Abstract
1. Enzymatically isolated skeletal muscle fibres were used to investigate the effects of applying acetylcholine (ACh) onto the endplate area on intracellular free calcium concentration ([Ca2+]i) measured using the indicator indo-1 and single channel activity using the patch clamp technique. 2. Using a Tyrode solution containing 5 microM tetrodotoxin (TTX) as extracellular solution, ACh applications (at 0.1 or 1 mM) onto the endplate induced intracellular free calcium transients the mean maximal amplitude of which was 360 +/- 30 nM from a mean resting value of 72 +/- 7 nM (n = 13). In cells bathed with a K(+)-rich solution (145 mM K+), applications of ACh (0.1 mM) induced transient rises in [Ca2+]i from a mean resting value of 53 +/- 7 nM to a maximum of 222 +/- 24 nM (n = 33). 3. In cell-attached membrane patches at the endplate membrane of muscle fibres bathed in a K(+)-rich external solution, using a pipette filled with Tyrode solution, external application of 0.1 mM ACh could induce a transient burst opening of channels carrying an outward current of an average amplitude of 4.6 +/- 0.2 pA at 0 mV (n = 8). 4. These channels were characterized as Ca2(+)-activated K+ channels. At 0 mV, in inside-out patches excised from the endplate membrane area, they displayed a conductance of 60 and 224 pS in the presence of Tyrode and K(+)-rich solution in the pipette, respectively. Half-maximum activation was found for a [Ca2+]i close to 4 microM. The channels showed a typical voltage dependence. In outside-out patches these channels were shown to be blocked by 100 nM charybdotoxin (CTX). 5. In fibres bathed in a Tyrode solution containing TTX (5 microM), CTX had no clear effect on the change in membrane voltage, recorded near the endplate with a single intracellular microelectrode, in response to the application of ACh. 6. Although the physiological relevance of this ACh-induced K+ channel activation remains unclear, results suggest that, in the presence of a physiological extracellular [Ca2+], Ca2+ entry through the endplate nicotinic receptors can produce a local increase in [Ca2+]i, sufficient to trigger the opening of Ca2+-activated K+ channels in the adjacent surface membrane.
Full text
PDF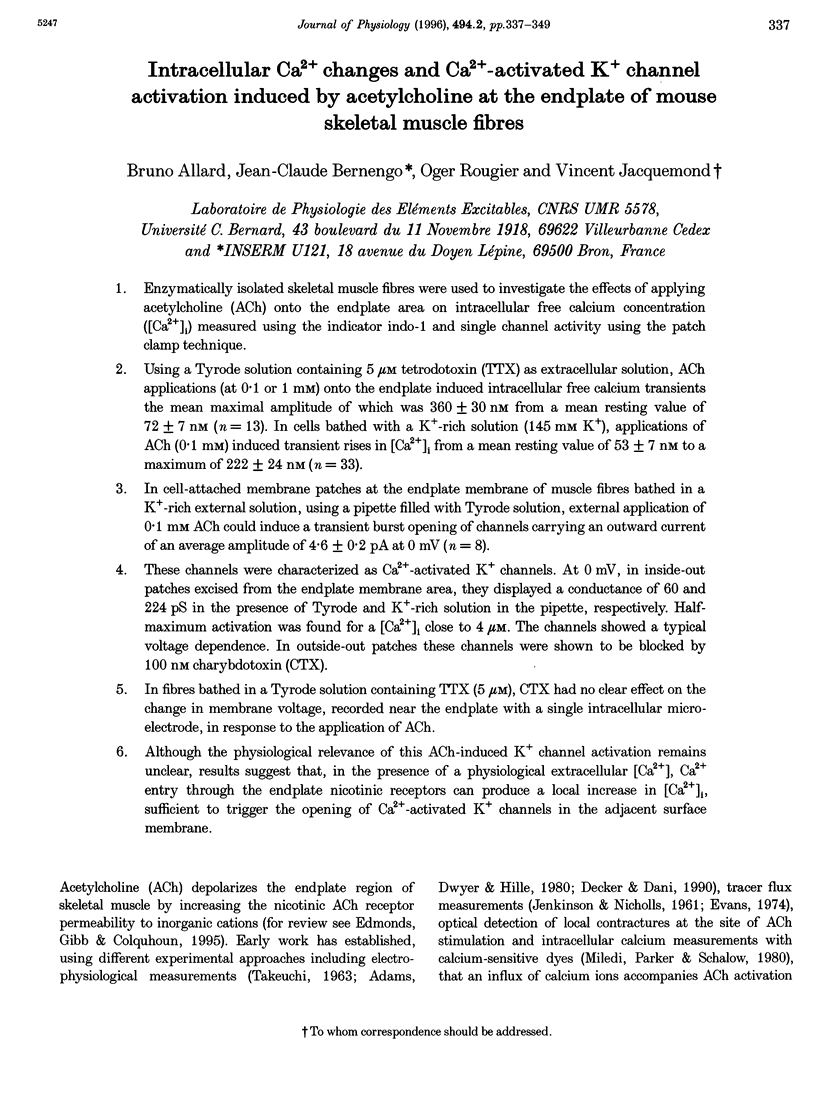
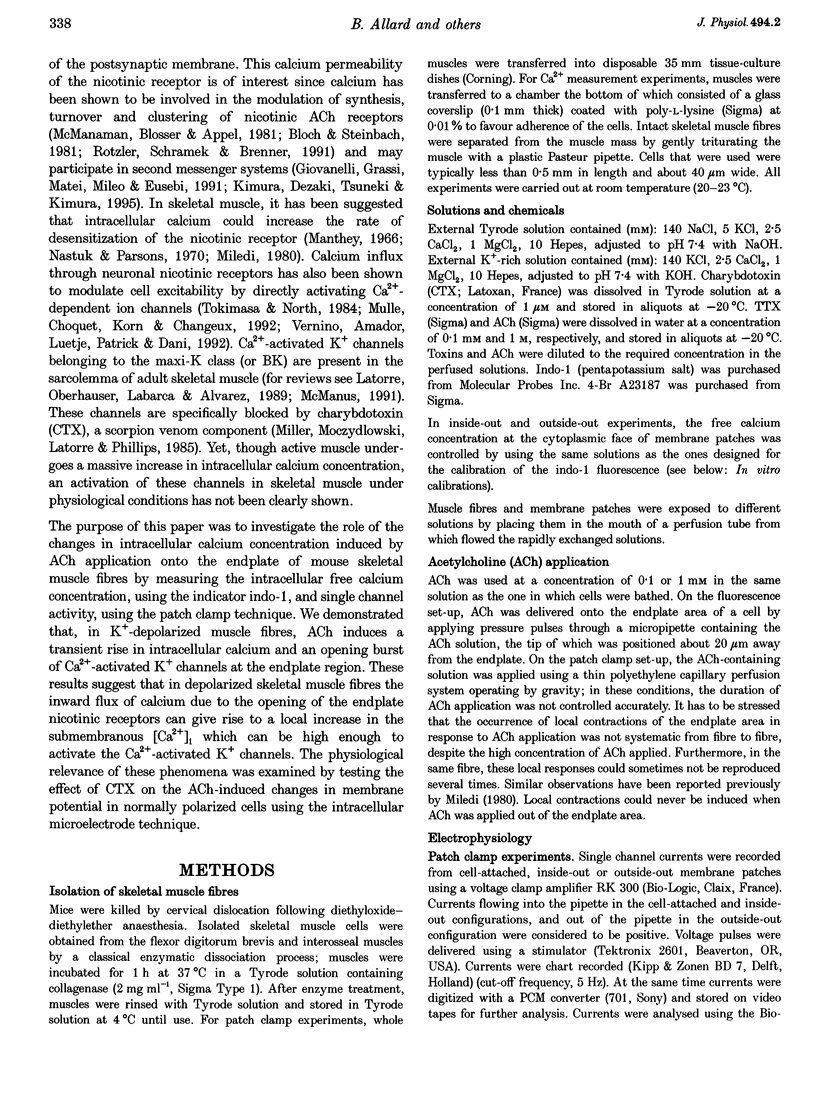
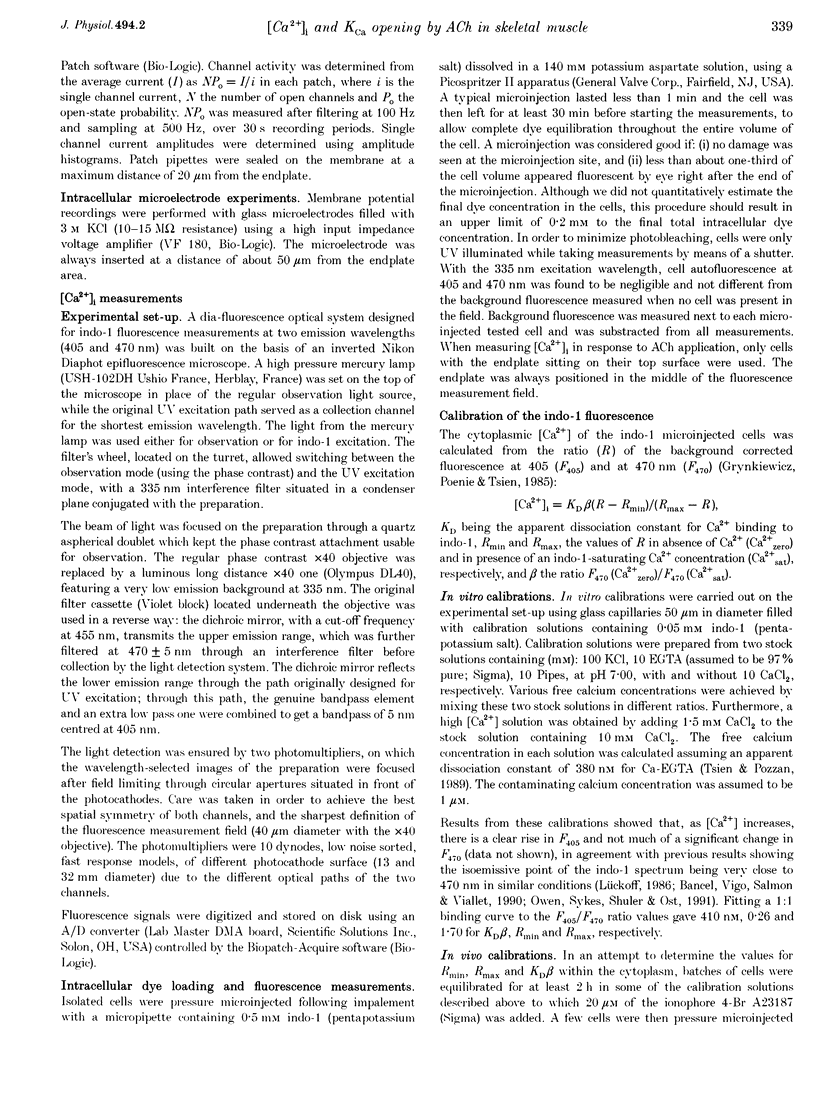
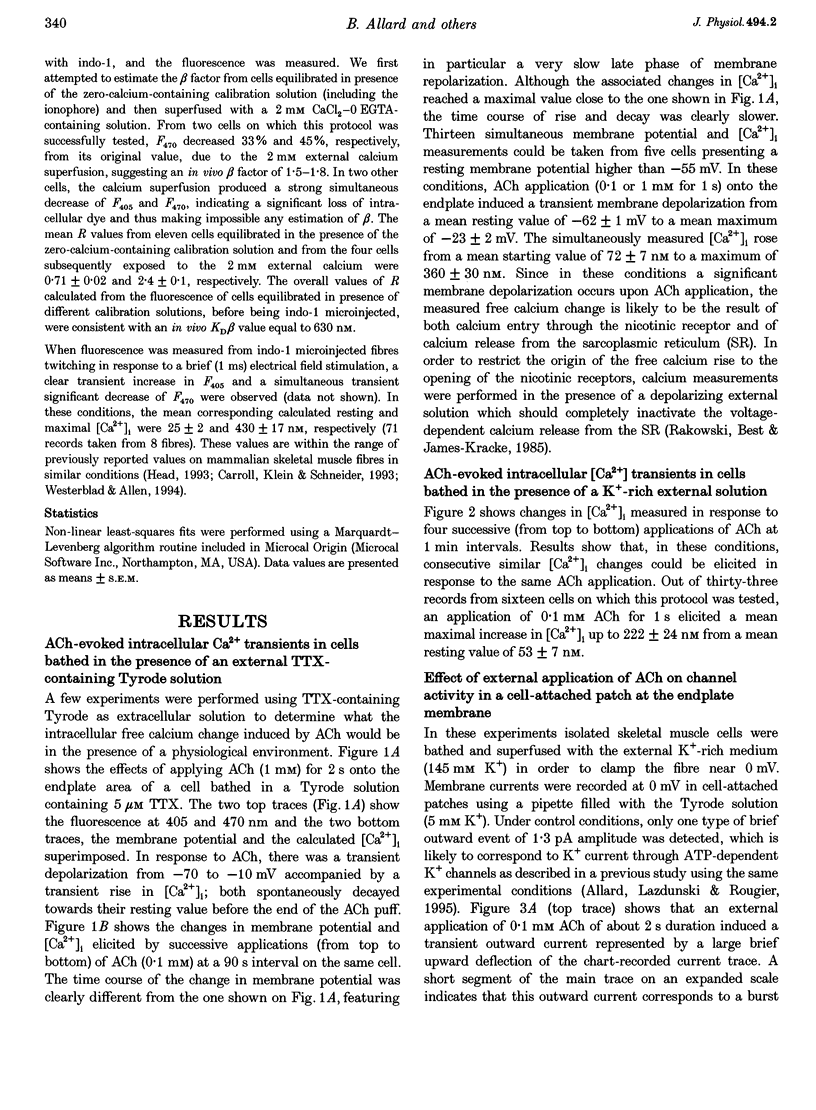
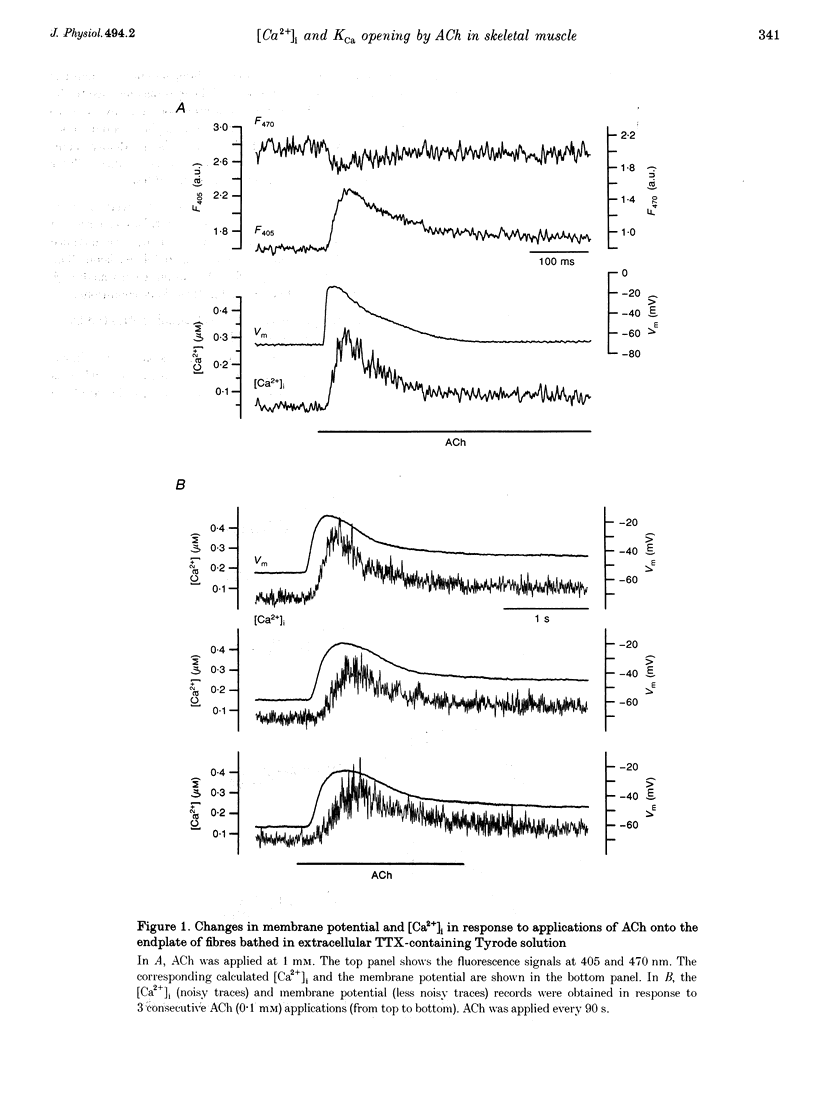
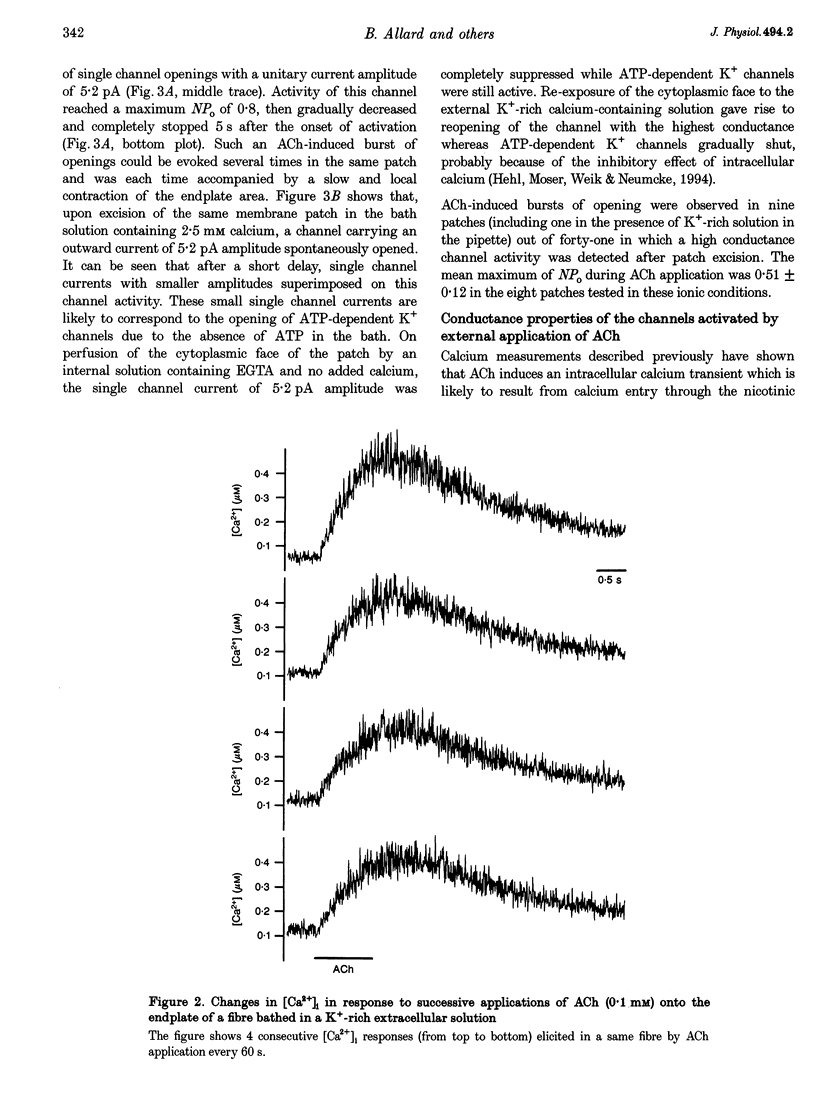
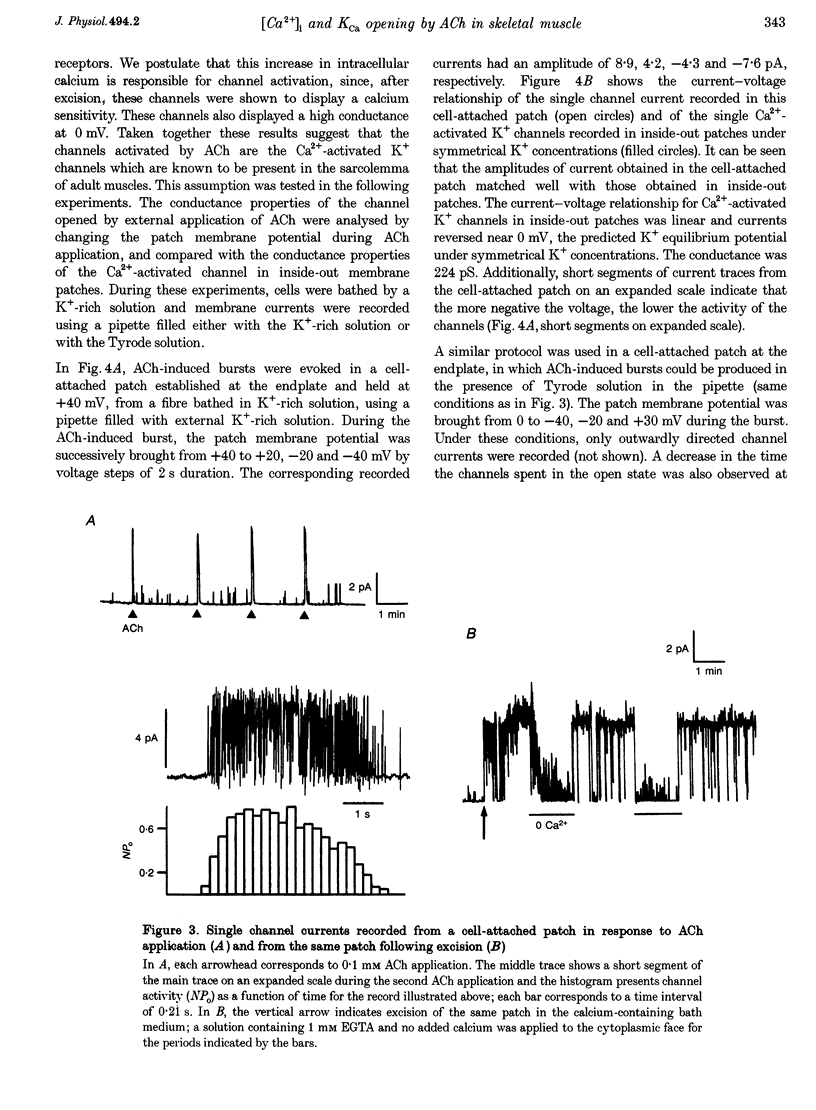
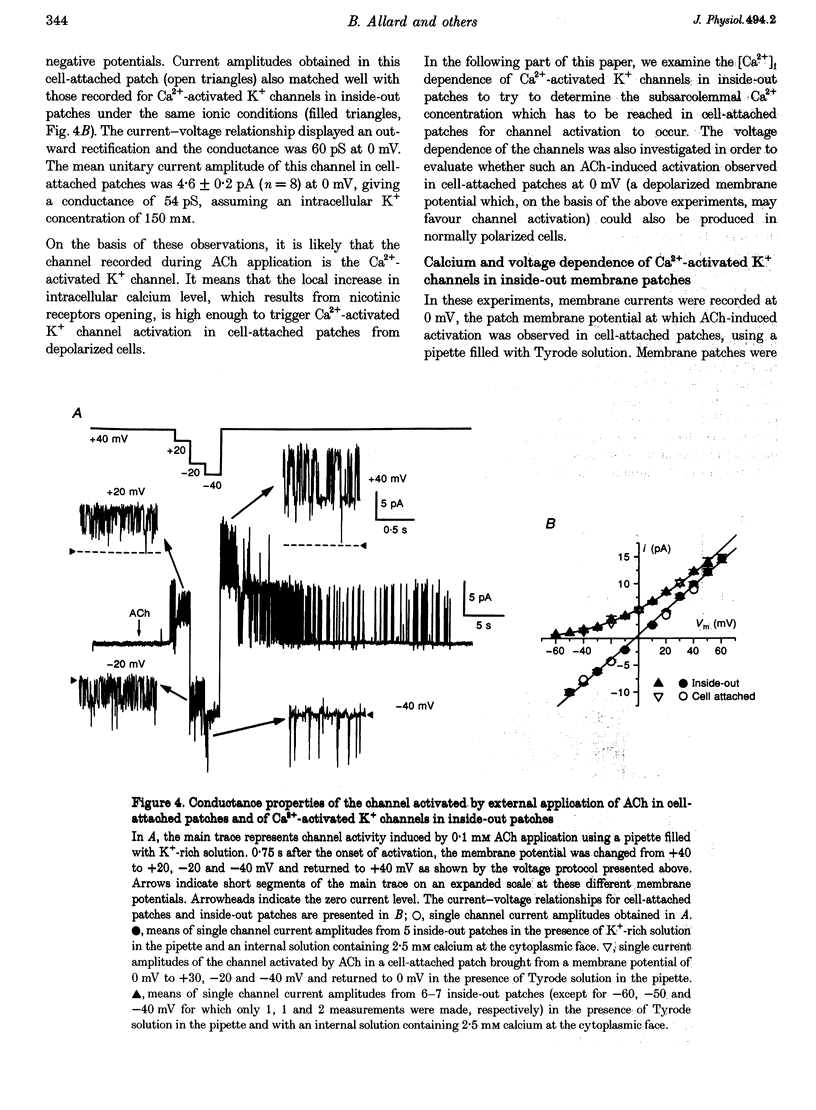
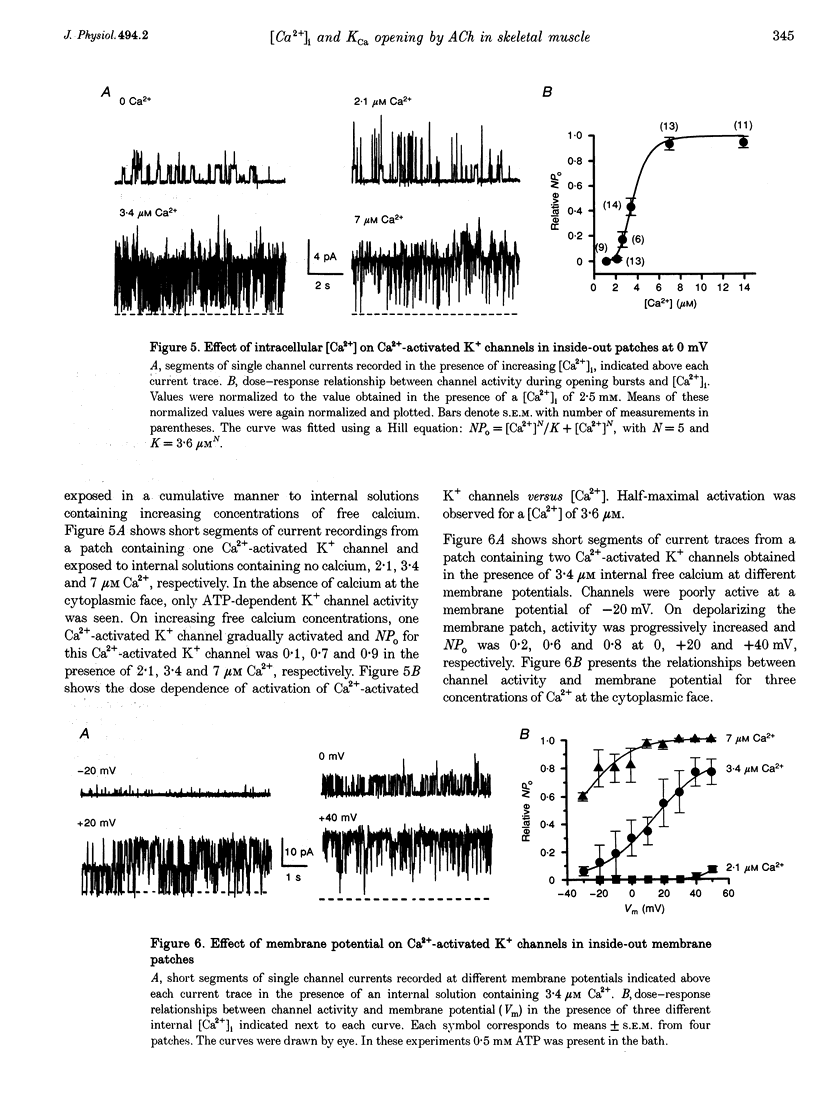
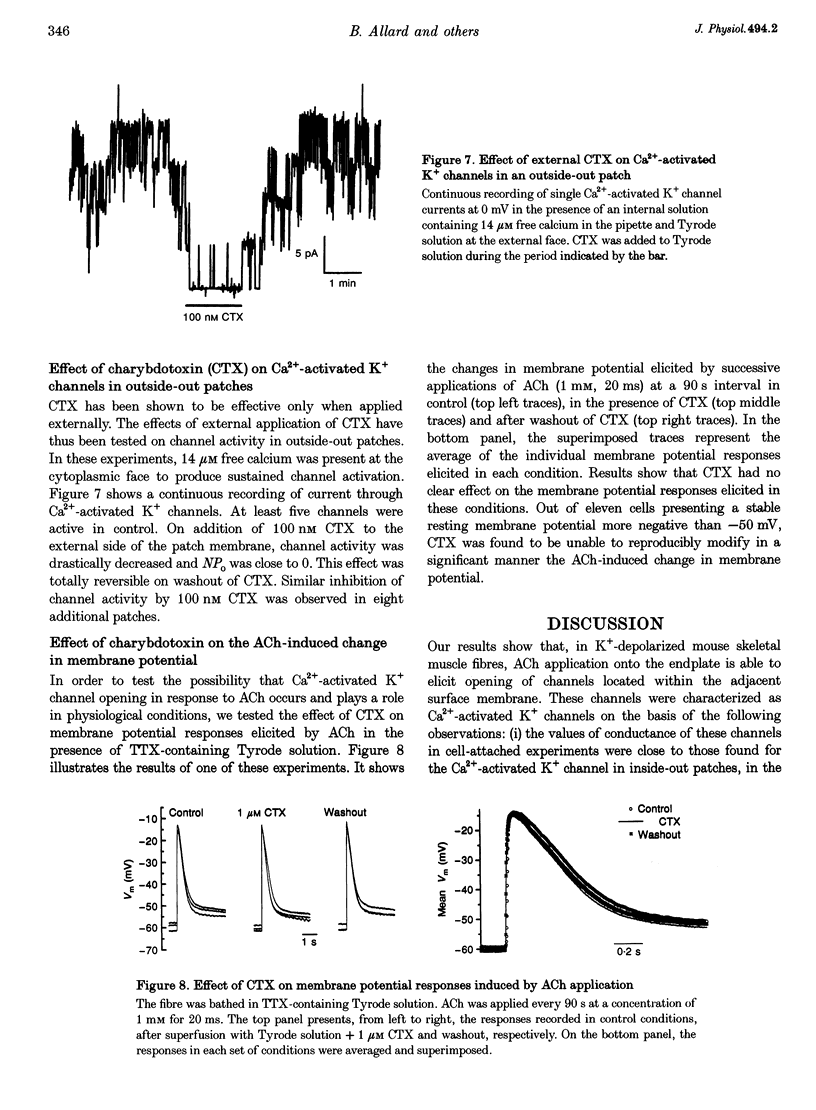
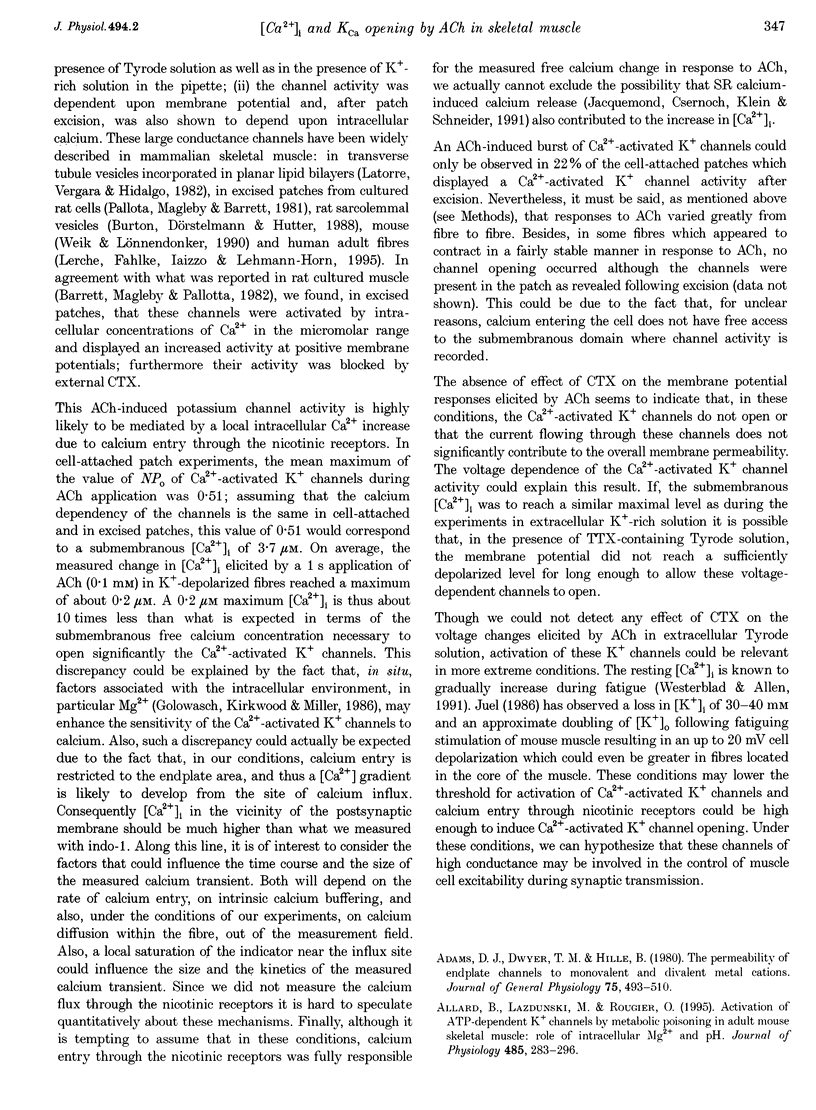
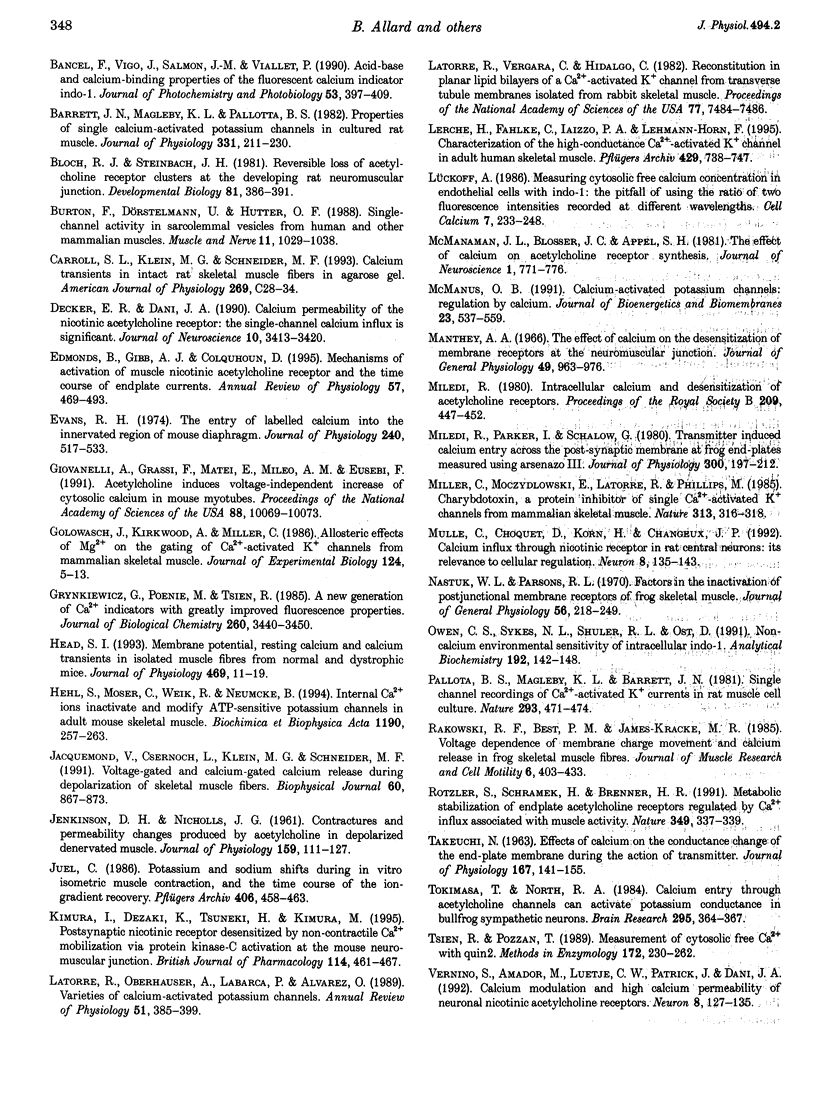
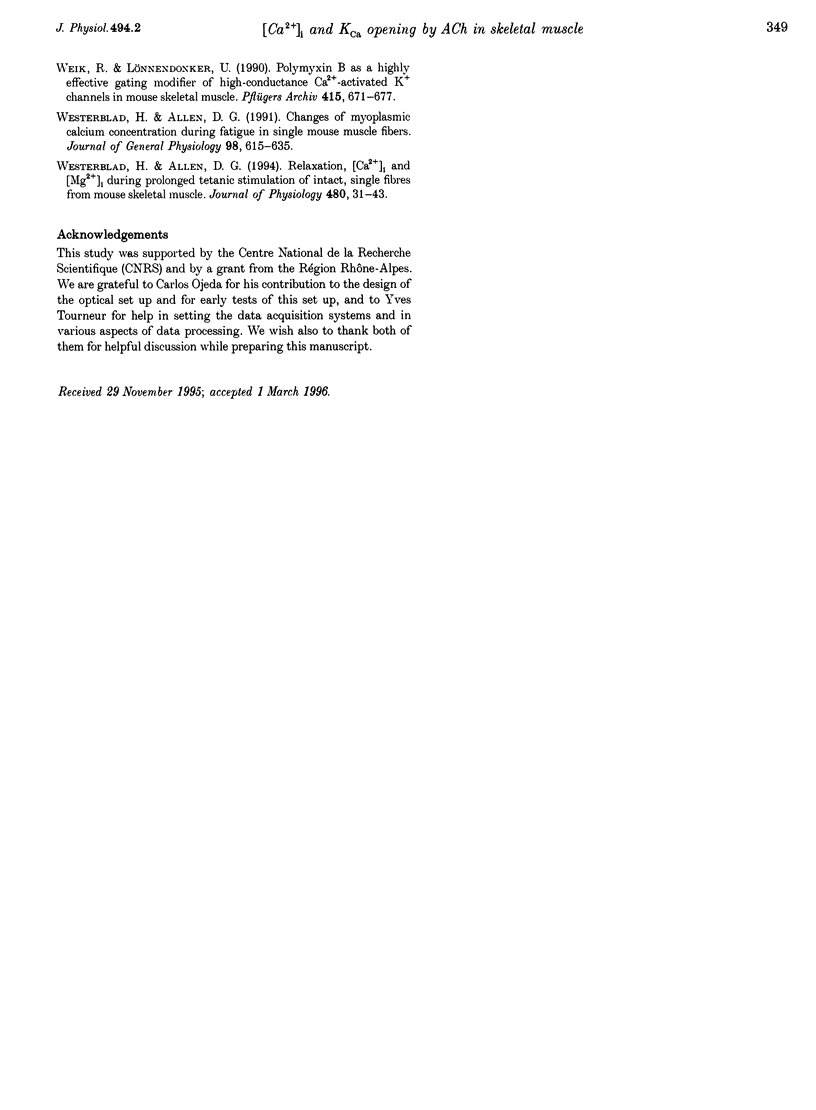
Selected References
These references are in PubMed. This may not be the complete list of references from this article.
- Adams D. J., Dwyer T. M., Hille B. The permeability of endplate channels to monovalent and divalent metal cations. J Gen Physiol. 1980 May;75(5):493–510. doi: 10.1085/jgp.75.5.493. [DOI] [PMC free article] [PubMed] [Google Scholar]
- Allard B., Lazdunski M., Rougier O. Activation of ATP-dependent K+ channels by metabolic poisoning in adult mouse skeletal muscle: role of intracellular Mg(2+) and pH. J Physiol. 1995 Jun 1;485(Pt 2):283–296. doi: 10.1113/jphysiol.1995.sp020730. [DOI] [PMC free article] [PubMed] [Google Scholar]
- Barrett J. N., Magleby K. L., Pallotta B. S. Properties of single calcium-activated potassium channels in cultured rat muscle. J Physiol. 1982 Oct;331:211–230. doi: 10.1113/jphysiol.1982.sp014370. [DOI] [PMC free article] [PubMed] [Google Scholar]
- Bloch R. J., Steinbach J. H. Reversible loss of acetylcholine receptor clusters at the developing rat neuromuscular junction. Dev Biol. 1981 Jan 30;81(2):386–391. doi: 10.1016/0012-1606(81)90305-5. [DOI] [PubMed] [Google Scholar]
- Burton F., Dörstelmann U., Hutter O. F. Single-channel activity in sarcolemmal vesicles from human and other mammalian muscles. Muscle Nerve. 1988 Oct;11(10):1029–1038. doi: 10.1002/mus.880111004. [DOI] [PubMed] [Google Scholar]
- Carroll S. L., Klein M. G., Schneider M. F. Calcium transients in intact rat skeletal muscle fibers in agarose gel. Am J Physiol. 1995 Jul;269(1 Pt 1):C28–C34. doi: 10.1152/ajpcell.1995.269.1.C28. [DOI] [PubMed] [Google Scholar]
- Decker E. R., Dani J. A. Calcium permeability of the nicotinic acetylcholine receptor: the single-channel calcium influx is significant. J Neurosci. 1990 Oct;10(10):3413–3420. doi: 10.1523/JNEUROSCI.10-10-03413.1990. [DOI] [PMC free article] [PubMed] [Google Scholar]
- Edmonds B., Gibb A. J., Colquhoun D. Mechanisms of activation of muscle nicotinic acetylcholine receptors and the time course of endplate currents. Annu Rev Physiol. 1995;57:469–493. doi: 10.1146/annurev.ph.57.030195.002345. [DOI] [PubMed] [Google Scholar]
- Evans R. H. The entry of labelled calcium into the innervated region of the mouse diaphragm muscle. J Physiol. 1974 Aug;240(3):517–533. doi: 10.1113/jphysiol.1974.sp010621. [DOI] [PMC free article] [PubMed] [Google Scholar]
- Giovannelli A., Grassi F., Mattei E., Mileo A. M., Eusebi F., Giovanelli A. Acetylcholine induces voltage-independent increase of cytosolic calcium in mouse myotubes. Proc Natl Acad Sci U S A. 1991 Nov 15;88(22):10069–10073. doi: 10.1073/pnas.88.22.10069. [DOI] [PMC free article] [PubMed] [Google Scholar]
- Golowasch J., Kirkwood A., Miller C. Allosteric effects of Mg2+ on the gating of Ca2+-activated K+ channels from mammalian skeletal muscle. J Exp Biol. 1986 Sep;124:5–13. doi: 10.1242/jeb.124.1.5. [DOI] [PubMed] [Google Scholar]
- Grynkiewicz G., Poenie M., Tsien R. Y. A new generation of Ca2+ indicators with greatly improved fluorescence properties. J Biol Chem. 1985 Mar 25;260(6):3440–3450. [PubMed] [Google Scholar]
- Head S. I. Membrane potential, resting calcium and calcium transients in isolated muscle fibres from normal and dystrophic mice. J Physiol. 1993 Sep;469:11–19. doi: 10.1113/jphysiol.1993.sp019801. [DOI] [PMC free article] [PubMed] [Google Scholar]
- Hehl S., Moser C., Weik R., Neumcke B. Internal Ca2+ ions inactivate and modify ATP-sensitive potassium channels in adult mouse skeletal muscle. Biochim Biophys Acta. 1994 Mar 23;1190(2):257–263. doi: 10.1016/0005-2736(94)90082-5. [DOI] [PubMed] [Google Scholar]
- JENKINSON D. H., NICHOLLS J. G. Contractures and permeability changes produced by acetylcholine in depolarized denervated muscle. J Physiol. 1961 Nov;159:111–127. doi: 10.1113/jphysiol.1961.sp006796. [DOI] [PMC free article] [PubMed] [Google Scholar]
- Jacquemond V., Csernoch L., Klein M. G., Schneider M. F. Voltage-gated and calcium-gated calcium release during depolarization of skeletal muscle fibers. Biophys J. 1991 Oct;60(4):867–873. doi: 10.1016/S0006-3495(91)82120-8. [DOI] [PMC free article] [PubMed] [Google Scholar]
- Juel C. Potassium and sodium shifts during in vitro isometric muscle contraction, and the time course of the ion-gradient recovery. Pflugers Arch. 1986 May;406(5):458–463. doi: 10.1007/BF00583367. [DOI] [PubMed] [Google Scholar]
- Kimura I., Dezaki K., Tsuneki H., Kimura M. Postsynaptic nicotinic receptor desensitized by non-contractile Ca2+ mobilization via protein kinase-C activation at the mouse neuromuscular junction. Br J Pharmacol. 1995 Jan;114(2):461–467. doi: 10.1111/j.1476-5381.1995.tb13249.x. [DOI] [PMC free article] [PubMed] [Google Scholar]
- Latorre R., Oberhauser A., Labarca P., Alvarez O. Varieties of calcium-activated potassium channels. Annu Rev Physiol. 1989;51:385–399. doi: 10.1146/annurev.ph.51.030189.002125. [DOI] [PubMed] [Google Scholar]
- Lerche H., Fahlke C., Iaizzo P. A., Lehmann-Horn F. Characterization of the high-conductance Ca(2+)-activated K+ channel in adult human skeletal muscle. Pflugers Arch. 1995 Mar;429(5):738–747. doi: 10.1007/BF00373997. [DOI] [PubMed] [Google Scholar]
- Lückhoff A. Measuring cytosolic free calcium concentration in endothelial cells with indo-1: the pitfall of using the ratio of two fluorescence intensities recorded at different wavelengths. Cell Calcium. 1986 Aug;7(4):233–248. doi: 10.1016/0143-4160(86)90003-5. [DOI] [PubMed] [Google Scholar]
- Manthey A. A. The effect of calcium on the desensitization of membrane receptors at the neuromuscular junction. J Gen Physiol. 1966 May;49(5):963–976. doi: 10.1085/jgp.49.5.963. [DOI] [PMC free article] [PubMed] [Google Scholar]
- Miledi R. Intracellular calcium and desensitization of acetylcholine receptors. Proc R Soc Lond B Biol Sci. 1980 Sep 26;209(1176):447–452. doi: 10.1098/rspb.1980.0106. [DOI] [PubMed] [Google Scholar]
- Miller C., Moczydlowski E., Latorre R., Phillips M. Charybdotoxin, a protein inhibitor of single Ca2+-activated K+ channels from mammalian skeletal muscle. Nature. 1985 Jan 24;313(6000):316–318. doi: 10.1038/313316a0. [DOI] [PubMed] [Google Scholar]
- Mulle C., Choquet D., Korn H., Changeux J. P. Calcium influx through nicotinic receptor in rat central neurons: its relevance to cellular regulation. Neuron. 1992 Jan;8(1):135–143. doi: 10.1016/0896-6273(92)90115-t. [DOI] [PubMed] [Google Scholar]
- Nastuk W. L., Parsons R. L. Factors in the inactivation of postjunctional membrane receptors of frog skeletal muscle. J Gen Physiol. 1970 Aug;56(2):218–249. doi: 10.1085/jgp.56.2.218. [DOI] [PMC free article] [PubMed] [Google Scholar]
- Owen C. S., Sykes N. L., Shuler R. L., Ost D. Non-calcium environmental sensitivity of intracellular Indo-1. Anal Biochem. 1991 Jan;192(1):142–148. doi: 10.1016/0003-2697(91)90199-4. [DOI] [PubMed] [Google Scholar]
- Pallotta B. S., Magleby K. L., Barrett J. N. Single channel recordings of Ca2+-activated K+ currents in rat muscle cell culture. Nature. 1981 Oct 8;293(5832):471–474. doi: 10.1038/293471a0. [DOI] [PubMed] [Google Scholar]
- Rakowski R. F., Best P. M., James-Kracke M. R. Voltage dependence of membrane charge movement and calcium release in frog skeletal muscle fibres. J Muscle Res Cell Motil. 1985 Aug;6(4):403–433. doi: 10.1007/BF00712580. [DOI] [PubMed] [Google Scholar]
- Rotzler S., Schramek H., Brenner H. R. Metabolic stabilization of endplate acetylcholine receptors regulated by Ca2+ influx associated with muscle activity. Nature. 1991 Jan 24;349(6307):337–339. doi: 10.1038/349337a0. [DOI] [PubMed] [Google Scholar]
- TAKEUCHI N. Effects of calcium on the conductance change of the end-plate membrane during the action of transmitter. J Physiol. 1963 Jun;167:141–155. doi: 10.1113/jphysiol.1963.sp007137. [DOI] [PMC free article] [PubMed] [Google Scholar]
- Tokimasa T., North R. A. Calcium entry through acetylcholine-channels can activate potassium conductance in bullfrog sympathetic neurons. Brain Res. 1984 Mar 19;295(2):364–367. doi: 10.1016/0006-8993(84)90987-9. [DOI] [PubMed] [Google Scholar]
- Tsien R., Pozzan T. Measurement of cytosolic free Ca2+ with quin2. Methods Enzymol. 1989;172:230–262. doi: 10.1016/s0076-6879(89)72017-6. [DOI] [PubMed] [Google Scholar]
- Vernino S., Amador M., Luetje C. W., Patrick J., Dani J. A. Calcium modulation and high calcium permeability of neuronal nicotinic acetylcholine receptors. Neuron. 1992 Jan;8(1):127–134. doi: 10.1016/0896-6273(92)90114-s. [DOI] [PubMed] [Google Scholar]
- Weik R., Lönnendonker U. Polymyxin B as a highly effective gating modifier of high-conductance Ca2(+)-activated K+ channels in mouse skeletal muscle. Pflugers Arch. 1990 Mar;415(6):671–677. doi: 10.1007/BF02584004. [DOI] [PubMed] [Google Scholar]
- Westerblad H., Allen D. G. Changes of myoplasmic calcium concentration during fatigue in single mouse muscle fibers. J Gen Physiol. 1991 Sep;98(3):615–635. doi: 10.1085/jgp.98.3.615. [DOI] [PMC free article] [PubMed] [Google Scholar]
- Westerblad H., Allen D. G. Relaxation, [Ca2+]i and [Mg2+]i during prolonged tetanic stimulation of intact, single fibres from mouse skeletal muscle. J Physiol. 1994 Oct 1;480(Pt 1):31–43. doi: 10.1113/jphysiol.1994.sp020338. [DOI] [PMC free article] [PubMed] [Google Scholar]