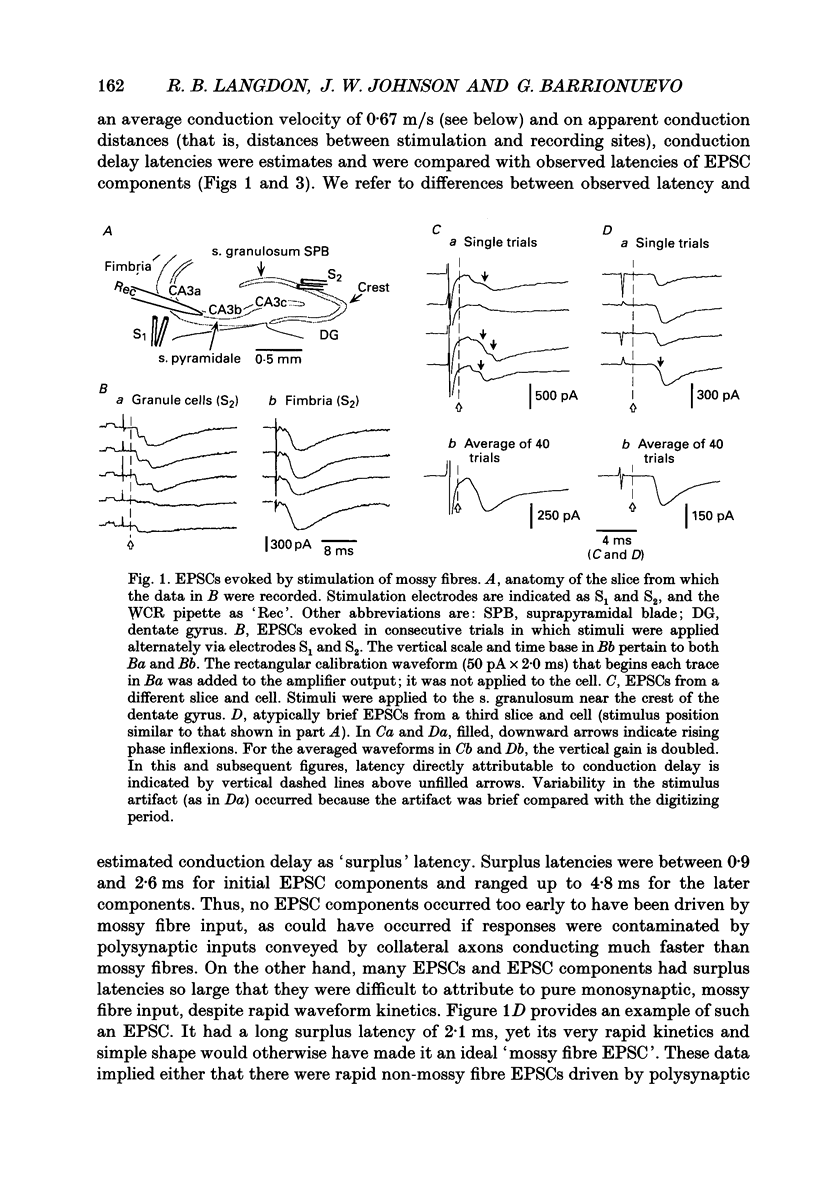
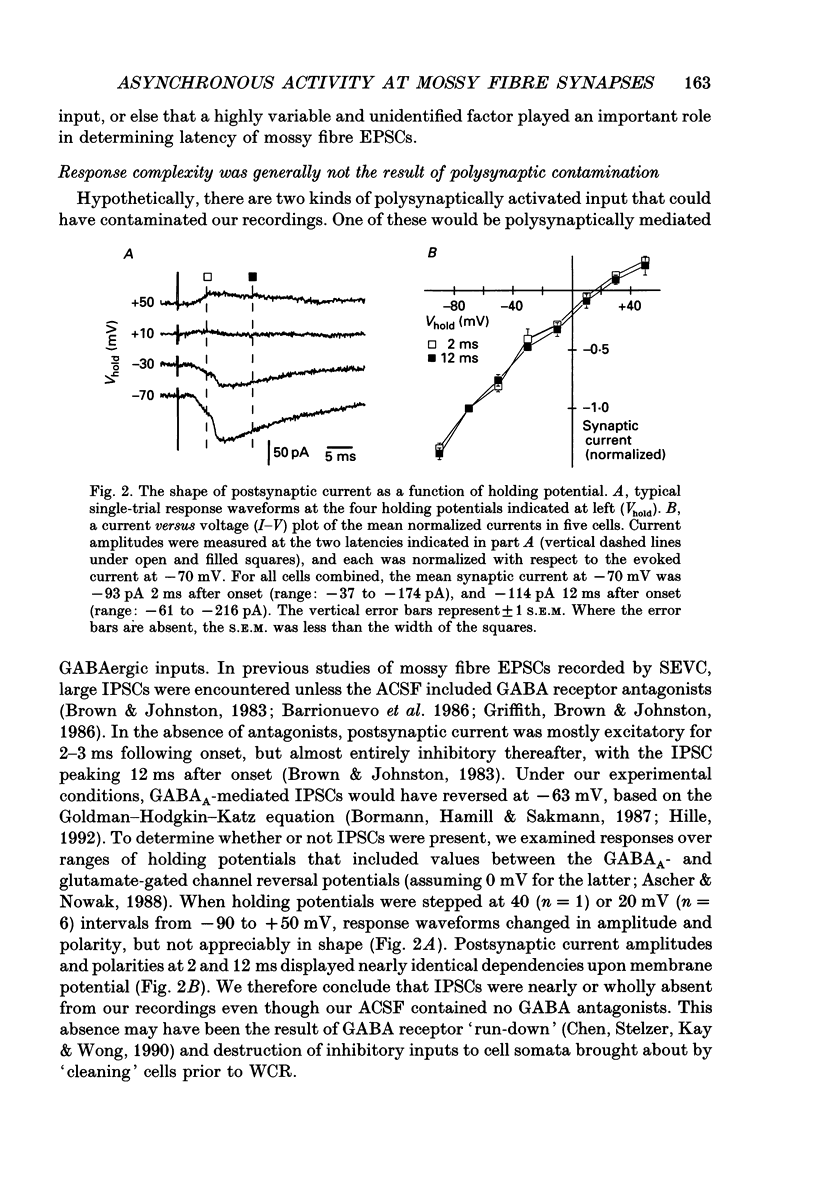
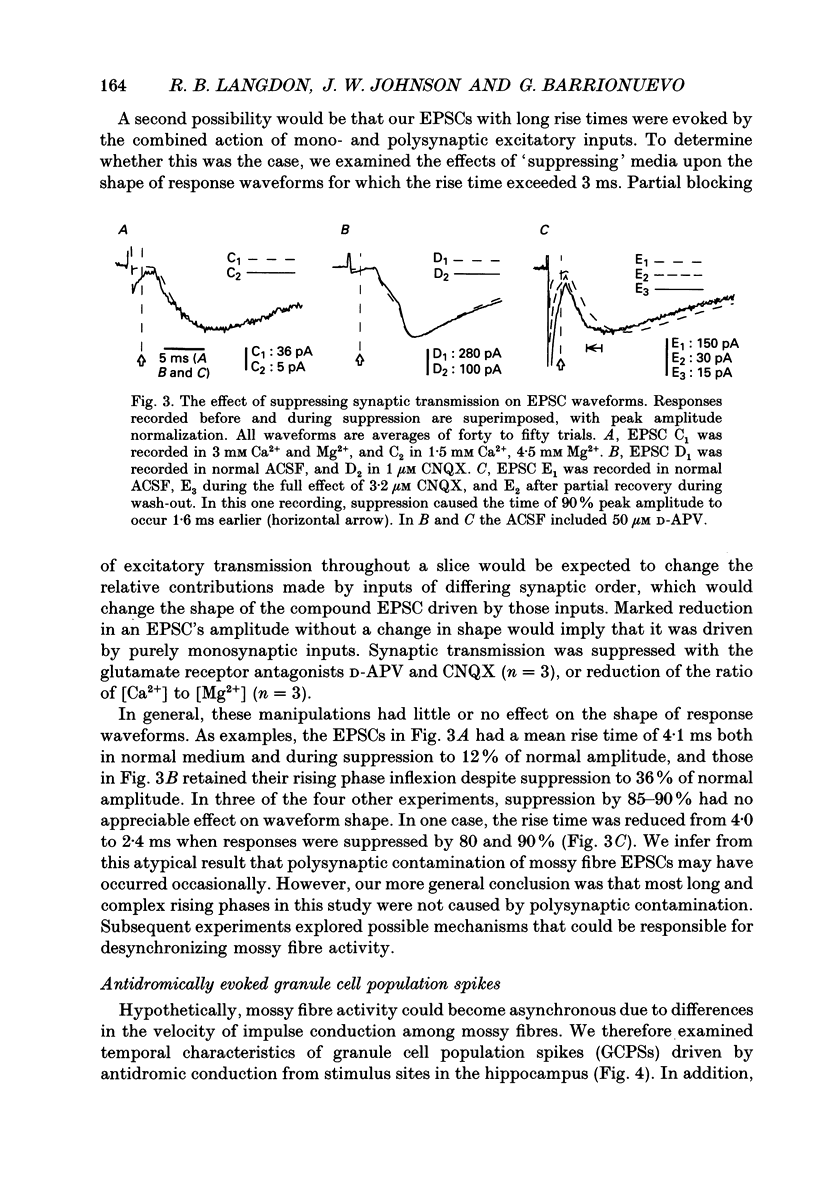
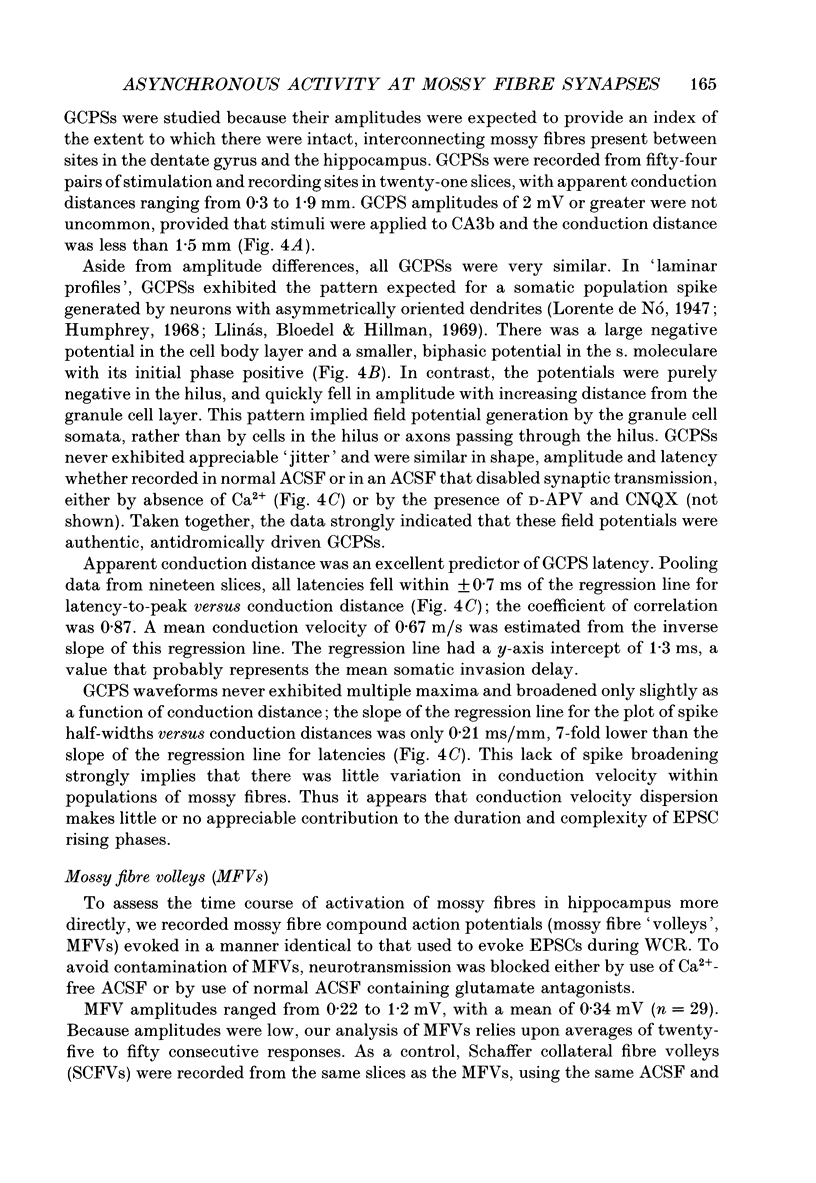
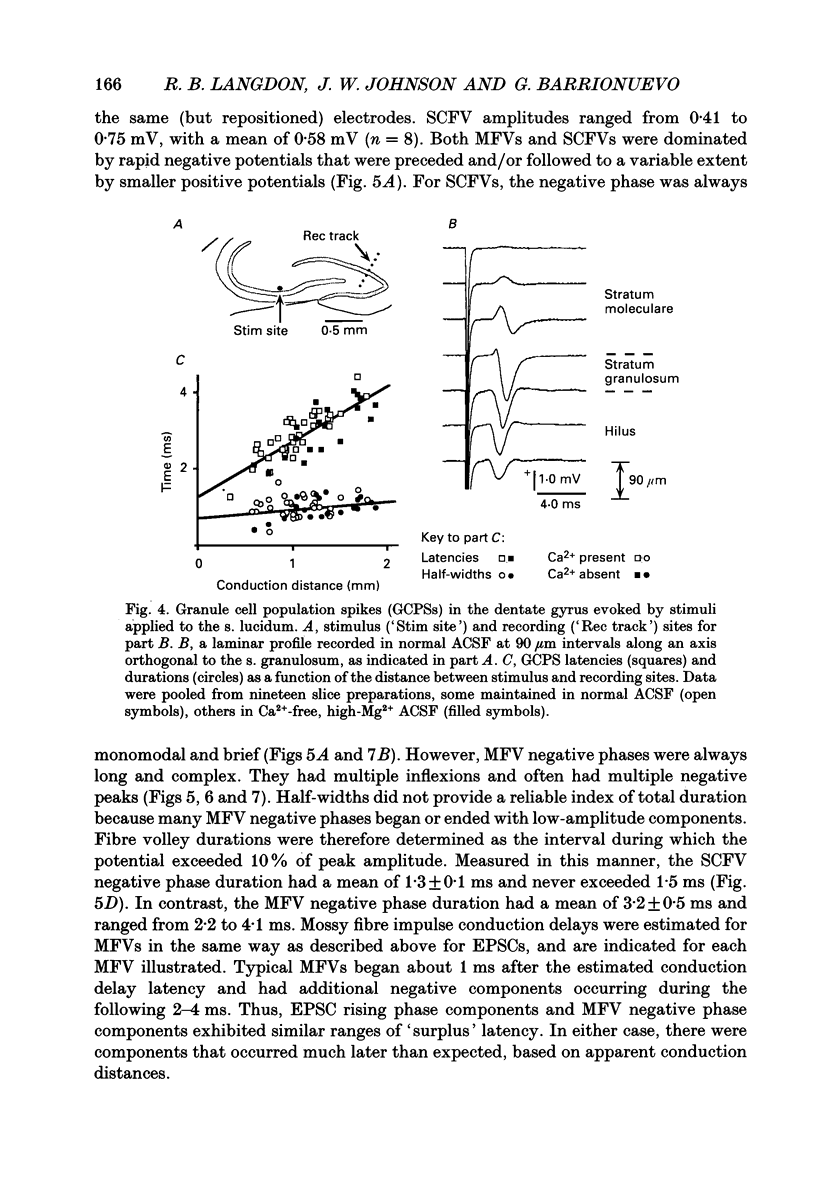
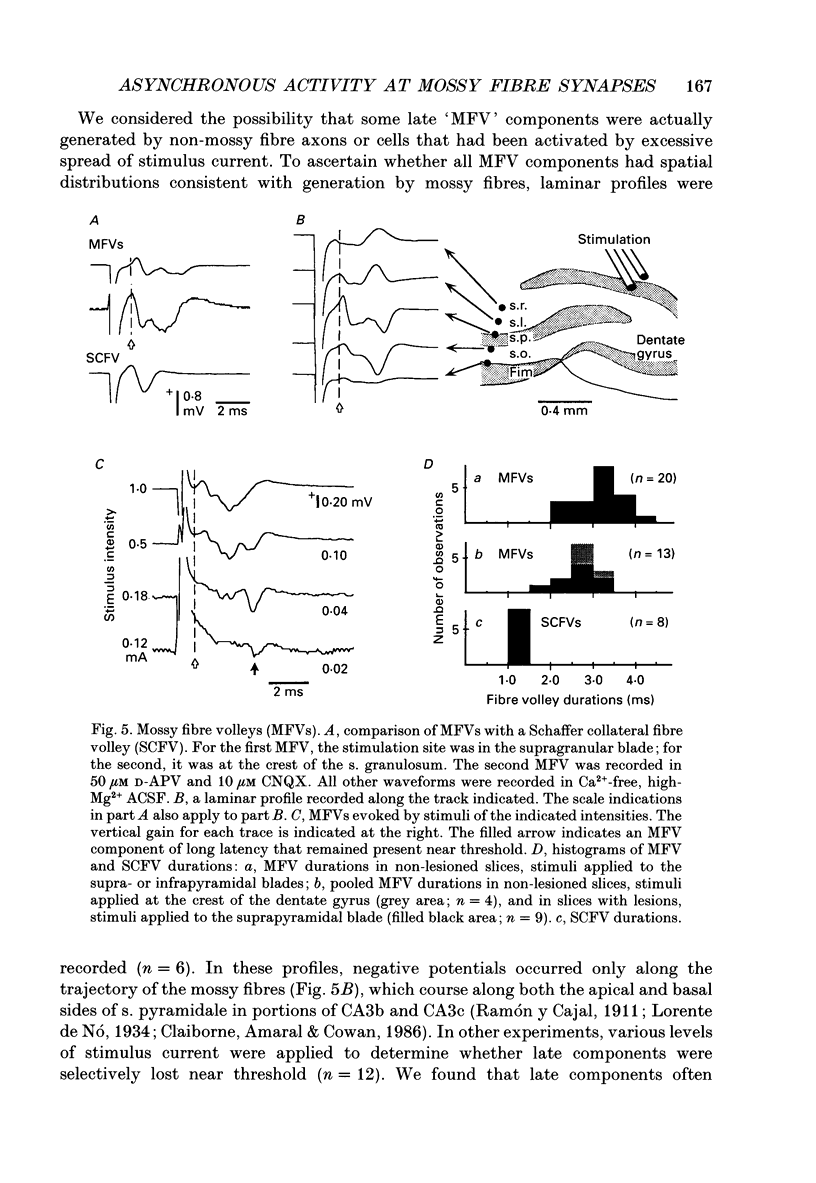
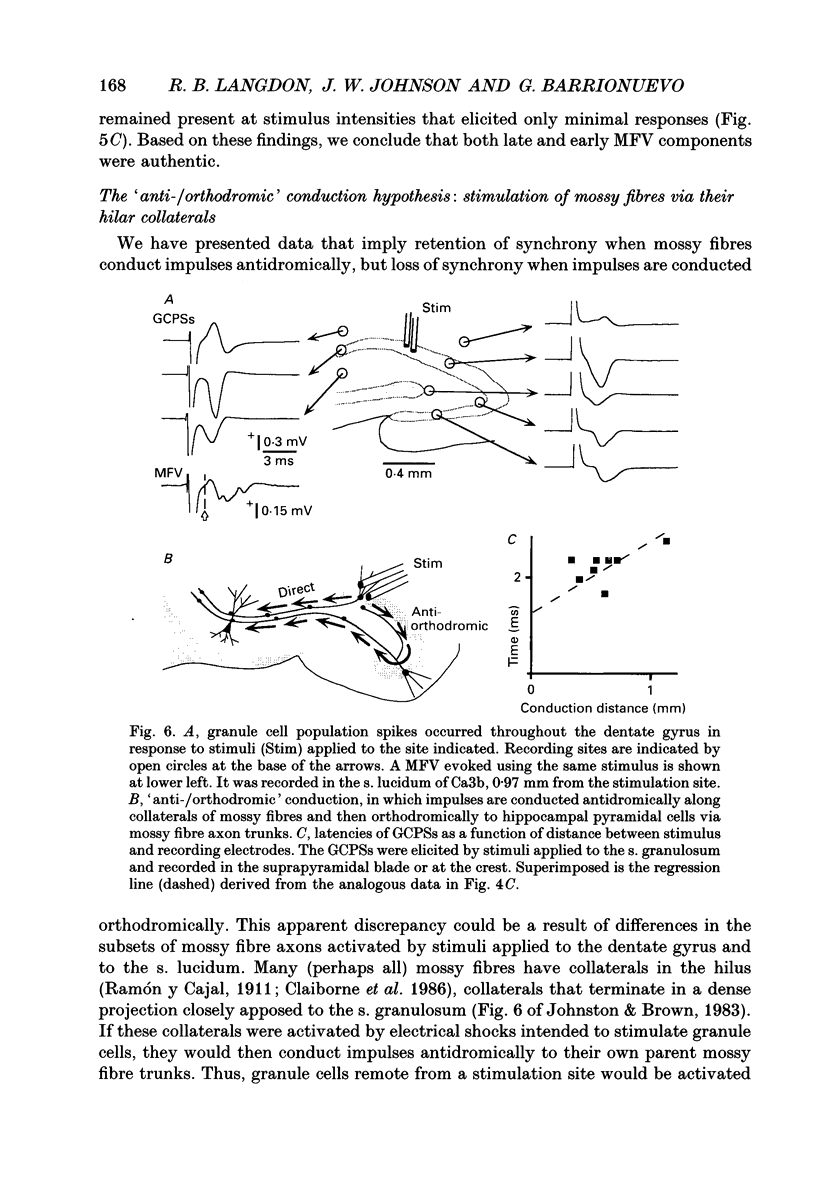
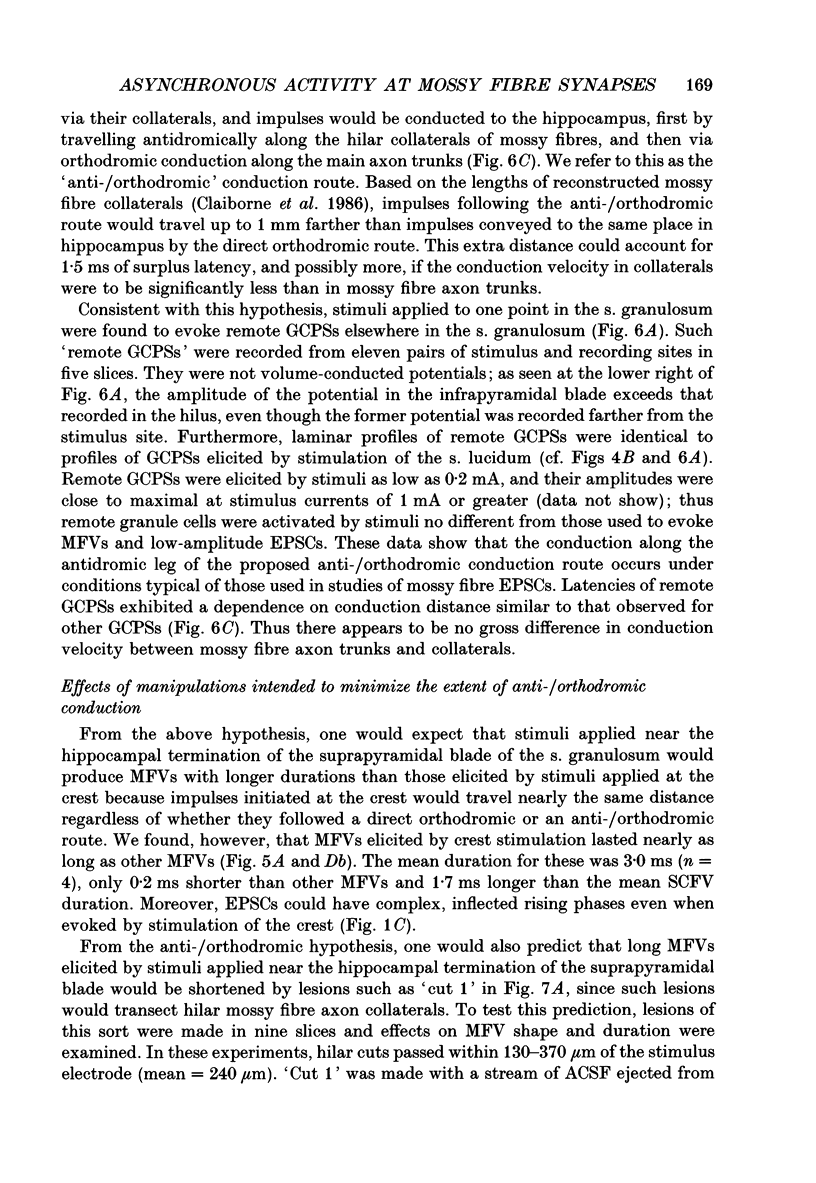
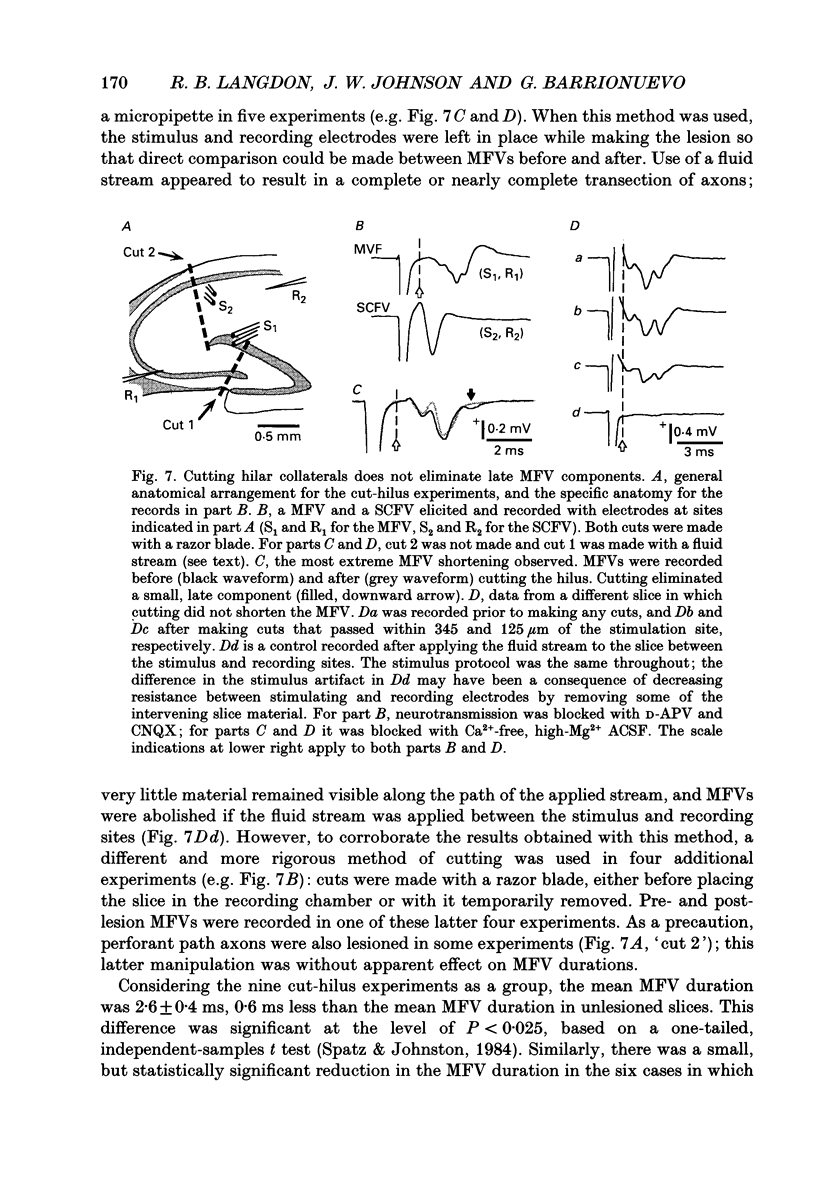
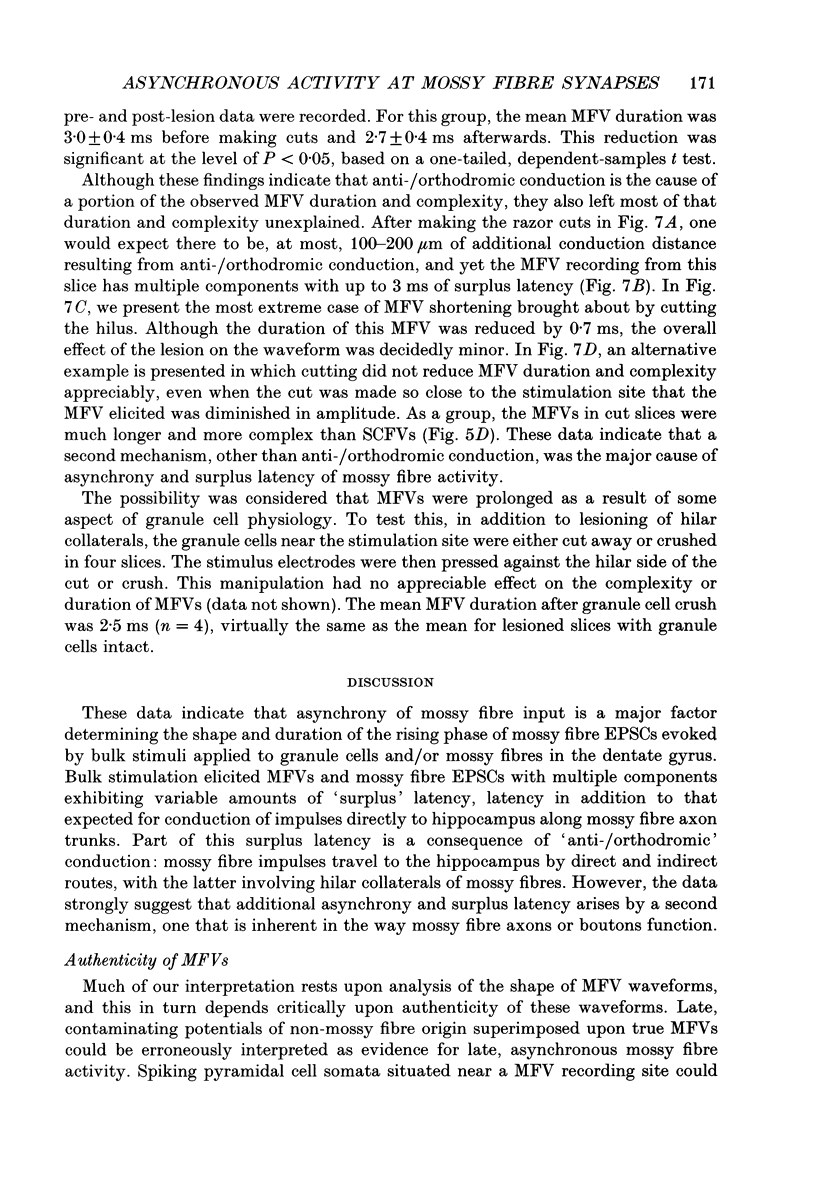
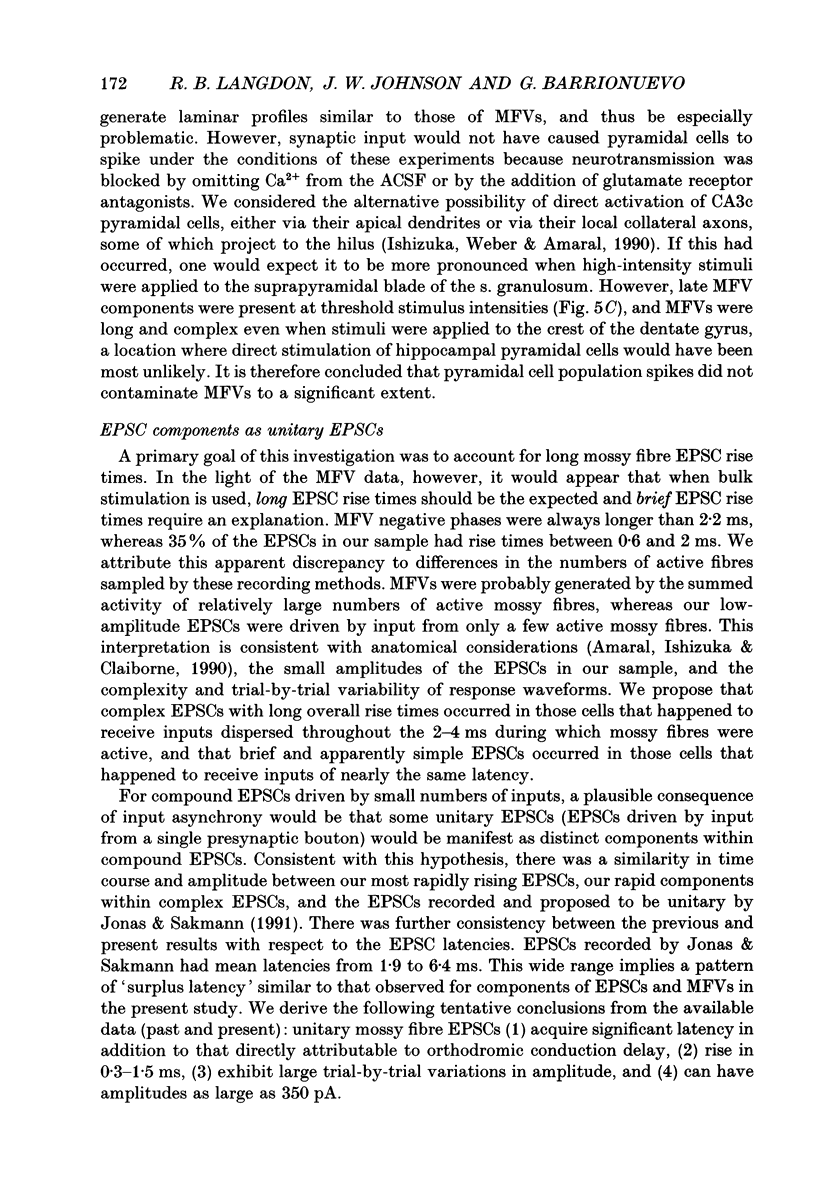
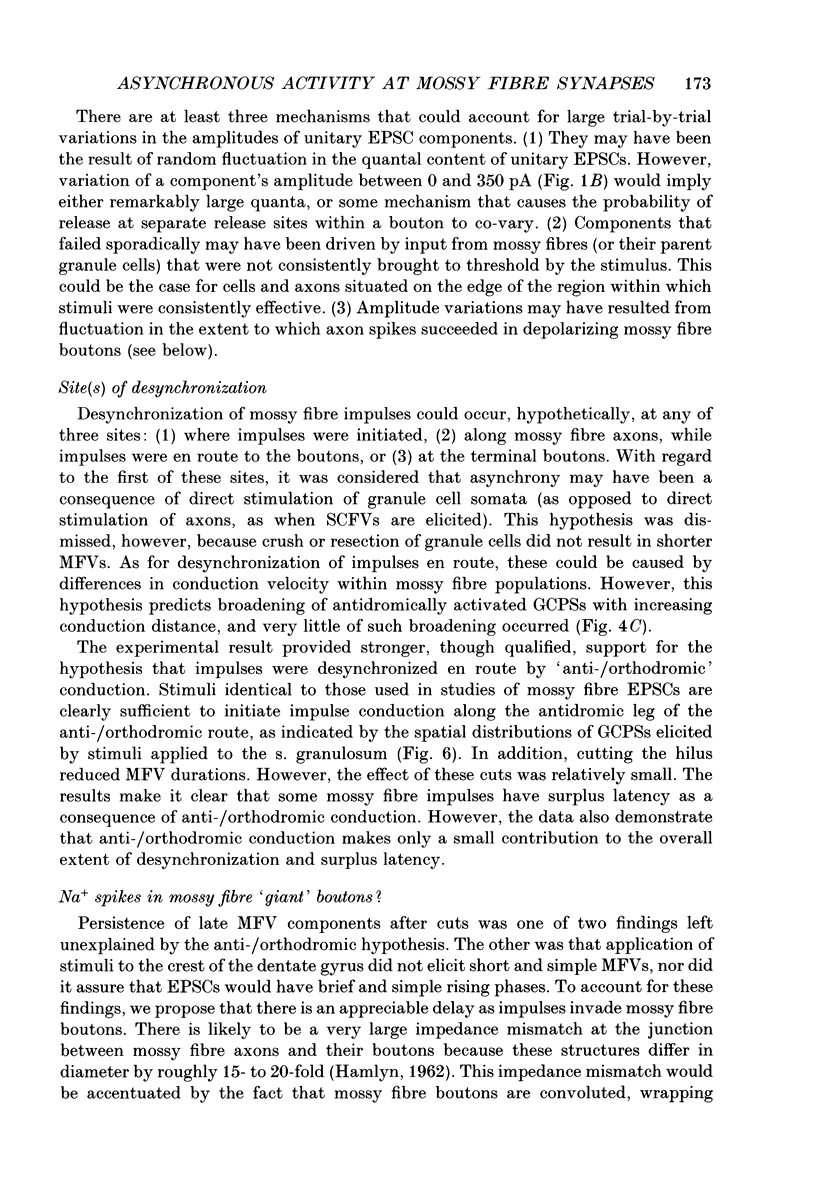
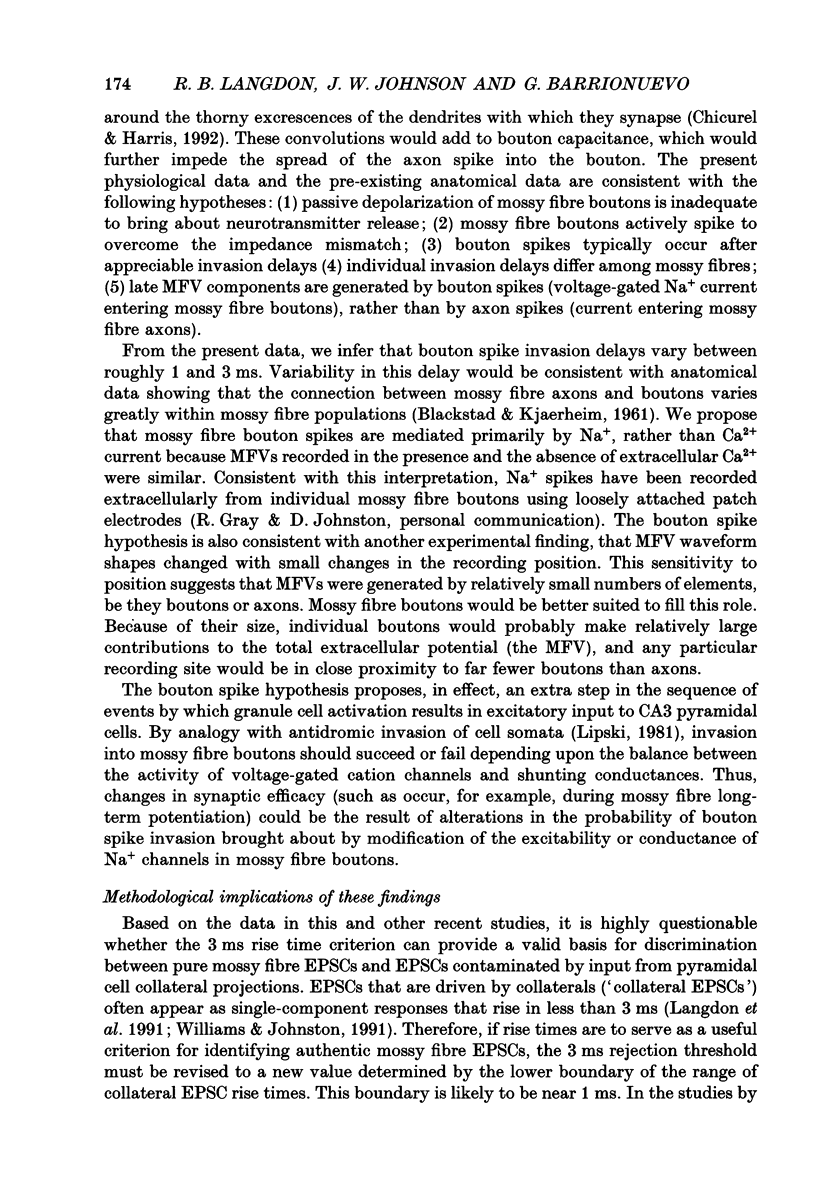
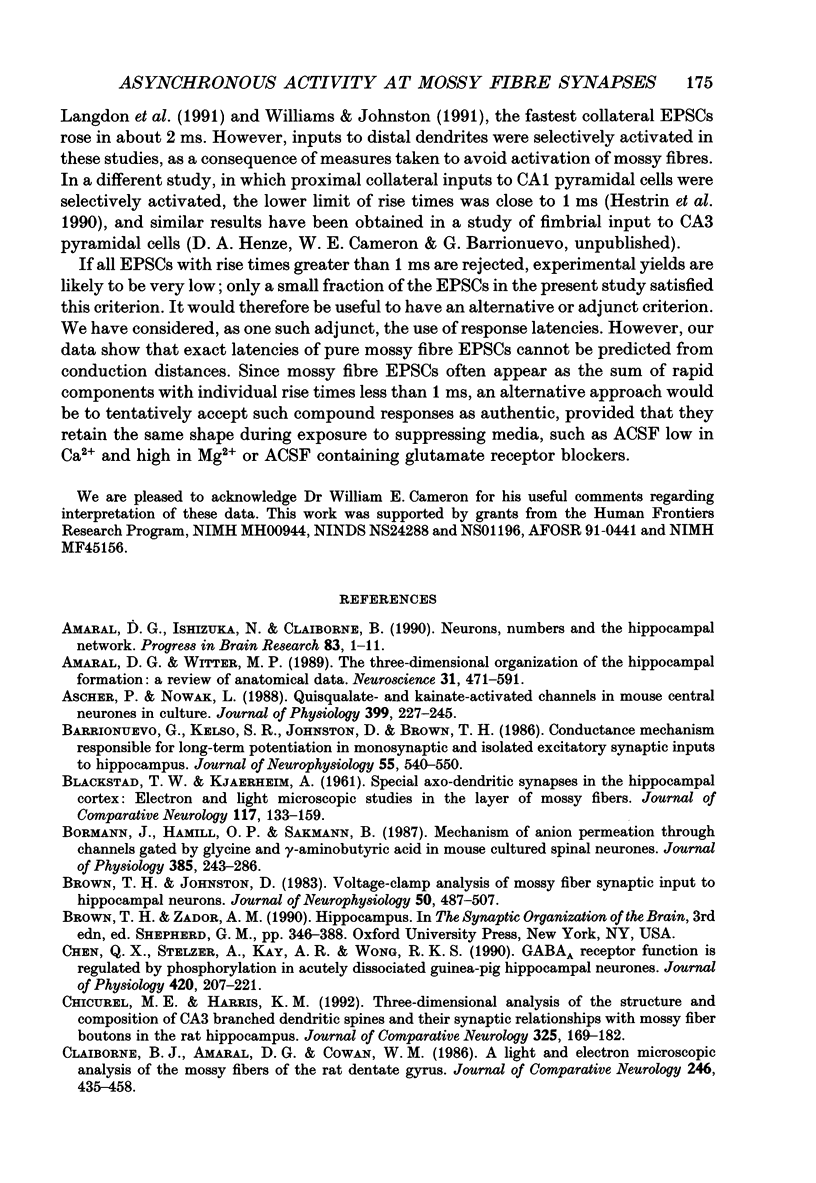
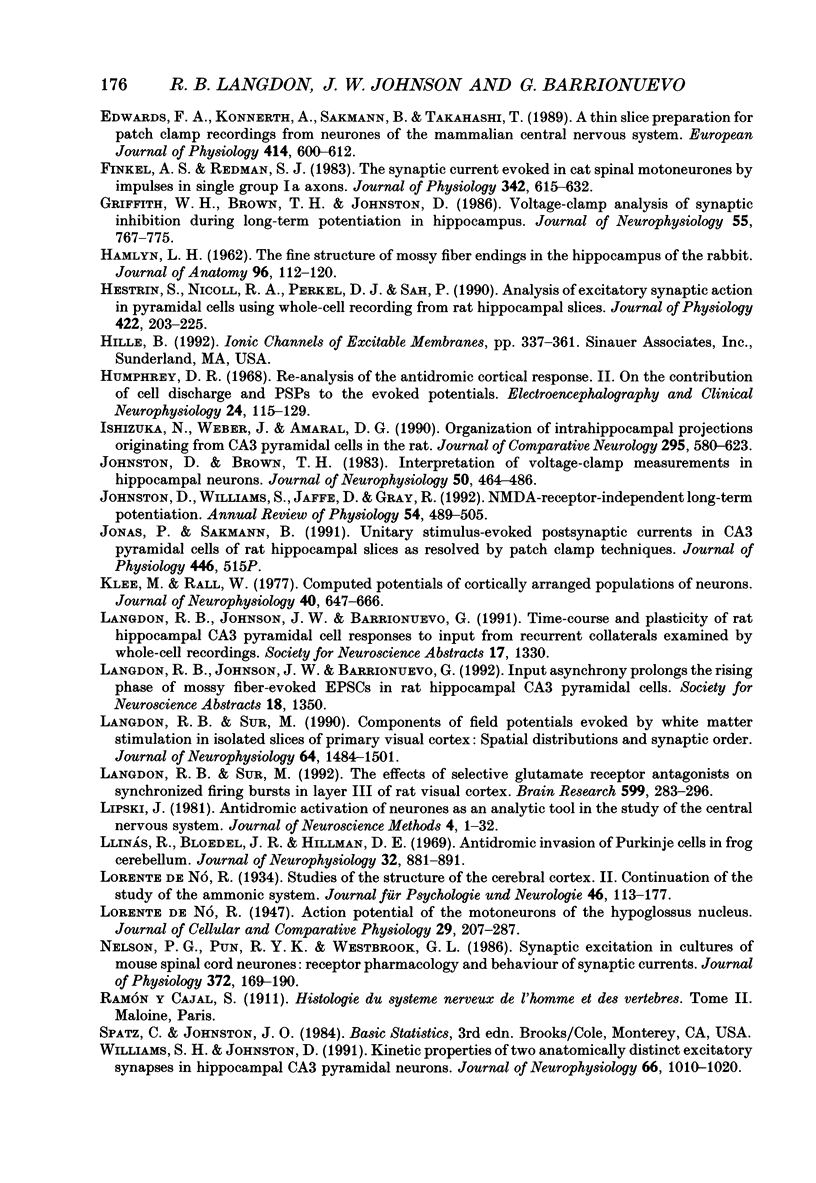
Images in this article
Selected References
These references are in PubMed. This may not be the complete list of references from this article.
- Amaral D. G., Ishizuka N., Claiborne B. Neurons, numbers and the hippocampal network. Prog Brain Res. 1990;83:1–11. doi: 10.1016/s0079-6123(08)61237-6. [DOI] [PubMed] [Google Scholar]
- Amaral D. G., Witter M. P. The three-dimensional organization of the hippocampal formation: a review of anatomical data. Neuroscience. 1989;31(3):571–591. doi: 10.1016/0306-4522(89)90424-7. [DOI] [PubMed] [Google Scholar]
- Ascher P., Nowak L. Quisqualate- and kainate-activated channels in mouse central neurones in culture. J Physiol. 1988 May;399:227–245. doi: 10.1113/jphysiol.1988.sp017077. [DOI] [PMC free article] [PubMed] [Google Scholar]
- BLACKSTAD T. W., KJAERHEIM A. Special axo-dendritic synapses in the hippocampal cortex: electron and light microscopic studies on the layer of mossy fibers. J Comp Neurol. 1961 Oct;117:133–159. doi: 10.1002/cne.901170202. [DOI] [PubMed] [Google Scholar]
- Barrionuevo G., Kelso S. R., Johnston D., Brown T. H. Conductance mechanism responsible for long-term potentiation in monosynaptic and isolated excitatory synaptic inputs to hippocampus. J Neurophysiol. 1986 Mar;55(3):540–550. doi: 10.1152/jn.1986.55.3.540. [DOI] [PubMed] [Google Scholar]
- Bormann J., Hamill O. P., Sakmann B. Mechanism of anion permeation through channels gated by glycine and gamma-aminobutyric acid in mouse cultured spinal neurones. J Physiol. 1987 Apr;385:243–286. doi: 10.1113/jphysiol.1987.sp016493. [DOI] [PMC free article] [PubMed] [Google Scholar]
- Brown T. H., Johnston D. Voltage-clamp analysis of mossy fiber synaptic input to hippocampal neurons. J Neurophysiol. 1983 Aug;50(2):487–507. doi: 10.1152/jn.1983.50.2.487. [DOI] [PubMed] [Google Scholar]
- Chen Q. X., Stelzer A., Kay A. R., Wong R. K. GABAA receptor function is regulated by phosphorylation in acutely dissociated guinea-pig hippocampal neurones. J Physiol. 1990 Jan;420:207–221. doi: 10.1113/jphysiol.1990.sp017908. [DOI] [PMC free article] [PubMed] [Google Scholar]
- Chicurel M. E., Harris K. M. Three-dimensional analysis of the structure and composition of CA3 branched dendritic spines and their synaptic relationships with mossy fiber boutons in the rat hippocampus. J Comp Neurol. 1992 Nov 8;325(2):169–182. doi: 10.1002/cne.903250204. [DOI] [PubMed] [Google Scholar]
- Claiborne B. J., Amaral D. G., Cowan W. M. A light and electron microscopic analysis of the mossy fibers of the rat dentate gyrus. J Comp Neurol. 1986 Apr 22;246(4):435–458. doi: 10.1002/cne.902460403. [DOI] [PubMed] [Google Scholar]
- Edwards F. A., Konnerth A., Sakmann B., Takahashi T. A thin slice preparation for patch clamp recordings from neurones of the mammalian central nervous system. Pflugers Arch. 1989 Sep;414(5):600–612. doi: 10.1007/BF00580998. [DOI] [PubMed] [Google Scholar]
- Finkel A. S., Redman S. J. The synaptic current evoked in cat spinal motoneurones by impulses in single group 1a axons. J Physiol. 1983 Sep;342:615–632. doi: 10.1113/jphysiol.1983.sp014872. [DOI] [PMC free article] [PubMed] [Google Scholar]
- Griffith W. H., Brown T. H., Johnston D. Voltage-clamp analysis of synaptic inhibition during long-term potentiation in hippocampus. J Neurophysiol. 1986 Apr;55(4):767–775. doi: 10.1152/jn.1986.55.4.767. [DOI] [PubMed] [Google Scholar]
- HAMLYN L. H. The fine structure of the mossy fibre endings in the hippocampus of the rabbit. J Anat. 1962 Jan;96:112–120. [PMC free article] [PubMed] [Google Scholar]
- Hestrin S., Nicoll R. A., Perkel D. J., Sah P. Analysis of excitatory synaptic action in pyramidal cells using whole-cell recording from rat hippocampal slices. J Physiol. 1990 Mar;422:203–225. doi: 10.1113/jphysiol.1990.sp017980. [DOI] [PMC free article] [PubMed] [Google Scholar]
- Ishizuka N., Weber J., Amaral D. G. Organization of intrahippocampal projections originating from CA3 pyramidal cells in the rat. J Comp Neurol. 1990 May 22;295(4):580–623. doi: 10.1002/cne.902950407. [DOI] [PubMed] [Google Scholar]
- Johnston D., Brown T. H. Interpretation of voltage-clamp measurements in hippocampal neurons. J Neurophysiol. 1983 Aug;50(2):464–486. doi: 10.1152/jn.1983.50.2.464. [DOI] [PubMed] [Google Scholar]
- Johnston D., Williams S., Jaffe D., Gray R. NMDA-receptor-independent long-term potentiation. Annu Rev Physiol. 1992;54:489–505. doi: 10.1146/annurev.ph.54.030192.002421. [DOI] [PubMed] [Google Scholar]
- Klee M., Rall W. Computed potentials of cortically arranged populations of neurons. J Neurophysiol. 1977 May;40(3):647–666. doi: 10.1152/jn.1977.40.3.647. [DOI] [PubMed] [Google Scholar]
- Langdon R. B., Sur M. Components of field potentials evoked by white matter stimulation in isolated slices of primary visual cortex: spatial distributions and synaptic order. J Neurophysiol. 1990 Nov;64(5):1484–1501. doi: 10.1152/jn.1990.64.5.1484. [DOI] [PubMed] [Google Scholar]
- Langdon R. B., Sur M. The effects of selective glutamate receptor antagonists on synchronized firing bursts in layer III of rat visual cortex. Brain Res. 1992 Dec 25;599(2):283–296. doi: 10.1016/0006-8993(92)90403-v. [DOI] [PubMed] [Google Scholar]
- Lipski J. Antidromic activation of neurones as an analytic tool in the study of the central nervous system. J Neurosci Methods. 1981 Jun;4(1):1–32. doi: 10.1016/0165-0270(81)90015-7. [DOI] [PubMed] [Google Scholar]
- Llinas R., Bloedel J. R., Roberts W. Antidromic invasion of Purkinje cells in frog cerebellum. J Neurophysiol. 1969 Nov;32(6):881–891. doi: 10.1152/jn.1969.32.6.881. [DOI] [PubMed] [Google Scholar]
- Nelson P. G., Pun R. Y., Westbrook G. L. Synaptic excitation in cultures of mouse spinal cord neurones: receptor pharmacology and behaviour of synaptic currents. J Physiol. 1986 Mar;372:169–190. doi: 10.1113/jphysiol.1986.sp016003. [DOI] [PMC free article] [PubMed] [Google Scholar]
- Williams S. H., Johnston D. Kinetic properties of two anatomically distinct excitatory synapses in hippocampal CA3 pyramidal neurons. J Neurophysiol. 1991 Sep;66(3):1010–1020. doi: 10.1152/jn.1991.66.3.1010. [DOI] [PubMed] [Google Scholar]