Abstract
1. Voltage changes associated with currents crossing the internodal axolemma were monitored using a microelectrode inserted into the myelin sheath (peri-internodal region) of rat phrenic nerve fibres. This microelectrode was also used to change the potential and the ionic environment in the peri-internodal region. 2. Following stimulation of the proximal nerve trunk, the peri-internodal electrode recorded a positive-going action potential whose amplitude increased (up to 75 mV) with increasing depth of microelectrode penetration into the myelin. The resting potential recorded by the peri-internodal electrode remained within 4 mV of bath ground. 3. Confocal imaging of fibres injected peri-internodally with the fluorescent dye Lucifer Yellow revealed a staining pattern consistent with spread of dye throughout the myelin sheath of the injected internode. 4. After ionophoresis of K+ (but not Na+) into the peri-internodal region, the action potential was followed by a prolonged negative potential (PNP) lasting hundreds of milliseconds to several seconds. The duration of the PNP increased as the frequency of stimulation decreased. PNPs could also be evoked by sub-threshold depolarization of the internodal axolemma with peri-internodally applied current pulses. In the absence of action potentials or applied depolarization PNPs sometimes appeared spontaneously. 5. Peri-internodal application of Rb+ also produced evoked and spontaneous PNPs. These PNPs had longer durations (up to 20 s) than those recorded from K(+)-loaded internodes. 6. Spontaneous action potentials sometimes appeared during the onset of the PNP, suggesting that PNPs are associated with depolarization of the underlying axon. 7. Passage of current pulses during the PNP demonstrated that the PNP is associated with an increased conductance of the pathway linking the peri-internodal recording site to the bath. At least part of this conductance increase occurs across the internodal axolemma, since peri-internodally recorded action potentials evoked during the PNP had larger amplitudes than those evoked before or after the PNP. 8. PNPs were suppressed by tetraethylammonium (TEA, 10-20 mM) and by 4-aminopyridine (1 mM). 9. These results suggest that the PNPs recorded in K(+)- or Rb(+)-loaded myelin sheaths are produced by a regenerative K+ or Rb+ current that enters the internodal axolemma via K+ channels opened by action potentials or subthreshold depolarizations. 10. When normal extracellular [K+] was preserved (by using Na+ rather than K+ salts in the peri-internodal electrode), action potentials recorded within the myelin sheath were instead followed by a brief, positive after-potential that was inhibited by TEA.(ABSTRACT TRUNCATED AT 400 WORDS)
Full text
PDF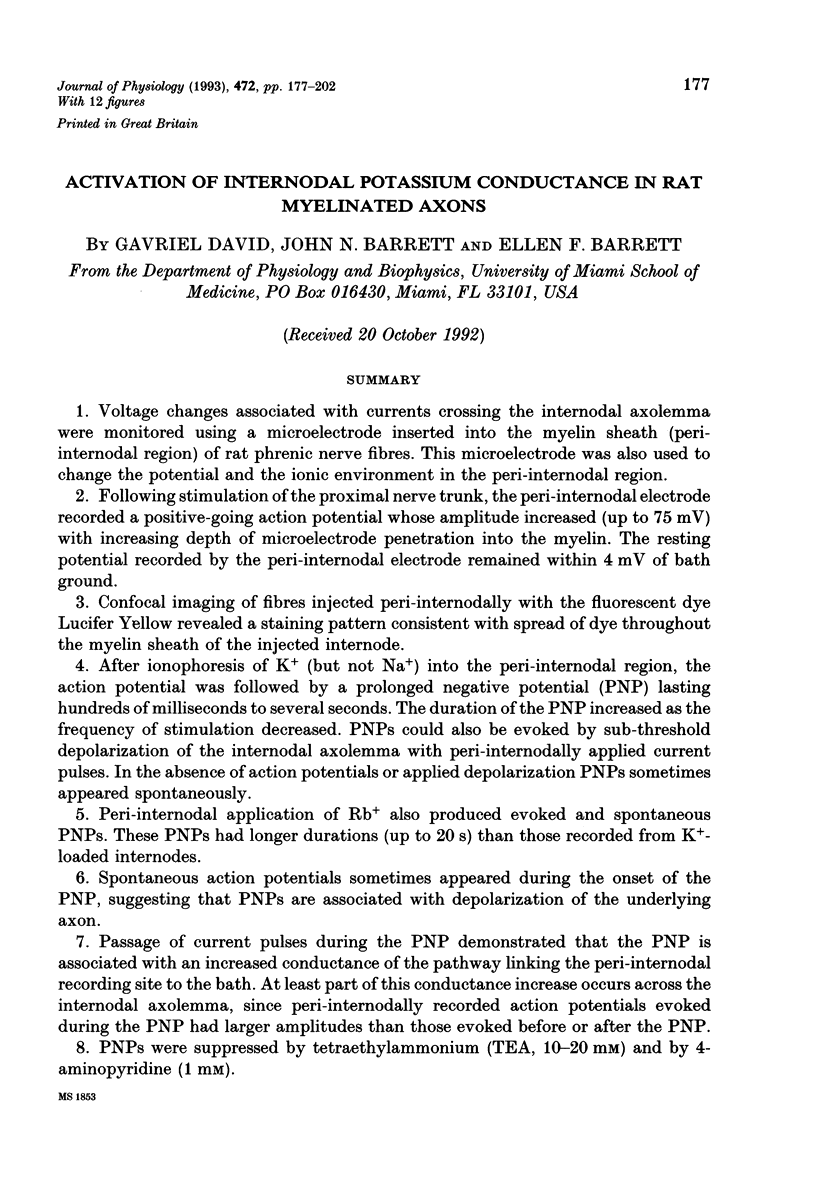
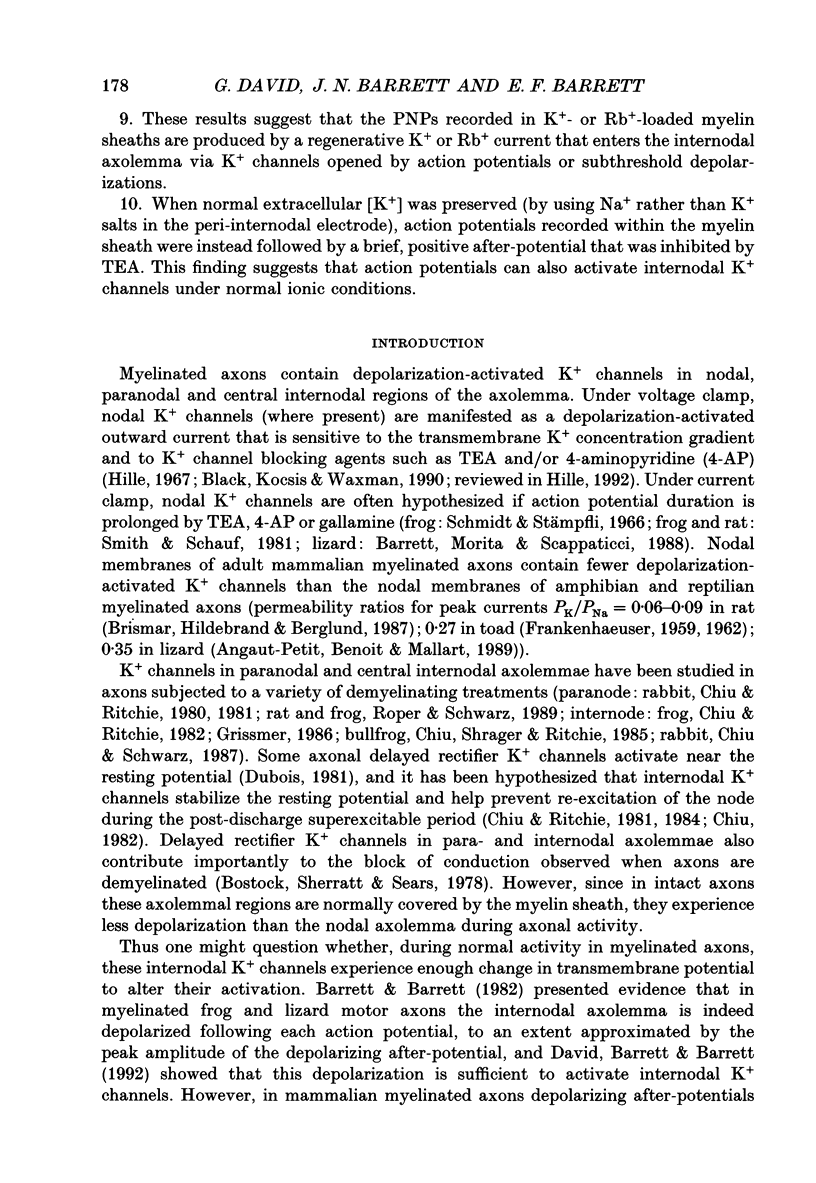
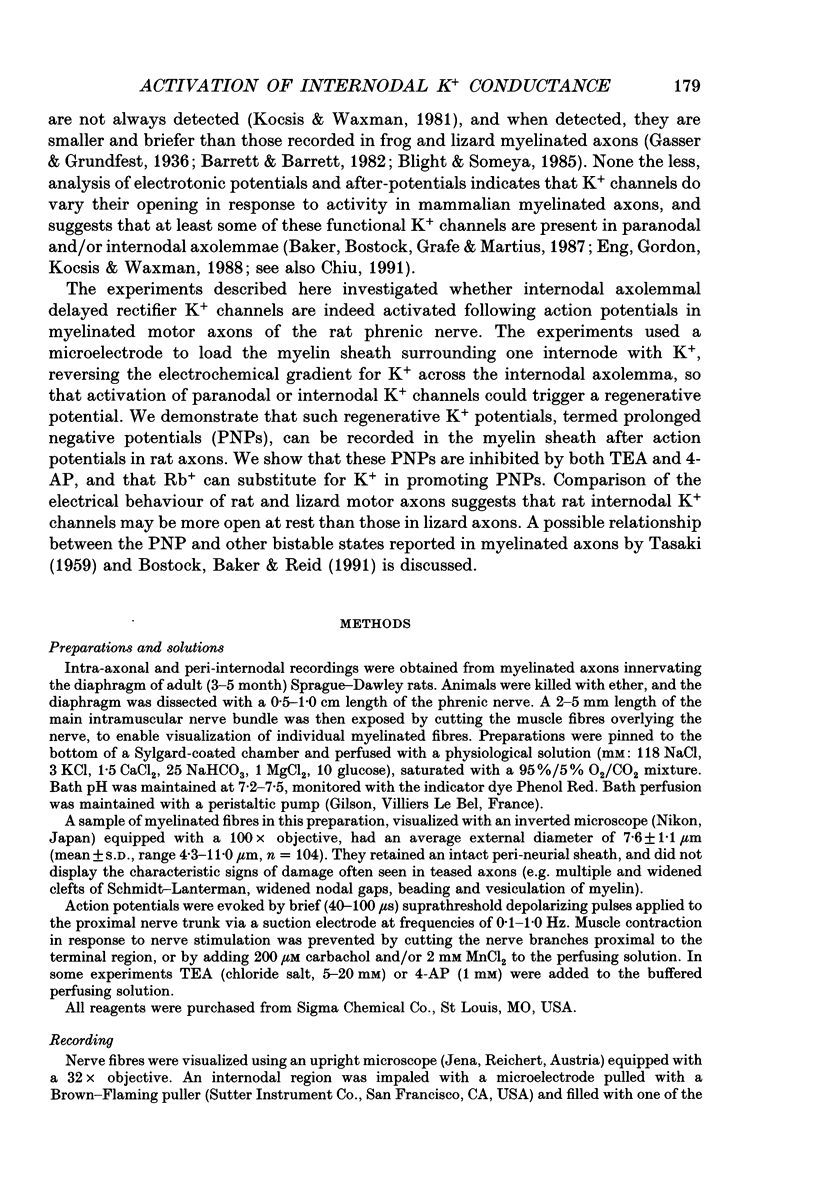
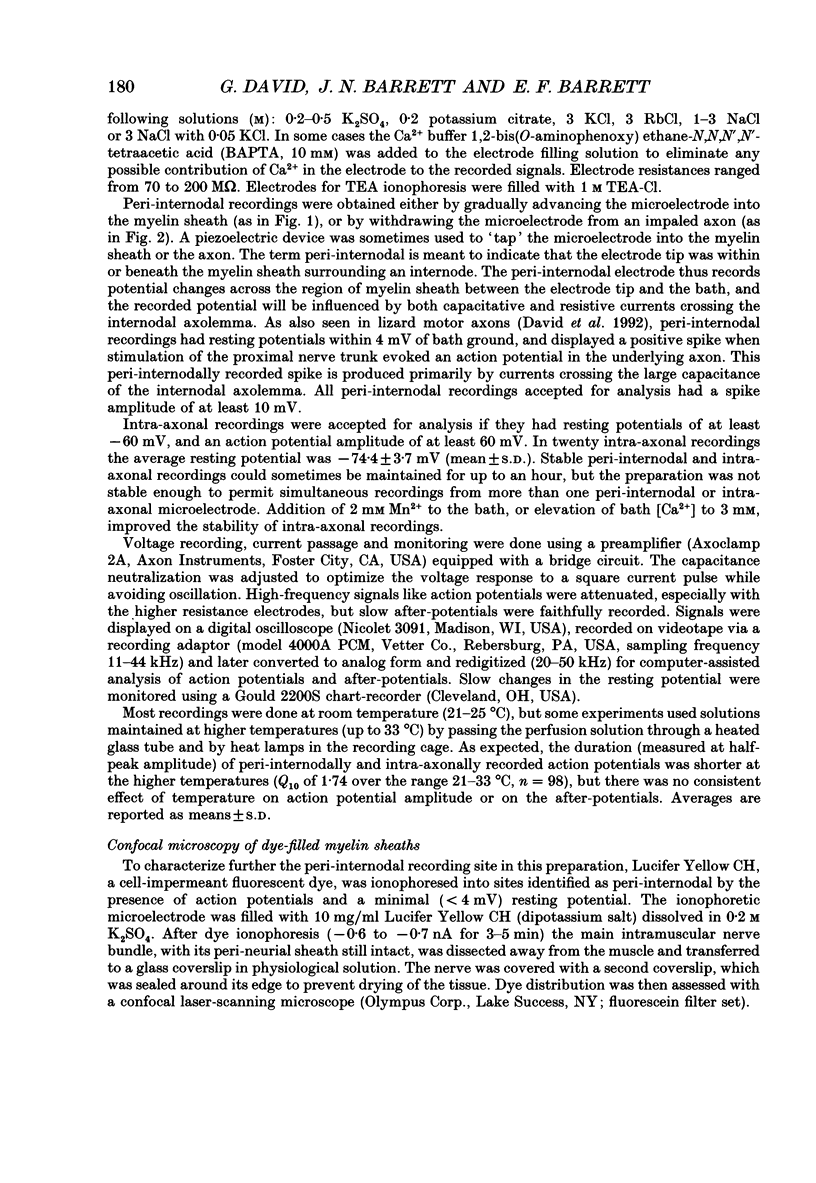
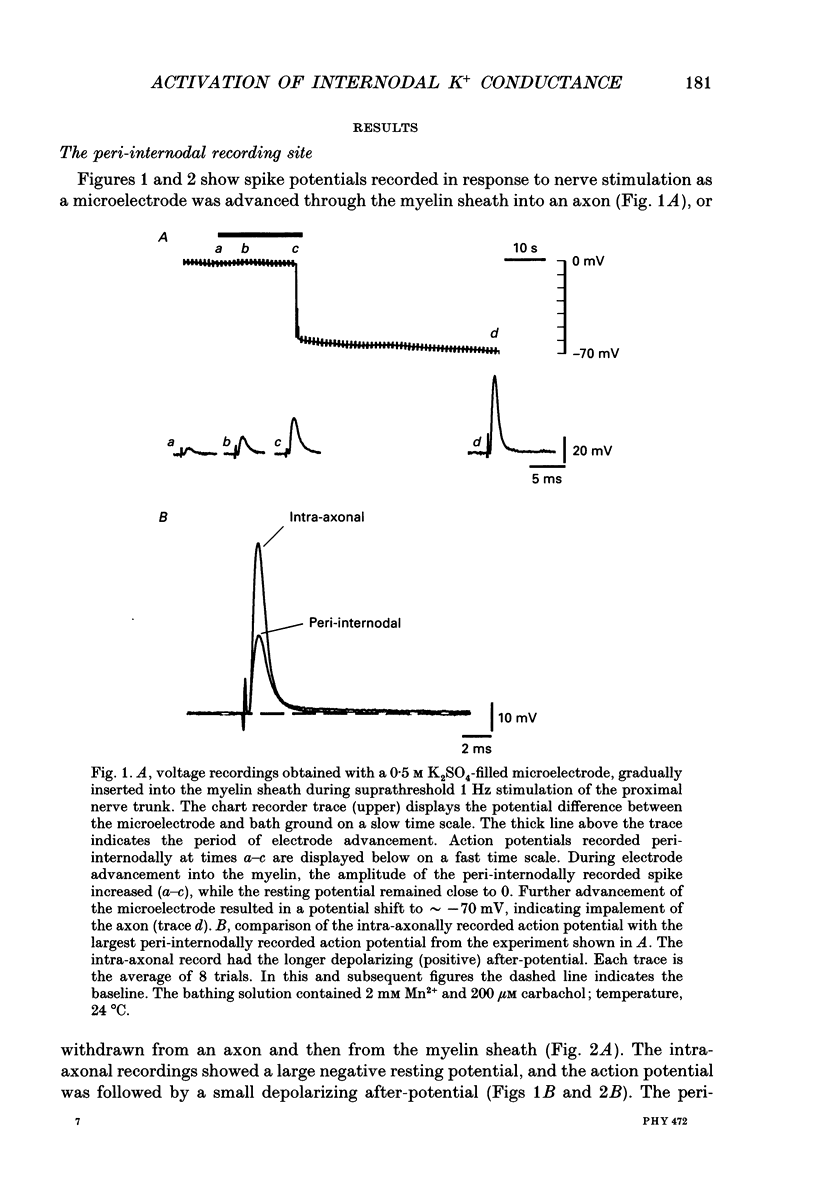
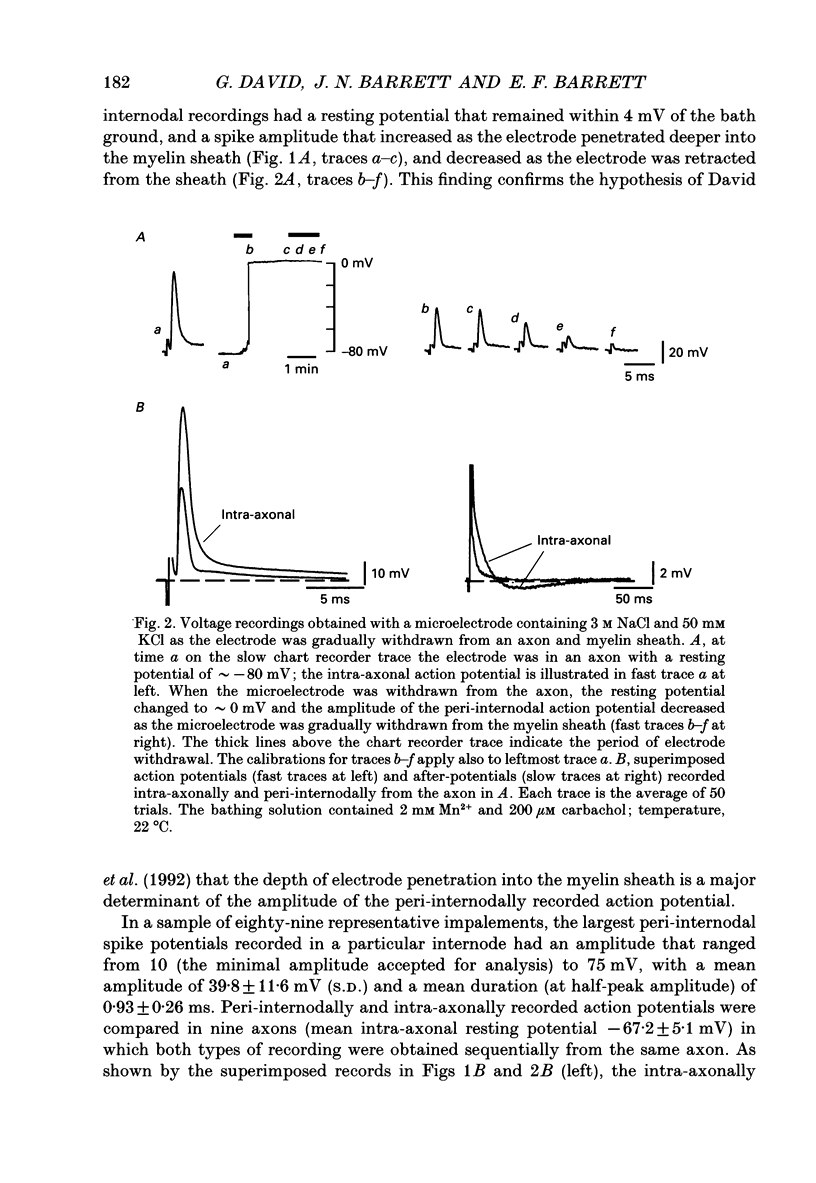
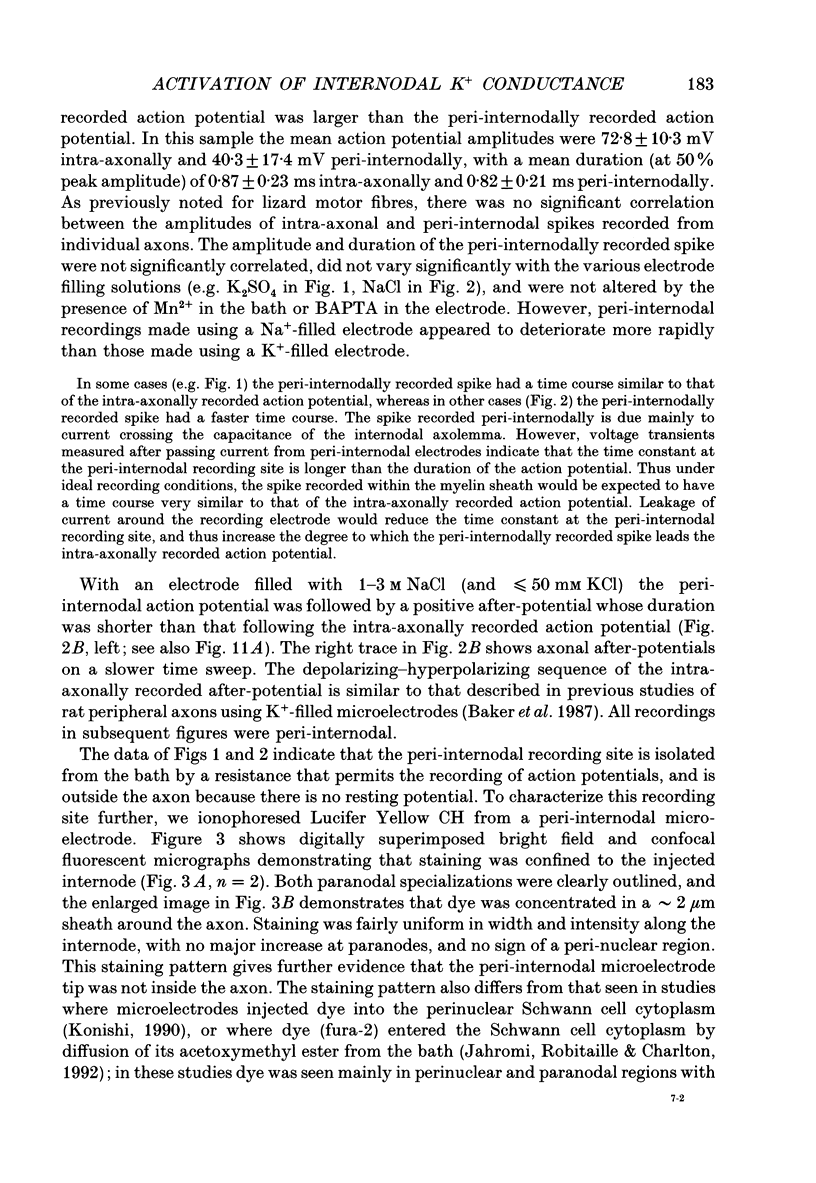
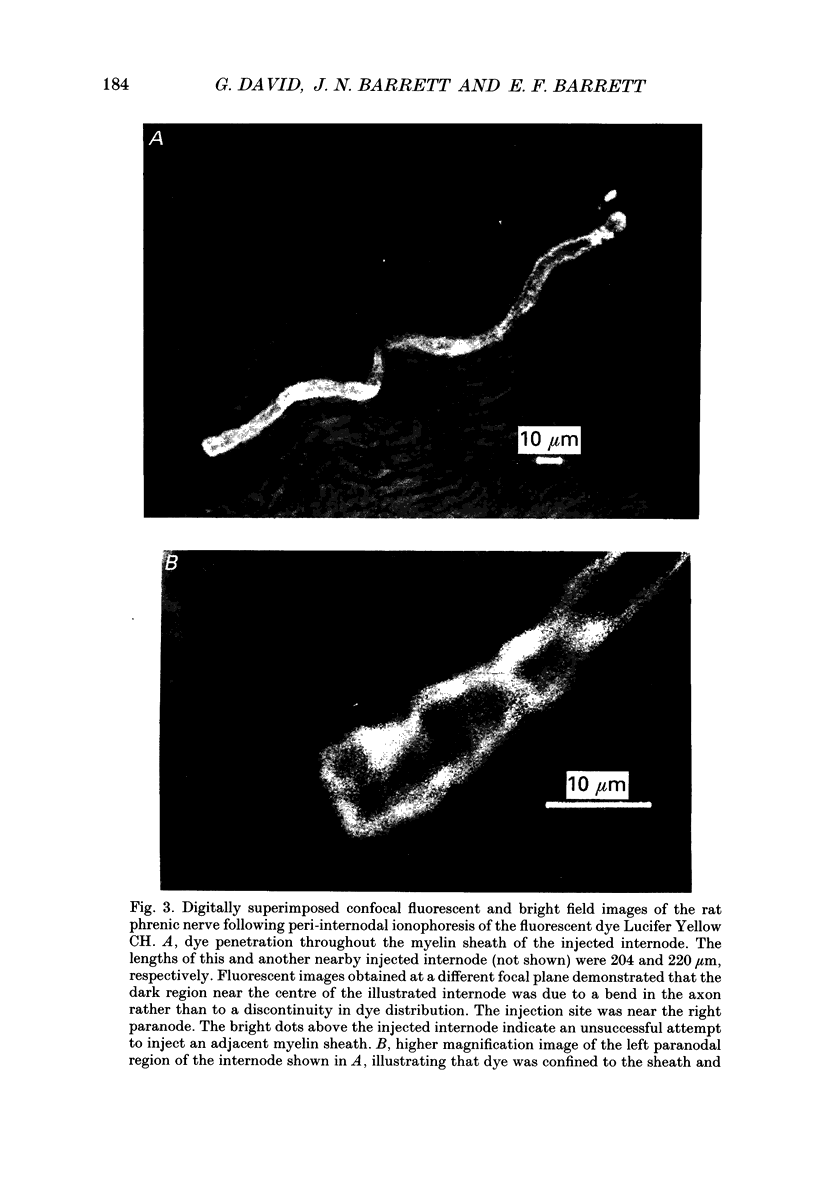
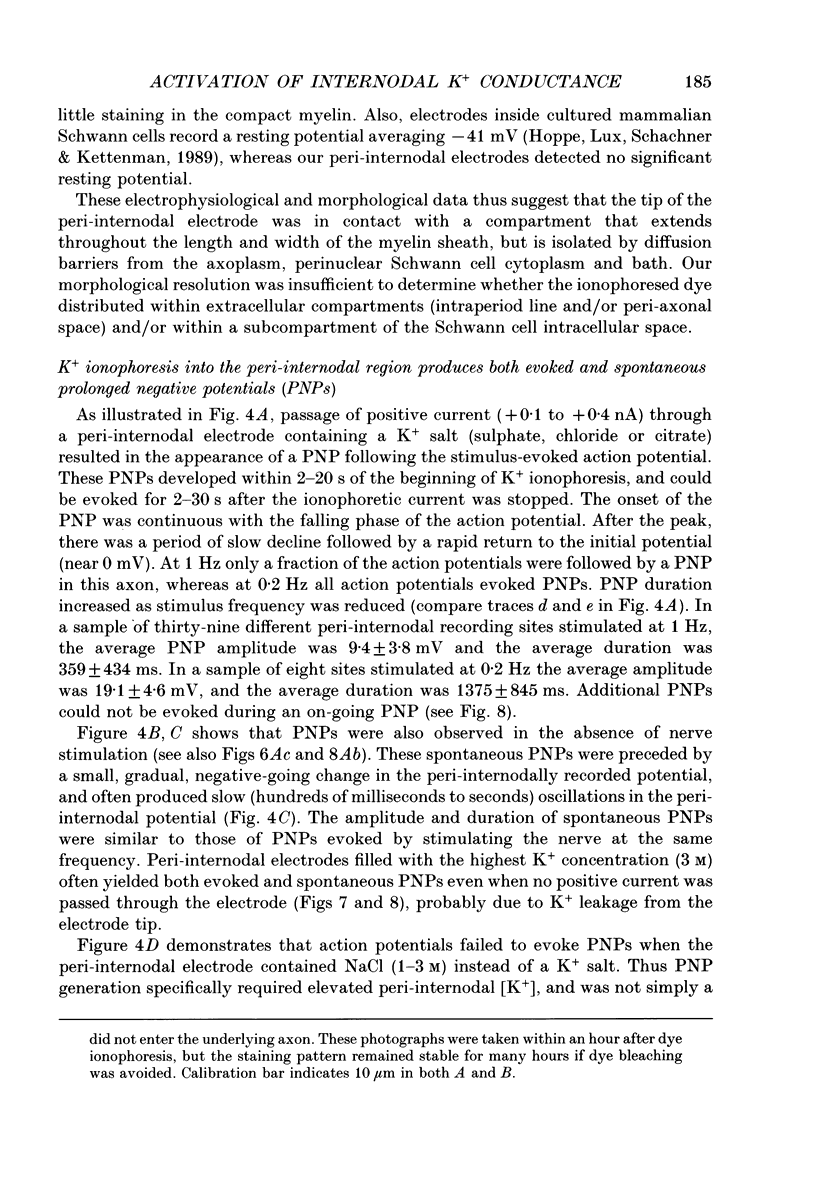
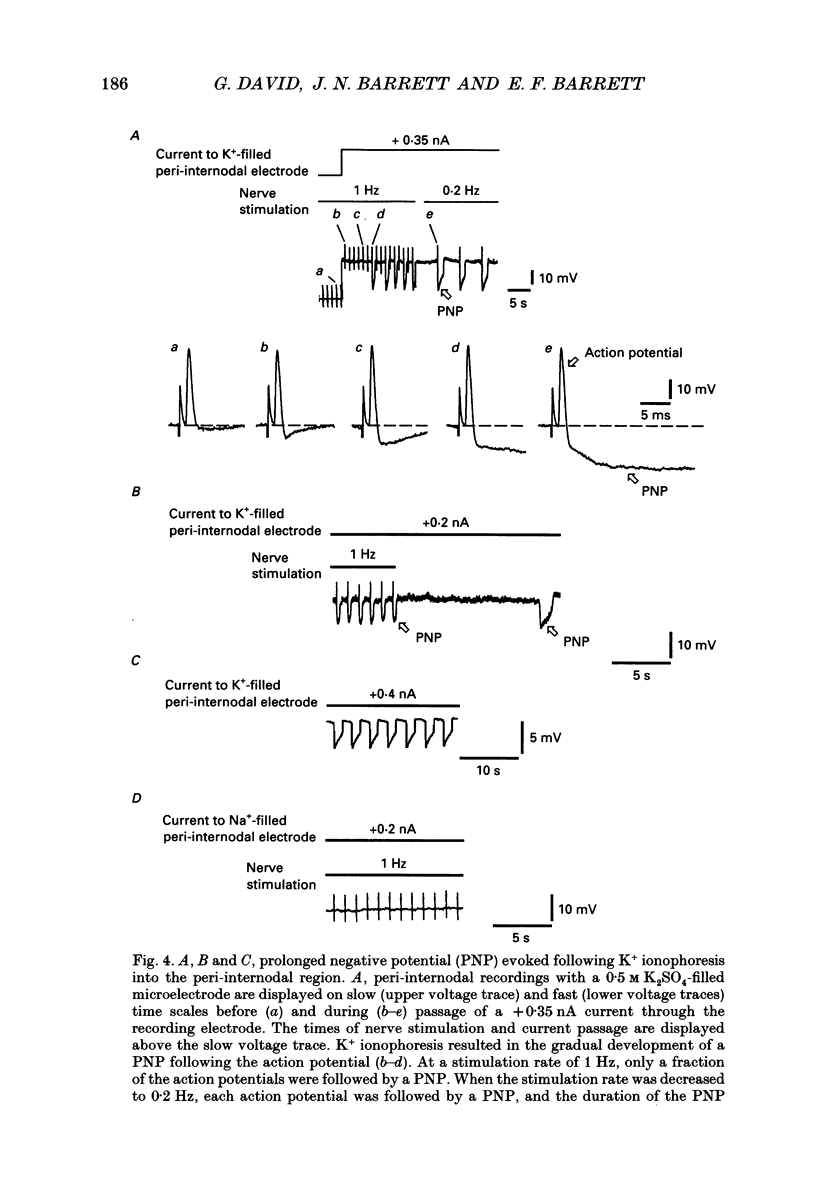
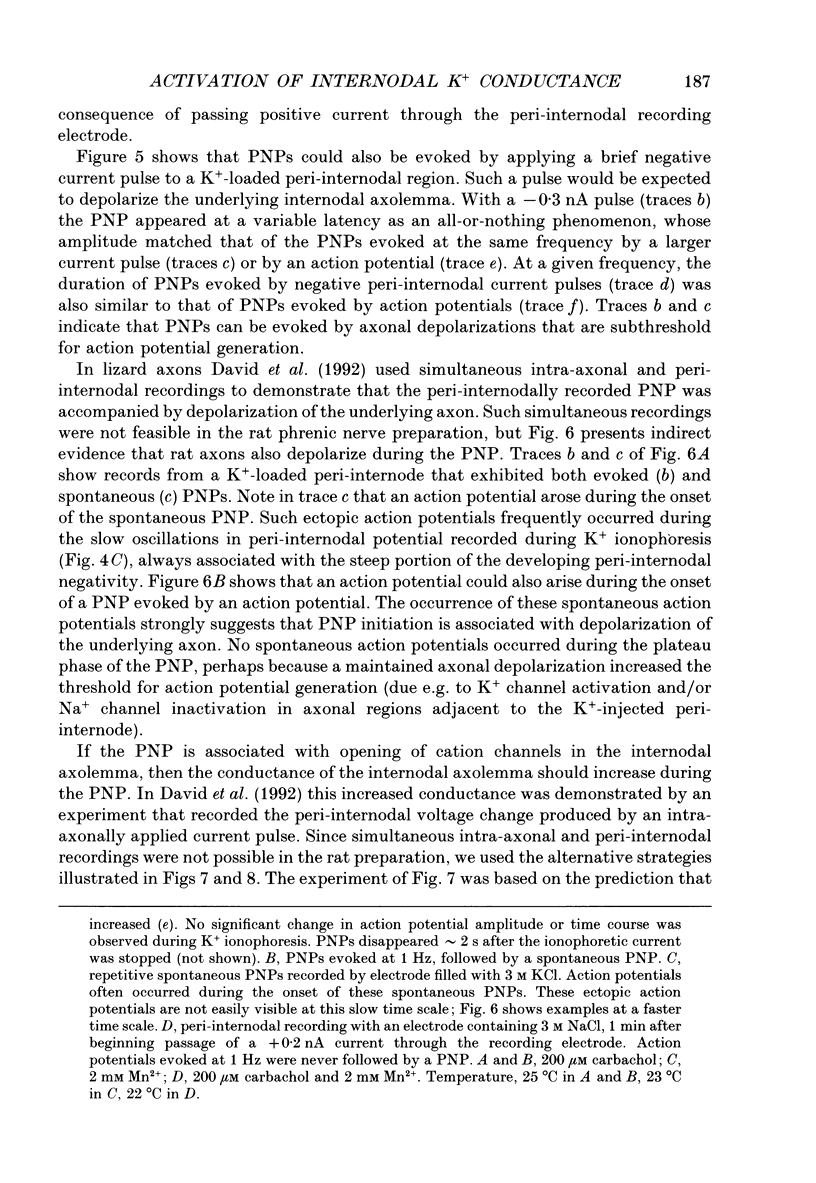
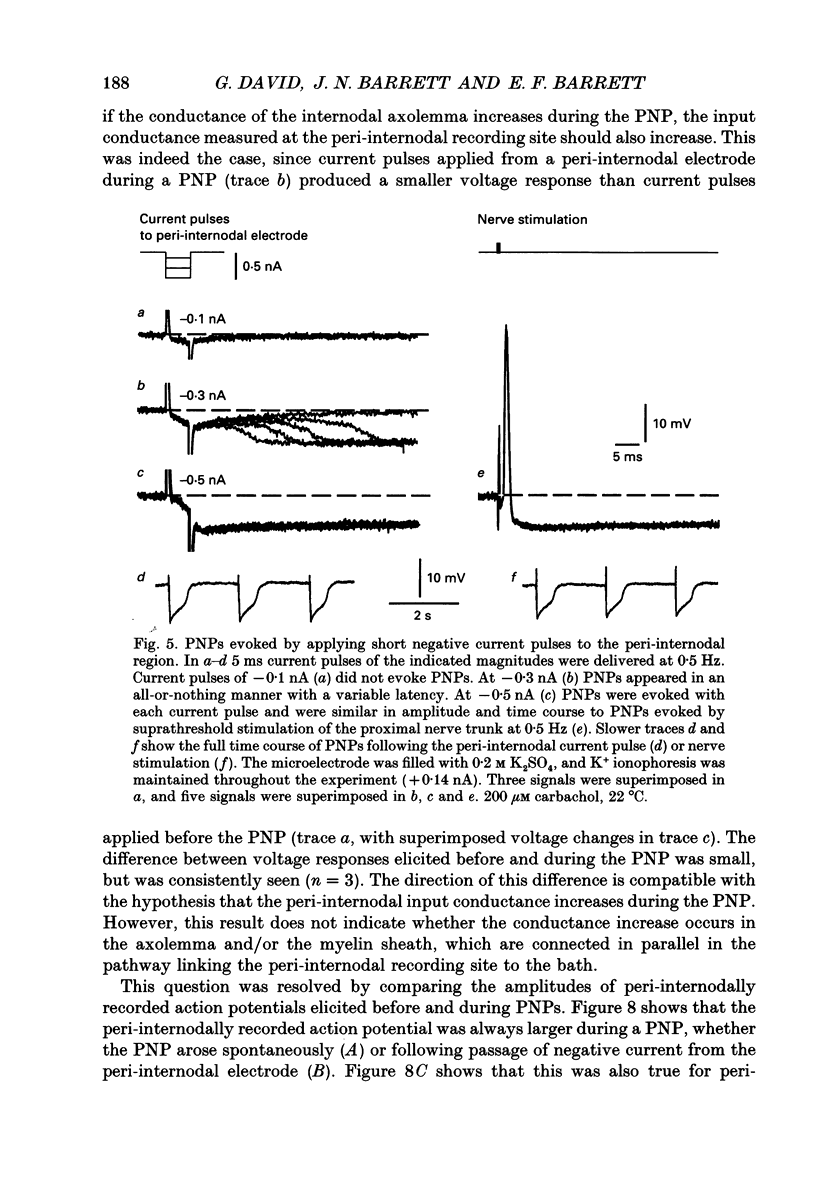
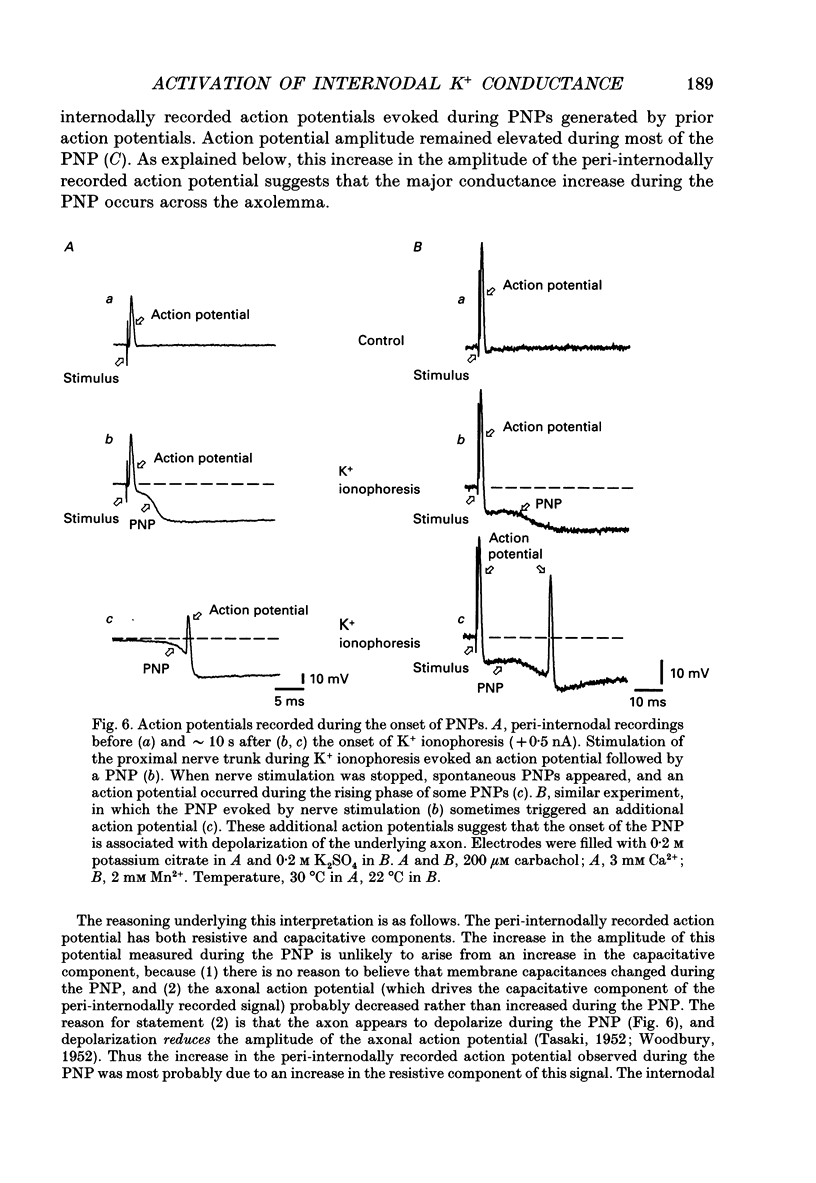
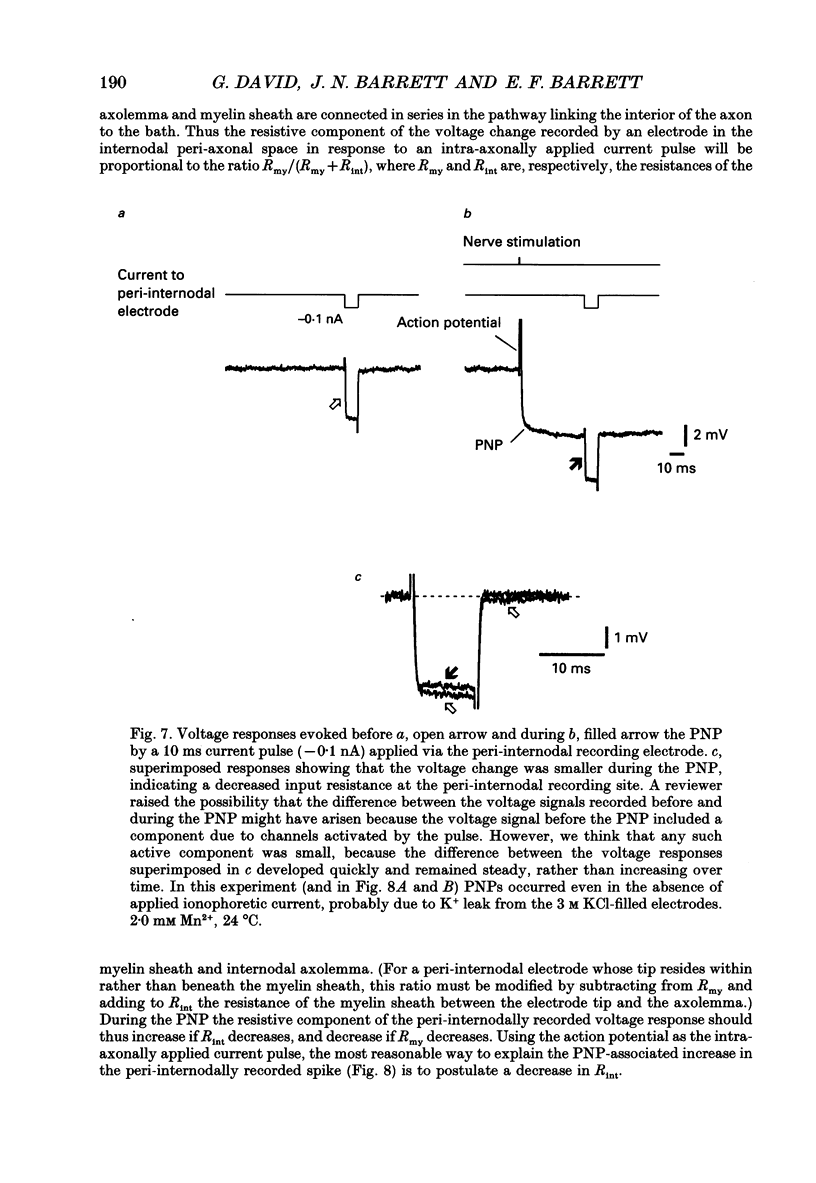
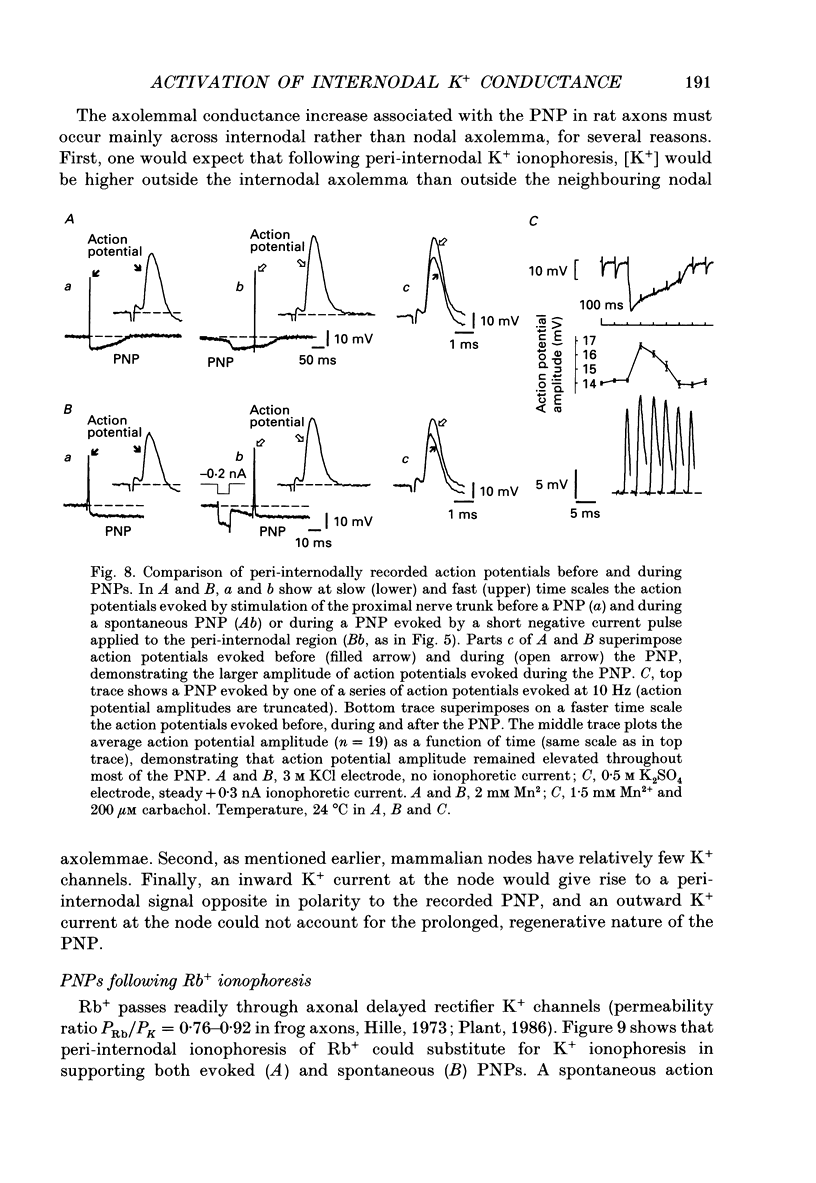
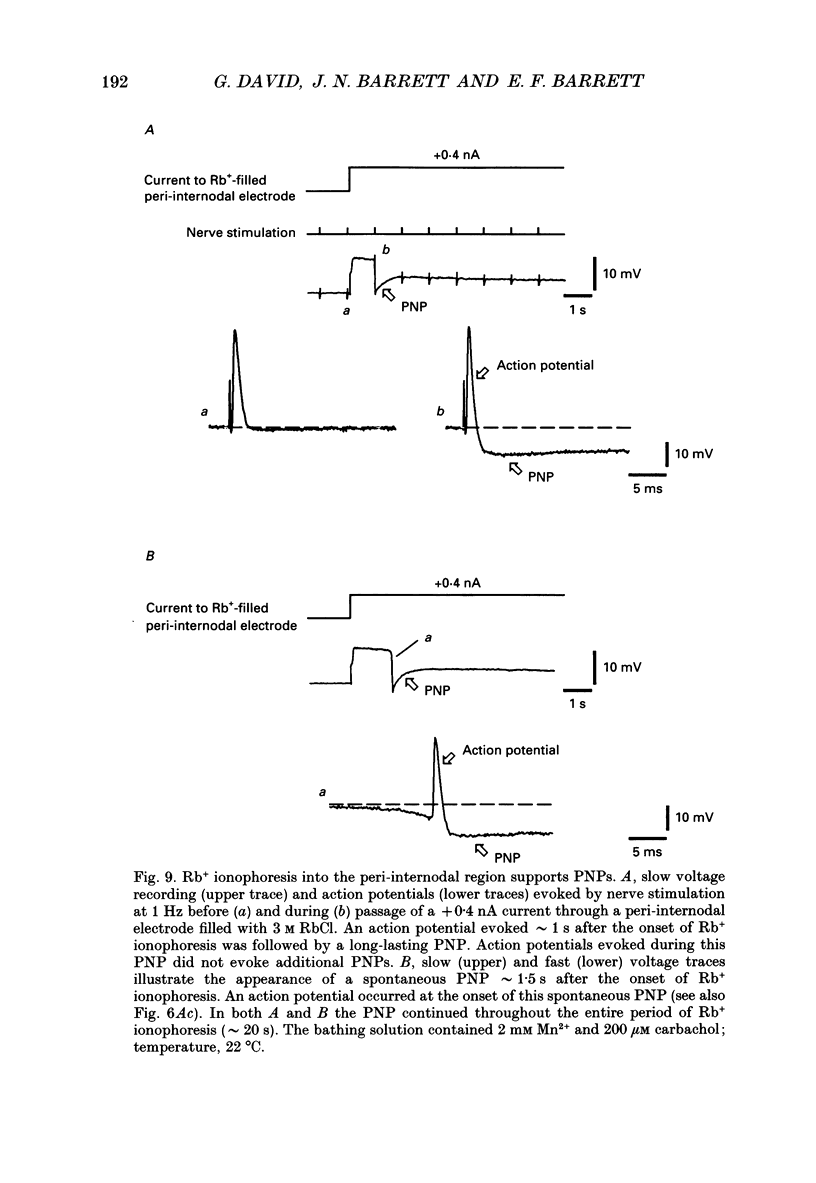
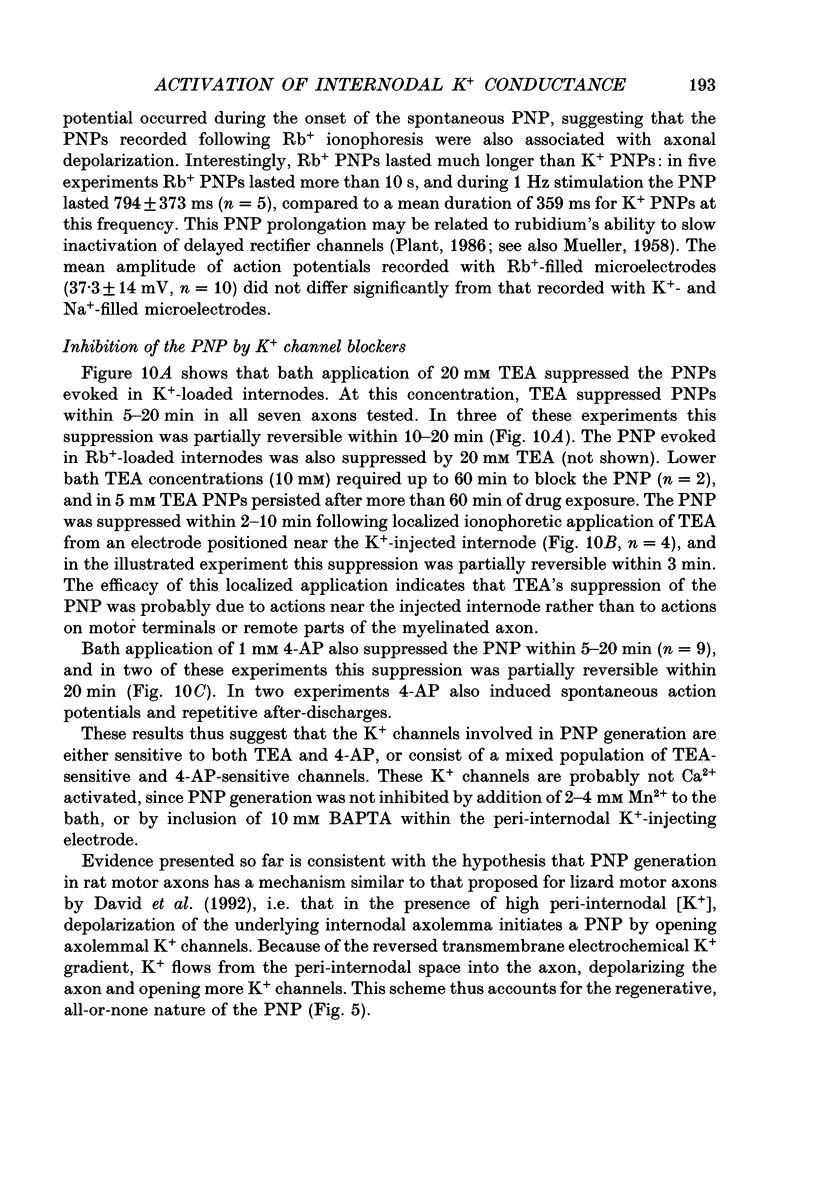
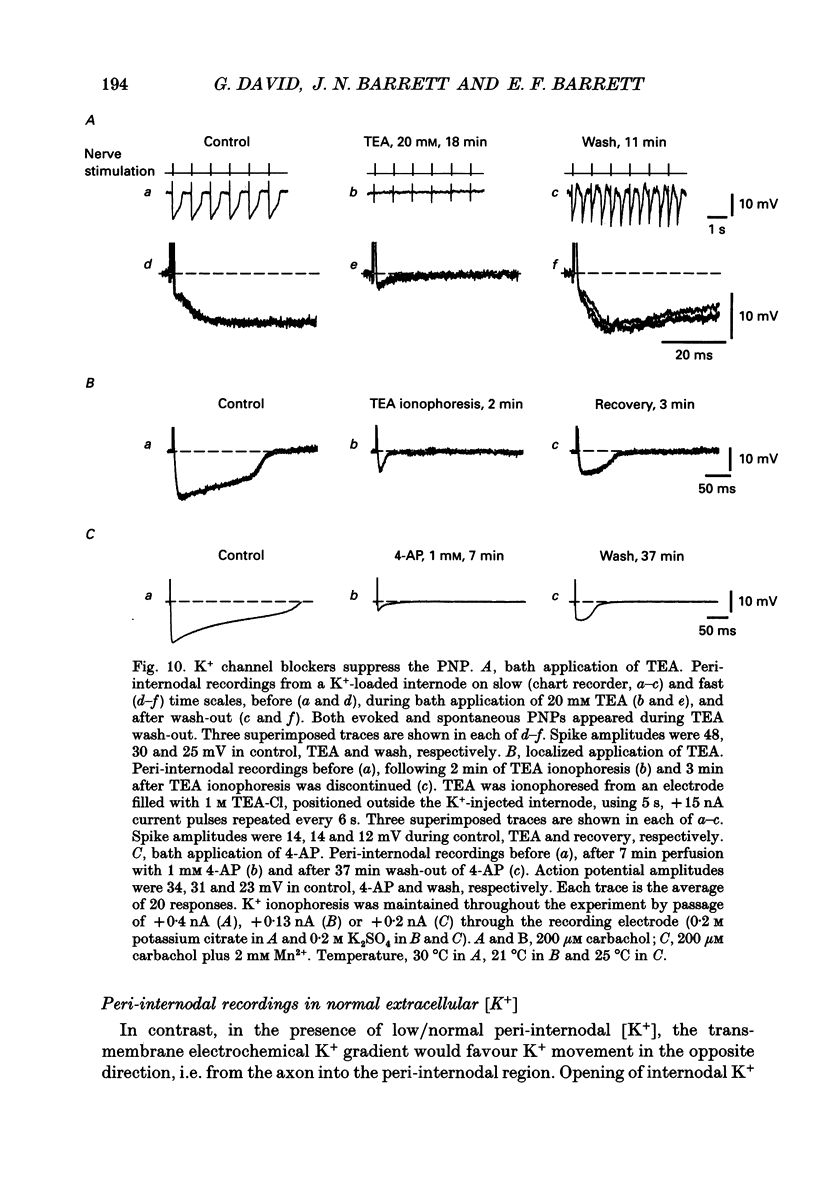
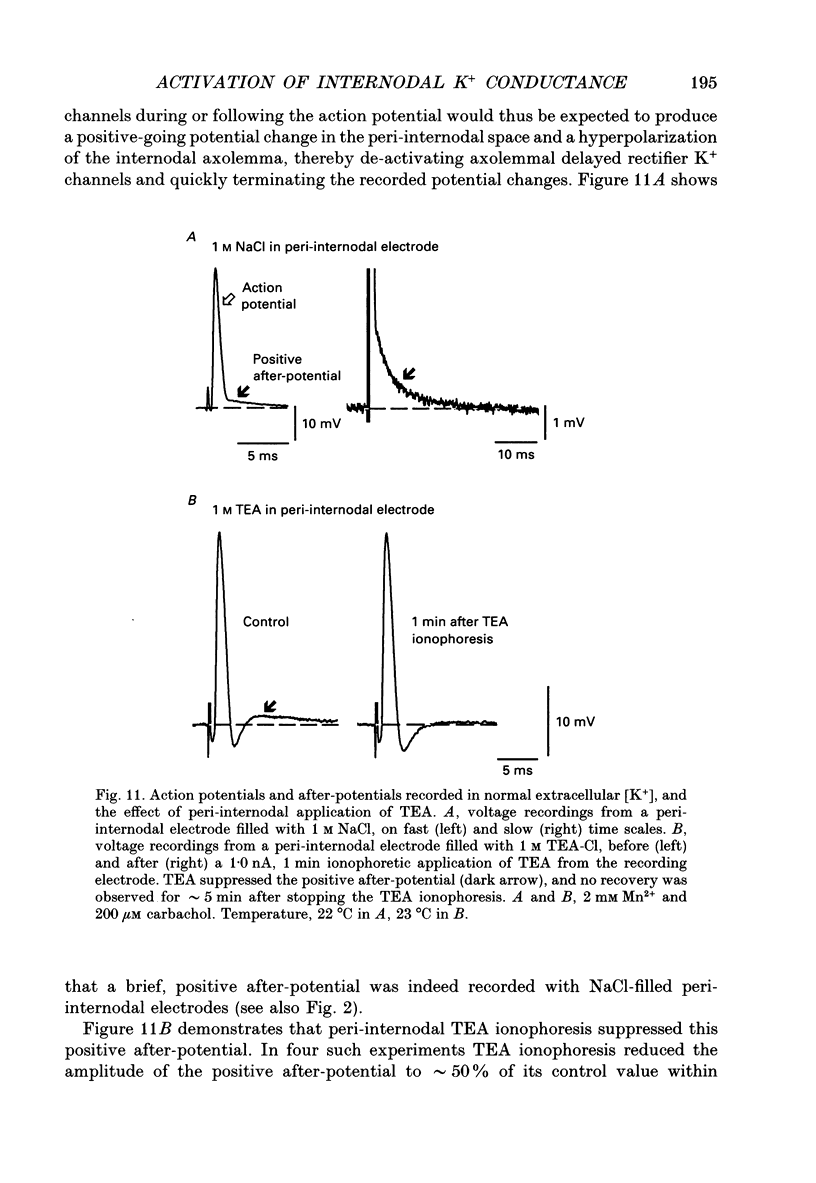
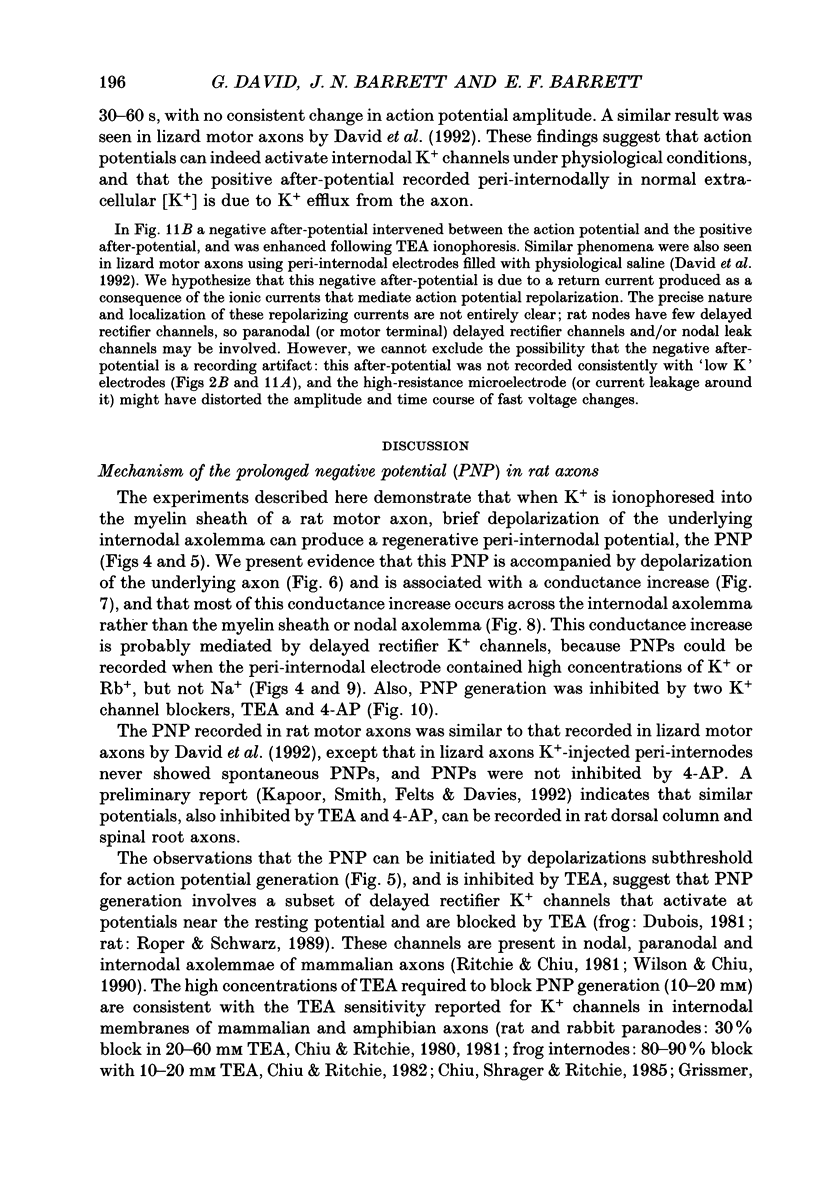
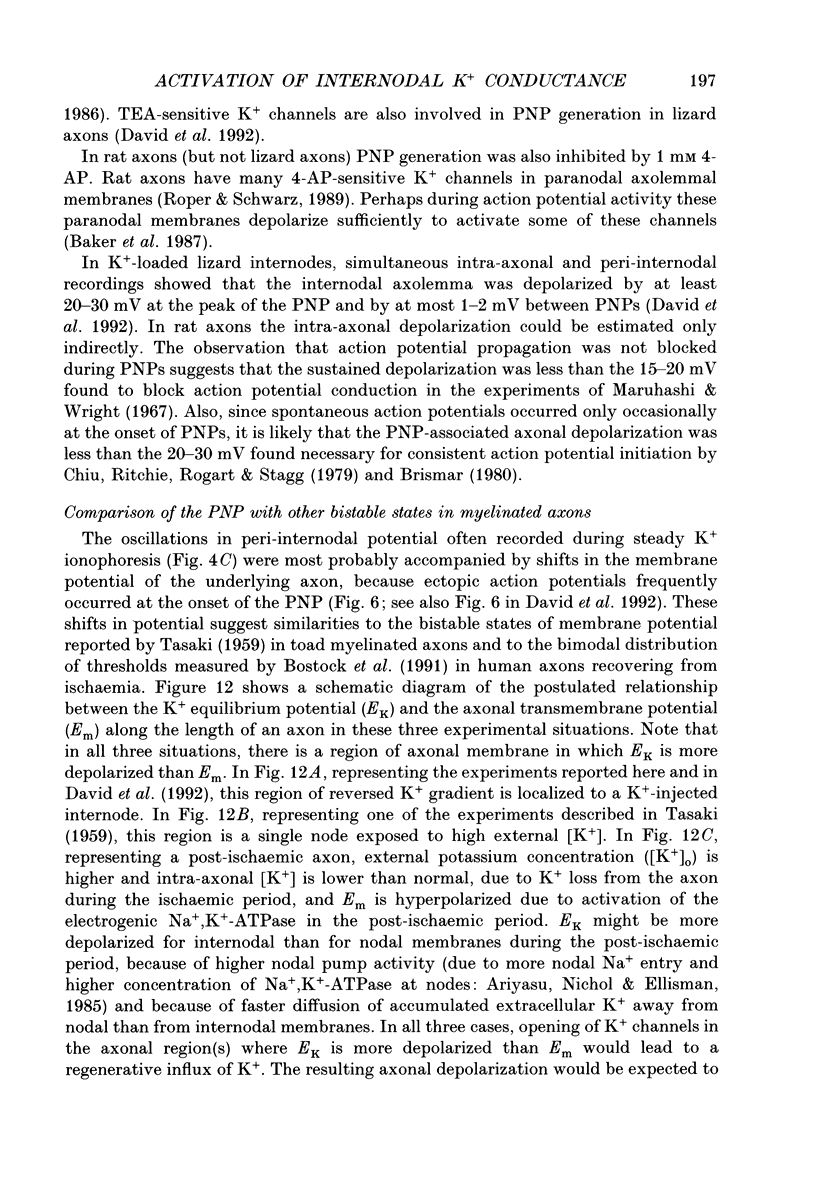
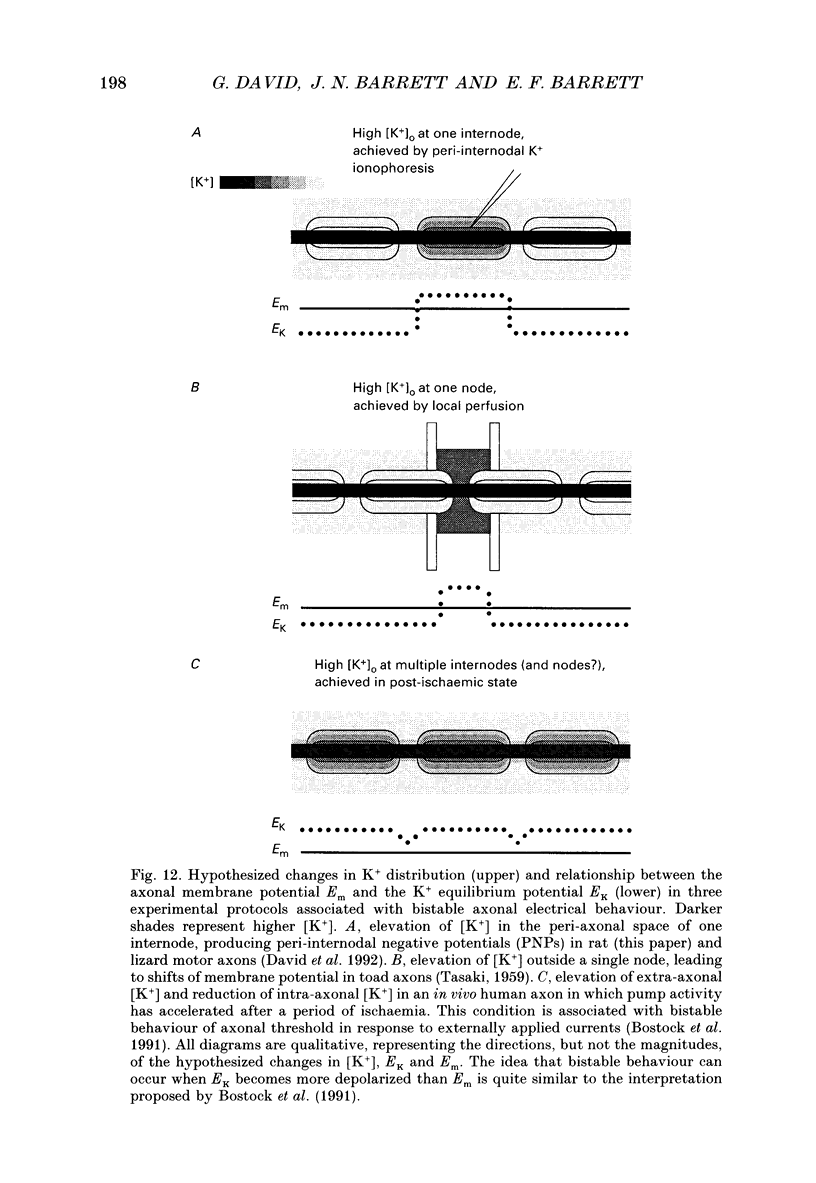
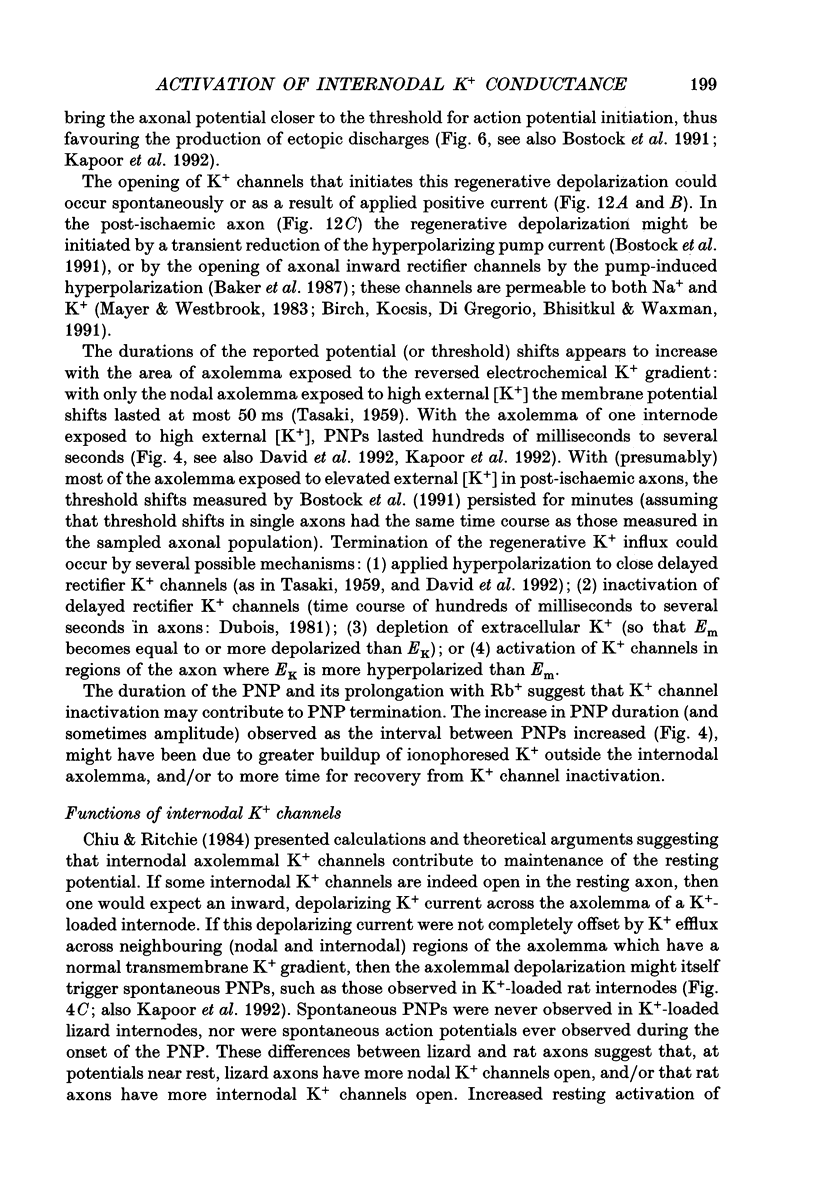
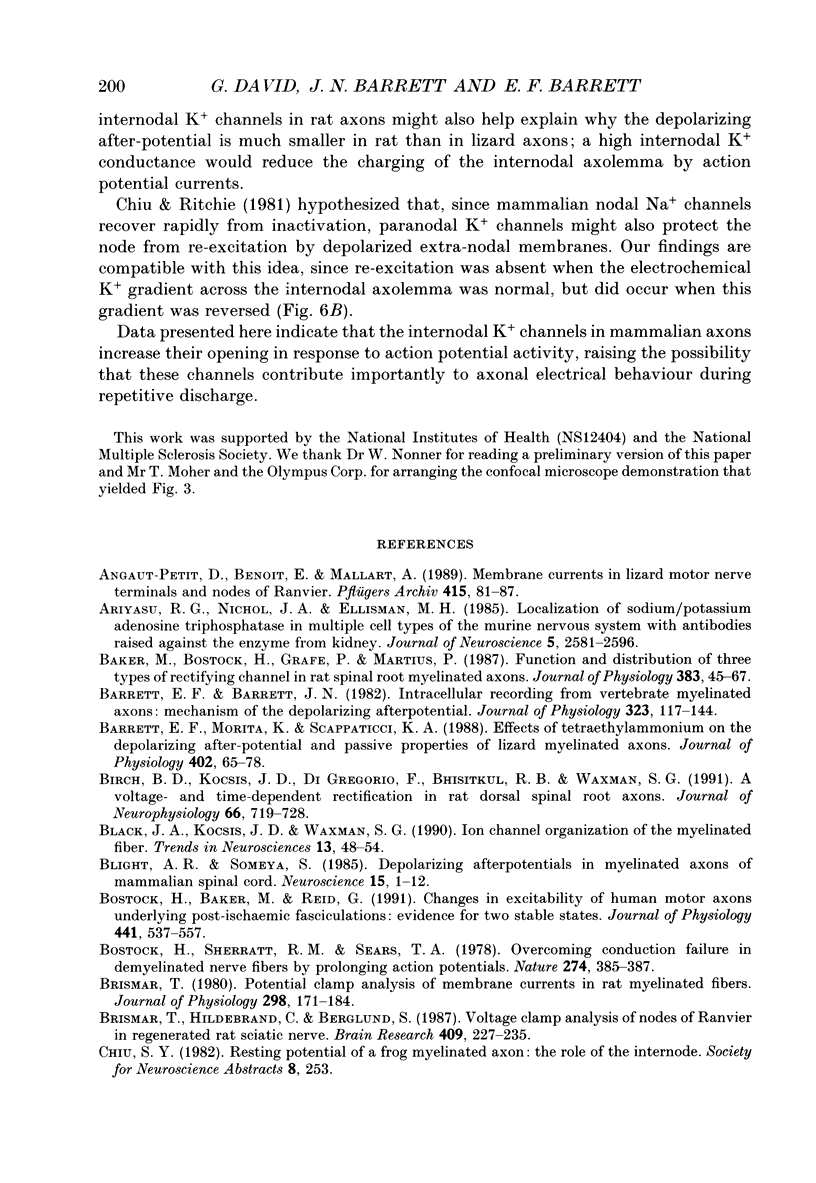
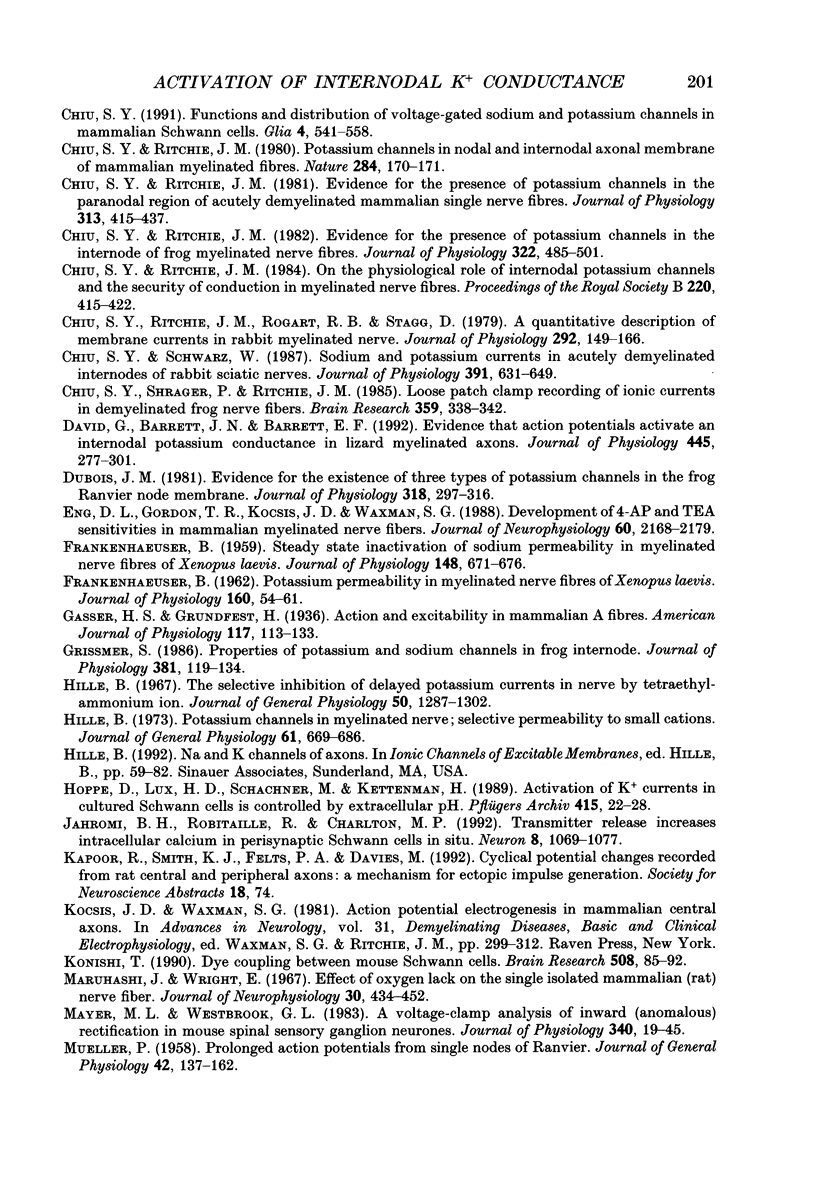
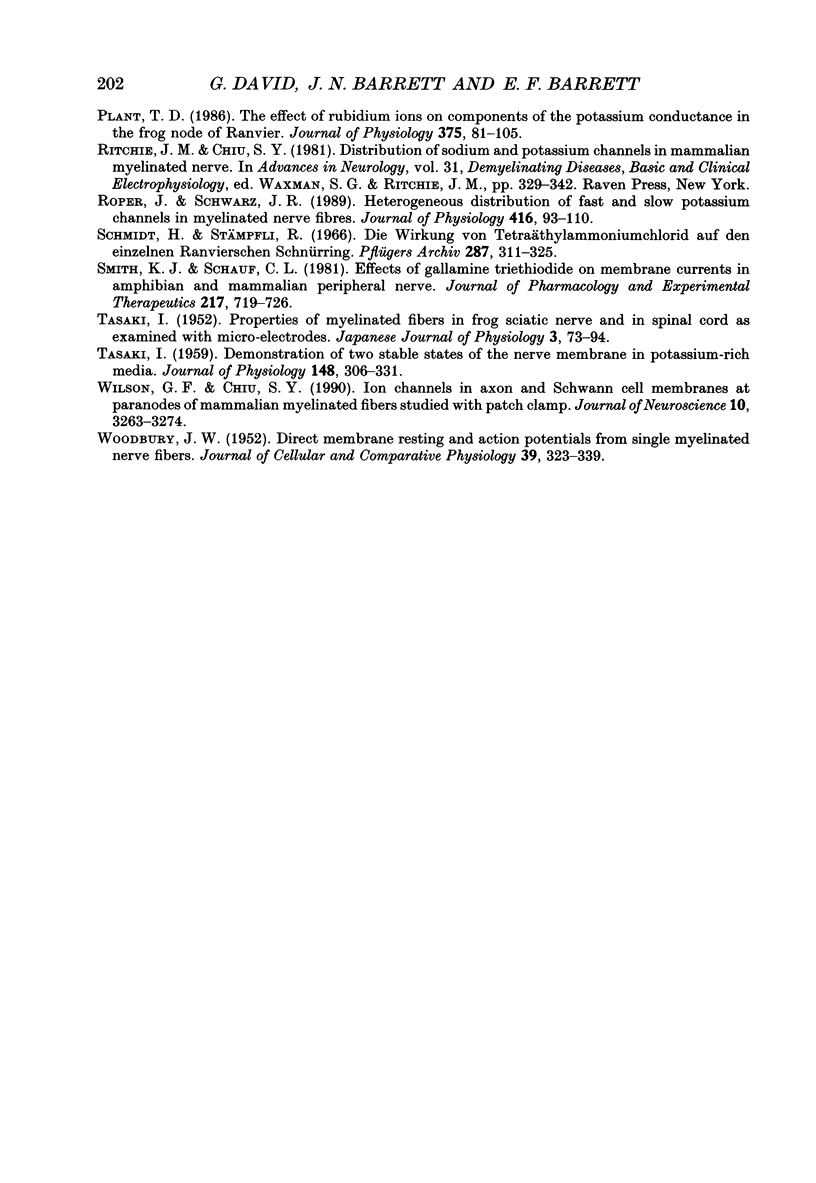
Images in this article
Selected References
These references are in PubMed. This may not be the complete list of references from this article.
- Angaut-Petit D., Benoit E., Mallart A. Membrane currents in lizard motor nerve terminals and nodes of Ranvier. Pflugers Arch. 1989 Oct;415(1):81–87. doi: 10.1007/BF00373144. [DOI] [PubMed] [Google Scholar]
- Ariyasu R. G., Nichol J. A., Ellisman M. H. Localization of sodium/potassium adenosine triphosphatase in multiple cell types of the murine nervous system with antibodies raised against the enzyme from kidney. J Neurosci. 1985 Oct;5(10):2581–2596. doi: 10.1523/JNEUROSCI.05-10-02581.1985. [DOI] [PMC free article] [PubMed] [Google Scholar]
- Baker M., Bostock H., Grafe P., Martius P. Function and distribution of three types of rectifying channel in rat spinal root myelinated axons. J Physiol. 1987 Feb;383:45–67. doi: 10.1113/jphysiol.1987.sp016395. [DOI] [PMC free article] [PubMed] [Google Scholar]
- Barrett E. F., Barrett J. N. Intracellular recording from vertebrate myelinated axons: mechanism of the depolarizing afterpotential. J Physiol. 1982 Feb;323:117–144. doi: 10.1113/jphysiol.1982.sp014064. [DOI] [PMC free article] [PubMed] [Google Scholar]
- Barrett E. F., Morita K., Scappaticci K. A. Effects of tetraethylammonium on the depolarizing after-potential and passive properties of lizard myelinated axons. J Physiol. 1988 Aug;402:65–78. doi: 10.1113/jphysiol.1988.sp017194. [DOI] [PMC free article] [PubMed] [Google Scholar]
- Birch B. D., Kocsis J. D., Di Gregorio F., Bhisitkul R. B., Waxman S. G. A voltage- and time-dependent rectification in rat dorsal spinal root axons. J Neurophysiol. 1991 Sep;66(3):719–728. doi: 10.1152/jn.1991.66.3.719. [DOI] [PubMed] [Google Scholar]
- Black J. A., Kocsis J. D., Waxman S. G. Ion channel organization of the myelinated fiber. Trends Neurosci. 1990 Feb;13(2):48–54. doi: 10.1016/0166-2236(90)90068-l. [DOI] [PubMed] [Google Scholar]
- Blight A. R., Someya S. Depolarizing afterpotentials in myelinated axons of mammalian spinal cord. Neuroscience. 1985 May;15(1):1–12. doi: 10.1016/0306-4522(85)90118-6. [DOI] [PubMed] [Google Scholar]
- Bostock H., Baker M., Reid G. Changes in excitability of human motor axons underlying post-ischaemic fasciculations: evidence for two stable states. J Physiol. 1991 Sep;441:537–557. doi: 10.1113/jphysiol.1991.sp018766. [DOI] [PMC free article] [PubMed] [Google Scholar]
- Bostock H., Sherratt R. M., Sears T. A. Overcoming conduction failure in demyelinated nerve fibres by prolonging action potentials. Nature. 1978 Jul 27;274(5669):385–387. doi: 10.1038/274385a0. [DOI] [PubMed] [Google Scholar]
- Brismar T., Hildebrand C., Berglund S. Voltage-clamp analysis of nodes of Ranvier in regenerated rat sciatic nerve. Brain Res. 1987 Apr 21;409(2):227–235. doi: 10.1016/0006-8993(87)90706-2. [DOI] [PubMed] [Google Scholar]
- Brismar T. Potential clamp analysis of membrane currents in rat myelinated nerve fibres. J Physiol. 1980 Jan;298:171–184. doi: 10.1113/jphysiol.1980.sp013074. [DOI] [PMC free article] [PubMed] [Google Scholar]
- Chiu S. Y. Functions and distribution of voltage-gated sodium and potassium channels in mammalian Schwann cells. Glia. 1991;4(6):541–558. doi: 10.1002/glia.440040602. [DOI] [PubMed] [Google Scholar]
- Chiu S. Y., Ritchie J. M. Evidence for the presence of potassium channels in the internode of frog myelinated nerve fibres. J Physiol. 1982 Jan;322:485–501. doi: 10.1113/jphysiol.1982.sp014051. [DOI] [PMC free article] [PubMed] [Google Scholar]
- Chiu S. Y., Ritchie J. M. Evidence for the presence of potassium channels in the paranodal region of acutely demyelinated mammalian single nerve fibres. J Physiol. 1981;313:415–437. doi: 10.1113/jphysiol.1981.sp013674. [DOI] [PMC free article] [PubMed] [Google Scholar]
- Chiu S. Y., Ritchie J. M. On the physiological role of internodal potassium channels and the security of conduction in myelinated nerve fibres. Proc R Soc Lond B Biol Sci. 1984 Feb 22;220(1221):415–422. doi: 10.1098/rspb.1984.0010. [DOI] [PubMed] [Google Scholar]
- Chiu S. Y., Ritchie J. M. Potassium channels in nodal and internodal axonal membrane of mammalian myelinated fibres. Nature. 1980 Mar 13;284(5752):170–171. doi: 10.1038/284170a0. [DOI] [PubMed] [Google Scholar]
- Chiu S. Y., Ritchie J. M., Rogart R. B., Stagg D. A quantitative description of membrane currents in rabbit myelinated nerve. J Physiol. 1979 Jul;292:149–166. doi: 10.1113/jphysiol.1979.sp012843. [DOI] [PMC free article] [PubMed] [Google Scholar]
- Chiu S. Y., Schwarz W. Sodium and potassium currents in acutely demyelinated internodes of rabbit sciatic nerves. J Physiol. 1987 Oct;391:631–649. doi: 10.1113/jphysiol.1987.sp016760. [DOI] [PMC free article] [PubMed] [Google Scholar]
- Chiu S. Y., Shrager P., Ritchie J. M. Loose patch clamp recording of ionic currents in demyelinated frog nerve fibers. Brain Res. 1985 Dec 16;359(1-2):338–342. doi: 10.1016/0006-8993(85)91446-5. [DOI] [PubMed] [Google Scholar]
- David G., Barrett J. N., Barrett E. F. Evidence that action potentials activate an internodal potassium conductance in lizard myelinated axons. J Physiol. 1992 Jan;445:277–301. doi: 10.1113/jphysiol.1992.sp018924. [DOI] [PMC free article] [PubMed] [Google Scholar]
- Dubois J. M. Evidence for the existence of three types of potassium channels in the frog Ranvier node membrane. J Physiol. 1981 Sep;318:297–316. doi: 10.1113/jphysiol.1981.sp013865. [DOI] [PMC free article] [PubMed] [Google Scholar]
- Eng D. L., Gordon T. R., Kocsis J. D., Waxman S. G. Development of 4-AP and TEA sensitivities in mammalian myelinated nerve fibers. J Neurophysiol. 1988 Dec;60(6):2168–2179. doi: 10.1152/jn.1988.60.6.2168. [DOI] [PubMed] [Google Scholar]
- FRANKENHAEUSER B. Potassium permeability in myelinated nerve fibres of Xenopus laevis. J Physiol. 1962 Jan;160:54–61. doi: 10.1113/jphysiol.1962.sp006834. [DOI] [PMC free article] [PubMed] [Google Scholar]
- FRANKENHAEUSER B. Steady state inactivation of sodium permeability in myelinated nerve fibres of Xenopus laevis. J Physiol. 1959 Oct;148:671–676. doi: 10.1113/jphysiol.1959.sp006316. [DOI] [PMC free article] [PubMed] [Google Scholar]
- Grissmer S. Properties of potassium and sodium channels in frog internode. J Physiol. 1986 Dec;381:119–134. doi: 10.1113/jphysiol.1986.sp016317. [DOI] [PMC free article] [PubMed] [Google Scholar]
- Hille B. Potassium channels in myelinated nerve. Selective permeability to small cations. J Gen Physiol. 1973 Jun;61(6):669–686. doi: 10.1085/jgp.61.6.669. [DOI] [PMC free article] [PubMed] [Google Scholar]
- Hille B. The selective inhibition of delayed potassium currents in nerve by tetraethylammonium ion. J Gen Physiol. 1967 May;50(5):1287–1302. doi: 10.1085/jgp.50.5.1287. [DOI] [PMC free article] [PubMed] [Google Scholar]
- Hoppe D., Lux H. D., Schachner M., Kettenmann H. Activation of K+ currents in cultured Schwann cells is controlled by extracellular pH. Pflugers Arch. 1989 Oct;415(1):22–28. doi: 10.1007/BF00373137. [DOI] [PubMed] [Google Scholar]
- Jahromi B. S., Robitaille R., Charlton M. P. Transmitter release increases intracellular calcium in perisynaptic Schwann cells in situ. Neuron. 1992 Jun;8(6):1069–1077. doi: 10.1016/0896-6273(92)90128-z. [DOI] [PubMed] [Google Scholar]
- Kocsis J. D., Waxman S. G. Action potential electrogenesis in mammalian central axons. Adv Neurol. 1981;31:299–312. [PubMed] [Google Scholar]
- Konishi T. Dye coupling between mouse Schwann cells. Brain Res. 1990 Jan 29;508(1):85–92. doi: 10.1016/0006-8993(90)91121-v. [DOI] [PubMed] [Google Scholar]
- MUELLER P. Prolonged action potentials from single nodes of Ranvier. J Gen Physiol. 1958 Sep 20;42(1):137–162. doi: 10.1085/jgp.42.1.137. [DOI] [PMC free article] [PubMed] [Google Scholar]
- Maruhashi J., Wright E. B. Effect of oxygen lack on the single isolated mammalian (rat) nerve fiber. J Neurophysiol. 1967 May;30(3):434–452. doi: 10.1152/jn.1967.30.3.434. [DOI] [PubMed] [Google Scholar]
- Mayer M. L., Westbrook G. L. A voltage-clamp analysis of inward (anomalous) rectification in mouse spinal sensory ganglion neurones. J Physiol. 1983 Jul;340:19–45. doi: 10.1113/jphysiol.1983.sp014747. [DOI] [PMC free article] [PubMed] [Google Scholar]
- Plant T. D. The effects of rubidium ions on components of the potassium conductance in the frog node of Ranvier. J Physiol. 1986 Jun;375:81–105. doi: 10.1113/jphysiol.1986.sp016107. [DOI] [PMC free article] [PubMed] [Google Scholar]
- Ritchie J. M., Chiu S. Y. Distribution of sodium and potassium channels in mammalian myelinated nerve. Adv Neurol. 1981;31:329–342. [PubMed] [Google Scholar]
- Röper J., Schwarz J. R. Heterogeneous distribution of fast and slow potassium channels in myelinated rat nerve fibres. J Physiol. 1989 Sep;416:93–110. doi: 10.1113/jphysiol.1989.sp017751. [DOI] [PMC free article] [PubMed] [Google Scholar]
- Schmidt H., Stämpfli R. Die Wirkung von Tetraäthylammoniumchlorid auf den einzelnen Ranvierschen Schnürring. Pflugers Arch Gesamte Physiol Menschen Tiere. 1966;287(4):311–325. [PubMed] [Google Scholar]
- Smith K. J., Schauf C. L. Effects of gallamine triethiodide on membrane currents in amphibian and mammalian peripheral nerve. J Pharmacol Exp Ther. 1981 Jun;217(3):719–726. [PubMed] [Google Scholar]
- TASAKI I. Demonstration of two stable states of the nerve membrane in potassium-rich media. J Physiol. 1959 Oct;148:306–331. doi: 10.1113/jphysiol.1959.sp006290. [DOI] [PMC free article] [PubMed] [Google Scholar]
- TASAKI I. Properties of myelinated fibers in frog sciatic nerve and in spinal cord as examined with micro-electrodes. Jpn J Physiol. 1952 Nov;3(1):73–94. doi: 10.2170/jjphysiol.3.73. [DOI] [PubMed] [Google Scholar]
- WOODBURY J. W. Direct membrane resting and action potentials from single myelinated nerve fibers. J Cell Physiol. 1952 Apr;39(2):323–339. doi: 10.1002/jcp.1030390210. [DOI] [PubMed] [Google Scholar]
- Wilson G. F., Chiu S. Y. Ion channels in axon and Schwann cell membranes at paranodes of mammalian myelinated fibers studied with patch clamp. J Neurosci. 1990 Oct;10(10):3263–3274. doi: 10.1523/JNEUROSCI.10-10-03263.1990. [DOI] [PMC free article] [PubMed] [Google Scholar]