Abstract
1. In this study, the efficiency of energy conversion in skeletal muscles from the mouse was determined before and after a series of contractions that produced a moderate level of fatigue. 2. Initial mechanical efficiency was defined as the ratio of mechanical power output to the rate of initial enthalpy output. The rate of initial enthalpy output was the sum of the power output and rate of initial heat output. Heat output was measured using a thermopile with high temporal resolution. 3. Experiments were performed in vitro (25 degrees C) using bundles of fibres from fast-twitch extensor digitorum longus (EDL) and slow-twitch soleus muscles from mice. Muscles were fatigued using a series of thirty isometric tetani. Initial mechanical efficiency was determined before and again immediately after the fatigue protocol using a series of isovelocity contractions at shortening velocities between 0 and the maximum shortening velocity (Vmax). Efficiency was determined over the second half of the shortening at each velocity. 4. The fatigue protocol significantly reduced maximum isometric force Vmax, maximum power output and flattened the force-velocity curve. The magnitude of these effects was greater in EDL muscle than soleus muscle. In unfatigued muscle, the maximum mechanical efficiency was 0.333 for EDL muscles and 0.425 for soleus muscles. In both muscle types, the fatiguing contractions caused maximum efficiency to decrease. The magnitude of the decrease was 15% of the pre-fatigue value in EDL and 9% in soleus. 5. In a separate series of experiments, the effect of the fatigue protocol on the partitioning of energy expenditure between crossbridge and non-crossbridge sources was determined. Data from these experiments enabled the efficiency of energy conversion by the crossbridges to be estimated. It was concluded that the decrease in initial mechanical efficiency reflected a decrease in the efficiency of energy conversion by the crossbridges.
Full text
PDF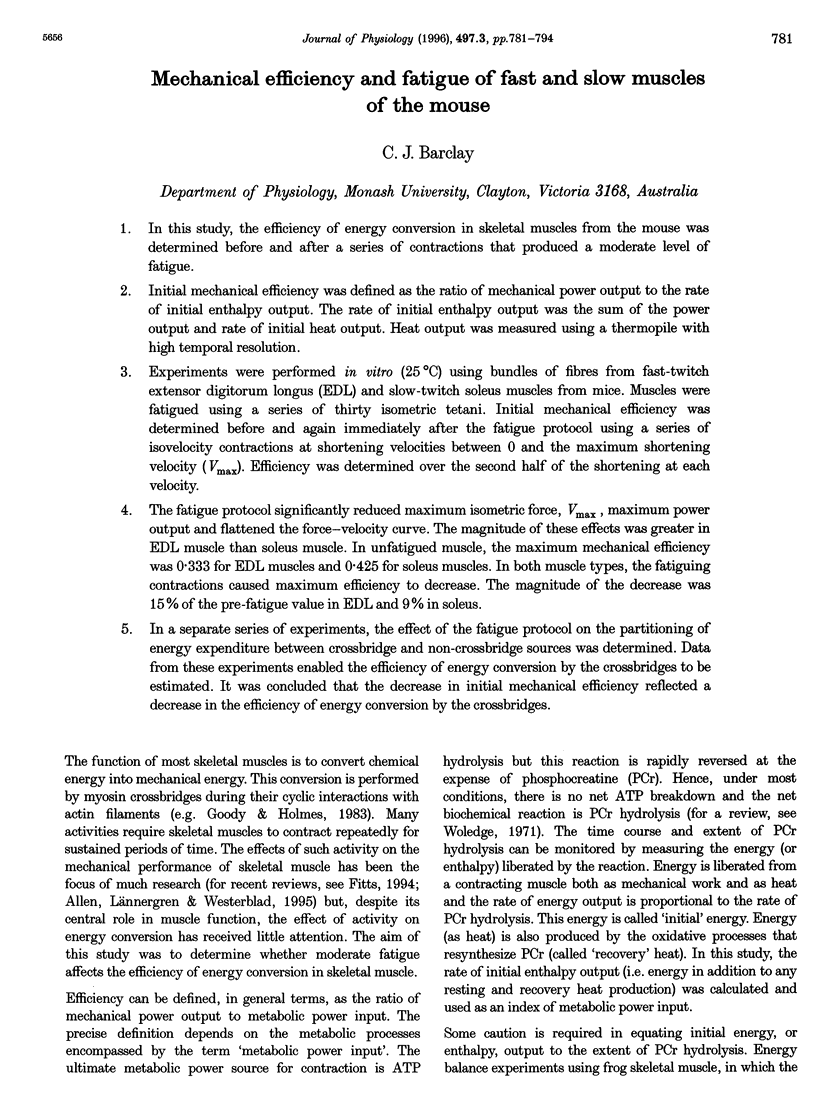
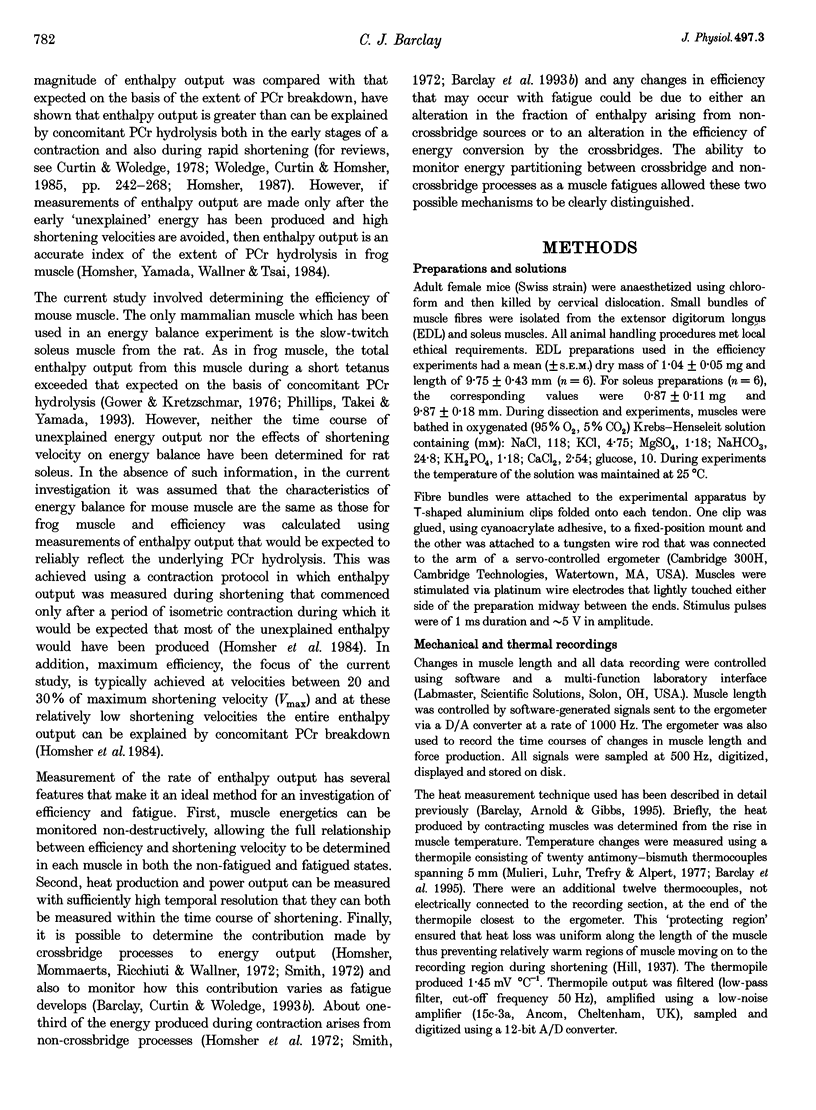
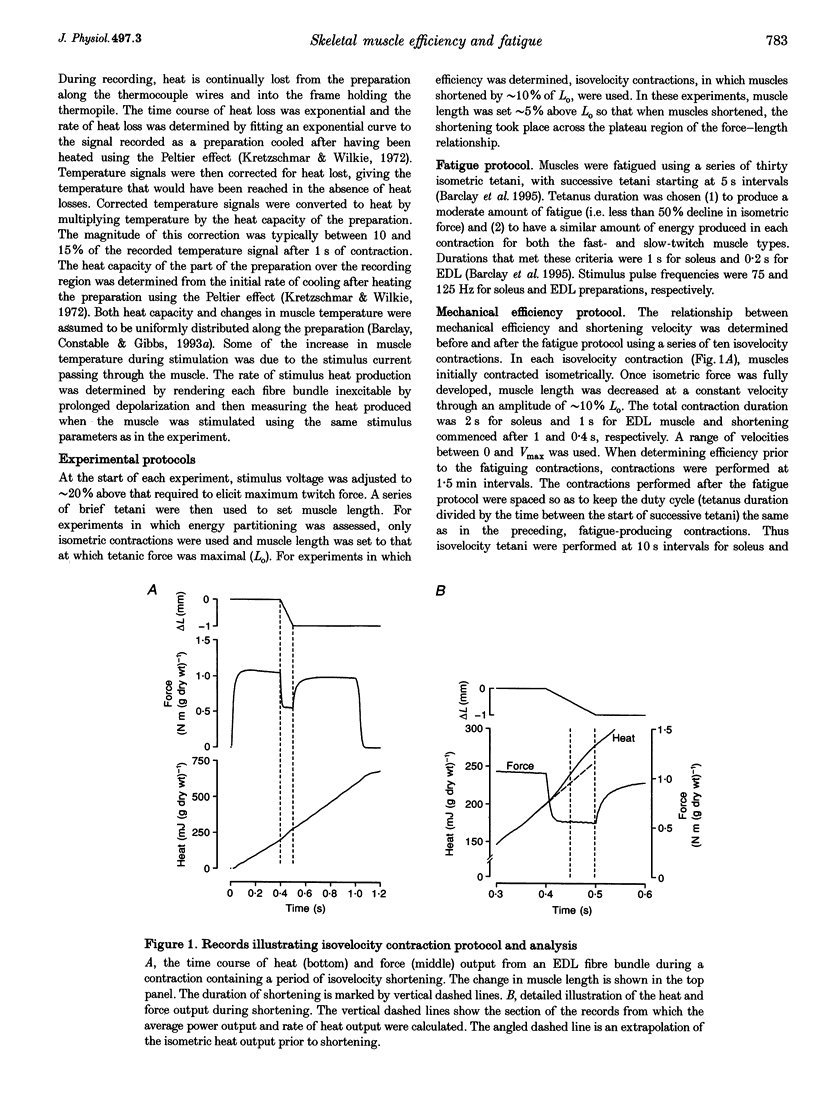
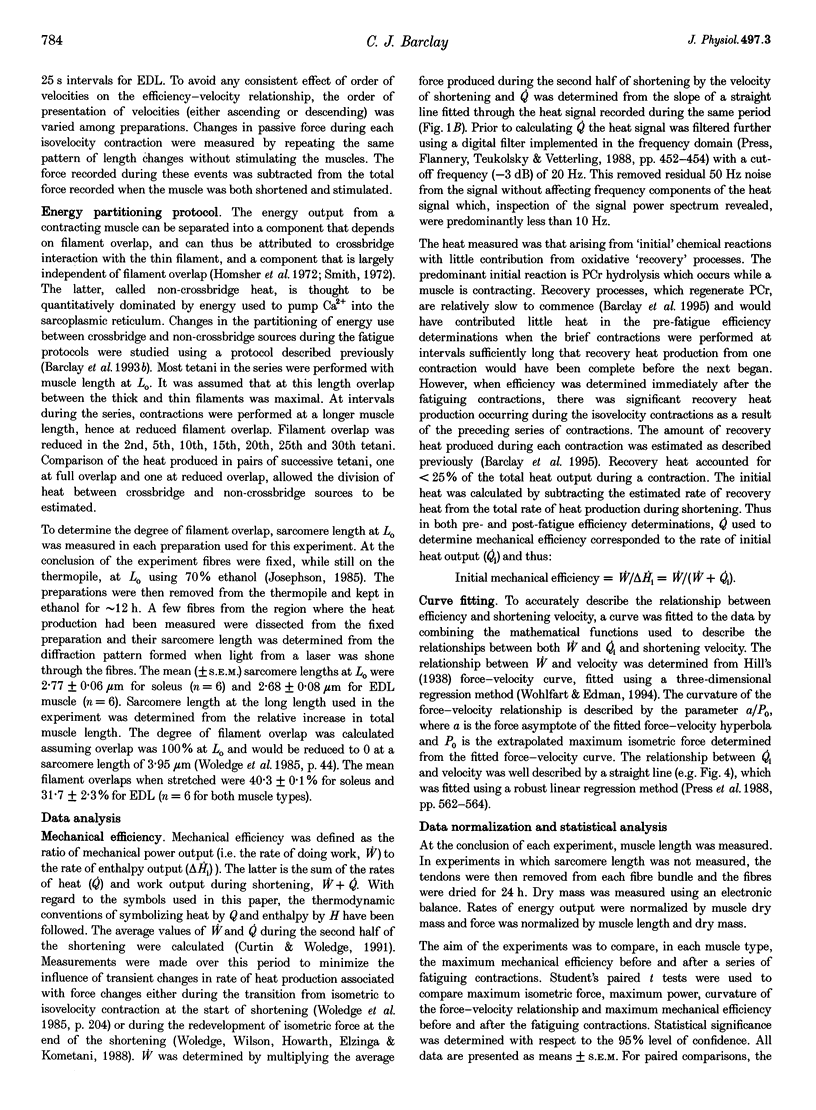
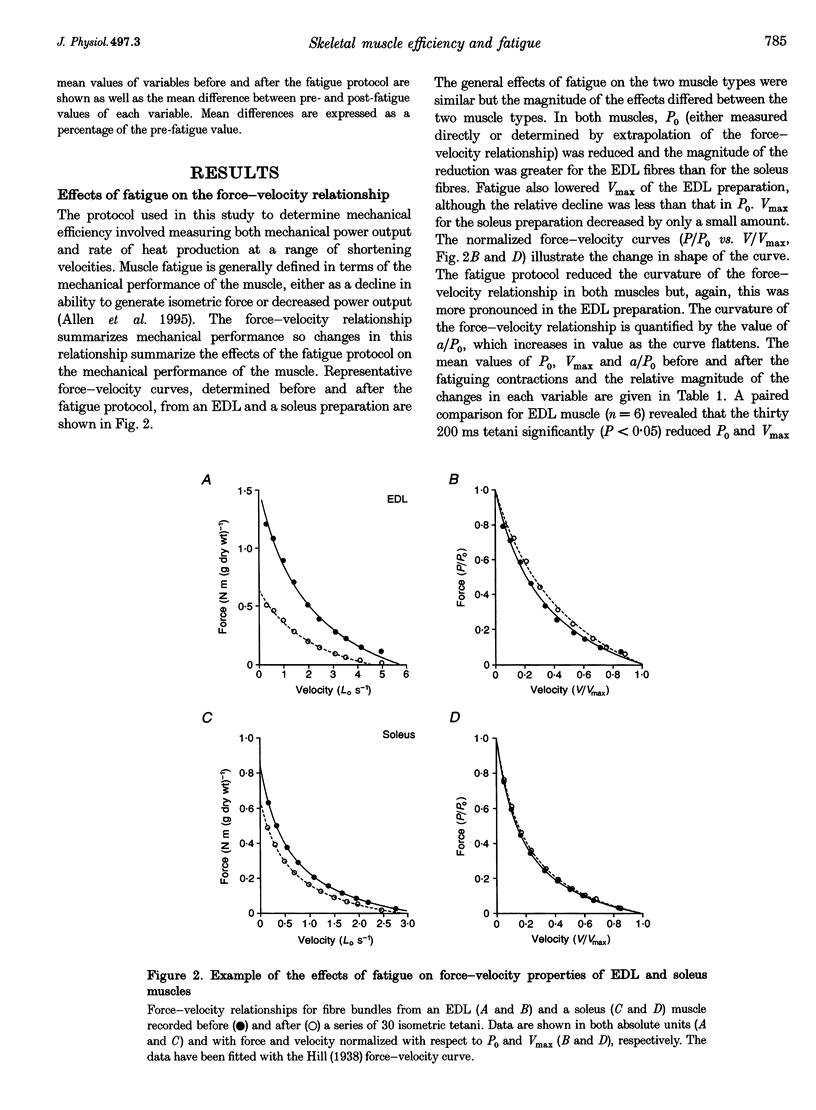
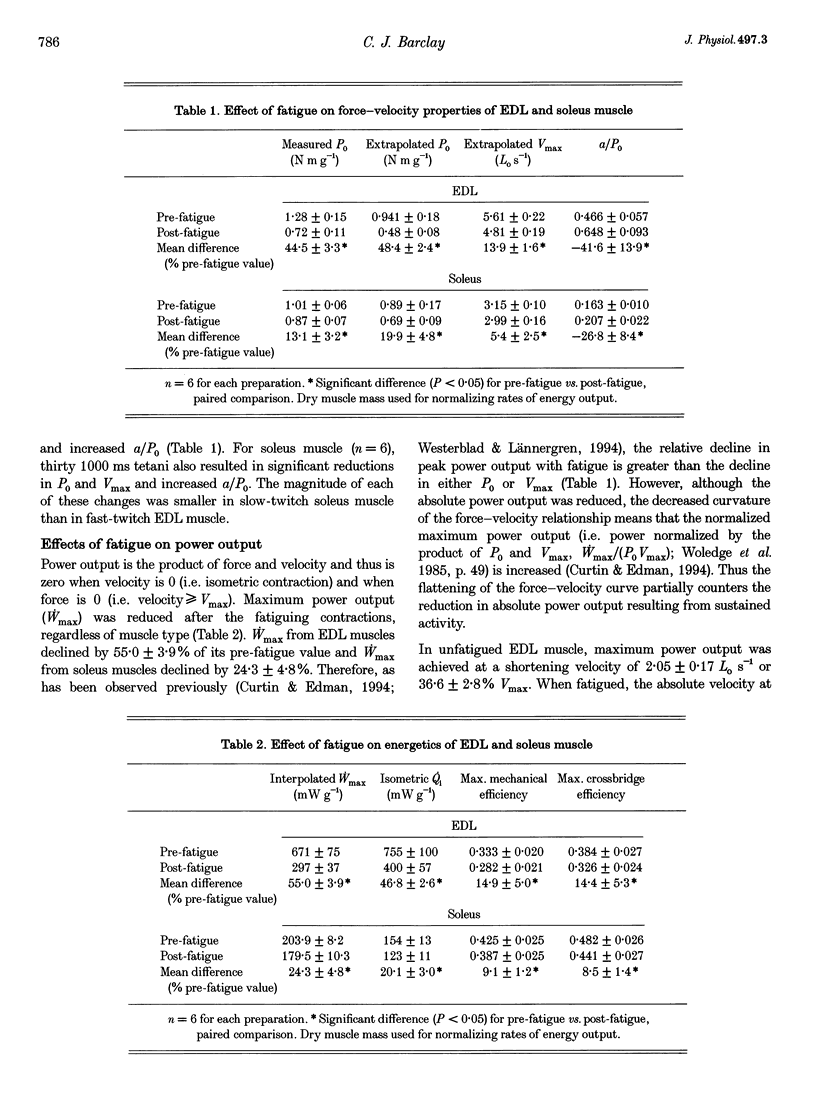
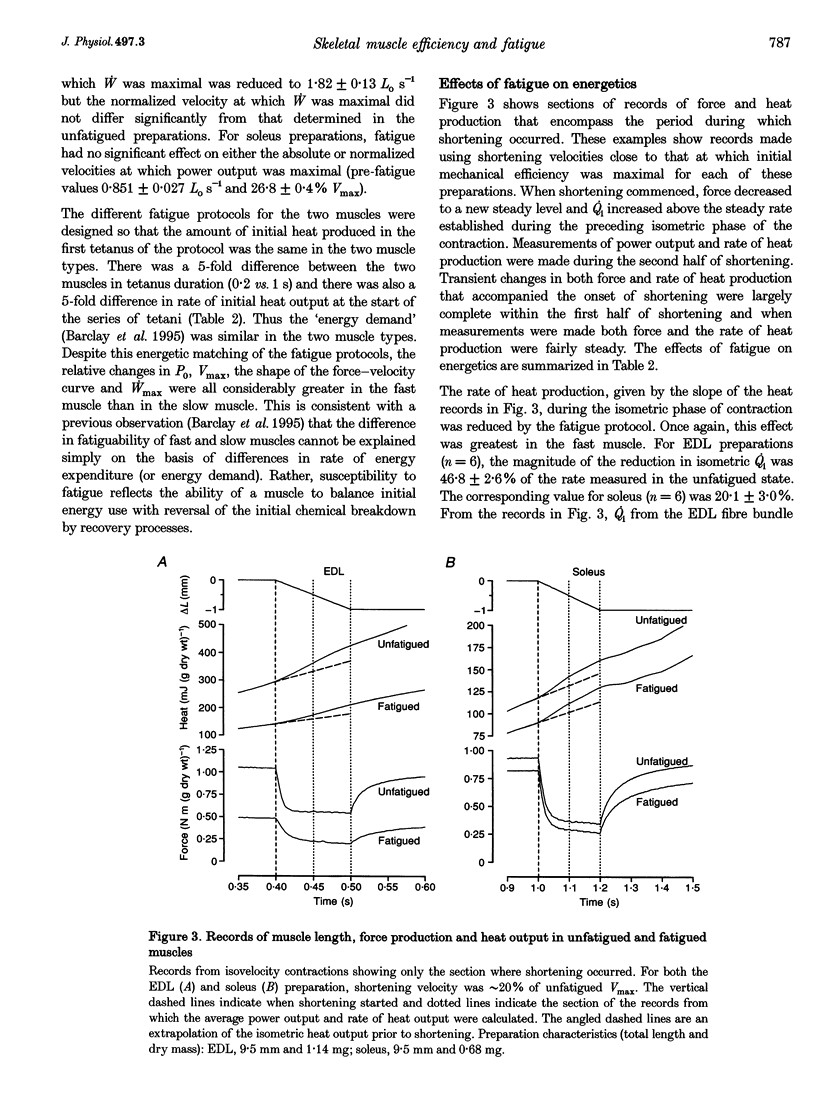
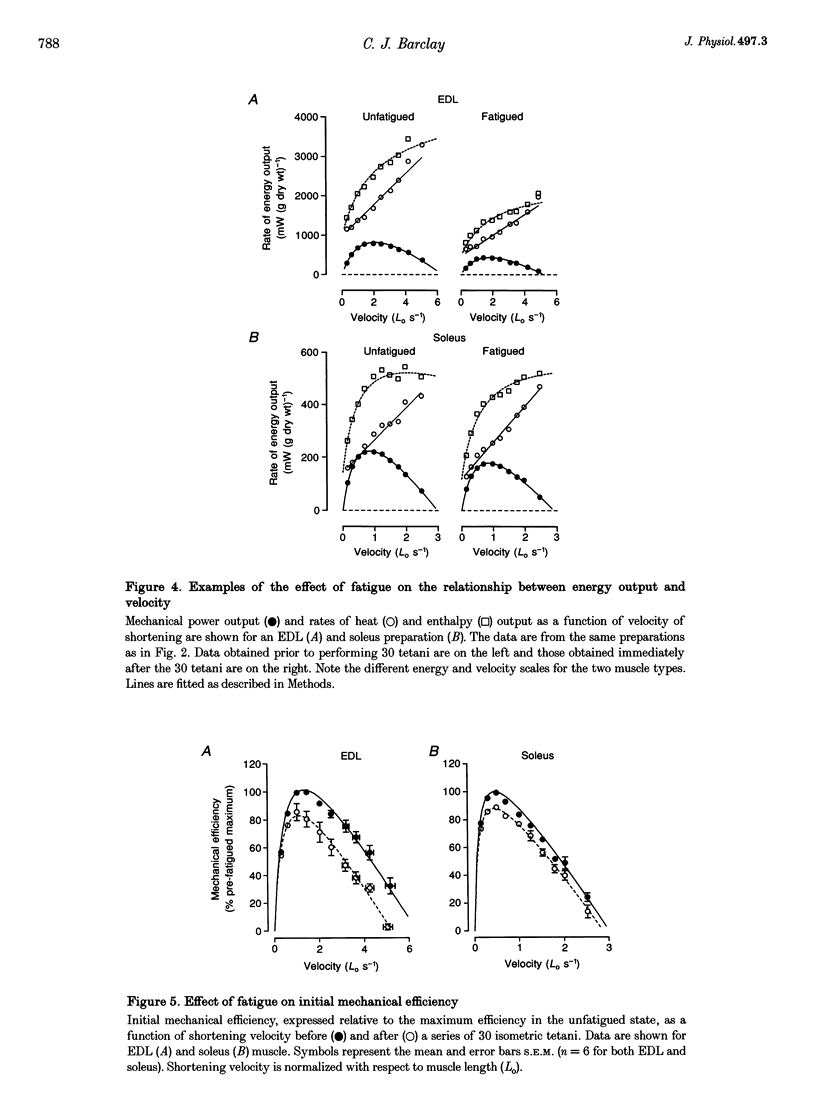
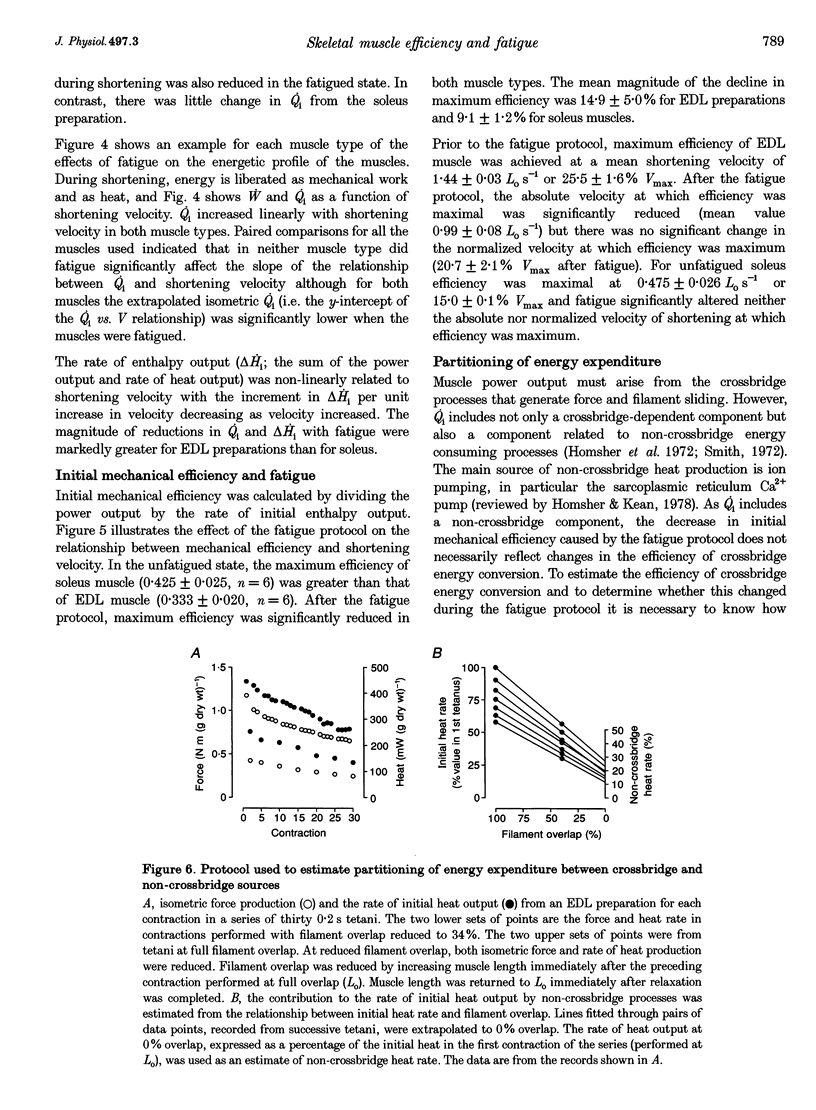
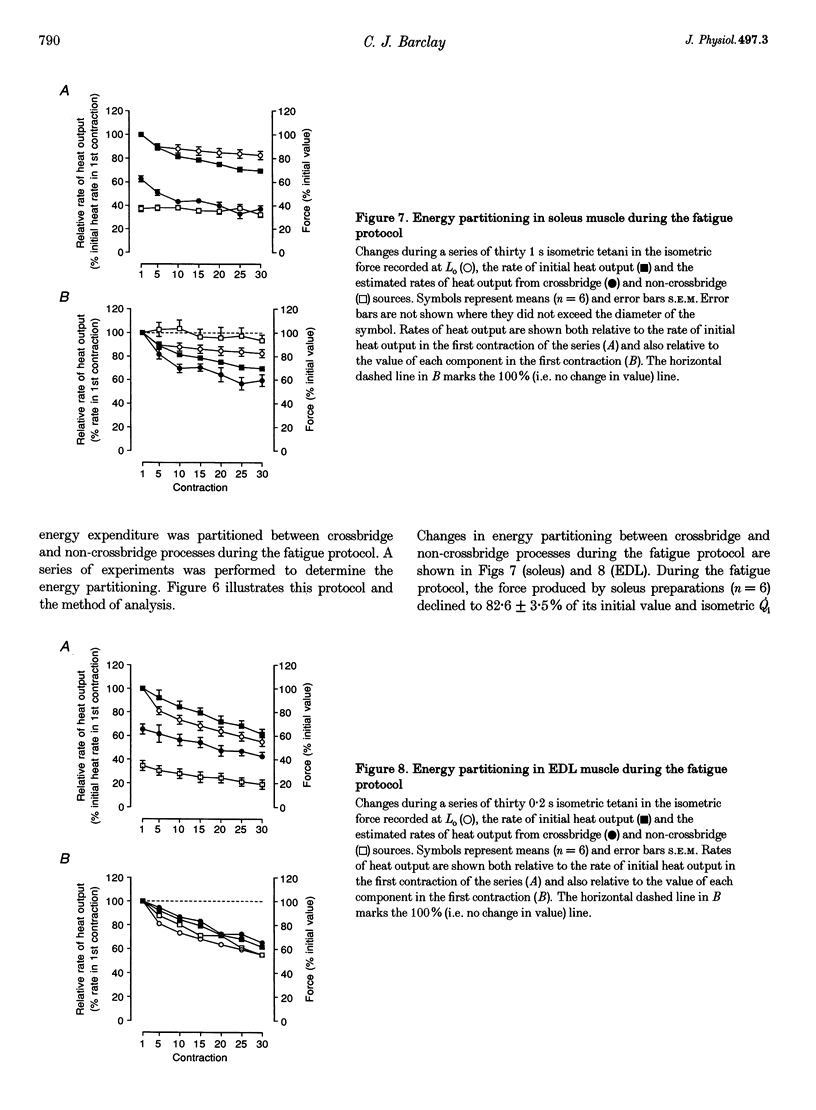
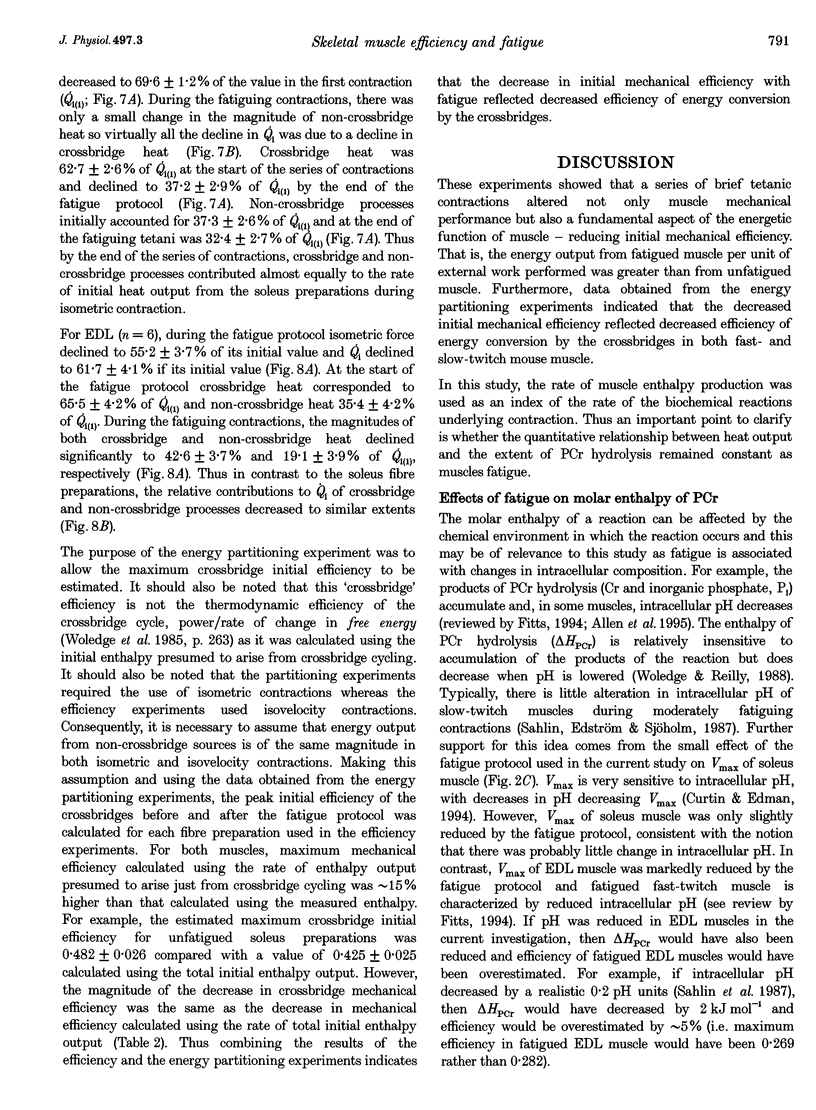
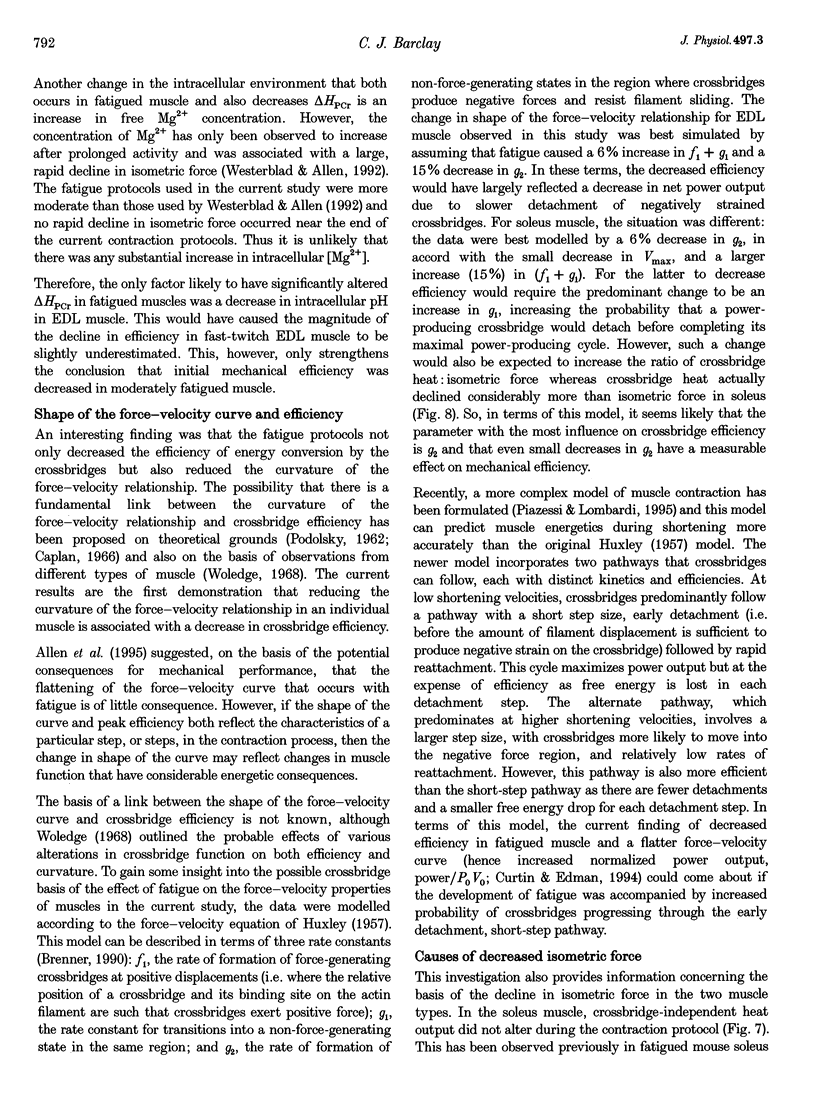
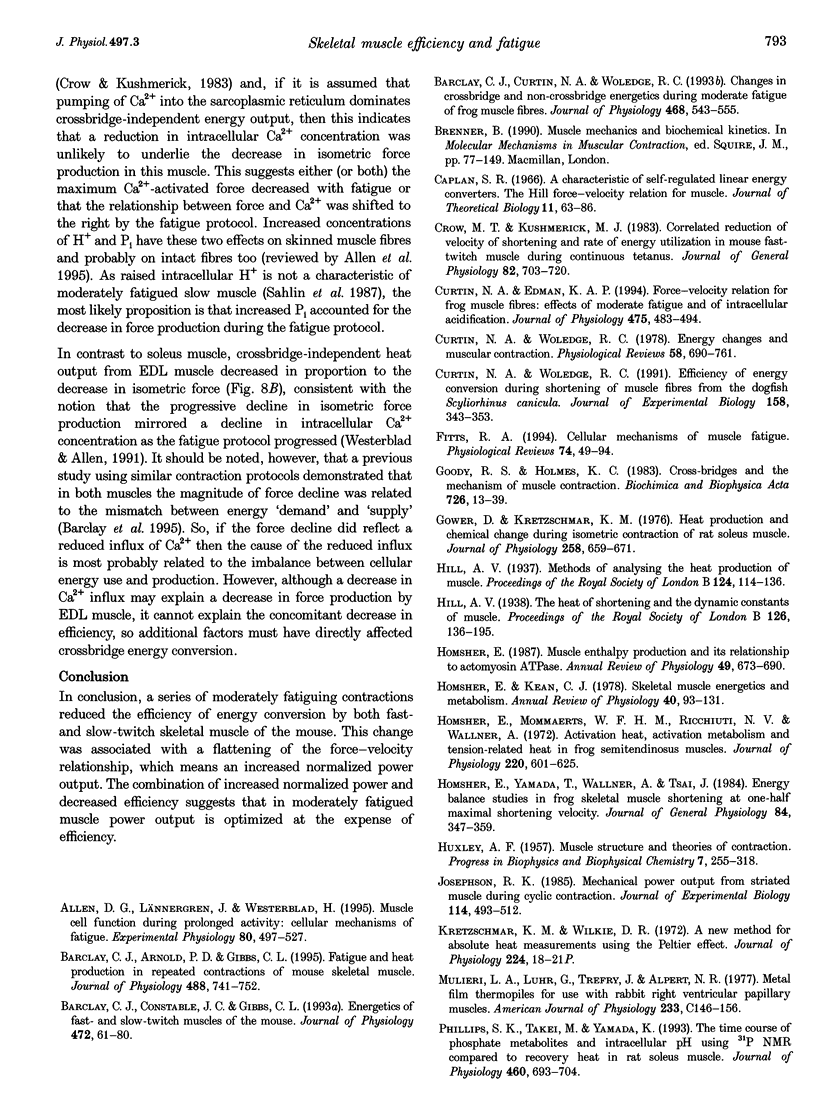
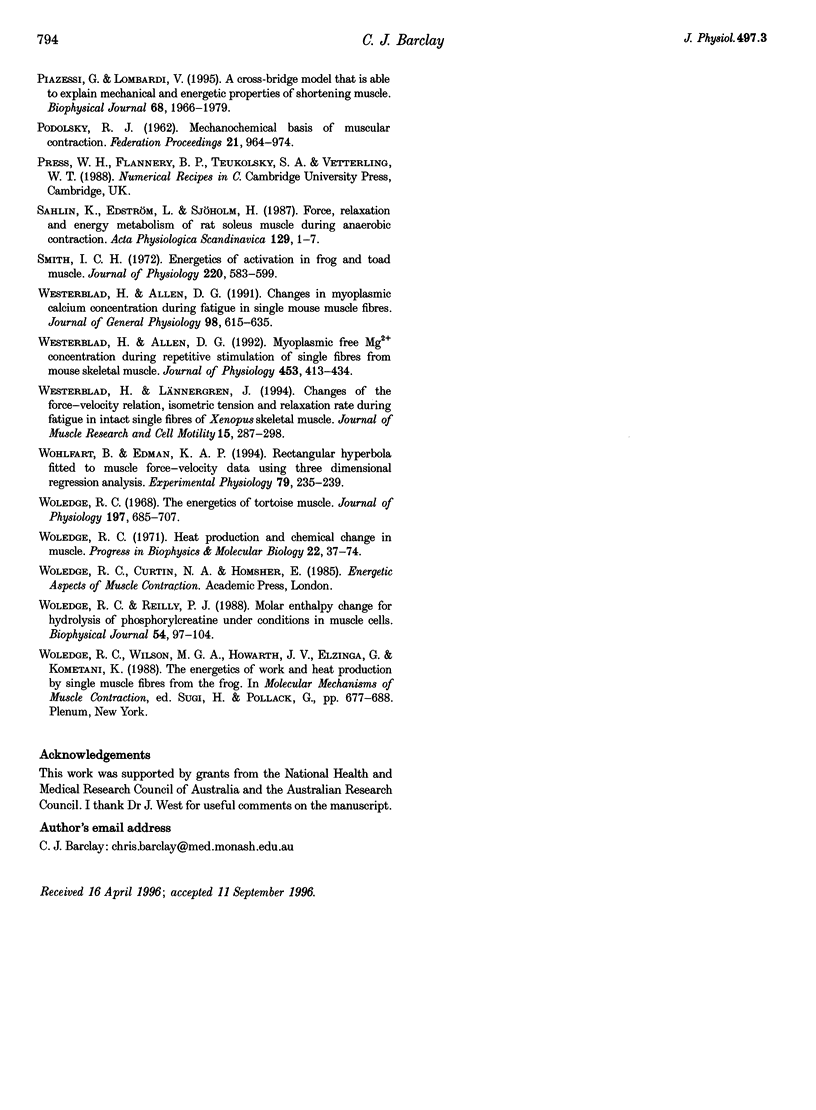
Selected References
These references are in PubMed. This may not be the complete list of references from this article.
- Allen D. G., Lännergren J., Westerblad H. Muscle cell function during prolonged activity: cellular mechanisms of fatigue. Exp Physiol. 1995 Jul;80(4):497–527. doi: 10.1113/expphysiol.1995.sp003864. [DOI] [PubMed] [Google Scholar]
- Barclay C. J., Arnold P. D., Gibbs C. L. Fatigue and heat production in repeated contractions of mouse skeletal muscle. J Physiol. 1995 Nov 1;488(Pt 3):741–752. doi: 10.1113/jphysiol.1995.sp021005. [DOI] [PMC free article] [PubMed] [Google Scholar]
- Barclay C. J., Constable J. K., Gibbs C. L. Energetics of fast- and slow-twitch muscles of the mouse. J Physiol. 1993 Dec;472:61–80. doi: 10.1113/jphysiol.1993.sp019937. [DOI] [PMC free article] [PubMed] [Google Scholar]
- Barclay C. J., Curtin N. A., Woledge R. C. Changes in crossbridge and non-crossbridge energetics during moderate fatigue of frog muscle fibres. J Physiol. 1993 Aug;468:543–556. doi: 10.1113/jphysiol.1993.sp019787. [DOI] [PMC free article] [PubMed] [Google Scholar]
- Caplan S. R. A characteristic of self-regulated linear energy converters. The Hill force-velocity relation for muscle. J Theor Biol. 1966 May;11(1):63–86. doi: 10.1016/0022-5193(66)90040-3. [DOI] [PubMed] [Google Scholar]
- Crow M. T., Kushmerick M. J. Correlated reduction of velocity of shortening and the rate of energy utilization in mouse fast-twitch muscle during a continuous tetanus. J Gen Physiol. 1983 Nov;82(5):703–720. doi: 10.1085/jgp.82.5.703. [DOI] [PMC free article] [PubMed] [Google Scholar]
- Curtin N. A., Edman K. A. Force-velocity relation for frog muscle fibres: effects of moderate fatigue and of intracellular acidification. J Physiol. 1994 Mar 15;475(3):483–494. doi: 10.1113/jphysiol.1994.sp020087. [DOI] [PMC free article] [PubMed] [Google Scholar]
- Curtin N. A., Woledge R. C. Efficiency of energy conversion during shortening of muscle fibres from the dogfish Scyliorhinus canicula. J Exp Biol. 1991 Jul;158:343–353. doi: 10.1242/jeb.158.1.343. [DOI] [PubMed] [Google Scholar]
- Curtin N. A., Woledge R. C. Energy changes and muscular contraction. Physiol Rev. 1978 Jul;58(3):690–761. doi: 10.1152/physrev.1978.58.3.690. [DOI] [PubMed] [Google Scholar]
- Fitts R. H. Cellular mechanisms of muscle fatigue. Physiol Rev. 1994 Jan;74(1):49–94. doi: 10.1152/physrev.1994.74.1.49. [DOI] [PubMed] [Google Scholar]
- Goody R. S., Holmes K. C. Cross-bridges and the mechanism of muscle contraction. Biochim Biophys Acta. 1983 Apr 15;726(1):13–39. doi: 10.1016/0304-4173(83)90009-5. [DOI] [PubMed] [Google Scholar]
- Gower D., Kretzschmar K. M. Heat production and chemical change during isometric contraction of rat soleus muscle. J Physiol. 1976 Jul;258(3):659–671. doi: 10.1113/jphysiol.1976.sp011439. [DOI] [PMC free article] [PubMed] [Google Scholar]
- HUXLEY A. F. Muscle structure and theories of contraction. Prog Biophys Biophys Chem. 1957;7:255–318. [PubMed] [Google Scholar]
- Homsher E., Kean C. J. Skeletal muscle energetics and metabolism. Annu Rev Physiol. 1978;40:93–131. doi: 10.1146/annurev.ph.40.030178.000521. [DOI] [PubMed] [Google Scholar]
- Homsher E., Mommaerts W. F., Ricchiuti N. V., Wallner A. Activation heat, activation metabolism and tension-related heat in frog semitendinosus muscles. J Physiol. 1972 Feb;220(3):601–625. doi: 10.1113/jphysiol.1972.sp009725. [DOI] [PMC free article] [PubMed] [Google Scholar]
- Homsher E. Muscle enthalpy production and its relationship to actomyosin ATPase. Annu Rev Physiol. 1987;49:673–690. doi: 10.1146/annurev.ph.49.030187.003325. [DOI] [PubMed] [Google Scholar]
- Homsher E., Yamada T., Wallner A., Tsai J. Energy balance studies in frog skeletal muscles shortening at one-half maximal velocity. J Gen Physiol. 1984 Sep;84(3):347–359. doi: 10.1085/jgp.84.3.347. [DOI] [PMC free article] [PubMed] [Google Scholar]
- Kretzschmar K. M., Wilkie D. R. A new method for absolute heat measurement, utilizing the Peltier effect. J Physiol. 1972 Jul;224(1):18P–21P. [PubMed] [Google Scholar]
- Mulieri L. A., Luhr G., Trefry J., Alpert N. R. Metal-film thermopiles for use with rabbit right ventricular papillary muscles. Am J Physiol. 1977 Nov;233(5):C146–C156. doi: 10.1152/ajpcell.1977.233.5.C146. [DOI] [PubMed] [Google Scholar]
- PODOLSKY R. J. Mechanochemical basis of muscular contraction. Fed Proc. 1962 Nov-Dec;21:964–974. [PubMed] [Google Scholar]
- Phillips S. K., Takei M., Yamada K. The time course of phosphate metabolites and intracellular pH using 31P NMR compared to recovery heat in rat soleus muscle. J Physiol. 1993 Jan;460:693–704. doi: 10.1113/jphysiol.1993.sp019494. [DOI] [PMC free article] [PubMed] [Google Scholar]
- Piazzesi G., Lombardi V. A cross-bridge model that is able to explain mechanical and energetic properties of shortening muscle. Biophys J. 1995 May;68(5):1966–1979. doi: 10.1016/S0006-3495(95)80374-7. [DOI] [PMC free article] [PubMed] [Google Scholar]
- Sahlin K., Edström L., Sjöholm H. Force, relaxation and energy metabolism of rat soleus muscle during anaerobic contraction. Acta Physiol Scand. 1987 Jan;129(1):1–7. doi: 10.1111/j.1748-1716.1987.tb08033.x. [DOI] [PubMed] [Google Scholar]
- Smith I. C. Energetics of activation in frog and toad muscle. J Physiol. 1972 Feb;220(3):583–599. doi: 10.1113/jphysiol.1972.sp009724. [DOI] [PMC free article] [PubMed] [Google Scholar]
- Westerblad H., Allen D. G. Changes of myoplasmic calcium concentration during fatigue in single mouse muscle fibers. J Gen Physiol. 1991 Sep;98(3):615–635. doi: 10.1085/jgp.98.3.615. [DOI] [PMC free article] [PubMed] [Google Scholar]
- Westerblad H., Allen D. G. Myoplasmic free Mg2+ concentration during repetitive stimulation of single fibres from mouse skeletal muscle. J Physiol. 1992;453:413–434. doi: 10.1113/jphysiol.1992.sp019236. [DOI] [PMC free article] [PubMed] [Google Scholar]
- Westerblad H., Lännergren J. Changes of the force-velocity relation, isometric tension and relaxation rate during fatigue in intact, single fibres of Xenopus skeletal muscle. J Muscle Res Cell Motil. 1994 Jun;15(3):287–298. doi: 10.1007/BF00123481. [DOI] [PubMed] [Google Scholar]
- Wohlfart B., Edman K. A. Rectangular hyperbola fitted to muscle force-velocity data using three-dimensional regression analysis. Exp Physiol. 1994 Mar;79(2):235–239. doi: 10.1113/expphysiol.1994.sp003756. [DOI] [PubMed] [Google Scholar]
- Woledge R. C., Reilly P. J. Molar enthalpy change for hydrolysis of phosphorylcreatine under conditions in muscle cells. Biophys J. 1988 Jul;54(1):97–104. doi: 10.1016/S0006-3495(88)82934-5. [DOI] [PMC free article] [PubMed] [Google Scholar]
- Woledge R. C. The energetics of tortoise muscle. J Physiol. 1968 Aug;197(3):685–707. doi: 10.1113/jphysiol.1968.sp008582. [DOI] [PMC free article] [PubMed] [Google Scholar]
- Woledge R. C., Wilson M. G., Howarth J. V., Elzinga G., Kometani K. The energetics of work and heat production by single muscle fibres from the frog. Adv Exp Med Biol. 1988;226:677–688. [PubMed] [Google Scholar]