Abstract
1. The activation of the L-type calcium current (ICa) was studied in normally polarized (-100 mV) cut skeletal muscle fibres of the frog with the double Vaseline-gap voltage-clamp technique. Both external and internal solutions were Ca2+ buffered. Solutions were made in order to minimize all but the Ca2+ current. 2. The voltage-dependent components of the time course of activation were determined by two procedures: fast and slow components were evaluated by multiexponential fitting to current traces elicited by long voltage pulses (5 s) after removing inactivation; fast components were also determined by short voltage pulses having different duration (0.5-70 ms). 3. The components of deactivation were evaluated after removing the charge-movement current from the total tail current by the difference between two short (50 and 70 ms) voltage pulses to 10 mV, moving the same intramembrane charge. Two exponential components, fast and slow (time constants, 6 +/- 0.3 and 90 +/- 7 ms at -100 mV; n = 26), were found. 4. The time onset of ICa was evaluated either by multiexponential fitting to the ICa activation or by pulses of different duration to test the beginning of the 'on' and 'off' inequality. This was at about 2 ms, denoting that it was very early. 5. The time constant vs. voltage plots indicated the presence of four voltage-dependent components in the activation pathway. Various kinetic models are discussed. Models with independent transitions, like a Hodgkin-Huxley scheme, were excluded. Suitable models were a five-state sequential and a four-state cyclic with a branch scheme. The latter gave the best simulation of the data. 6. The steady-state activation curve saturated at high potentials. It had a half-voltage value of 1 +/- 0.2 mV and the opening probability was only 0.82 +/- 0.2 at 20 mV (n = 32). This result implies a larger number of functional calcium channels than was previously supposed and is in agreement with the number of dihydropyridine (DHP) receptors calculated for the tubular system.
Full text
PDF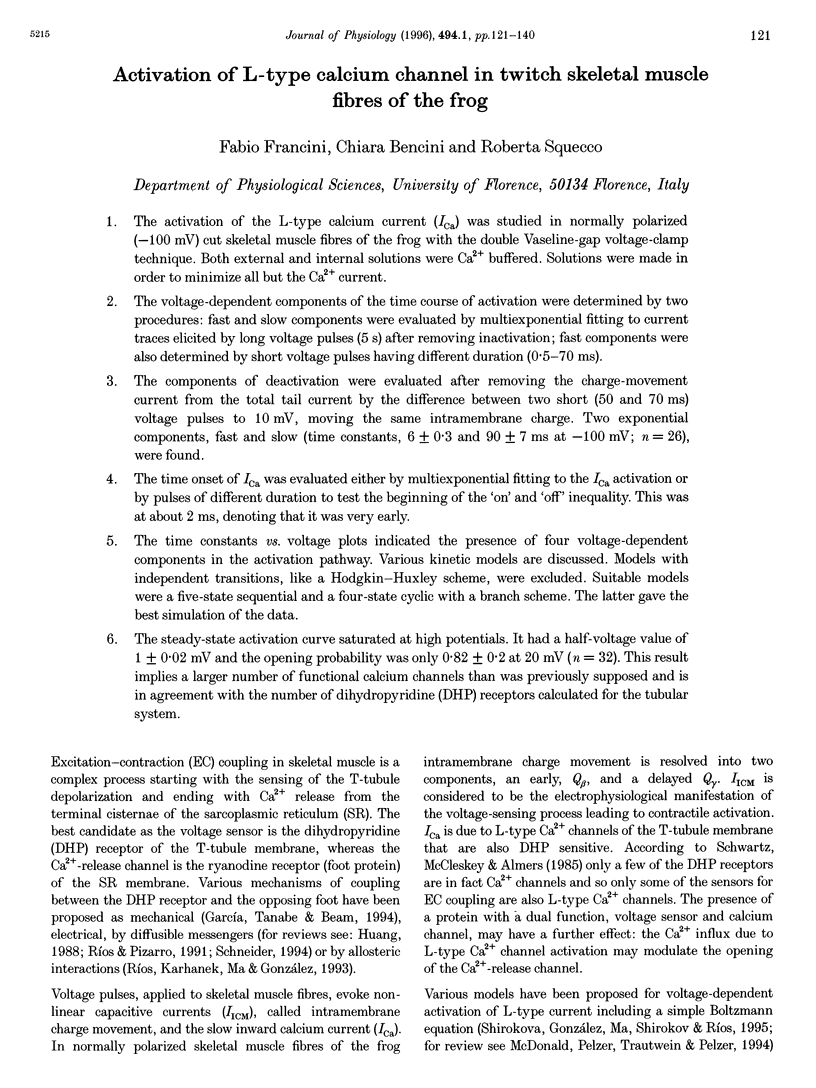
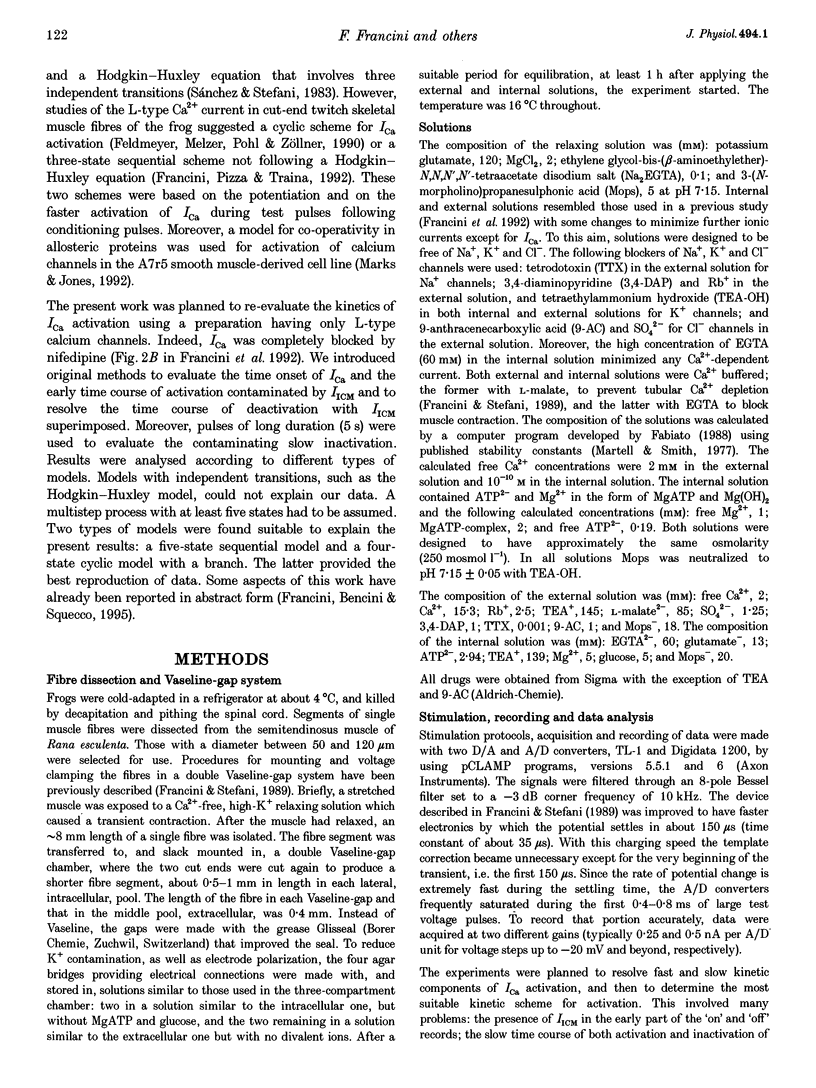
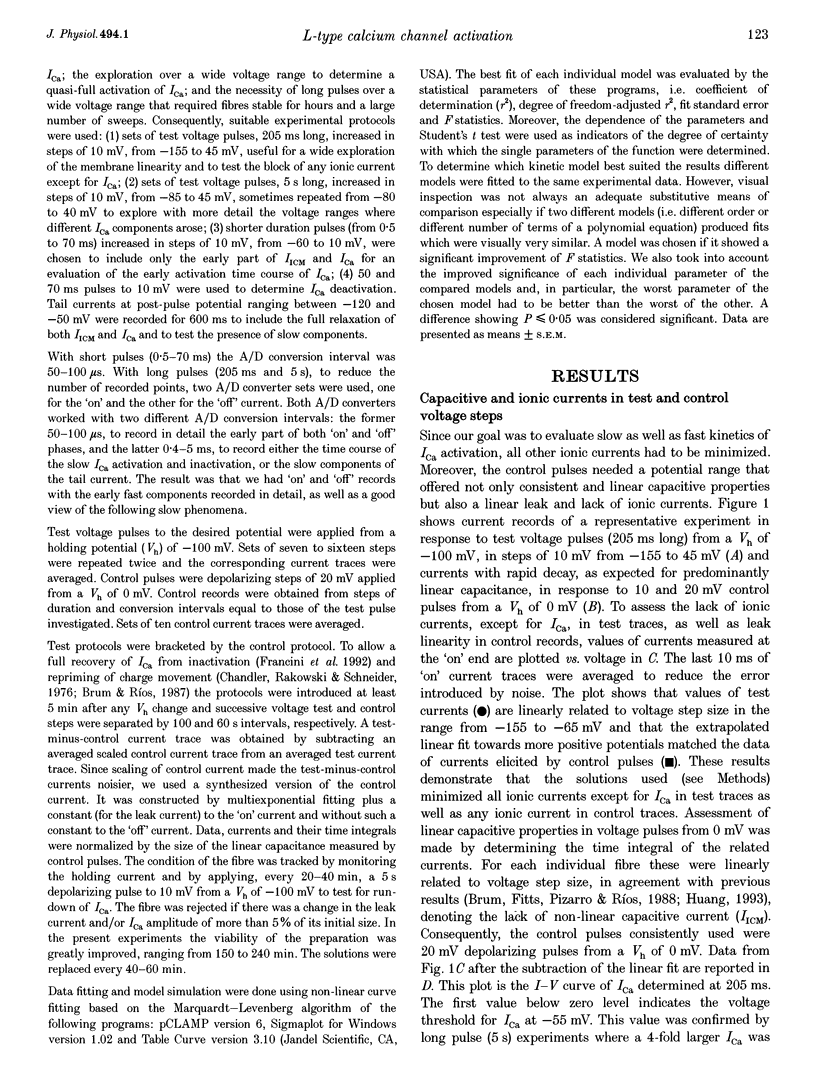
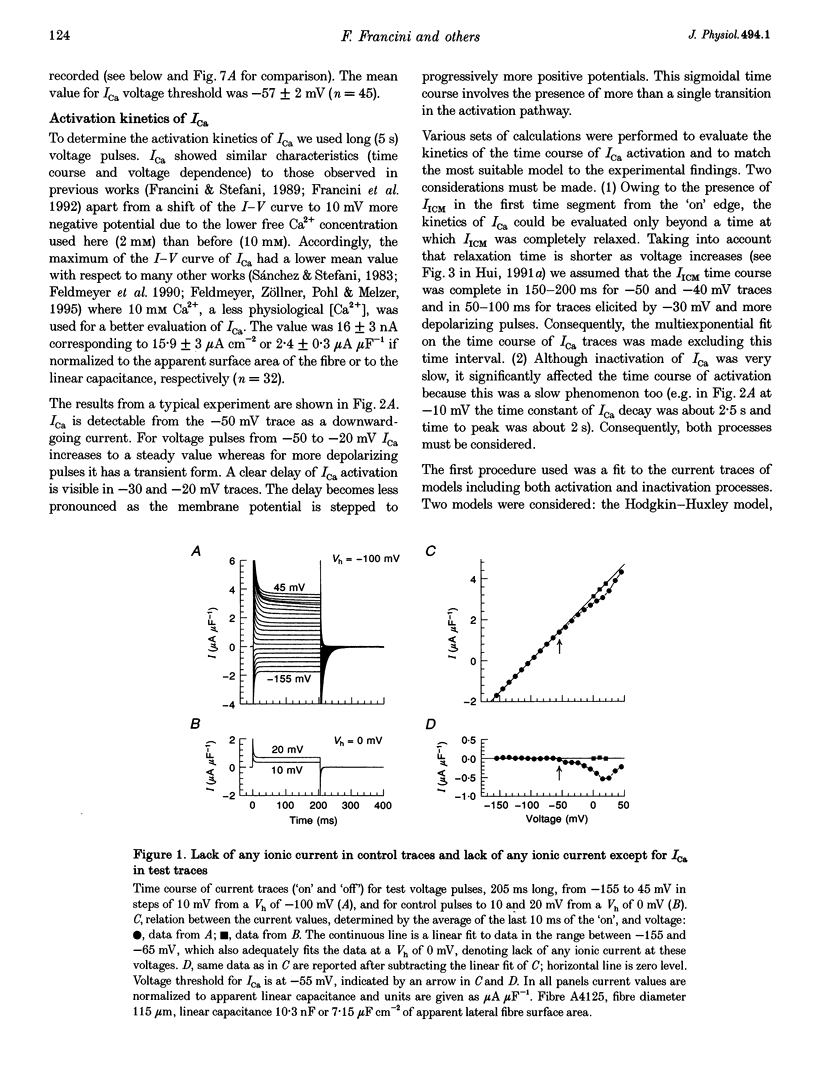
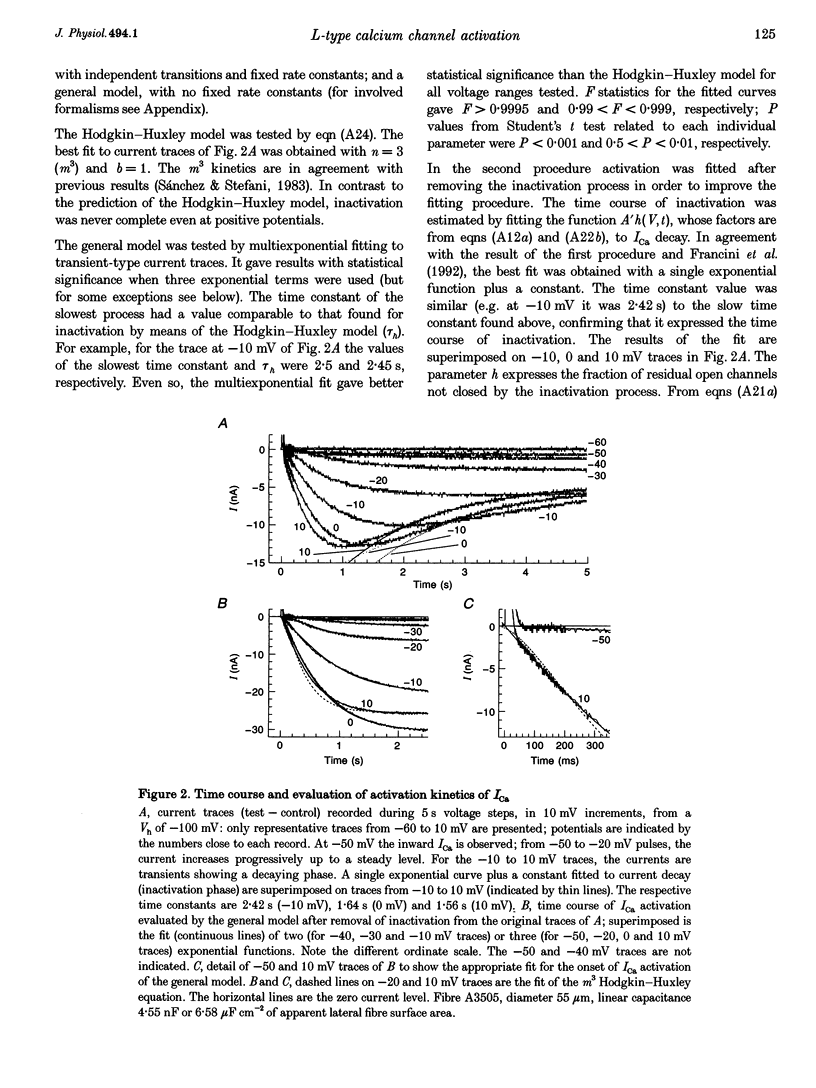
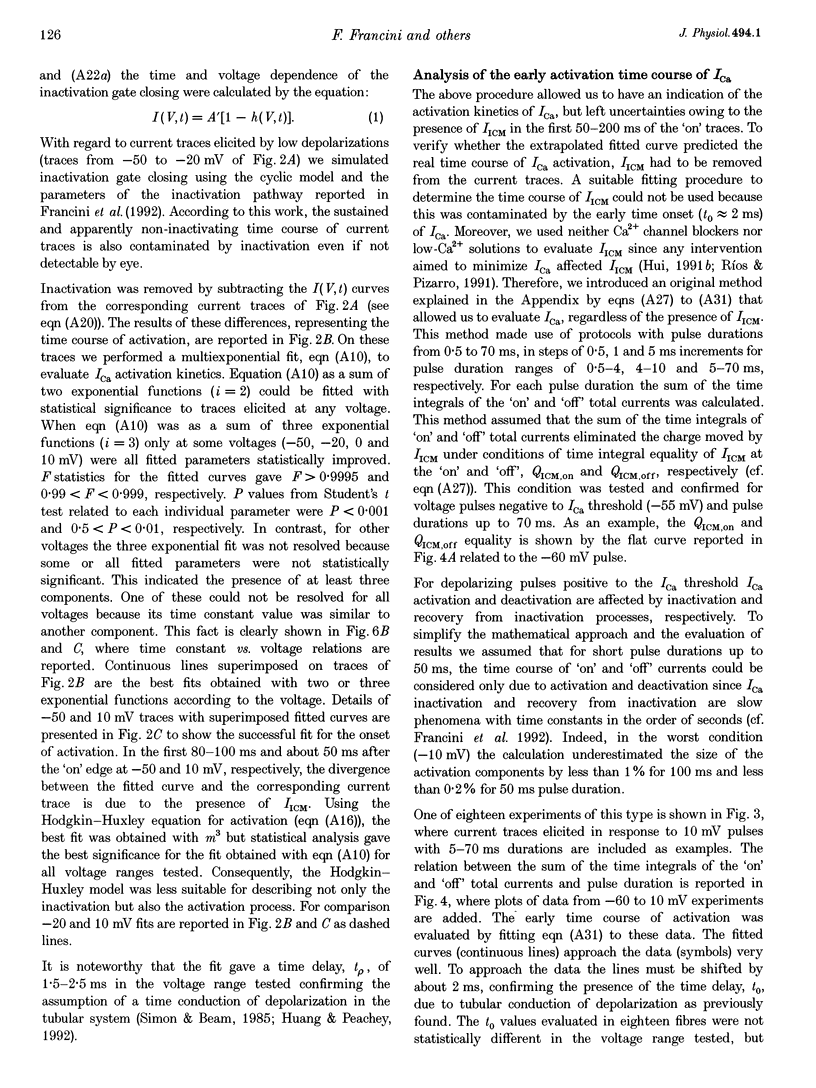
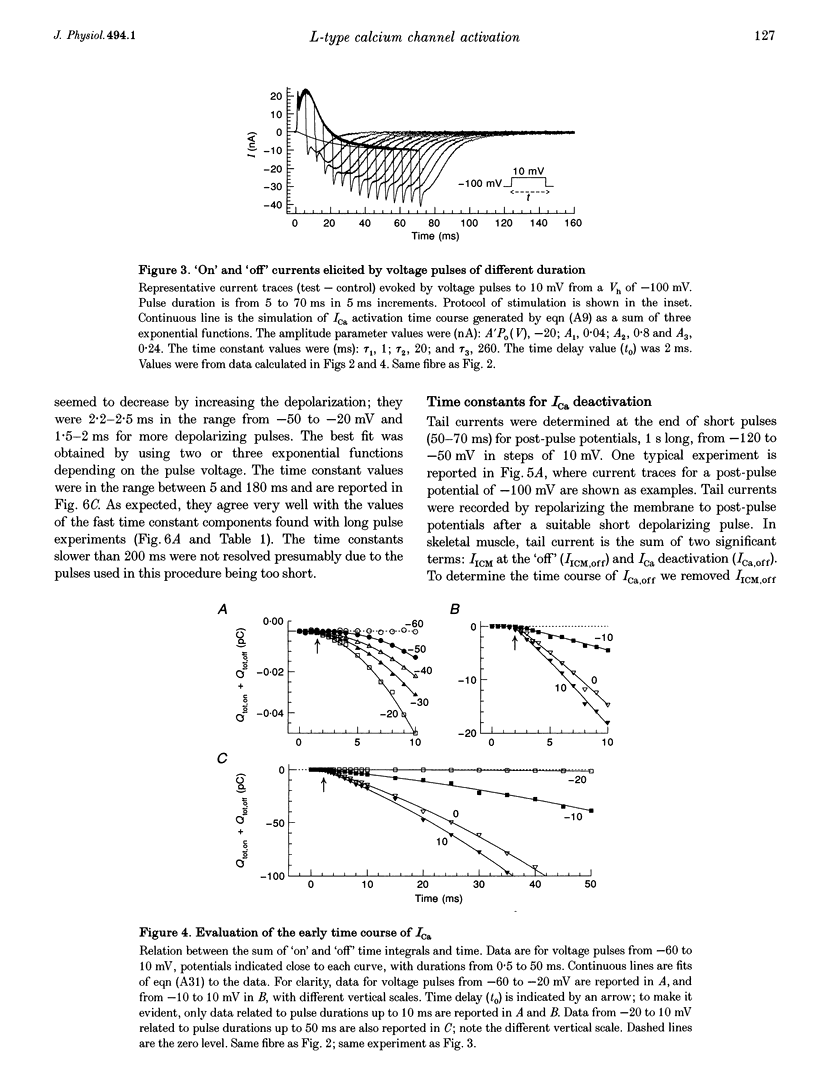
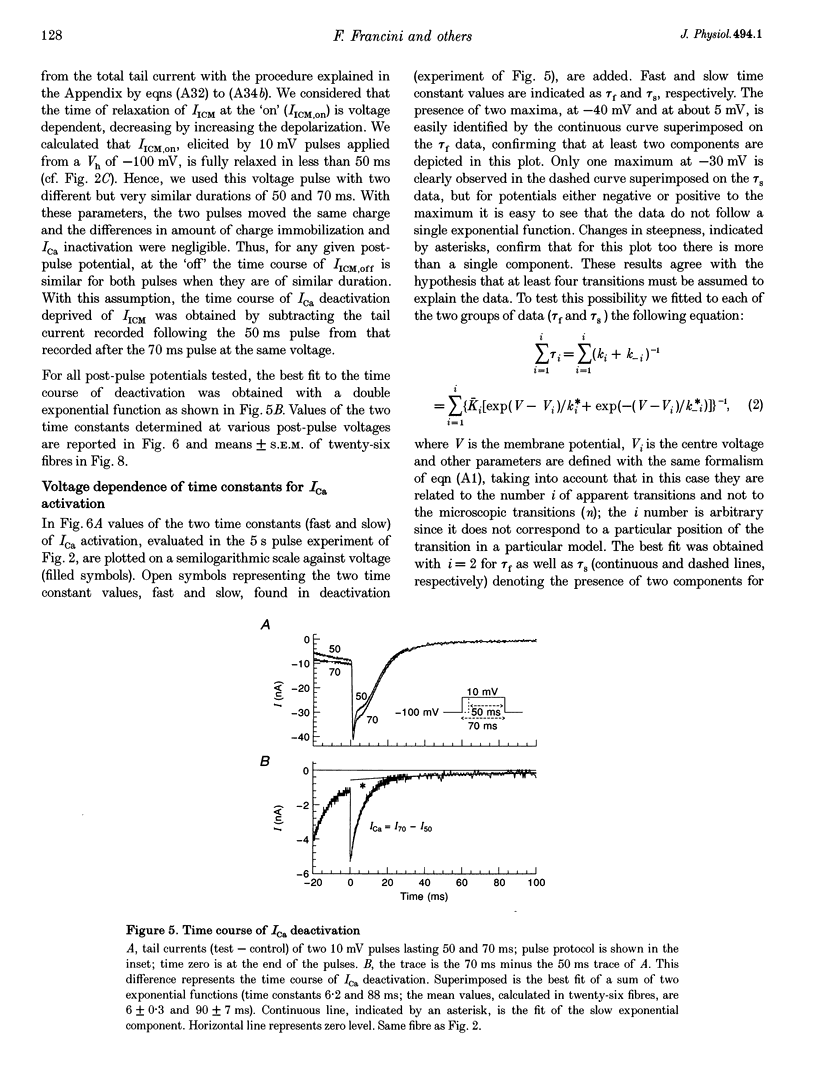
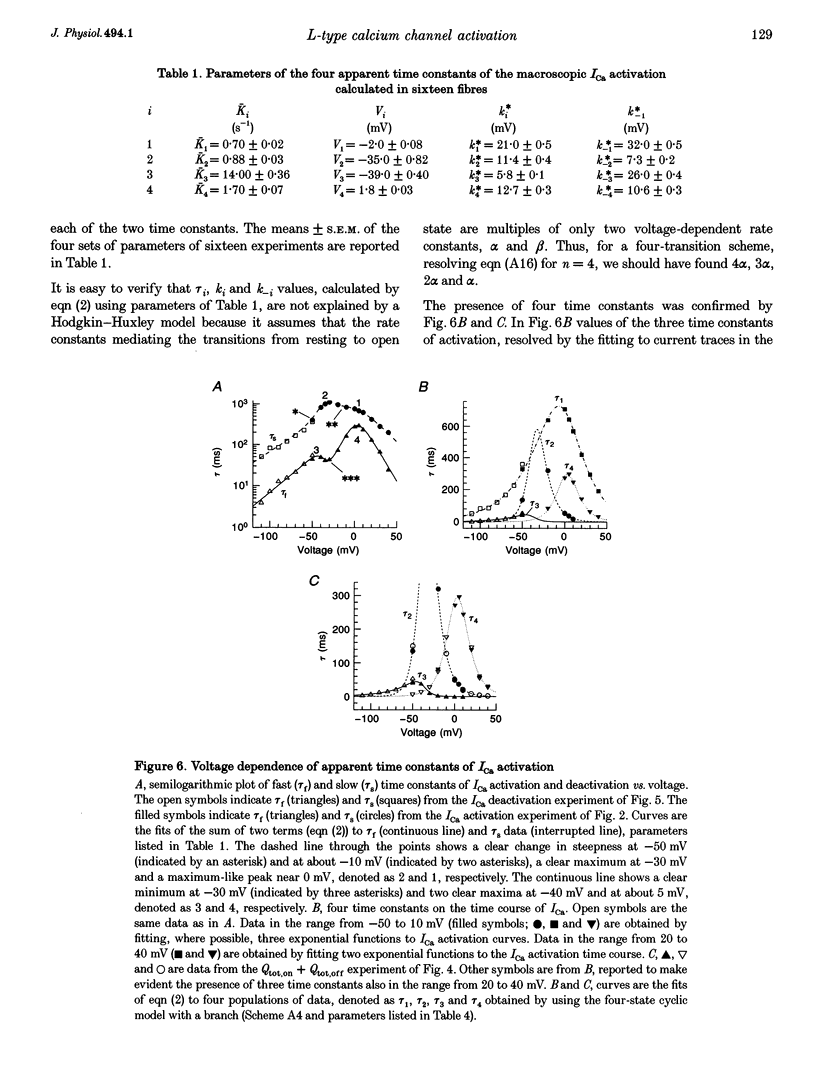
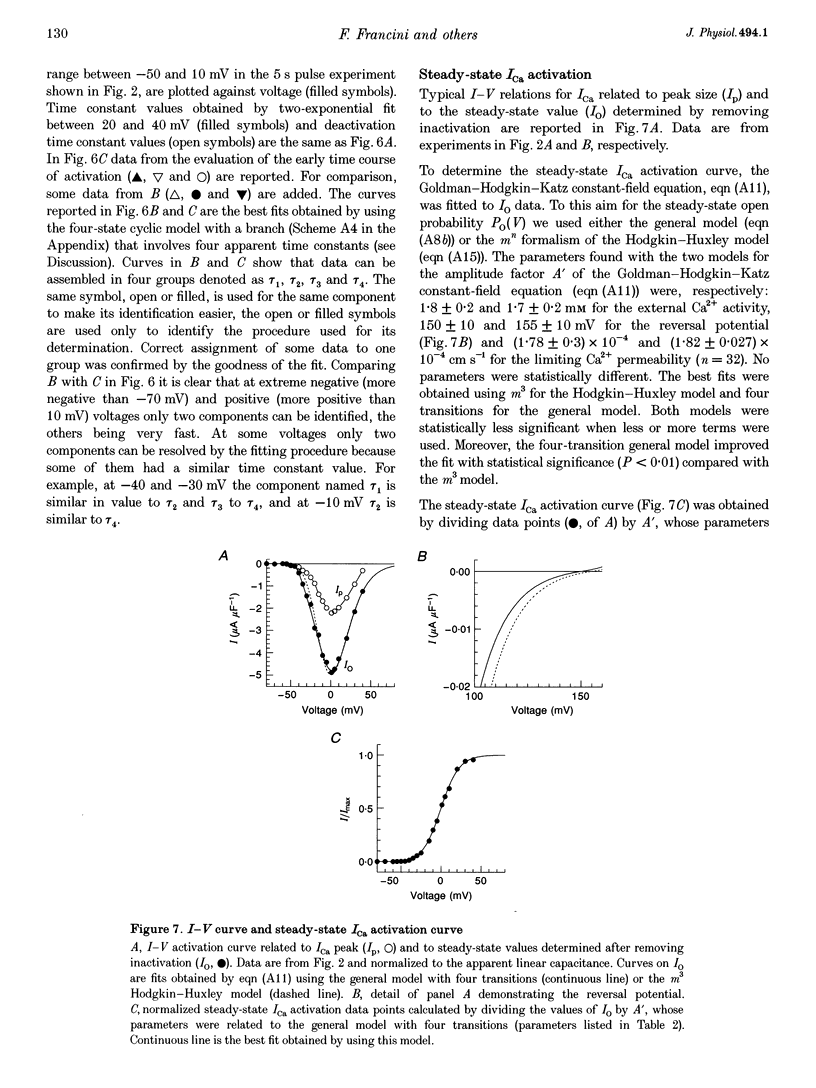
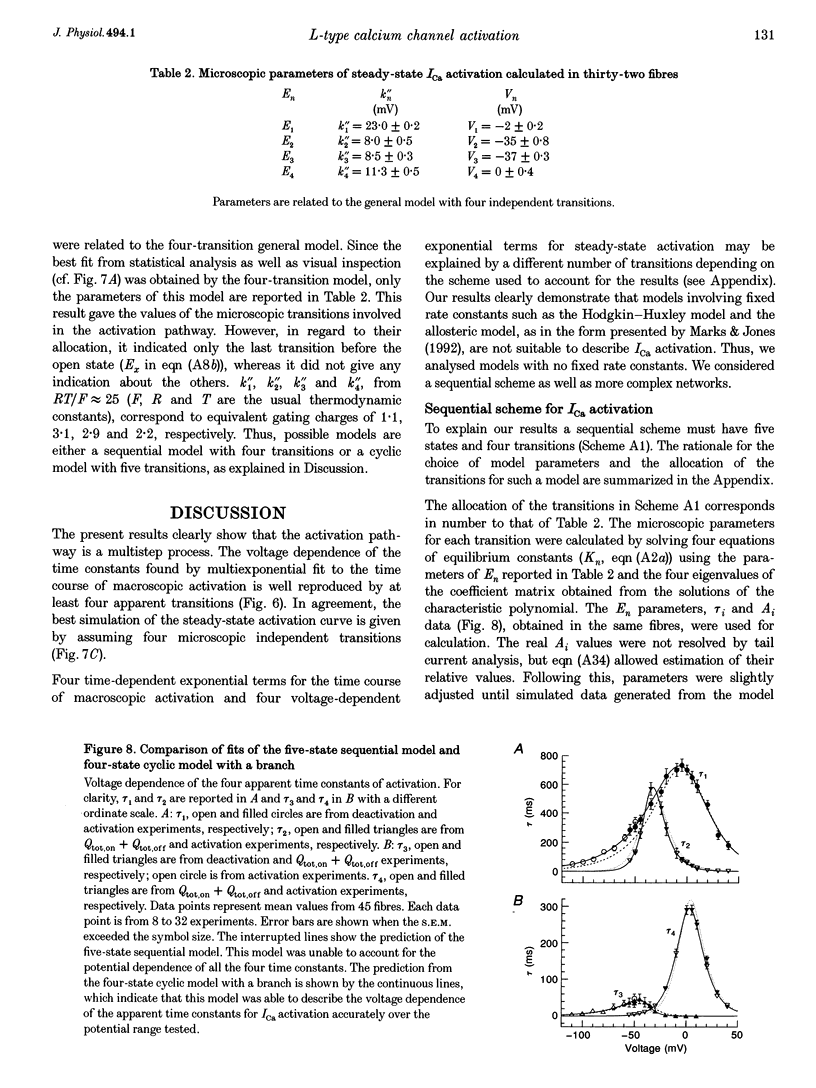
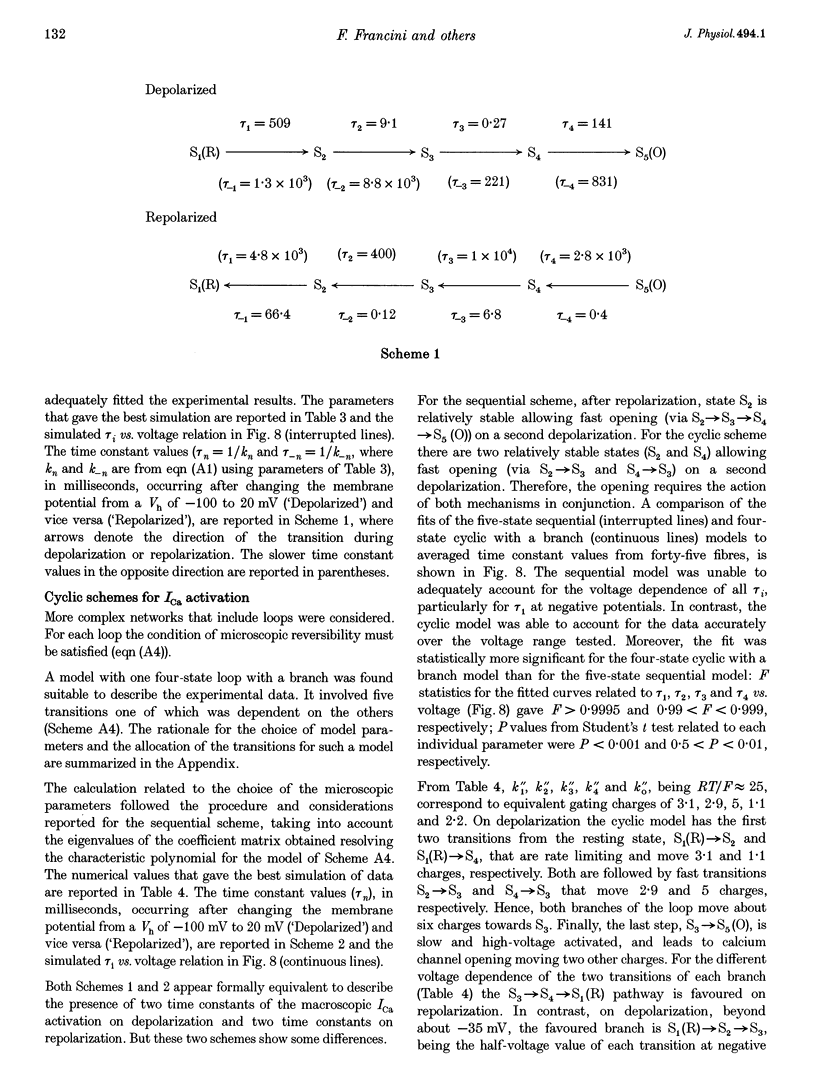
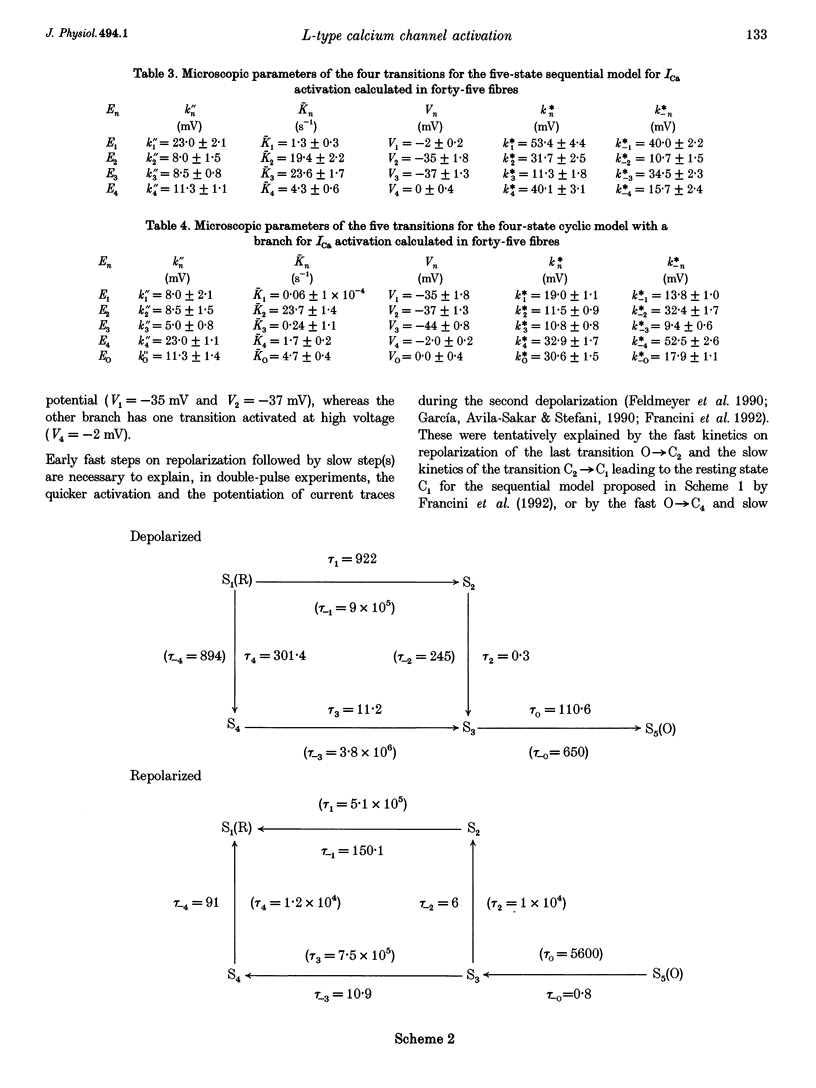
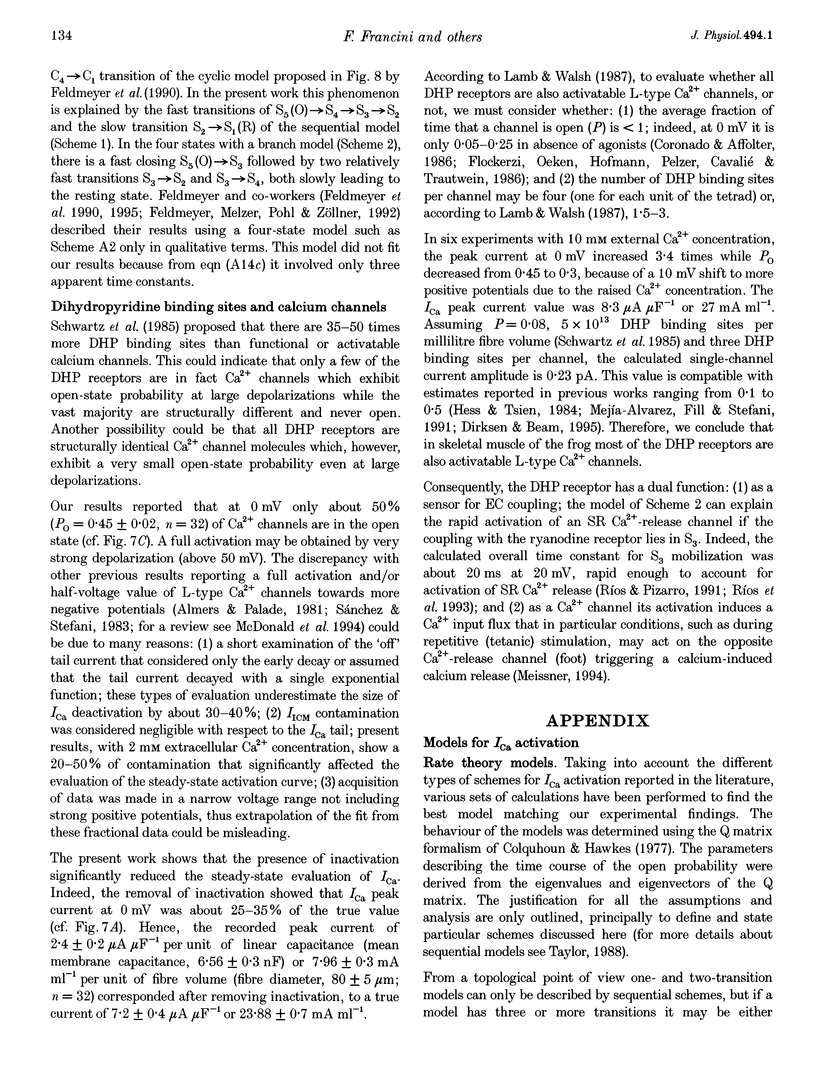
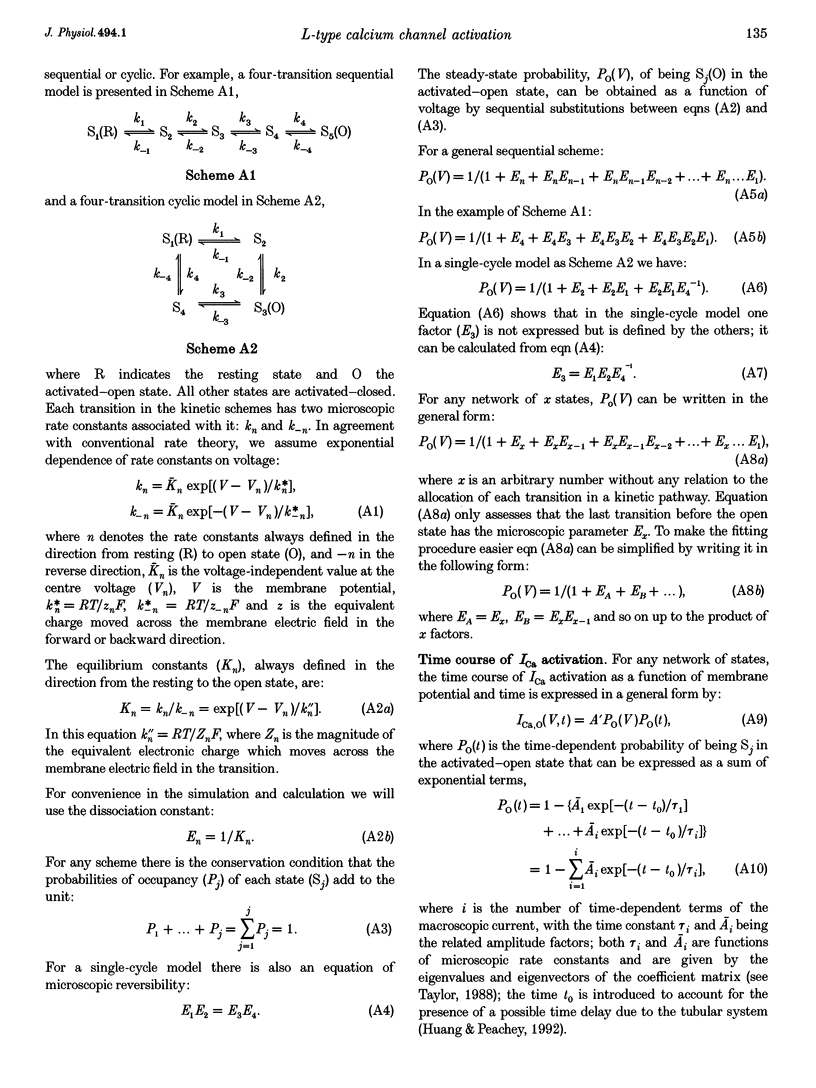
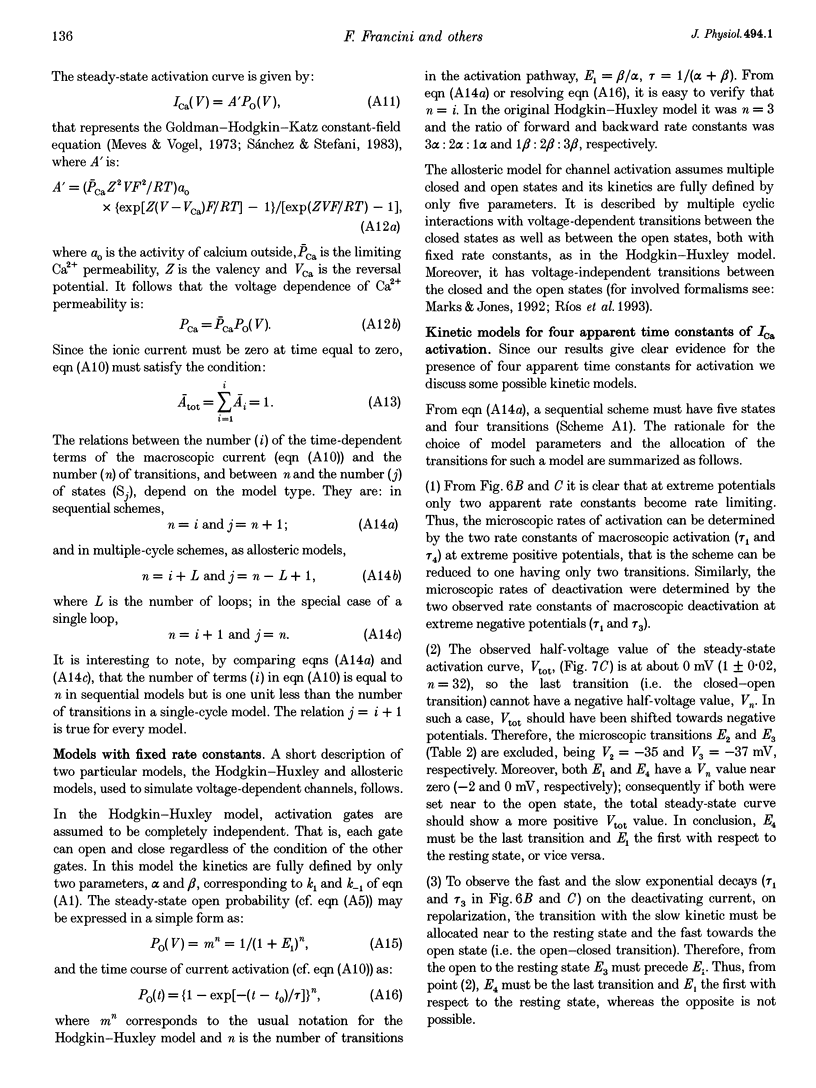
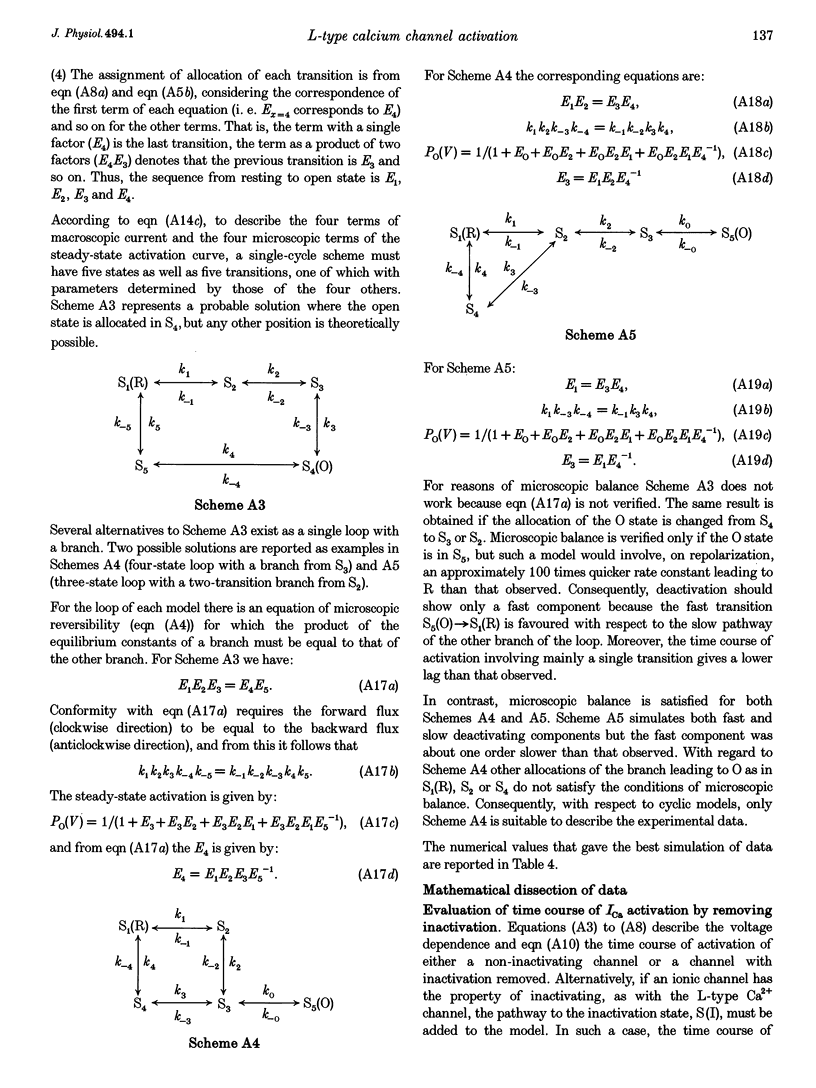
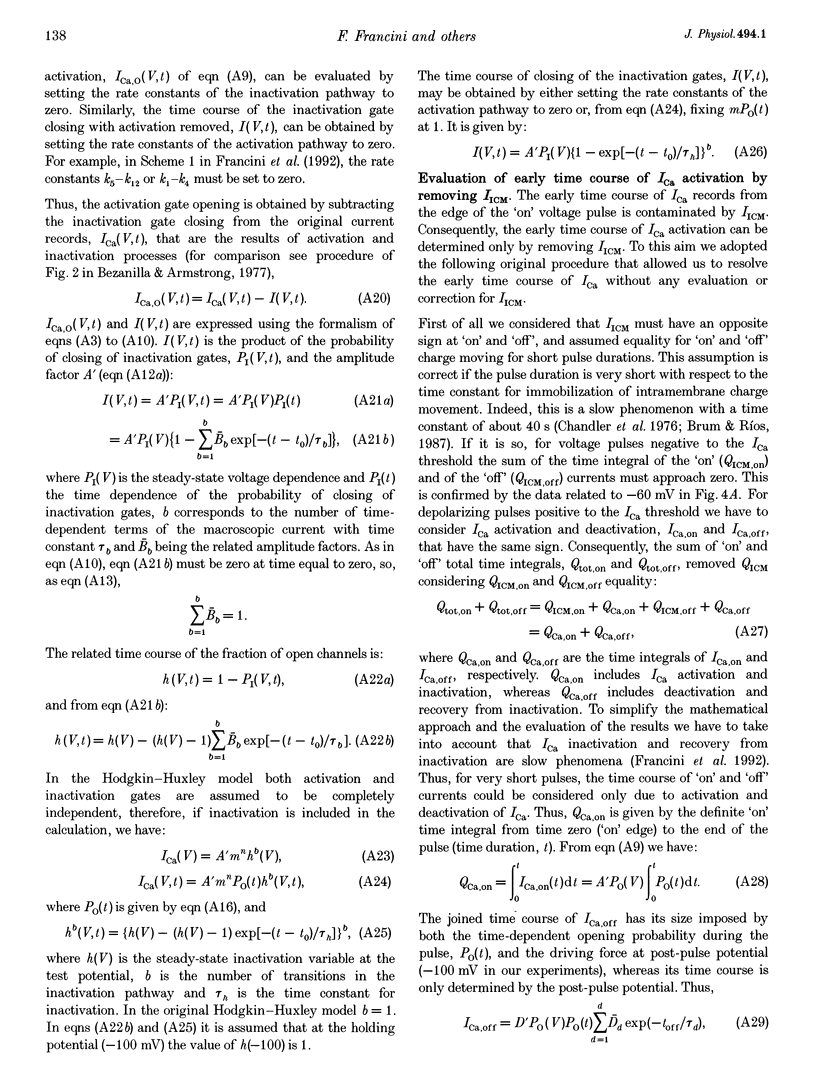
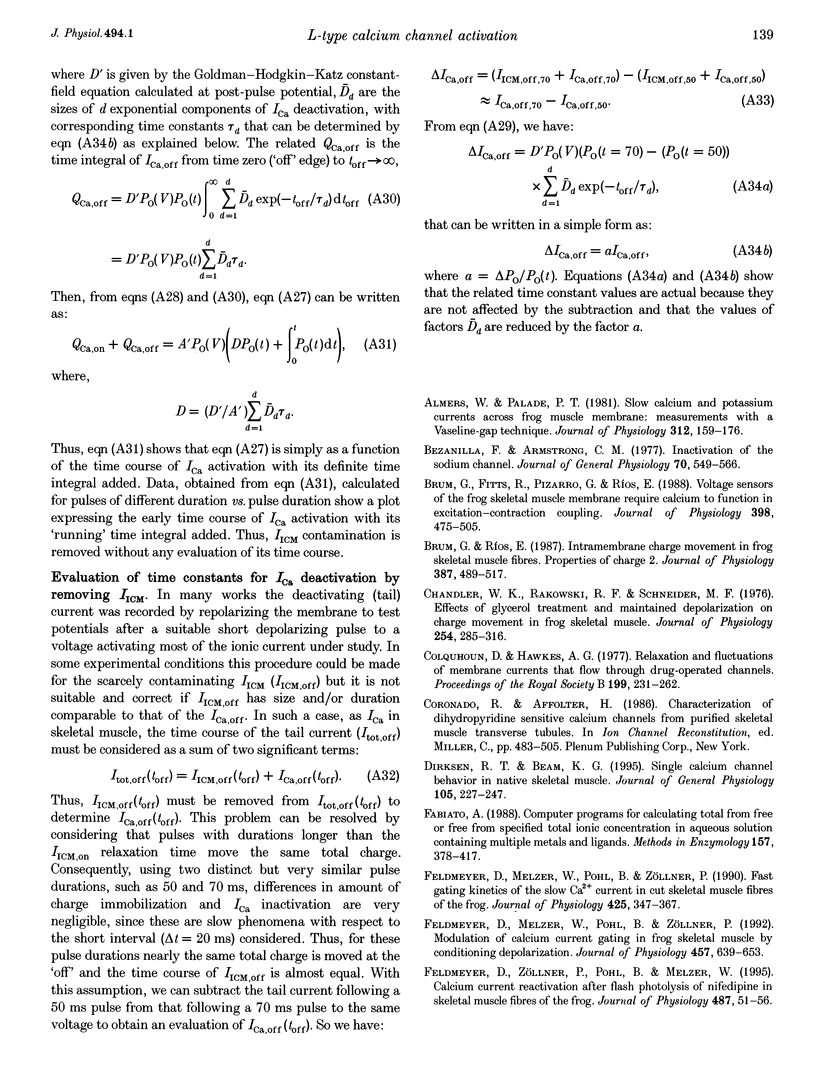
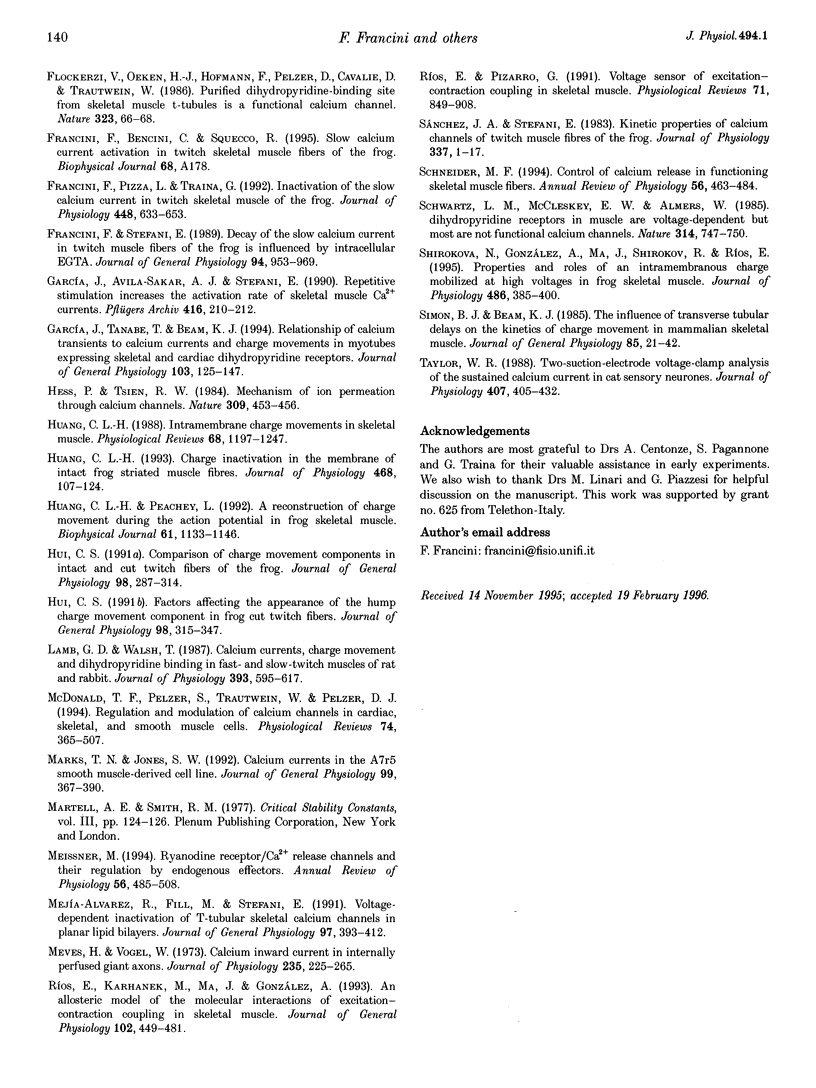
Selected References
These references are in PubMed. This may not be the complete list of references from this article.
- Almers W., Palade P. T. Slow calcium and potassium currents across frog muscle membrane: measurements with a vaseline-gap technique. J Physiol. 1981 Mar;312:159–176. doi: 10.1113/jphysiol.1981.sp013622. [DOI] [PMC free article] [PubMed] [Google Scholar]
- Bezanilla F., Armstrong C. M. Inactivation of the sodium channel. I. Sodium current experiments. J Gen Physiol. 1977 Nov;70(5):549–566. doi: 10.1085/jgp.70.5.549. [DOI] [PMC free article] [PubMed] [Google Scholar]
- Brum G., Fitts R., Pizarro G., Ríos E. Voltage sensors of the frog skeletal muscle membrane require calcium to function in excitation-contraction coupling. J Physiol. 1988 Apr;398:475–505. doi: 10.1113/jphysiol.1988.sp017053. [DOI] [PMC free article] [PubMed] [Google Scholar]
- Brum G., Rios E. Intramembrane charge movement in frog skeletal muscle fibres. Properties of charge 2. J Physiol. 1987 Jun;387:489–517. doi: 10.1113/jphysiol.1987.sp016586. [DOI] [PMC free article] [PubMed] [Google Scholar]
- Chandler W. K., Rakowski R. F., Schneider M. F. Effects of glycerol treatment and maintained depolarization on charge movement in skeletal muscle. J Physiol. 1976 Jan;254(2):285–316. doi: 10.1113/jphysiol.1976.sp011233. [DOI] [PMC free article] [PubMed] [Google Scholar]
- Colquhoun D., Hawkes A. G. Relaxation and fluctuations of membrane currents that flow through drug-operated channels. Proc R Soc Lond B Biol Sci. 1977 Nov 14;199(1135):231–262. doi: 10.1098/rspb.1977.0137. [DOI] [PubMed] [Google Scholar]
- Dirksen R. T., Beam K. G. Single calcium channel behavior in native skeletal muscle. J Gen Physiol. 1995 Feb;105(2):227–247. doi: 10.1085/jgp.105.2.227. [DOI] [PMC free article] [PubMed] [Google Scholar]
- Fabiato A. Computer programs for calculating total from specified free or free from specified total ionic concentrations in aqueous solutions containing multiple metals and ligands. Methods Enzymol. 1988;157:378–417. doi: 10.1016/0076-6879(88)57093-3. [DOI] [PubMed] [Google Scholar]
- Feldmeyer D., Melzer W., Pohl B., Zöllner P. Fast gating kinetics of the slow Ca2+ current in cut skeletal muscle fibres of the frog. J Physiol. 1990 Jun;425:347–367. doi: 10.1113/jphysiol.1990.sp018107. [DOI] [PMC free article] [PubMed] [Google Scholar]
- Feldmeyer D., Melzer W., Pohl B., Zöllner P. Modulation of calcium current gating in frog skeletal muscle by conditioning depolarization. J Physiol. 1992 Nov;457:639–653. doi: 10.1113/jphysiol.1992.sp019399. [DOI] [PMC free article] [PubMed] [Google Scholar]
- Feldmeyer D., Zöllner P., Pohl B., Melzer W. Calcium current reactivation after flash photolysis of nifedipine in skeletal muscle fibres of the frog. J Physiol. 1995 Aug 15;487(1):51–56. doi: 10.1113/jphysiol.1995.sp020860. [DOI] [PMC free article] [PubMed] [Google Scholar]
- Flockerzi V., Oeken H. J., Hofmann F., Pelzer D., Cavalié A., Trautwein W. Purified dihydropyridine-binding site from skeletal muscle t-tubules is a functional calcium channel. Nature. 1986 Sep 4;323(6083):66–68. doi: 10.1038/323066a0. [DOI] [PubMed] [Google Scholar]
- Francini F., Pizza L., Traina G. Inactivation of the slow calcium current in twitch skeletal muscle fibres of the frog. J Physiol. 1992 Mar;448:633–653. doi: 10.1113/jphysiol.1992.sp019062. [DOI] [PMC free article] [PubMed] [Google Scholar]
- Francini F., Stefani E. Decay of the slow calcium current in twitch muscle fibers of the frog is influenced by intracellular EGTA. J Gen Physiol. 1989 Nov;94(5):953–969. doi: 10.1085/jgp.94.5.953. [DOI] [PMC free article] [PubMed] [Google Scholar]
- Garcia J., Avila-Sakar A. J., Stefani E. Repetitive stimulation increases the activation rate of skeletal muscle Ca2+ currents. Pflugers Arch. 1990 Apr;416(1-2):210–212. doi: 10.1007/BF00370245. [DOI] [PubMed] [Google Scholar]
- García J., Tanabe T., Beam K. G. Relationship of calcium transients to calcium currents and charge movements in myotubes expressing skeletal and cardiac dihydropyridine receptors. J Gen Physiol. 1994 Jan;103(1):125–147. doi: 10.1085/jgp.103.1.125. [DOI] [PMC free article] [PubMed] [Google Scholar]
- Hess P., Tsien R. W. Mechanism of ion permeation through calcium channels. 1984 May 31-Jun 6Nature. 309(5967):453–456. doi: 10.1038/309453a0. [DOI] [PubMed] [Google Scholar]
- Huang C. L. Charge inactivation in the membrane of intact frog striated muscle fibers. J Physiol. 1993 Aug;468:107–124. doi: 10.1113/jphysiol.1993.sp019762. [DOI] [PMC free article] [PubMed] [Google Scholar]
- Huang C. L. Intramembrane charge movements in skeletal muscle. Physiol Rev. 1988 Oct;68(4):1197–1147. doi: 10.1152/physrev.1988.68.4.1197. [DOI] [PubMed] [Google Scholar]
- Huang C. L., Peachey L. D. A reconstruction of charge movement during the action potential in frog skeletal muscle. Biophys J. 1992 May;61(5):1133–1146. doi: 10.1016/S0006-3495(92)81923-9. [DOI] [PMC free article] [PubMed] [Google Scholar]
- Hui C. S. Comparison of charge movement components in intact and cut twitch fibers of the frog. Effects of stretch and temperature. J Gen Physiol. 1991 Aug;98(2):287–314. doi: 10.1085/jgp.98.2.287. [DOI] [PMC free article] [PubMed] [Google Scholar]
- Hui C. S. Factors affecting the appearance of the hump charge movement component in frog cut twitch fibers. J Gen Physiol. 1991 Aug;98(2):315–347. doi: 10.1085/jgp.98.2.315. [DOI] [PMC free article] [PubMed] [Google Scholar]
- Lamb G. D., Walsh T. Calcium currents, charge movement and dihydropyridine binding in fast- and slow-twitch muscles of rat and rabbit. J Physiol. 1987 Dec;393:595–617. doi: 10.1113/jphysiol.1987.sp016843. [DOI] [PMC free article] [PubMed] [Google Scholar]
- Marks T. N., Jones S. W. Calcium currents in the A7r5 smooth muscle-derived cell line. An allosteric model for calcium channel activation and dihydropyridine agonist action. J Gen Physiol. 1992 Mar;99(3):367–390. doi: 10.1085/jgp.99.3.367. [DOI] [PMC free article] [PubMed] [Google Scholar]
- McDonald T. F., Pelzer S., Trautwein W., Pelzer D. J. Regulation and modulation of calcium channels in cardiac, skeletal, and smooth muscle cells. Physiol Rev. 1994 Apr;74(2):365–507. doi: 10.1152/physrev.1994.74.2.365. [DOI] [PubMed] [Google Scholar]
- Meissner G. Ryanodine receptor/Ca2+ release channels and their regulation by endogenous effectors. Annu Rev Physiol. 1994;56:485–508. doi: 10.1146/annurev.ph.56.030194.002413. [DOI] [PubMed] [Google Scholar]
- Mejía-Alvarez R., Fill M., Stefani E. Voltage-dependent inactivation of T-tubular skeletal calcium channels in planar lipid bilayers. J Gen Physiol. 1991 Feb;97(2):393–412. doi: 10.1085/jgp.97.2.393. [DOI] [PMC free article] [PubMed] [Google Scholar]
- Meves H., Vogel W. Calcium inward currents in internally perfused giant axons. J Physiol. 1973 Nov;235(1):225–265. doi: 10.1113/jphysiol.1973.sp010386. [DOI] [PMC free article] [PubMed] [Google Scholar]
- Ríos E., Karhanek M., Ma J., González A. An allosteric model of the molecular interactions of excitation-contraction coupling in skeletal muscle. J Gen Physiol. 1993 Sep;102(3):449–481. doi: 10.1085/jgp.102.3.449. [DOI] [PMC free article] [PubMed] [Google Scholar]
- Ríos E., Pizarro G. Voltage sensor of excitation-contraction coupling in skeletal muscle. Physiol Rev. 1991 Jul;71(3):849–908. doi: 10.1152/physrev.1991.71.3.849. [DOI] [PubMed] [Google Scholar]
- Schneider M. F. Control of calcium release in functioning skeletal muscle fibers. Annu Rev Physiol. 1994;56:463–484. doi: 10.1146/annurev.ph.56.030194.002335. [DOI] [PubMed] [Google Scholar]
- Schwartz L. M., McCleskey E. W., Almers W. Dihydropyridine receptors in muscle are voltage-dependent but most are not functional calcium channels. 1985 Apr 25-May 1Nature. 314(6013):747–751. doi: 10.1038/314747a0. [DOI] [PubMed] [Google Scholar]
- Shirokova N., González A., Ma J., Shirokov R., Ríos E. Properties and roles of an intramembranous charge mobilized at high voltages in frog skeletal muscle. J Physiol. 1995 Jul 15;486(Pt 2):385–400. doi: 10.1113/jphysiol.1995.sp020820. [DOI] [PMC free article] [PubMed] [Google Scholar]
- Simon B. J., Beam K. G. The influence of transverse tubular delays on the kinetics of charge movement in mammalian skeletal muscle. J Gen Physiol. 1985 Jan;85(1):21–42. doi: 10.1085/jgp.85.1.21. [DOI] [PMC free article] [PubMed] [Google Scholar]
- Sánchez J. A., Stefani E. Kinetic properties of calcium channels of twitch muscle fibres of the frog. J Physiol. 1983 Apr;337:1–17. doi: 10.1113/jphysiol.1983.sp014607. [DOI] [PMC free article] [PubMed] [Google Scholar]
- Taylor W. R. Two-suction-electrode voltage-clamp analysis of the sustained calcium current in cat sensory neurones. J Physiol. 1988 Dec;407:405–432. doi: 10.1113/jphysiol.1988.sp017423. [DOI] [PMC free article] [PubMed] [Google Scholar]