Abstract
1. Cells from a pluripotent murine embryonal carcinoma cell line (P19) were differentiated in vitro into cells with neurone- and cardiac-like phenotypes. Cells treated with 0.5 microM retinoic acid developed into neurone-like cells possessing extensive neurites. Dimethyl sulphoxide treatment (0.5%) produced large, spontaneously contracting cell aggregates with many properties of cardiac cells. 2. The neurone- and cardiac-like cells contained voltage-sensitive Na+ channels with properties similar to those of native neuronal and cardiac cells. 3. We used whole-cell patch clamp techniques to measure inward currents from the neurone- and cardiac-like cells. Undifferentiated (untreated) cells had only small inward currents (peak of -0.15 nA in 150 mM external Na+). The peak inward current in the neurone-like and cardiac-like cells was -1.2 nA (in 154 mM external Na+) and -2.8 nA (in only 46 mM Na+), respectively. These large currents were absent when the external solution contained no Na+. 4. Tetrodotoxin (TTX) blocked the Na+ currents in the neurone- and cardiac-like cells in a dose-dependent manner. The Kd for TTX block of the Na+ current in the neurone-like cells was 6.7 nM. The Na+ current in the cardiac-like cells was much more resistant to TTX; the half-blocking concentration was two orders of magnitude higher, 710 nM. 5. The kinetic properties of the Na+ channel currents in the neurone- and cardiac-like cells were similar but developed over somewhat different voltage ranges. The voltage sensitivity of activation was similar in both cell types but the activation mid-point voltage was different: -12 mV in the neuronal cells and -34 mV for cardiac cells. Inactivation of the neuronal Na+ channels had a mid-point near -47 mV and was more sensitive to the membrane voltage than inactivation of the cardiac channels. The mid-point of inactivation for the cardiac Na+ channels was -80 mV.
Full text
PDF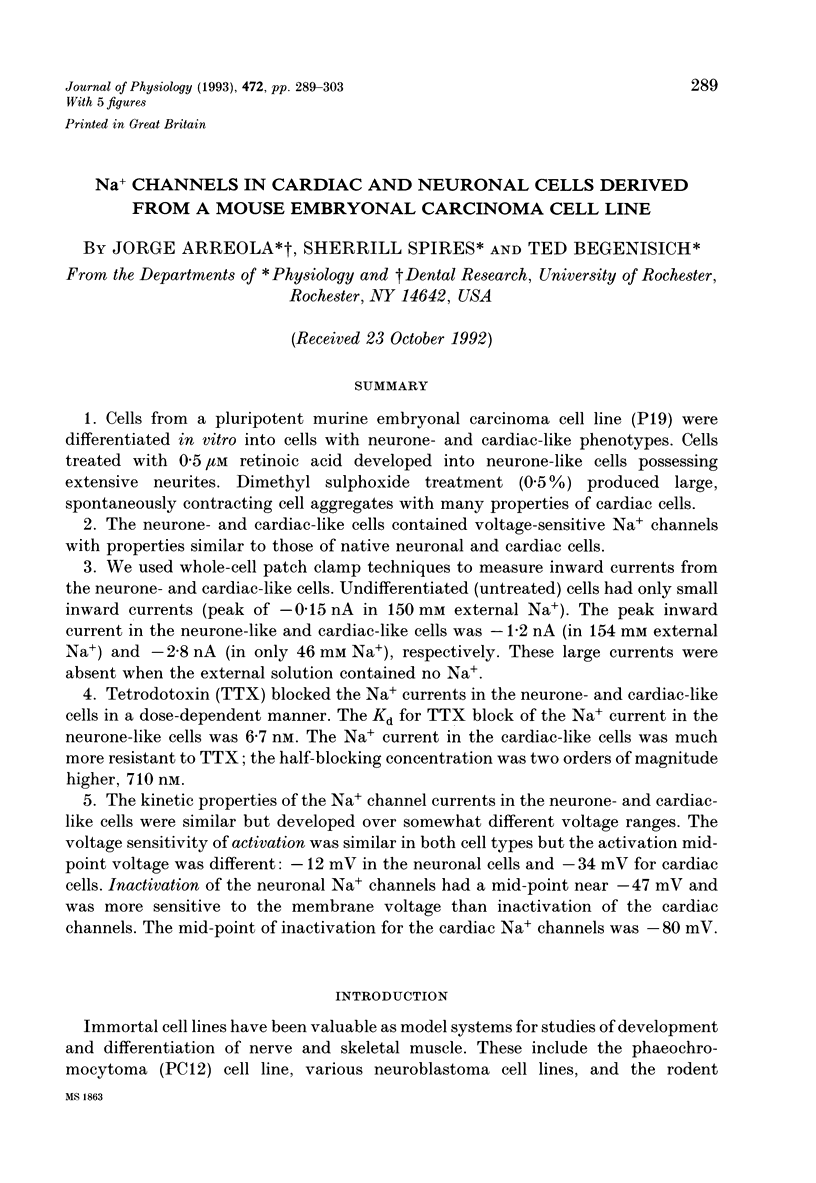
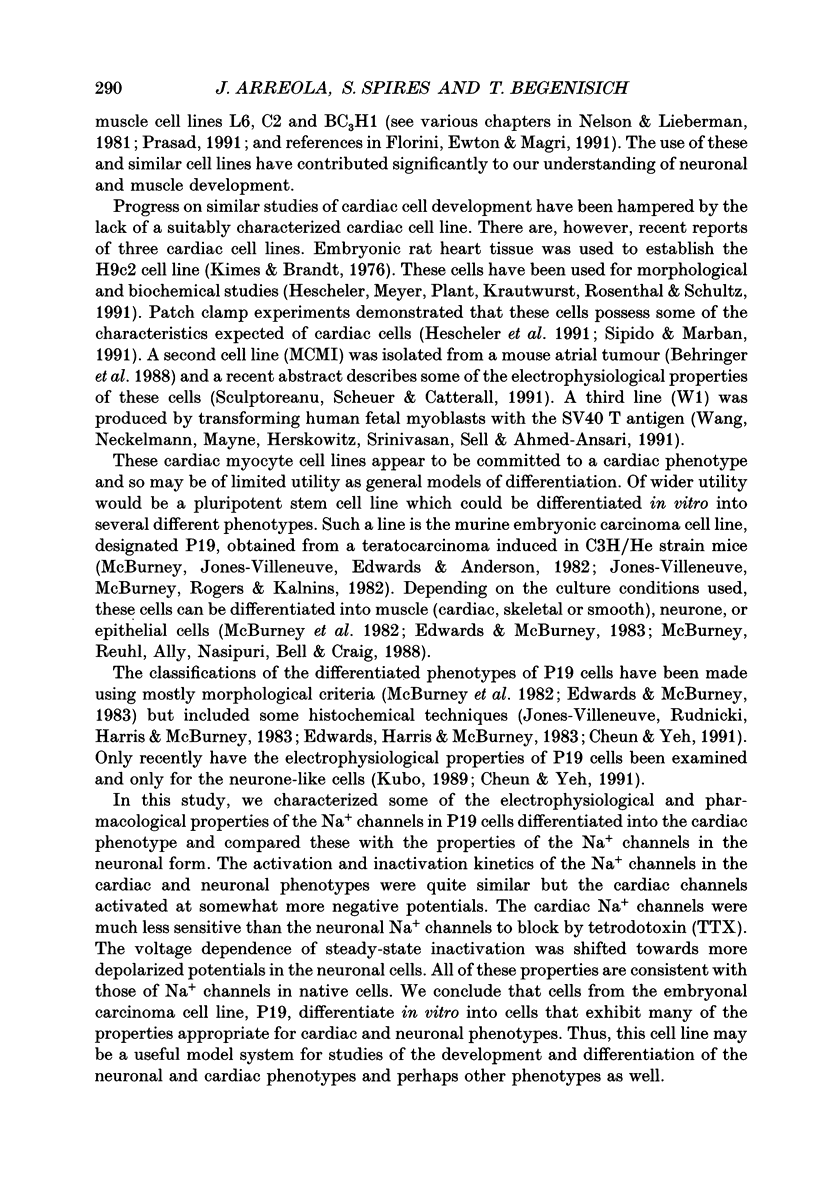
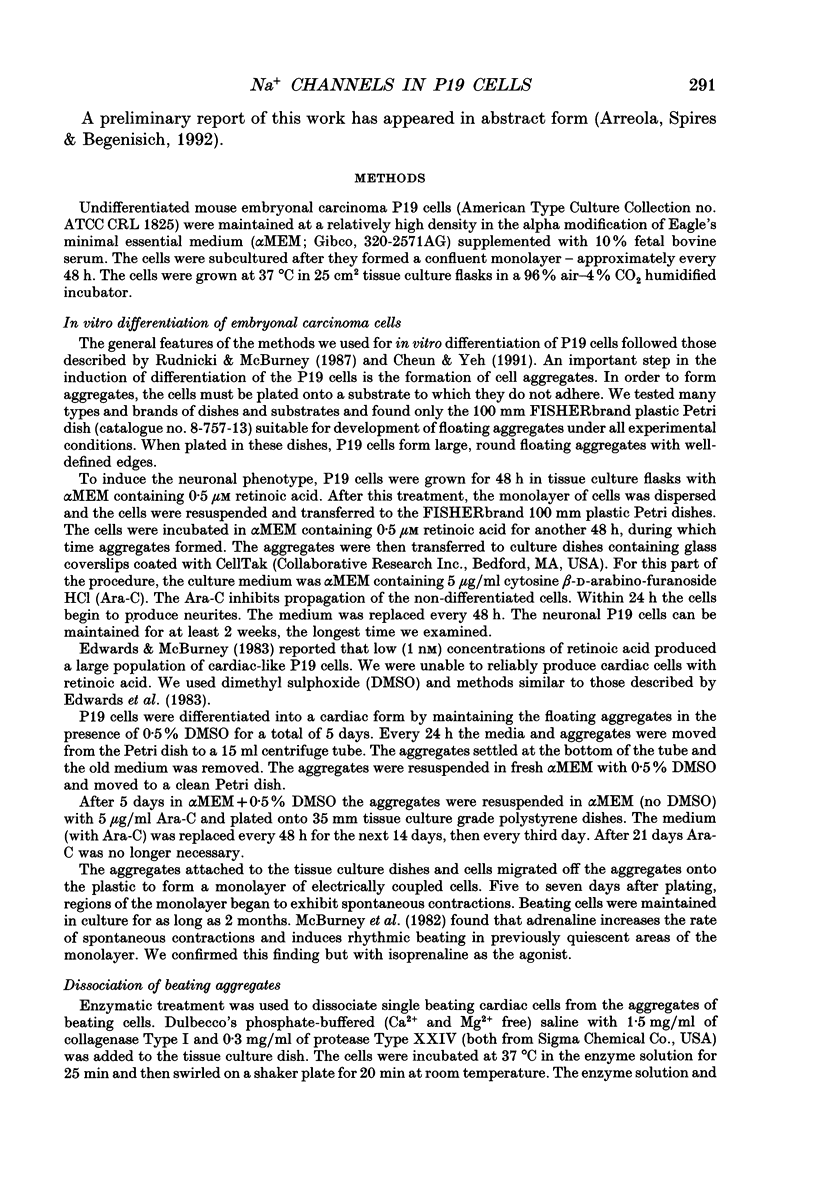
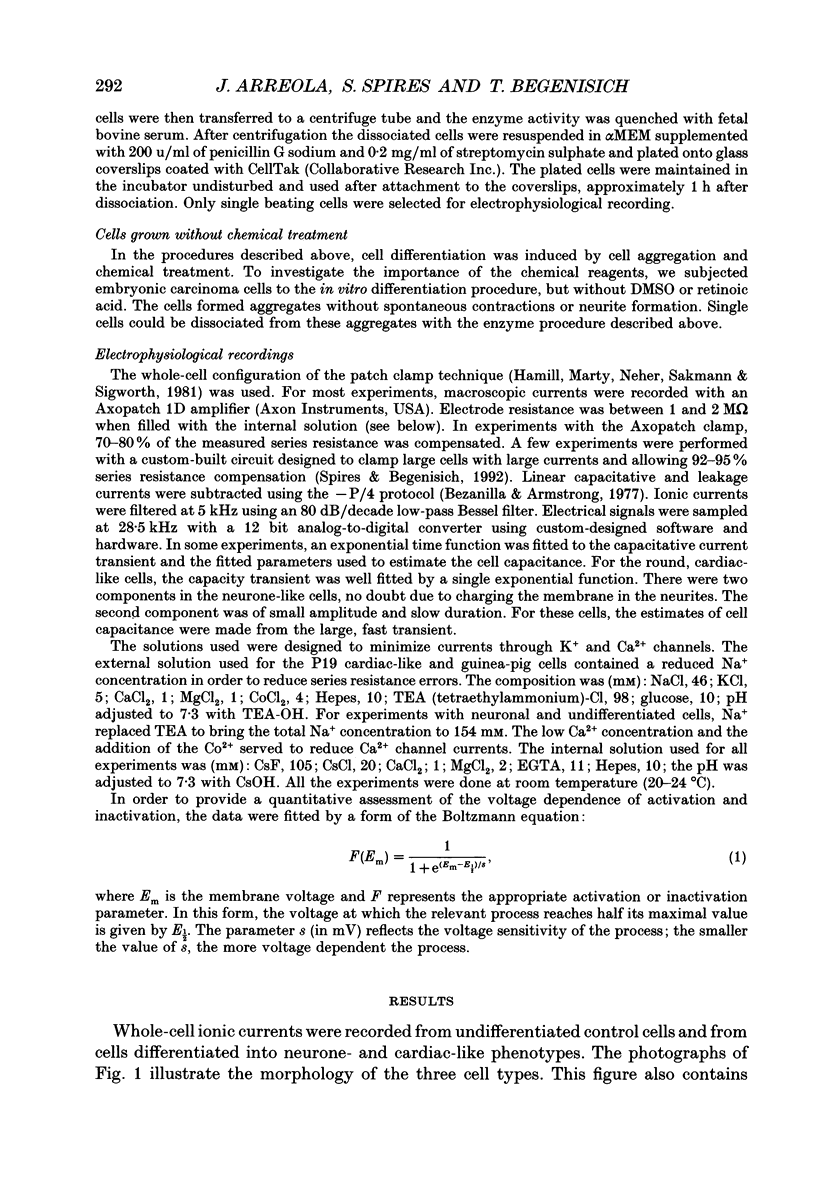
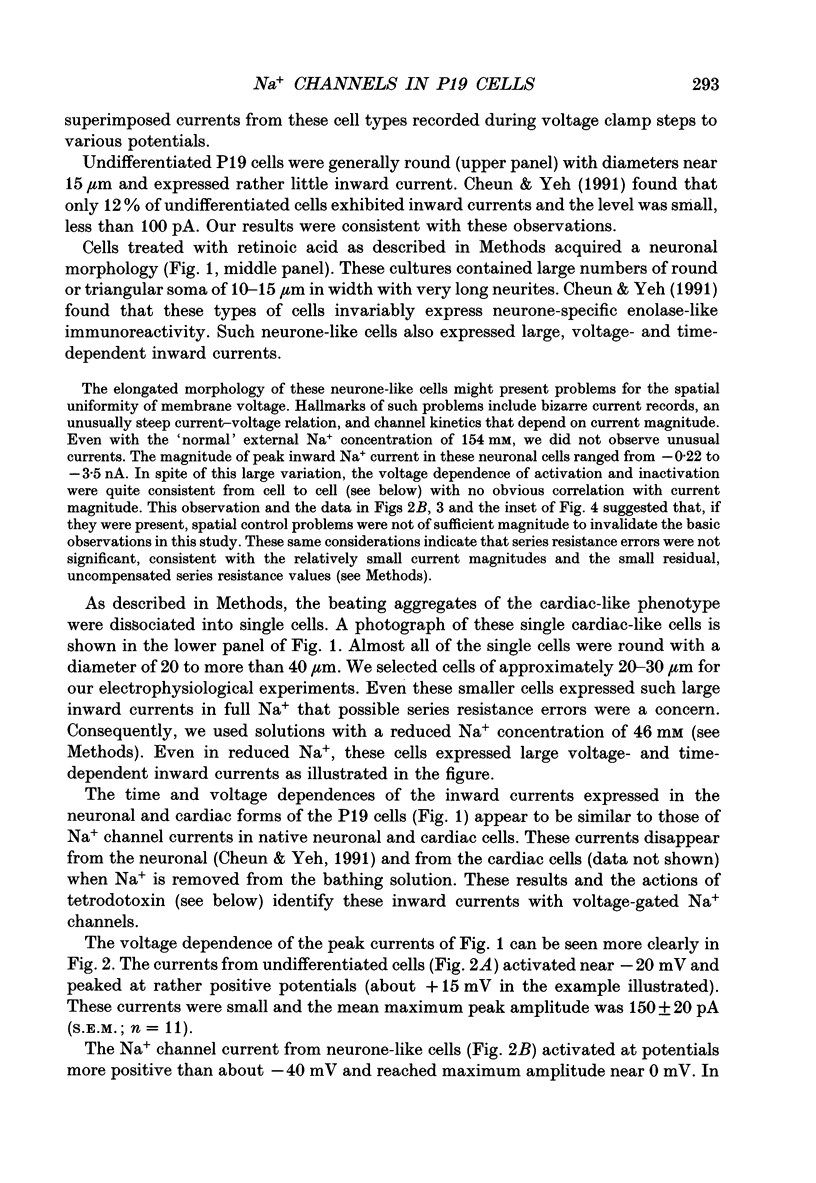
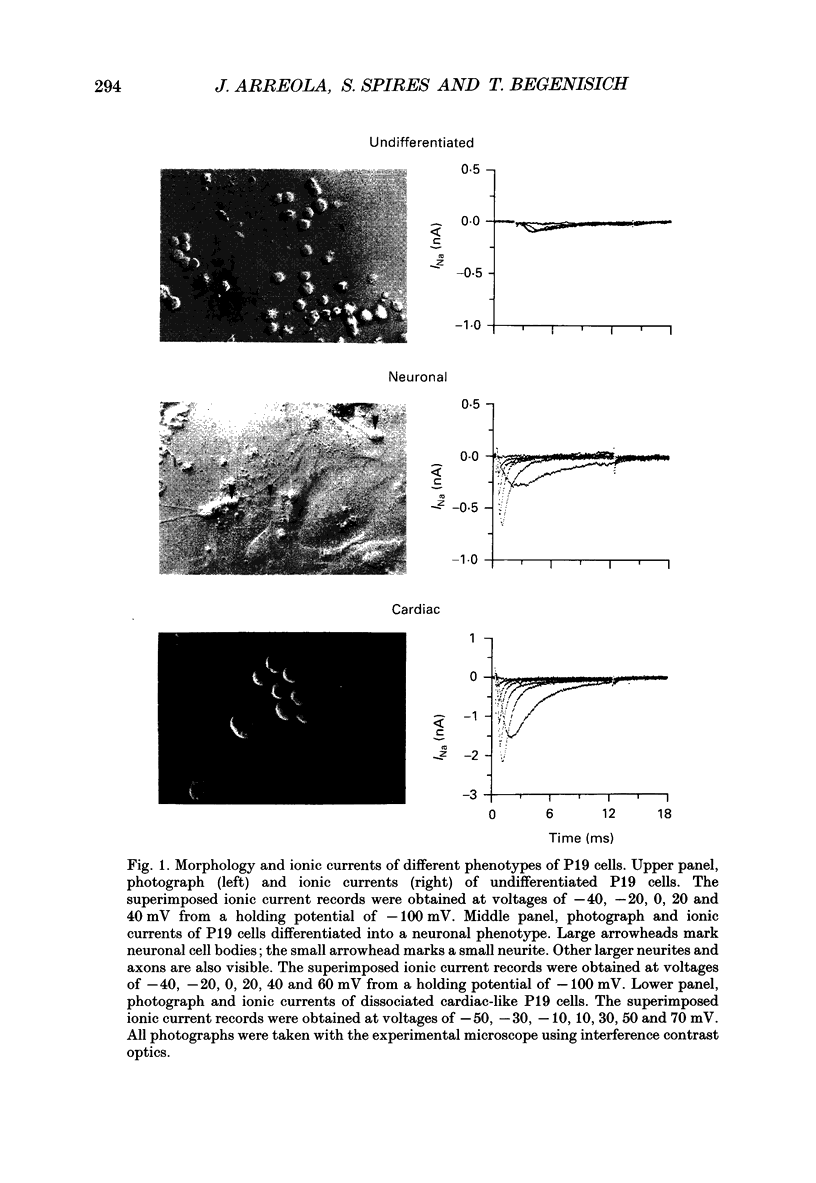
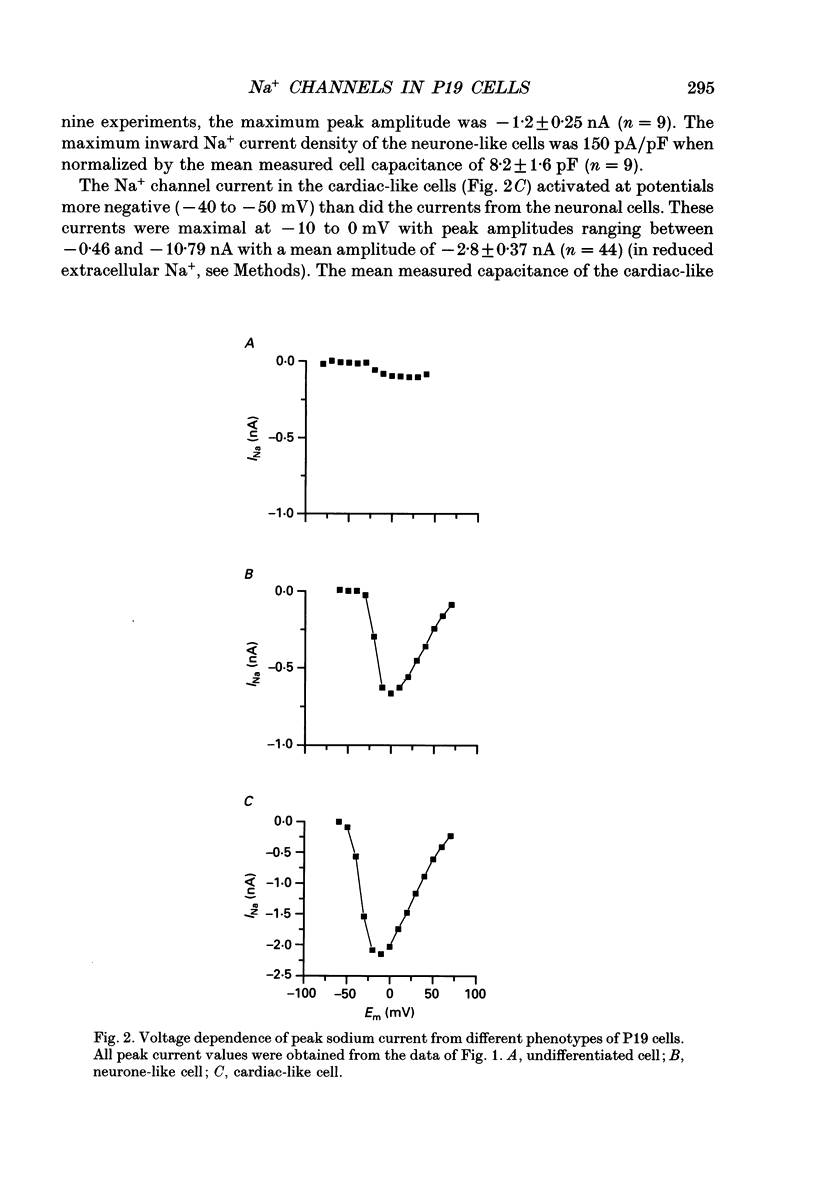
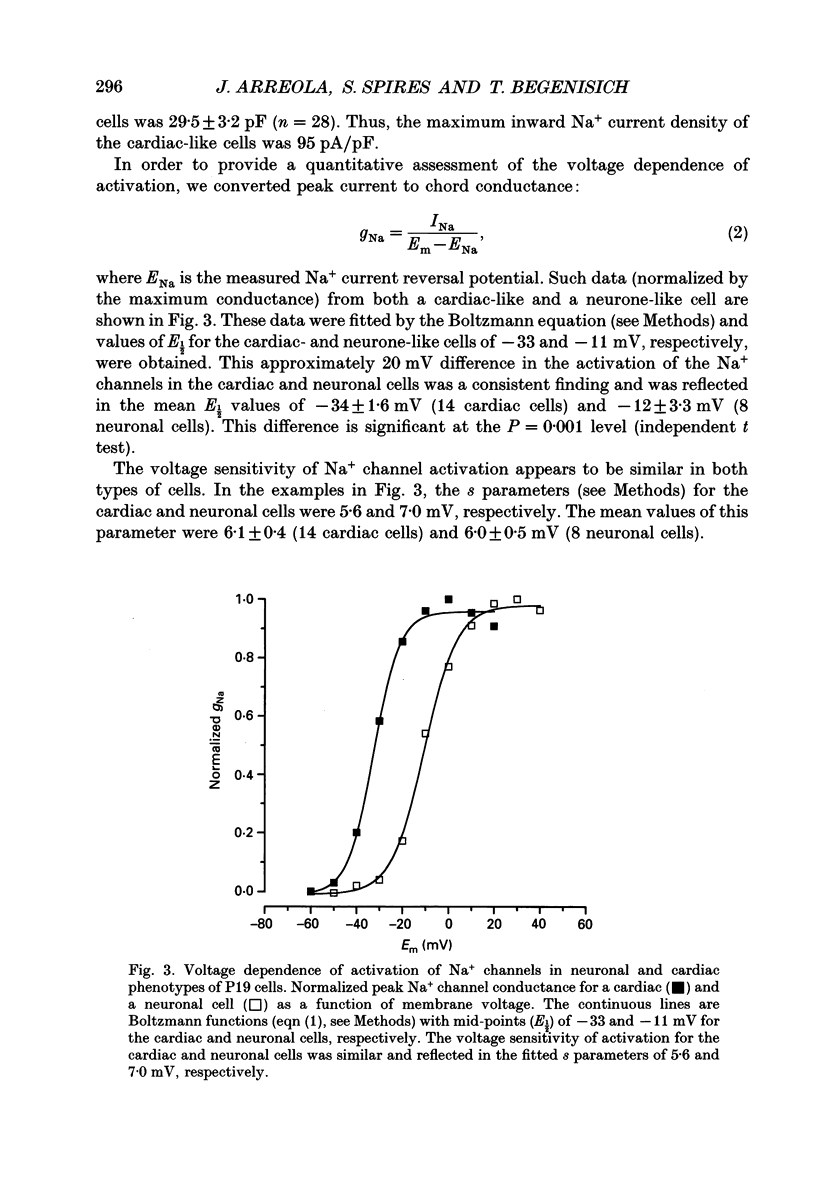
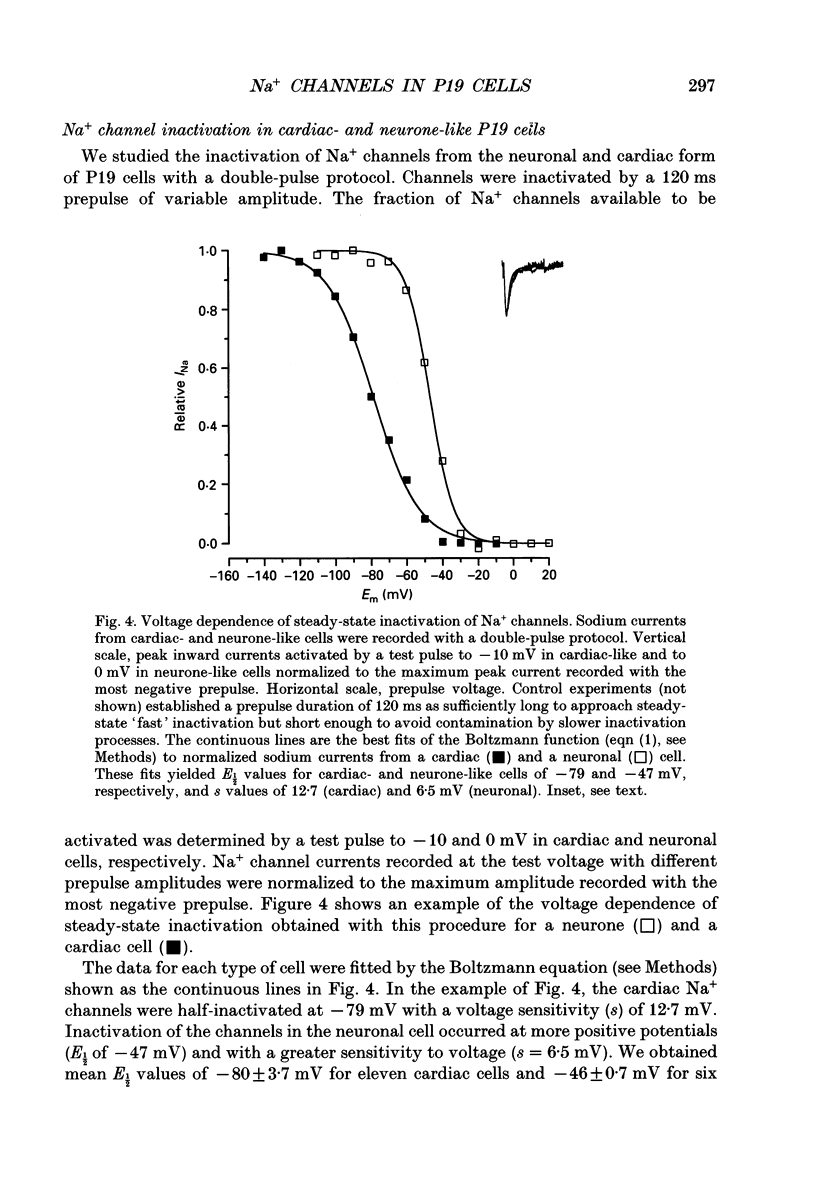
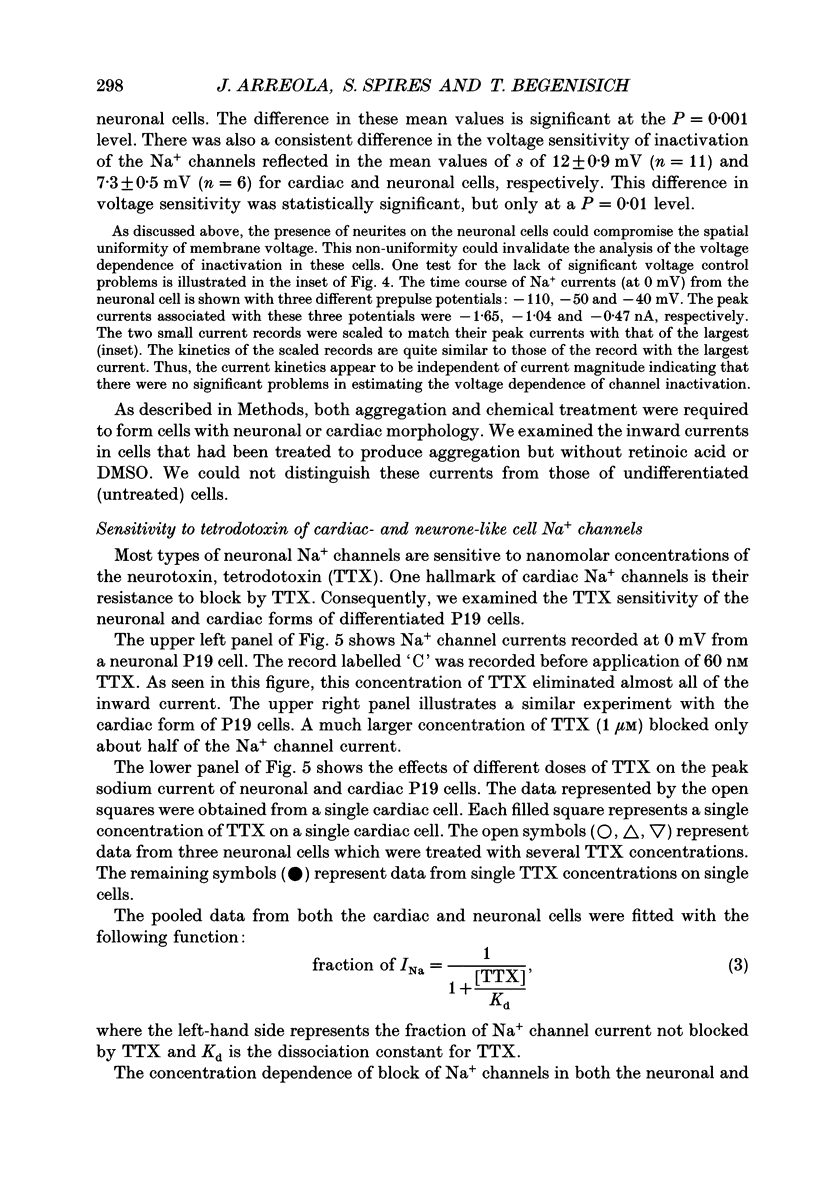
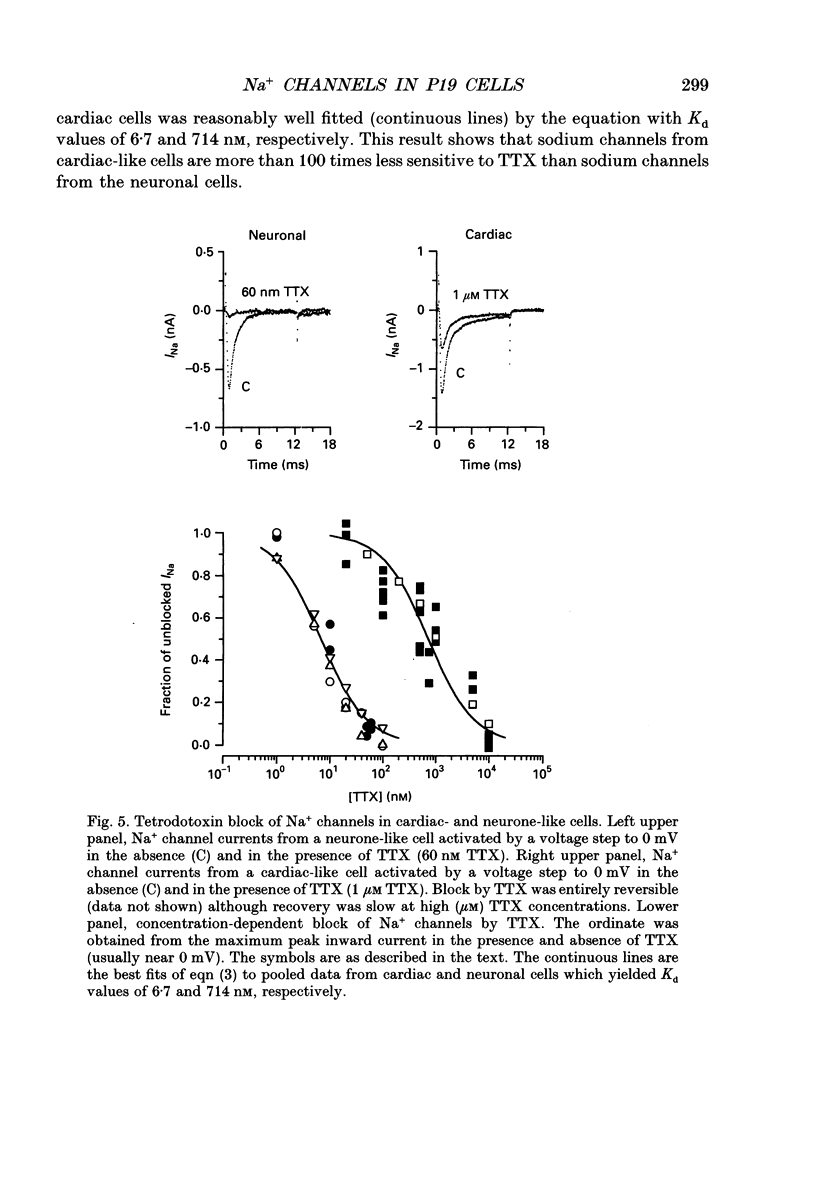
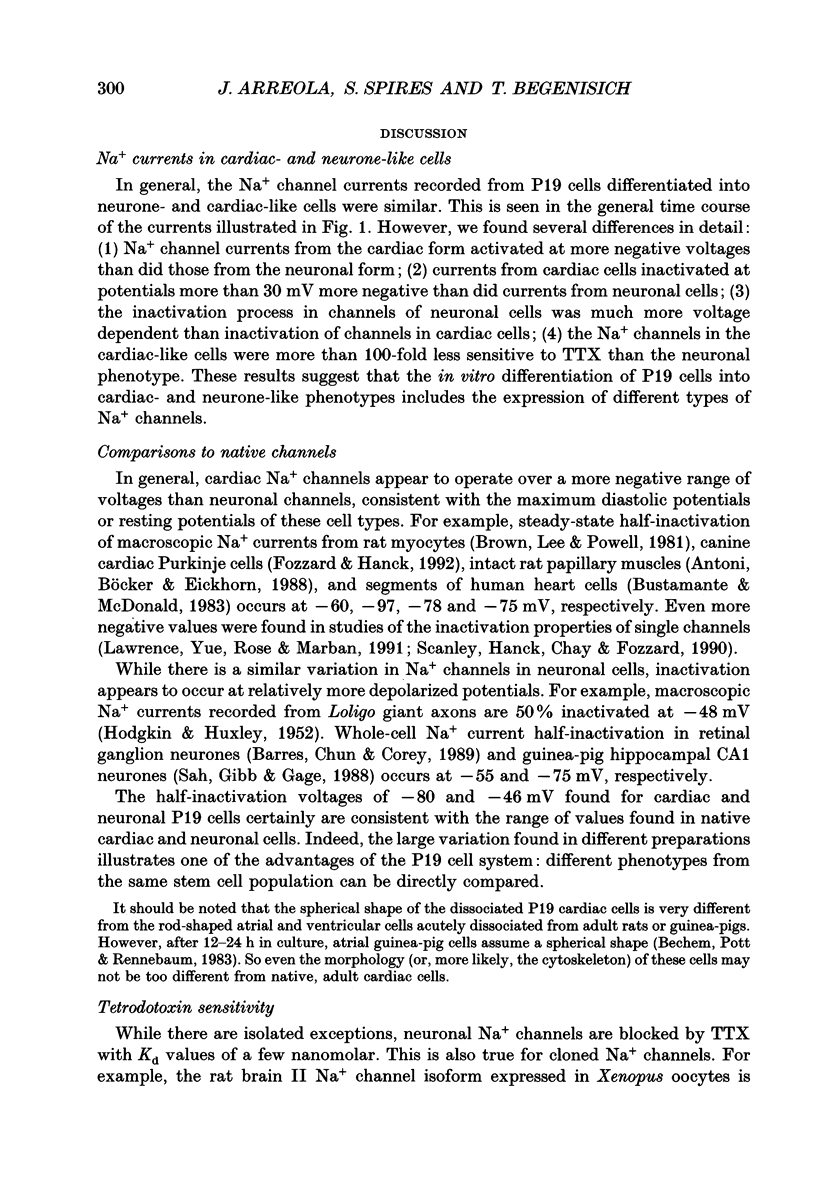
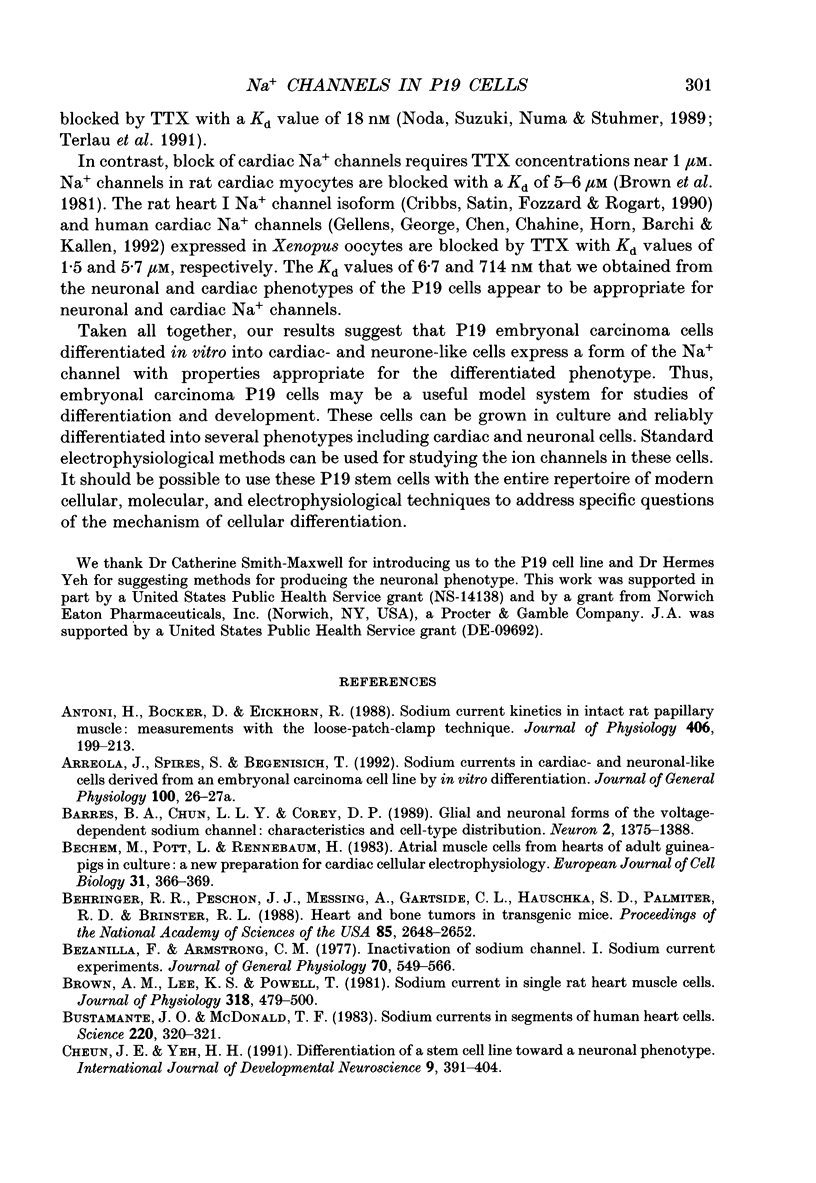
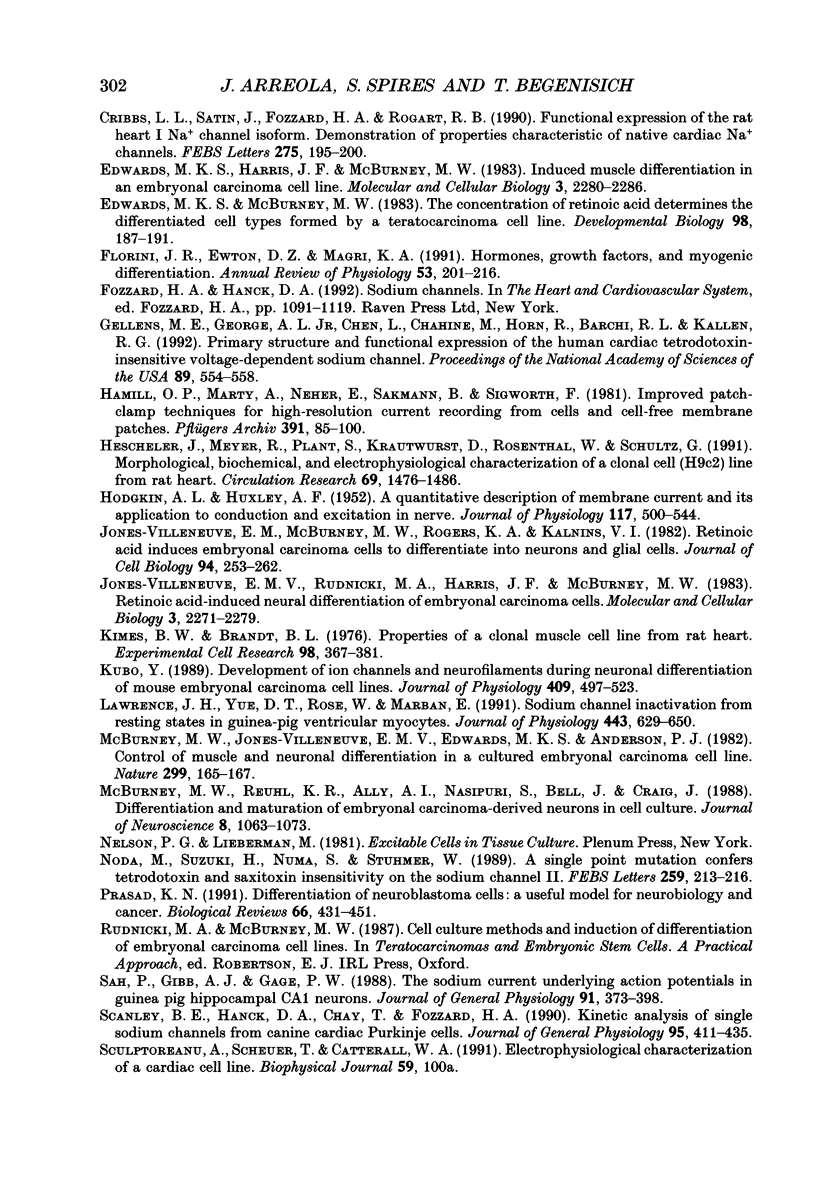
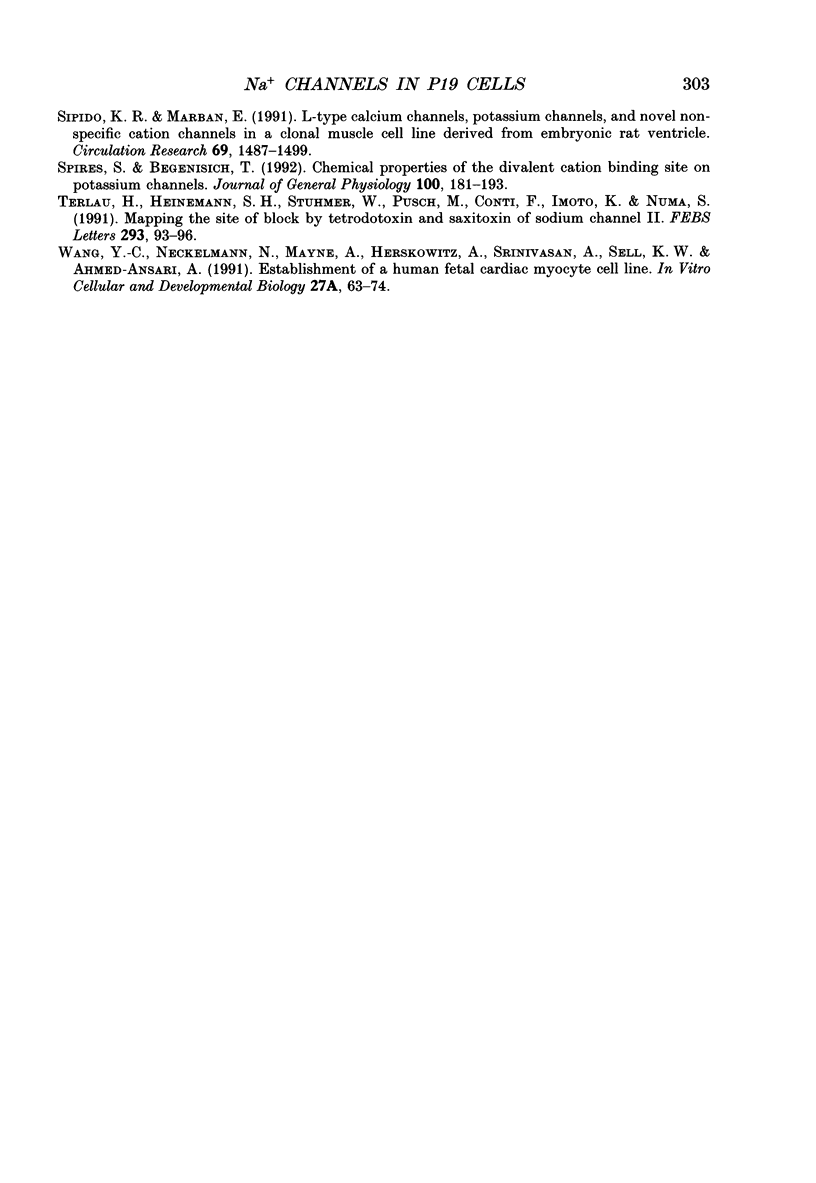
Images in this article
Selected References
These references are in PubMed. This may not be the complete list of references from this article.
- Antoni H., Böcker D., Eickhorn R. Sodium current kinetics in intact rat papillary muscle: measurements with the loose-patch-clamp technique. J Physiol. 1988 Dec;406:199–213. doi: 10.1113/jphysiol.1988.sp017376. [DOI] [PMC free article] [PubMed] [Google Scholar]
- Barres B. A., Chun L. L., Corey D. P. Glial and neuronal forms of the voltage-dependent sodium channel: characteristics and cell-type distribution. Neuron. 1989 Apr;2(4):1375–1388. doi: 10.1016/0896-6273(89)90076-7. [DOI] [PubMed] [Google Scholar]
- Bechem M., Pott L., Rennebaum H. Atrial muscle cells from hearts of adult guinea-pigs in culture: a new preparation for cardiac cellular electrophysiology. Eur J Cell Biol. 1983 Sep;31(2):366–369. [PubMed] [Google Scholar]
- Behringer R. R., Peschon J. J., Messing A., Gartside C. L., Hauschka S. D., Palmiter R. D., Brinster R. L. Heart and bone tumors in transgenic mice. Proc Natl Acad Sci U S A. 1988 Apr;85(8):2648–2652. doi: 10.1073/pnas.85.8.2648. [DOI] [PMC free article] [PubMed] [Google Scholar]
- Bezanilla F., Armstrong C. M. Inactivation of the sodium channel. I. Sodium current experiments. J Gen Physiol. 1977 Nov;70(5):549–566. doi: 10.1085/jgp.70.5.549. [DOI] [PMC free article] [PubMed] [Google Scholar]
- Brown A. M., Lee K. S., Powell T. Sodium current in single rat heart muscle cells. J Physiol. 1981 Sep;318:479–500. doi: 10.1113/jphysiol.1981.sp013879. [DOI] [PMC free article] [PubMed] [Google Scholar]
- Bustamante J. O., McDonald T. F. Sodium currents in segments of human heart cells. Science. 1983 Apr 15;220(4594):320–321. doi: 10.1126/science.6301004. [DOI] [PubMed] [Google Scholar]
- Cheun J. E., Yeh H. H. Differentiation of a stem cell line toward a neuronal phenotype. Int J Dev Neurosci. 1991;9(4):391–404. doi: 10.1016/0736-5748(91)90061-p. [DOI] [PubMed] [Google Scholar]
- Cribbs L. L., Satin J., Fozzard H. A., Rogart R. B. Functional expression of the rat heart I Na+ channel isoform. Demonstration of properties characteristic of native cardiac Na+ channels. FEBS Lett. 1990 Nov 26;275(1-2):195–200. doi: 10.1016/0014-5793(90)81470-9. [DOI] [PubMed] [Google Scholar]
- Edwards M. K., Harris J. F., McBurney M. W. Induced muscle differentiation in an embryonal carcinoma cell line. Mol Cell Biol. 1983 Dec;3(12):2280–2286. doi: 10.1128/mcb.3.12.2280. [DOI] [PMC free article] [PubMed] [Google Scholar]
- Edwards M. K., McBurney M. W. The concentration of retinoic acid determines the differentiated cell types formed by a teratocarcinoma cell line. Dev Biol. 1983 Jul;98(1):187–191. doi: 10.1016/0012-1606(83)90348-2. [DOI] [PubMed] [Google Scholar]
- Florini J. R., Ewton D. Z., Magri K. A. Hormones, growth factors, and myogenic differentiation. Annu Rev Physiol. 1991;53:201–216. doi: 10.1146/annurev.ph.53.030191.001221. [DOI] [PubMed] [Google Scholar]
- Gellens M. E., George A. L., Jr, Chen L. Q., Chahine M., Horn R., Barchi R. L., Kallen R. G. Primary structure and functional expression of the human cardiac tetrodotoxin-insensitive voltage-dependent sodium channel. Proc Natl Acad Sci U S A. 1992 Jan 15;89(2):554–558. doi: 10.1073/pnas.89.2.554. [DOI] [PMC free article] [PubMed] [Google Scholar]
- HODGKIN A. L., HUXLEY A. F. A quantitative description of membrane current and its application to conduction and excitation in nerve. J Physiol. 1952 Aug;117(4):500–544. doi: 10.1113/jphysiol.1952.sp004764. [DOI] [PMC free article] [PubMed] [Google Scholar]
- Hamill O. P., Marty A., Neher E., Sakmann B., Sigworth F. J. Improved patch-clamp techniques for high-resolution current recording from cells and cell-free membrane patches. Pflugers Arch. 1981 Aug;391(2):85–100. doi: 10.1007/BF00656997. [DOI] [PubMed] [Google Scholar]
- Hescheler J., Meyer R., Plant S., Krautwurst D., Rosenthal W., Schultz G. Morphological, biochemical, and electrophysiological characterization of a clonal cell (H9c2) line from rat heart. Circ Res. 1991 Dec;69(6):1476–1486. doi: 10.1161/01.res.69.6.1476. [DOI] [PubMed] [Google Scholar]
- Jones-Villeneuve E. M., McBurney M. W., Rogers K. A., Kalnins V. I. Retinoic acid induces embryonal carcinoma cells to differentiate into neurons and glial cells. J Cell Biol. 1982 Aug;94(2):253–262. doi: 10.1083/jcb.94.2.253. [DOI] [PMC free article] [PubMed] [Google Scholar]
- Jones-Villeneuve E. M., Rudnicki M. A., Harris J. F., McBurney M. W. Retinoic acid-induced neural differentiation of embryonal carcinoma cells. Mol Cell Biol. 1983 Dec;3(12):2271–2279. doi: 10.1128/mcb.3.12.2271. [DOI] [PMC free article] [PubMed] [Google Scholar]
- Kimes B. W., Brandt B. L. Properties of a clonal muscle cell line from rat heart. Exp Cell Res. 1976 Mar 15;98(2):367–381. doi: 10.1016/0014-4827(76)90447-x. [DOI] [PubMed] [Google Scholar]
- Kubo Y. Development of ion channels and neurofilaments during neuronal differentiation of mouse embryonal carcinoma cell lines. J Physiol. 1989 Feb;409:497–523. doi: 10.1113/jphysiol.1989.sp017510. [DOI] [PMC free article] [PubMed] [Google Scholar]
- Lawrence J. H., Yue D. T., Rose W. C., Marban E. Sodium channel inactivation from resting states in guinea-pig ventricular myocytes. J Physiol. 1991 Nov;443:629–650. doi: 10.1113/jphysiol.1991.sp018855. [DOI] [PMC free article] [PubMed] [Google Scholar]
- McBurney M. W., Jones-Villeneuve E. M., Edwards M. K., Anderson P. J. Control of muscle and neuronal differentiation in a cultured embryonal carcinoma cell line. Nature. 1982 Sep 9;299(5879):165–167. doi: 10.1038/299165a0. [DOI] [PubMed] [Google Scholar]
- McBurney M. W., Reuhl K. R., Ally A. I., Nasipuri S., Bell J. C., Craig J. Differentiation and maturation of embryonal carcinoma-derived neurons in cell culture. J Neurosci. 1988 Mar;8(3):1063–1073. doi: 10.1523/JNEUROSCI.08-03-01063.1988. [DOI] [PMC free article] [PubMed] [Google Scholar]
- Noda M., Suzuki H., Numa S., Stühmer W. A single point mutation confers tetrodotoxin and saxitoxin insensitivity on the sodium channel II. FEBS Lett. 1989 Dec 18;259(1):213–216. doi: 10.1016/0014-5793(89)81531-5. [DOI] [PubMed] [Google Scholar]
- Prasad K. N. Differentiation of neuroblastoma cells: a useful model for neurobiology and cancer. Biol Rev Camb Philos Soc. 1991 Nov;66(4):431–451. doi: 10.1111/j.1469-185x.1991.tb01148.x. [DOI] [PubMed] [Google Scholar]
- Sah P., Gibb A. J., Gage P. W. The sodium current underlying action potentials in guinea pig hippocampal CA1 neurons. J Gen Physiol. 1988 Mar;91(3):373–398. doi: 10.1085/jgp.91.3.373. [DOI] [PMC free article] [PubMed] [Google Scholar]
- Scanley B. E., Hanck D. A., Chay T., Fozzard H. A. Kinetic analysis of single sodium channels from canine cardiac Purkinje cells. J Gen Physiol. 1990 Mar;95(3):411–437. doi: 10.1085/jgp.95.3.411. [DOI] [PMC free article] [PubMed] [Google Scholar]
- Sipido K. R., Marban E. L-type calcium channels, potassium channels, and novel nonspecific cation channels in a clonal muscle cell line derived from embryonic rat ventricle. Circ Res. 1991 Dec;69(6):1487–1499. doi: 10.1161/01.res.69.6.1487. [DOI] [PubMed] [Google Scholar]
- Spires S., Begenisich T. Chemical properties of the divalent cation binding site on potassium channels. J Gen Physiol. 1992 Aug;100(2):181–193. doi: 10.1085/jgp.100.2.181. [DOI] [PMC free article] [PubMed] [Google Scholar]
- Terlau H., Heinemann S. H., Stühmer W., Pusch M., Conti F., Imoto K., Numa S. Mapping the site of block by tetrodotoxin and saxitoxin of sodium channel II. FEBS Lett. 1991 Nov 18;293(1-2):93–96. doi: 10.1016/0014-5793(91)81159-6. [DOI] [PubMed] [Google Scholar]
- Wang Y. C., Neckelmann N., Mayne A., Herskowitz A., Srinivasan A., Sell K. W., Ahmed-Ansari A. Establishment of a human fetal cardiac myocyte cell line. In Vitro Cell Dev Biol. 1991 Jan;27(1):63–74. doi: 10.1007/BF02630896. [DOI] [PubMed] [Google Scholar]