Abstract
1. Potassium currents were characterized in turtle cochlear hair cells by whole-cell voltage clamp during superfusion with the potassium channel antagonists, tetraethylammonium (TEA) and 4-aminopyridine (4-AP). The estimated resonant frequency, f0, was inferred from tau, the time constant of deactivation of outward current upon repolarization to -50 mV, according to the empirical relation, f0 = k1 tau-1/2 + k2. 2. Dose-response relations for TEA and 4-AP were obtained by exposing single cells to ten concentrations exponentially distributed over four orders of magnitude. Potassium current in cells tuned to low frequencies was carried by a single class of channels with an apparent affinity constant, K1, for TEA of 35.9 mM. Half-blocking concentrations of 4-AP were correlated with the time constant of deactivation and varied between 26.2 and 102 microM. In cells tuned to higher frequencies, K+ current was carried by a single class of channels with high affinity for TEA (K1 = 0.215 mM) and low affinity for 4-AP (K1 = 12.3 mM). This pharmacological profile suggests that K+ current in low frequency cells is purely voltage gated and in high frequency cells, it is gated by both Ca2+ and voltage. 3. For each current type, the voltage dependence of activation was determined from tail current amplitude at -50 mV. The purely voltage-gated current, IK(V), was found to increase e-fold in 4.0 +/- 0.3 mV (n = 3) in low frequency cells exposed to TEA (25 mM). The Ca(2+)- and voltage-gated current, IK(Ca), was more steeply voltage dependent, increasing e-fold in 1.9 mV (n = 2) in high frequency cells exposed to 4-AP (0.8 mM). 4. IK(V) was found to inactivate slowly during prolonged voltage steps (approximately 10 s). Steady-state inactivation increased with depolarization from -70 mV and was incomplete such that on average IK(v) did not fall below approximately 0.39 of its maximum value. 5. Superfusion of 4-AP (0.8 mM) reversibly depolarized a low frequency cell and eliminated steady voltage oscillations, while TEA (6 mM) had no effect. In a high frequency cell, voltage oscillations were abolished by TEA, but not by 4-AP. 6. The differential pharmacology of IK(V) and IK(Ca) was used to measure their contribution to K+ current in cells tuned to different frequencies. Both currents exhibited a frequency-dependent increase in maximum conductance. IK(V) accounted for nearly all K+ current in cells tuned to less than 60 Hz, while IK(Ca) was the dominant current in higher frequency cells. 7. Mapping resonant frequency onto epithelial position suggests an exponential relation between K+ current size and position. IK(V) appeared to be limited to the apical or low frequency portion of the basilar papilla and coincided with maximal expression of a K(+)-selective inward rectifier, IK(IR). This finding is consistent with the notion that low frequency resonance is produced by interaction of IK(V) and IK(IR) with the voltage-gated Ca2+ current, ICa, and the cell's capacitance. The ionic events underlying higher frequency resonance are dominated by the action of IK(Ca) and ICa and include a contribution from IK(IR).
Full text
PDF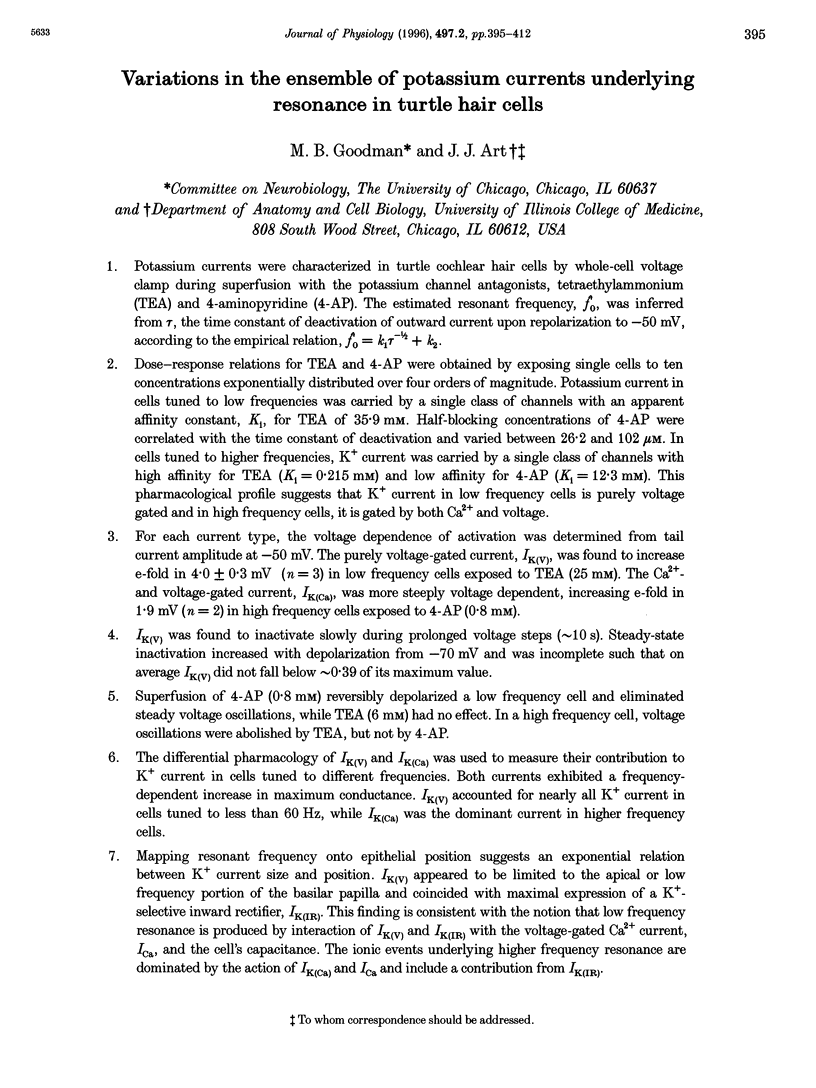
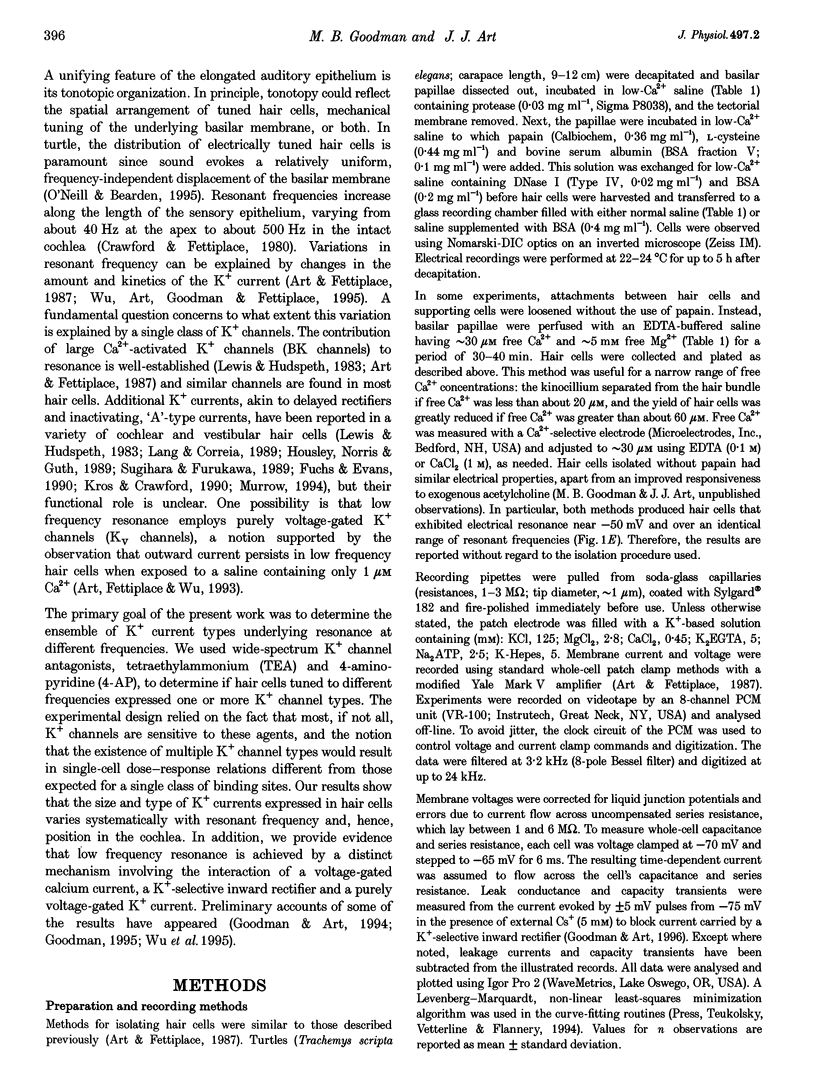
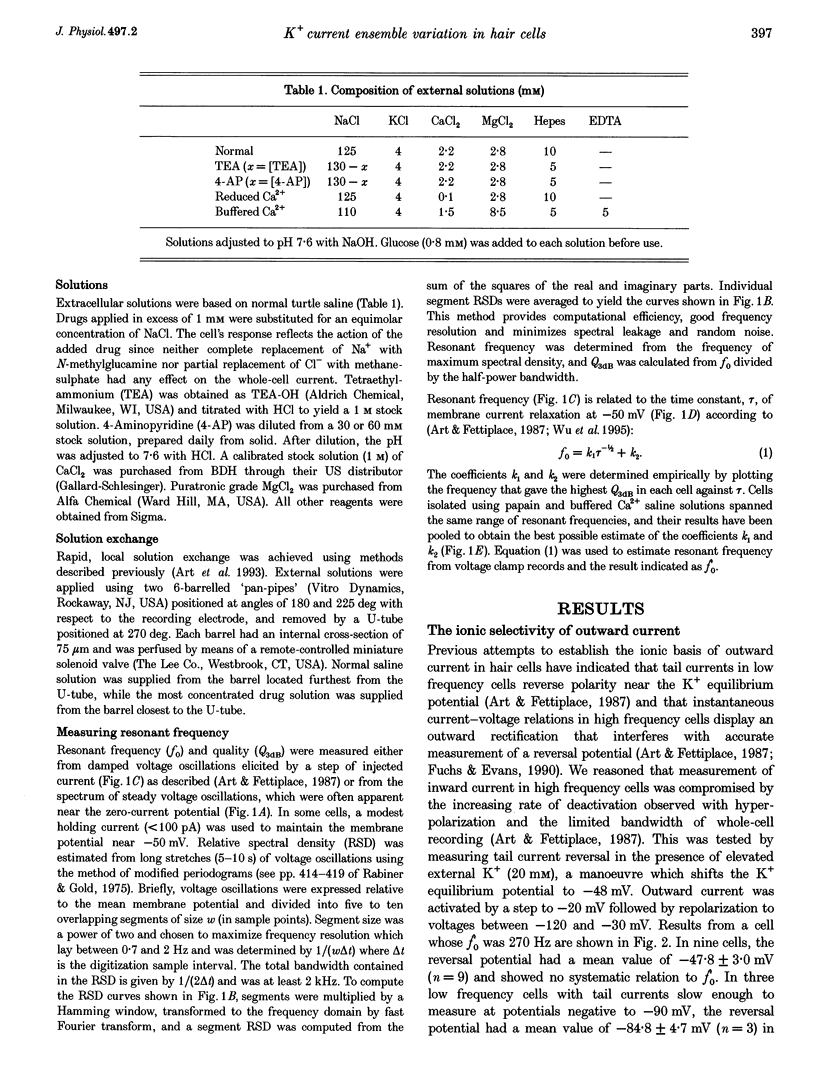
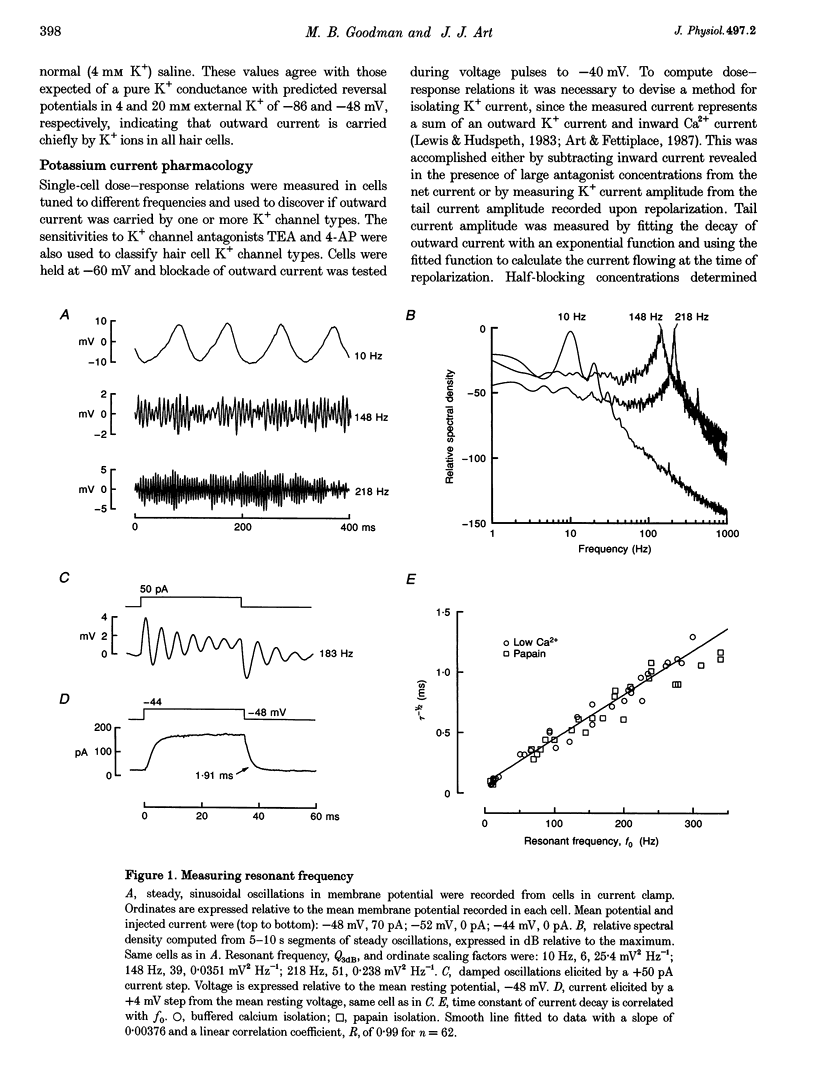
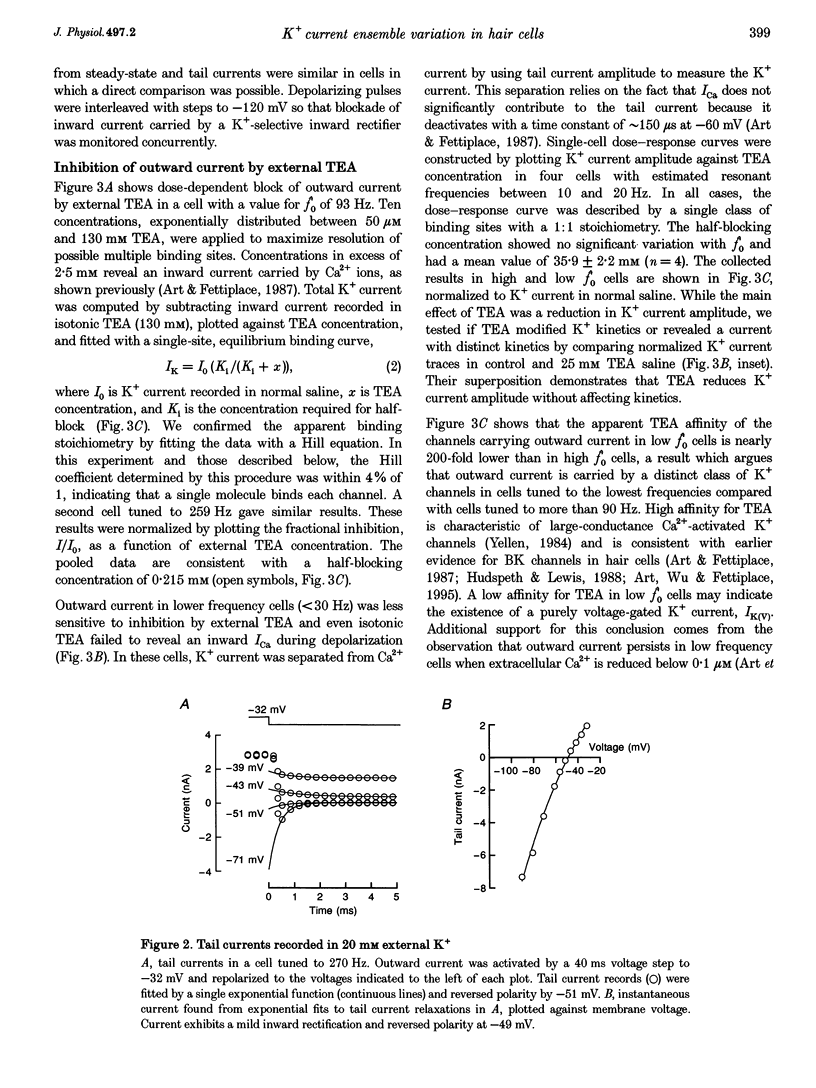
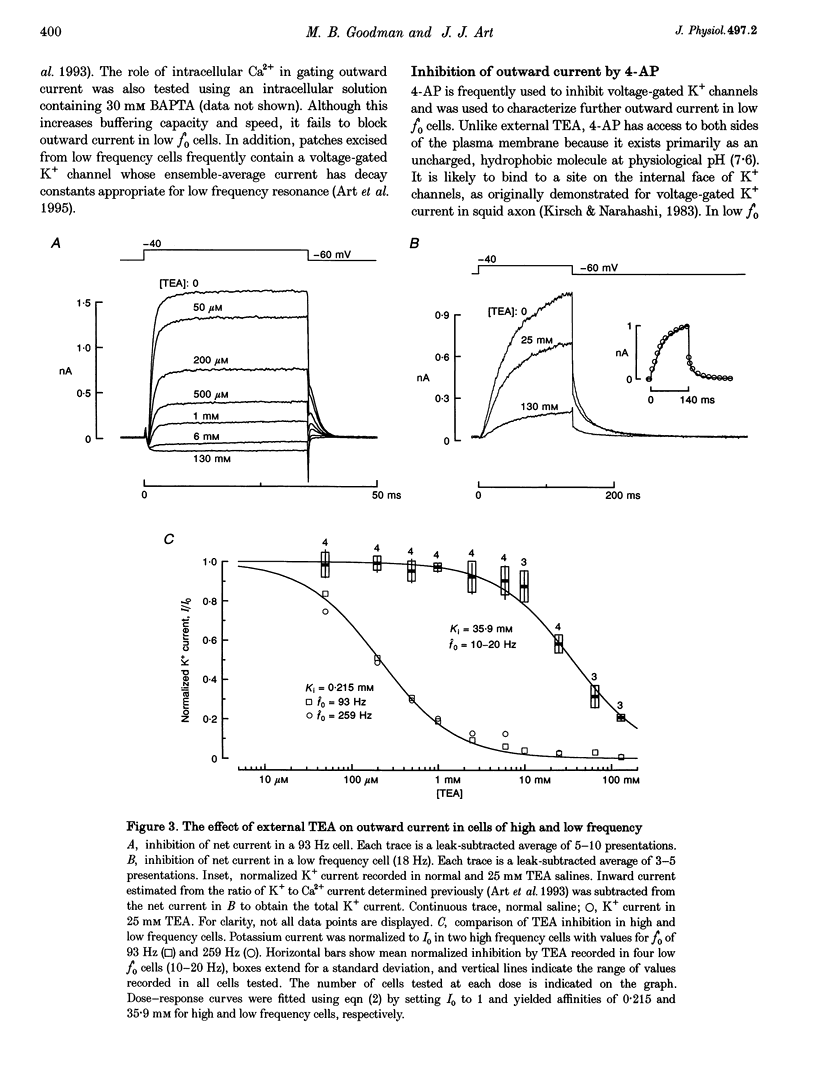
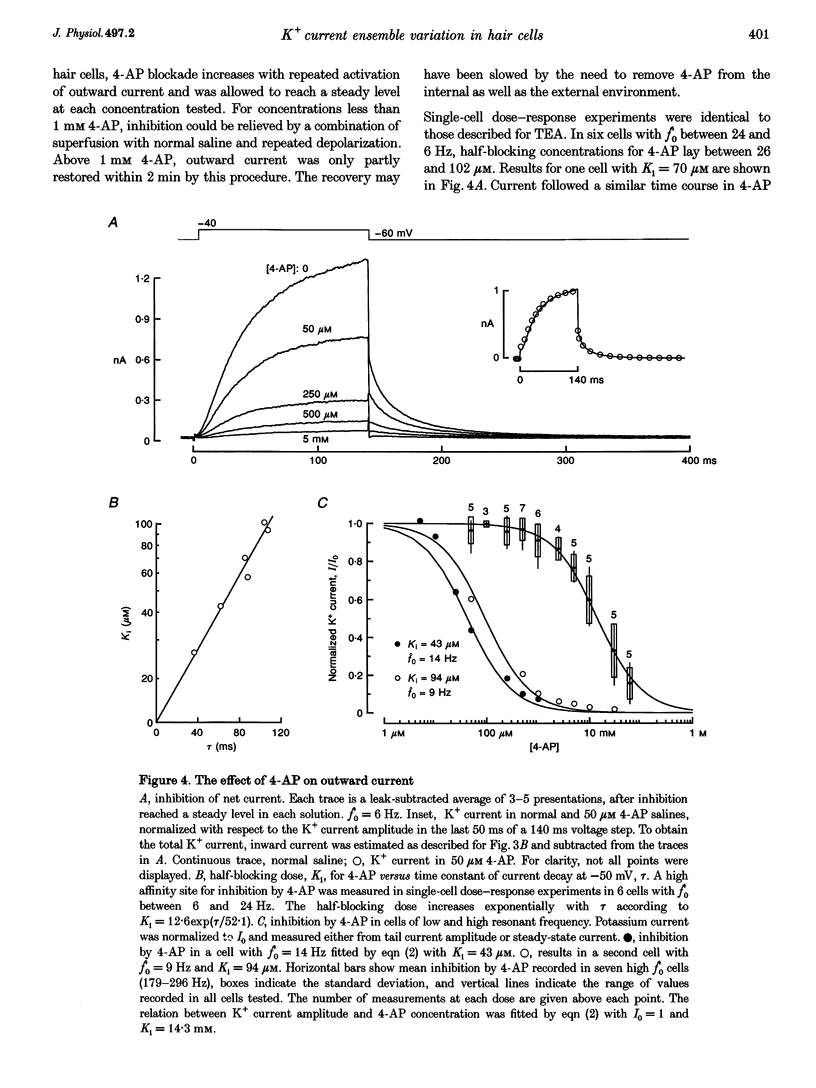
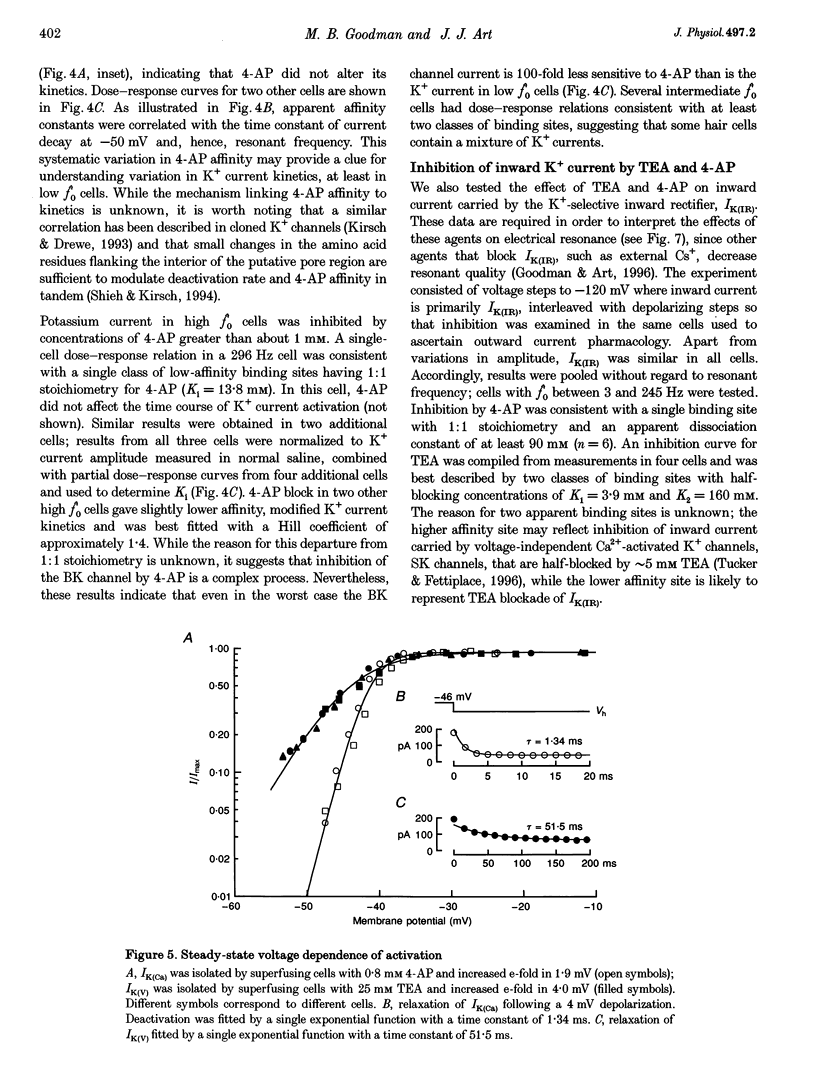
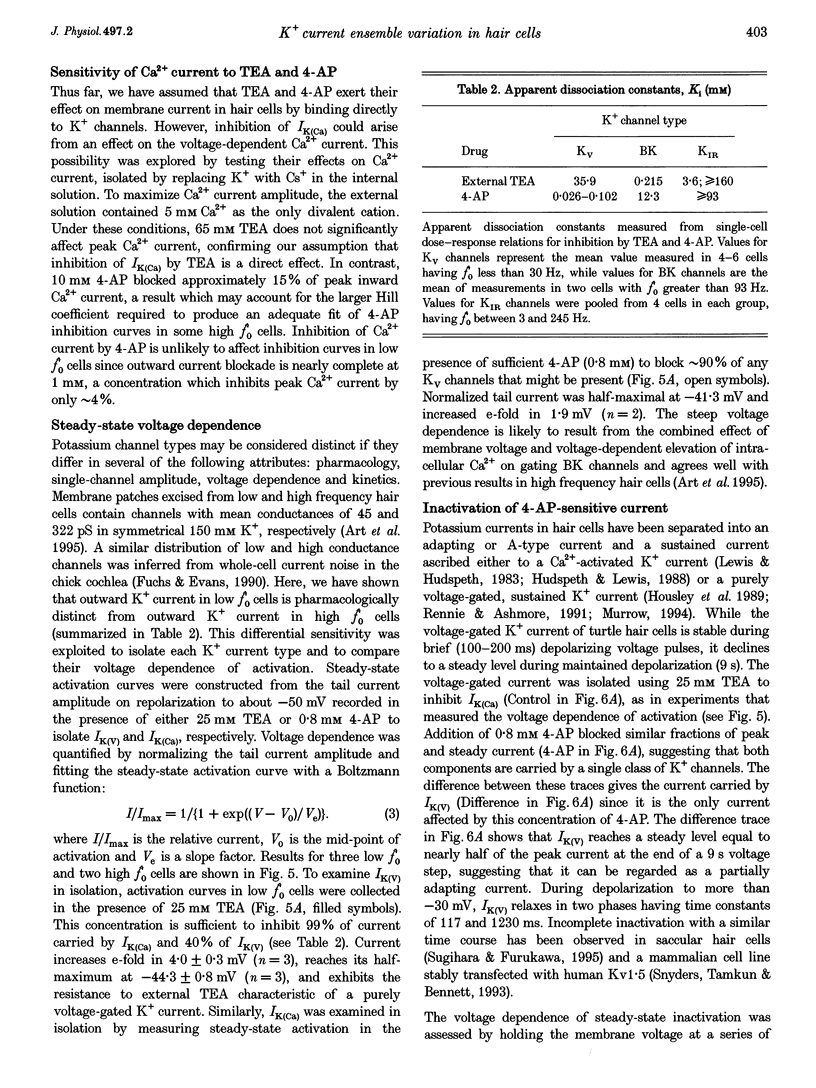
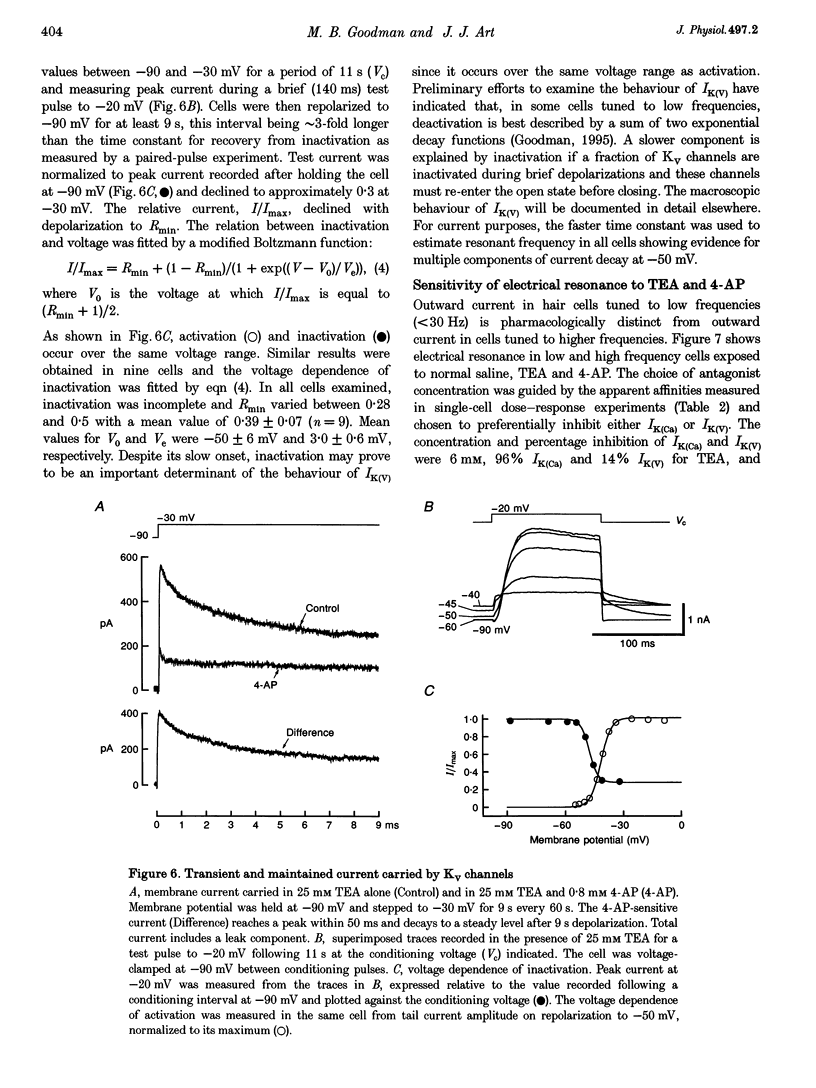
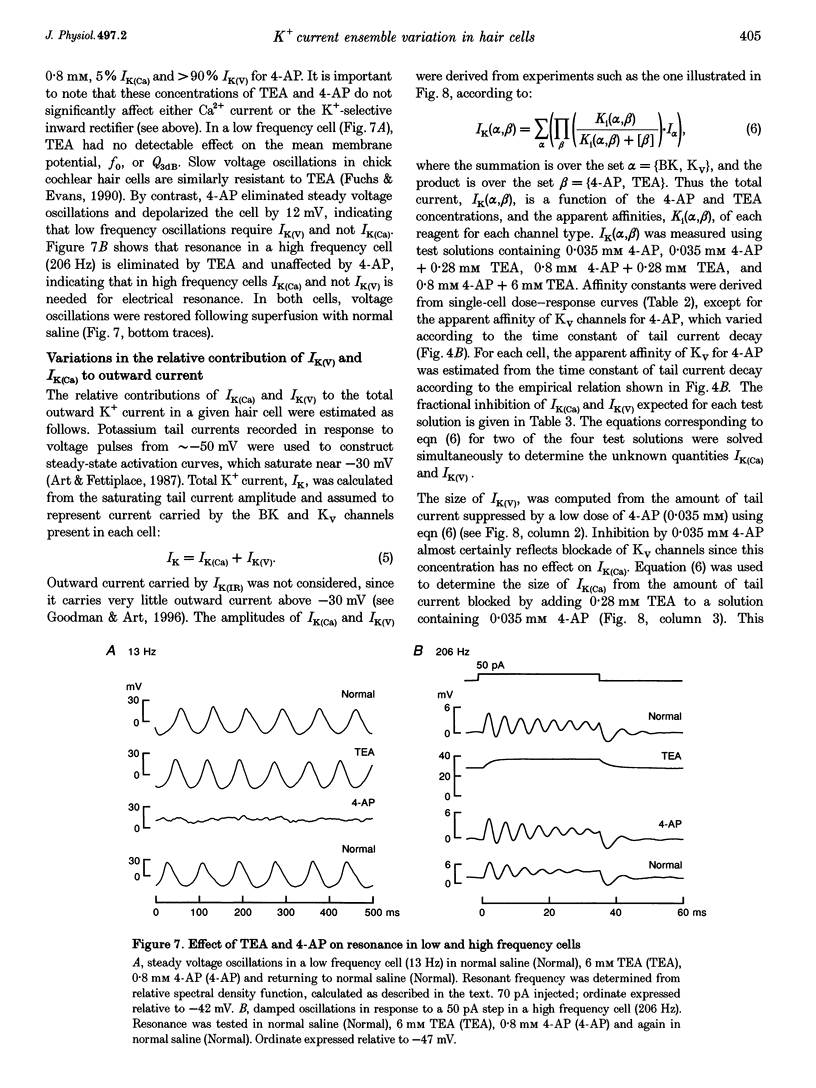
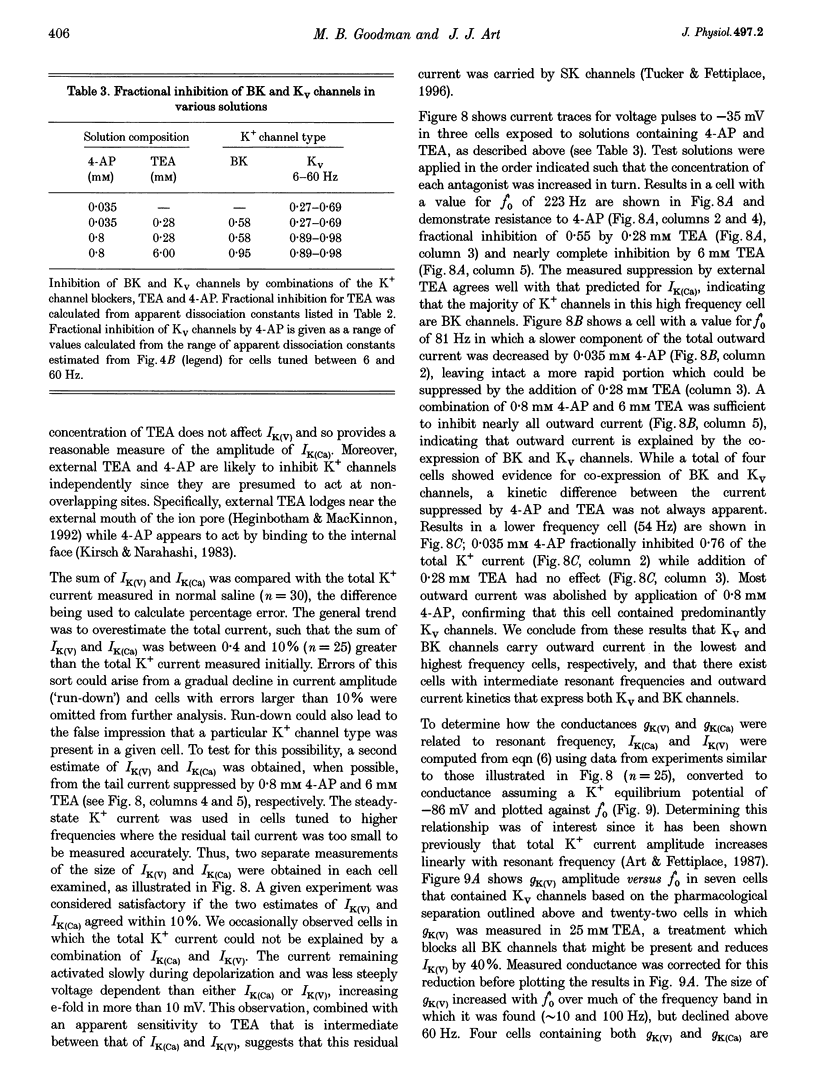
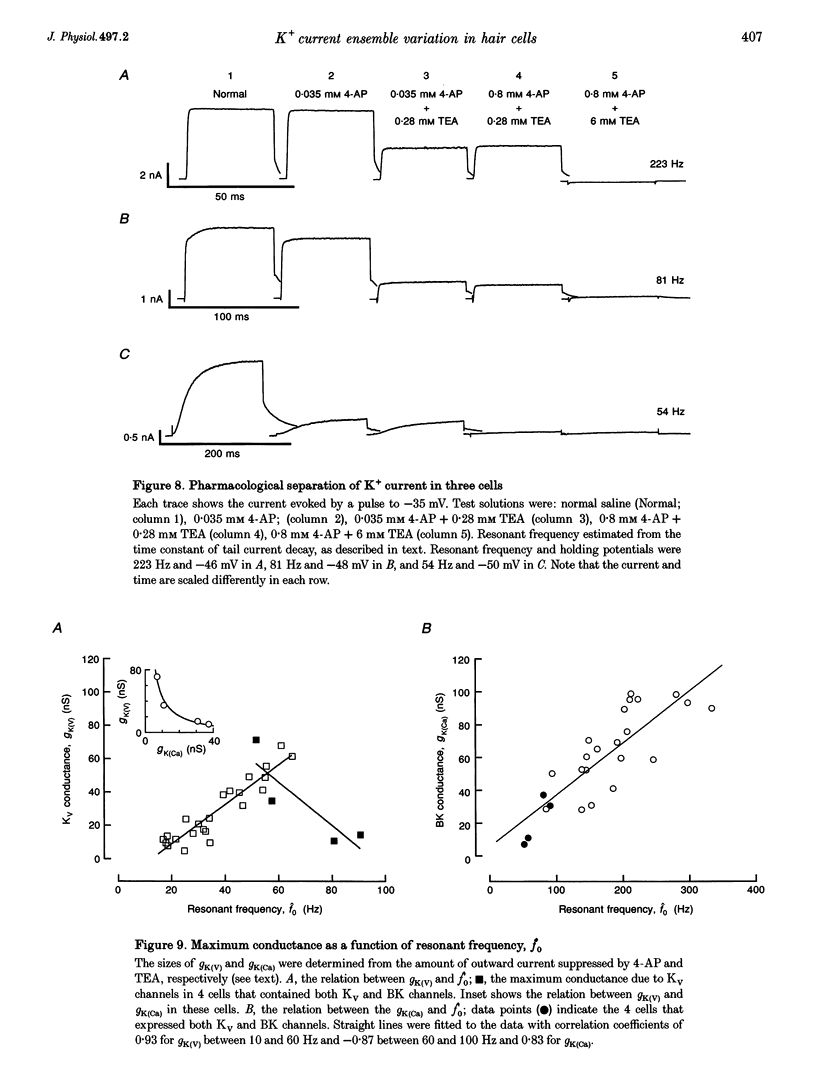
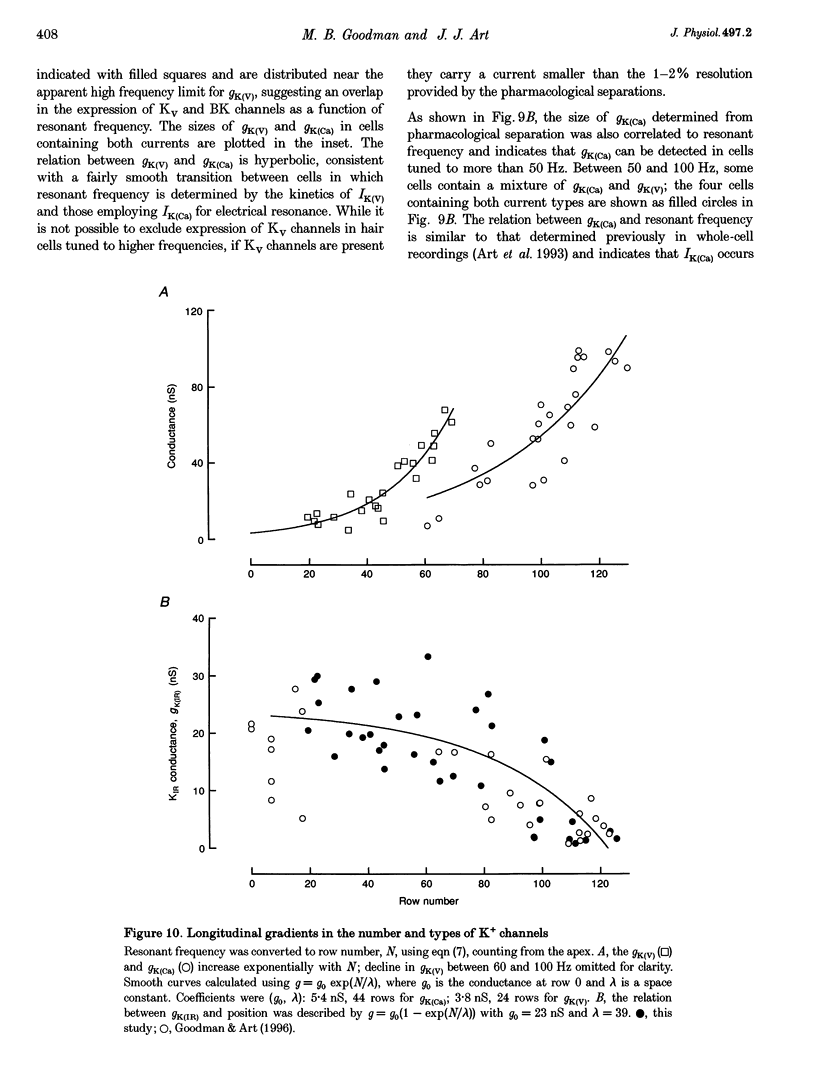
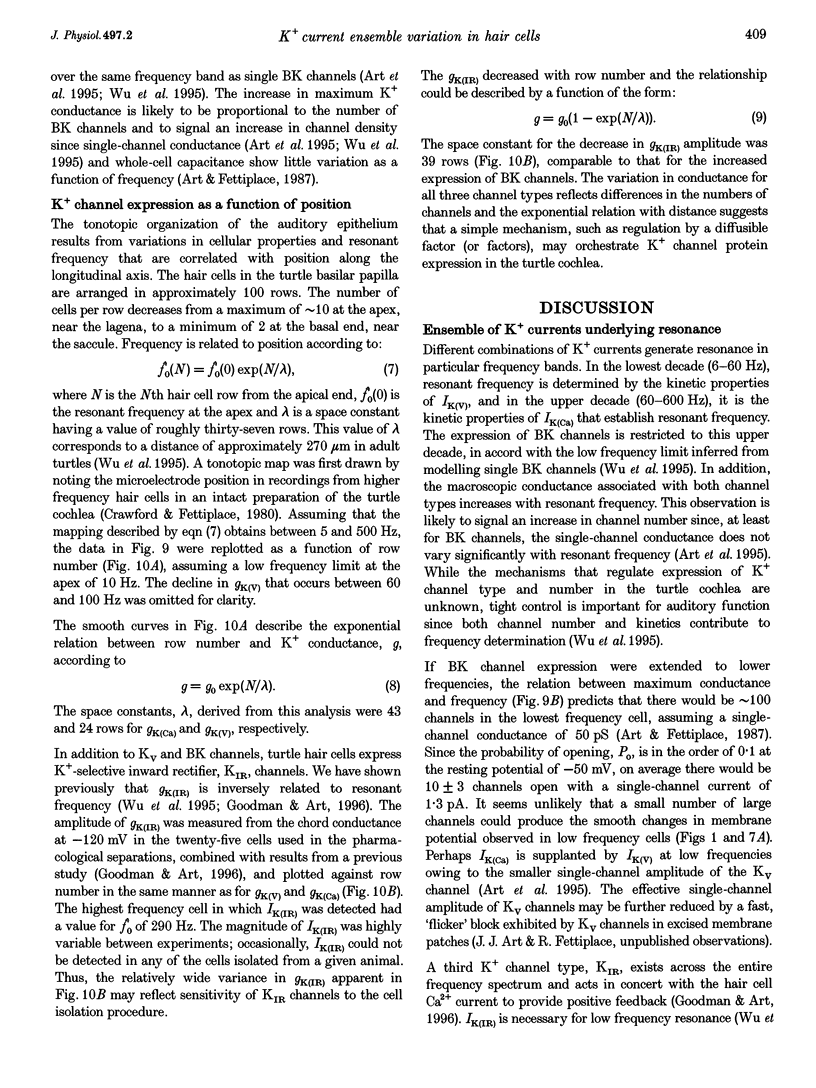
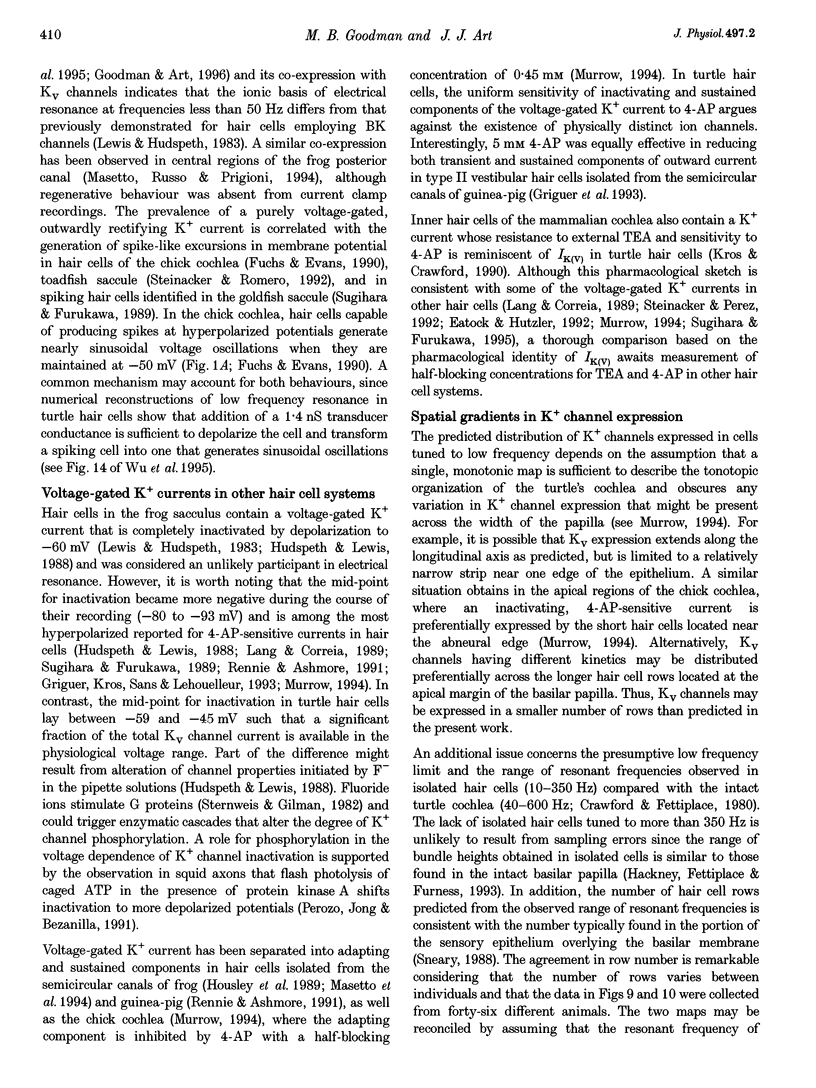
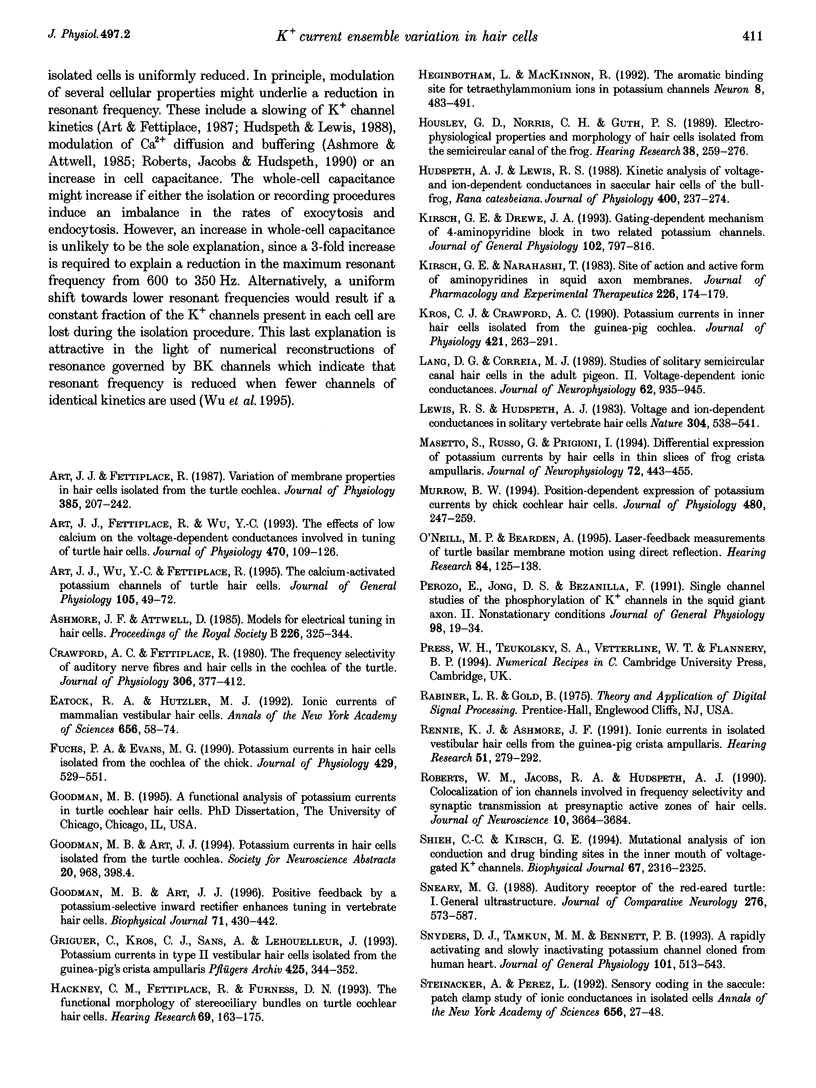
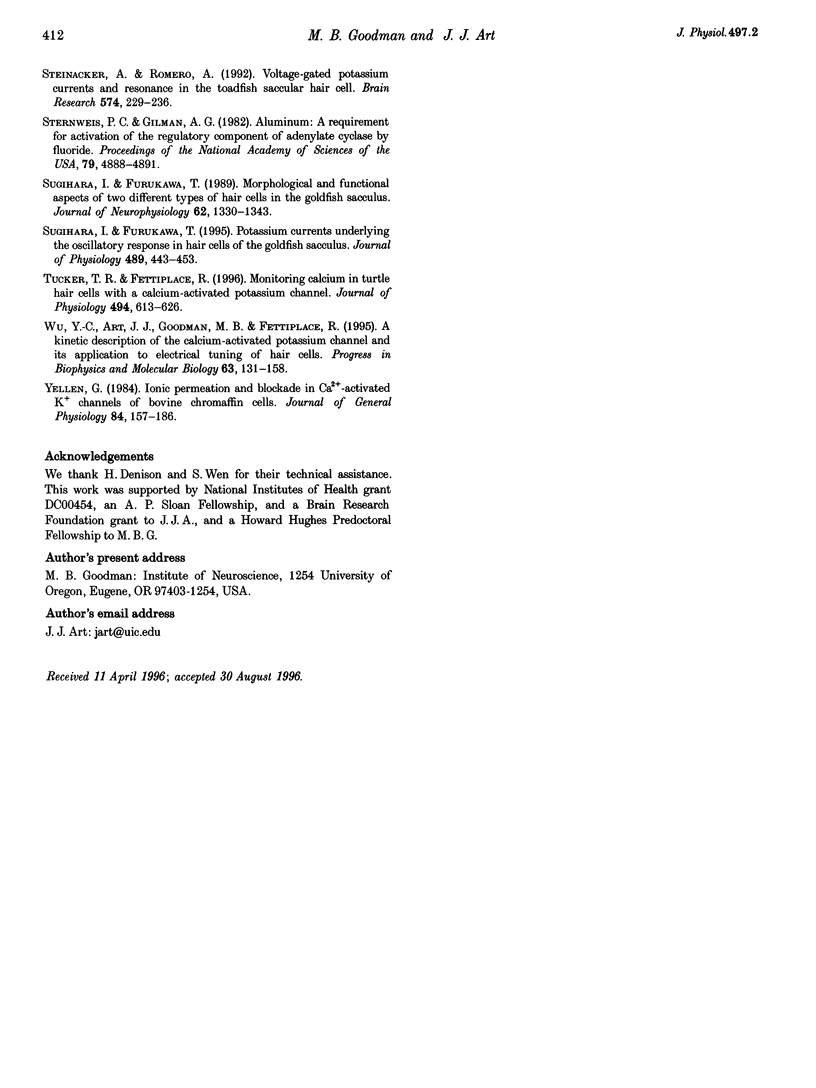
Selected References
These references are in PubMed. This may not be the complete list of references from this article.
- Art J. J., Fettiplace R. Variation of membrane properties in hair cells isolated from the turtle cochlea. J Physiol. 1987 Apr;385:207–242. doi: 10.1113/jphysiol.1987.sp016492. [DOI] [PMC free article] [PubMed] [Google Scholar]
- Art J. J., Fettiplace R., Wu Y. C. The effects of low calcium on the voltage-dependent conductances involved in tuning of turtle hair cells. J Physiol. 1993 Oct;470:109–126. doi: 10.1113/jphysiol.1993.sp019850. [DOI] [PMC free article] [PubMed] [Google Scholar]
- Art J. J., Wu Y. C., Fettiplace R. The calcium-activated potassium channels of turtle hair cells. J Gen Physiol. 1995 Jan;105(1):49–72. doi: 10.1085/jgp.105.1.49. [DOI] [PMC free article] [PubMed] [Google Scholar]
- Eatock R. A., Hutzler M. J. Ionic currents of mammalian vestibular hair cells. Ann N Y Acad Sci. 1992 May 22;656:58–74. doi: 10.1111/j.1749-6632.1992.tb25200.x. [DOI] [PubMed] [Google Scholar]
- Fuchs P. A., Evans M. G. Potassium currents in hair cells isolated from the cochlea of the chick. J Physiol. 1990 Oct;429:529–551. doi: 10.1113/jphysiol.1990.sp018271. [DOI] [PMC free article] [PubMed] [Google Scholar]
- Goodman M. B., Art J. J. Positive feedback by a potassium-selective inward rectifier enhances tuning in vertebrate hair cells. Biophys J. 1996 Jul;71(1):430–442. doi: 10.1016/S0006-3495(96)79245-7. [DOI] [PMC free article] [PubMed] [Google Scholar]
- Griguer C., Kros C. J., Sans A., Lehouelleur J. Potassium currents in type II vestibular hair cells isolated from the guinea-pig's crista ampullaris. Pflugers Arch. 1993 Nov;425(3-4):344–352. doi: 10.1007/BF00374185. [DOI] [PubMed] [Google Scholar]
- Hackney C. M., Fettiplace R., Furness D. N. The functional morphology of stereociliary bundles on turtle cochlear hair cells. Hear Res. 1993 Sep;69(1-2):163–175. doi: 10.1016/0378-5955(93)90104-9. [DOI] [PubMed] [Google Scholar]
- Heginbotham L., MacKinnon R. The aromatic binding site for tetraethylammonium ion on potassium channels. Neuron. 1992 Mar;8(3):483–491. doi: 10.1016/0896-6273(92)90276-j. [DOI] [PubMed] [Google Scholar]
- Housley G. D., Norris C. H., Guth P. S. Electrophysiological properties and morphology of hair cells isolated from the semicircular canal of the frog. Hear Res. 1989 Apr;38(3):259–276. doi: 10.1016/0378-5955(89)90070-1. [DOI] [PubMed] [Google Scholar]
- Hudspeth A. J., Lewis R. S. Kinetic analysis of voltage- and ion-dependent conductances in saccular hair cells of the bull-frog, Rana catesbeiana. J Physiol. 1988 Jun;400:237–274. doi: 10.1113/jphysiol.1988.sp017119. [DOI] [PMC free article] [PubMed] [Google Scholar]
- Kirsch G. E., Drewe J. A. Gating-dependent mechanism of 4-aminopyridine block in two related potassium channels. J Gen Physiol. 1993 Nov;102(5):797–816. doi: 10.1085/jgp.102.5.797. [DOI] [PMC free article] [PubMed] [Google Scholar]
- Kirsch G. E., Narahashi T. Site of action and active form of aminopyridines in squid axon membranes. J Pharmacol Exp Ther. 1983 Jul;226(1):174–179. [PubMed] [Google Scholar]
- Kros C. J., Crawford A. C. Potassium currents in inner hair cells isolated from the guinea-pig cochlea. J Physiol. 1990 Feb;421:263–291. doi: 10.1113/jphysiol.1990.sp017944. [DOI] [PMC free article] [PubMed] [Google Scholar]
- Lang D. G., Correia M. J. Studies of solitary semicircular canal hair cells in the adult pigeon. II. Voltage-dependent ionic conductances. J Neurophysiol. 1989 Oct;62(4):935–945. doi: 10.1152/jn.1989.62.4.935. [DOI] [PubMed] [Google Scholar]
- Lewis R. S., Hudspeth A. J. Voltage- and ion-dependent conductances in solitary vertebrate hair cells. Nature. 1983 Aug 11;304(5926):538–541. doi: 10.1038/304538a0. [DOI] [PubMed] [Google Scholar]
- Masetto S., Russo G., Prigioni I. Differential expression of potassium currents by hair cells in thin slices of frog crista ampullaris. J Neurophysiol. 1994 Jul;72(1):443–455. doi: 10.1152/jn.1994.72.1.443. [DOI] [PubMed] [Google Scholar]
- Murrow B. W. Position-dependent expression of potassium currents by chick cochlear hair cells. J Physiol. 1994 Oct 15;480(Pt 2):247–259. doi: 10.1113/jphysiol.1994.sp020357. [DOI] [PMC free article] [PubMed] [Google Scholar]
- O'Neill M. P., Bearden A. Laser-feedback measurements of turtle basilar membrane motion using direct reflection. Hear Res. 1995 Apr;84(1-2):125–138. doi: 10.1016/0378-5955(95)00018-y. [DOI] [PubMed] [Google Scholar]
- Pappone P. A. Voltage-clamp experiments in normal and denervated mammalian skeletal muscle fibres. J Physiol. 1980 Sep;306:377–410. doi: 10.1113/jphysiol.1980.sp013403. [DOI] [PMC free article] [PubMed] [Google Scholar]
- Perozo E., Jong D. S., Bezanilla F. Single channel studies of the phosphorylation of K+ channels in the squid giant axon. II. Nonstationary conditions. J Gen Physiol. 1991 Jul;98(1):19–34. doi: 10.1085/jgp.98.1.19. [DOI] [PMC free article] [PubMed] [Google Scholar]
- Rennie K. J., Ashmore J. F. Ionic currents in isolated vestibular hair cells from the guinea-pig crista ampullaris. Hear Res. 1991 Feb;51(2):279–291. doi: 10.1016/0378-5955(91)90044-a. [DOI] [PubMed] [Google Scholar]
- Roberts W. M., Jacobs R. A., Hudspeth A. J. Colocalization of ion channels involved in frequency selectivity and synaptic transmission at presynaptic active zones of hair cells. J Neurosci. 1990 Nov;10(11):3664–3684. doi: 10.1523/JNEUROSCI.10-11-03664.1990. [DOI] [PMC free article] [PubMed] [Google Scholar]
- Shieh C. C., Kirsch G. E. Mutational analysis of ion conduction and drug binding sites in the inner mouth of voltage-gated K+ channels. Biophys J. 1994 Dec;67(6):2316–2325. doi: 10.1016/S0006-3495(94)80718-0. [DOI] [PMC free article] [PubMed] [Google Scholar]
- Sneary M. G. Auditory receptor of the red-eared turtle: I. General ultrastructure. J Comp Neurol. 1988 Oct 22;276(4):573–587. doi: 10.1002/cne.902760410. [DOI] [PubMed] [Google Scholar]
- Snyders D. J., Tamkun M. M., Bennett P. B. A rapidly activating and slowly inactivating potassium channel cloned from human heart. Functional analysis after stable mammalian cell culture expression. J Gen Physiol. 1993 Apr;101(4):513–543. doi: 10.1085/jgp.101.4.513. [DOI] [PMC free article] [PubMed] [Google Scholar]
- Steinacker A., Perez L. Sensory coding in the saccule. Patch clamp study of ionic conductances in isolated cells. Ann N Y Acad Sci. 1992 May 22;656:27–48. doi: 10.1111/j.1749-6632.1992.tb25198.x. [DOI] [PubMed] [Google Scholar]
- Steinacker A., Romero A. Voltage-gated potassium current and resonance in the toadfish saccular hair cell. Brain Res. 1992 Mar 6;574(1-2):229–236. doi: 10.1016/0006-8993(92)90821-p. [DOI] [PubMed] [Google Scholar]
- Sternweis P. C., Gilman A. G. Aluminum: a requirement for activation of the regulatory component of adenylate cyclase by fluoride. Proc Natl Acad Sci U S A. 1982 Aug;79(16):4888–4891. doi: 10.1073/pnas.79.16.4888. [DOI] [PMC free article] [PubMed] [Google Scholar]
- Sugihara I., Furukawa T. Morphological and functional aspects of two different types of hair cells in the goldfish sacculus. J Neurophysiol. 1989 Dec;62(6):1330–1343. doi: 10.1152/jn.1989.62.6.1330. [DOI] [PubMed] [Google Scholar]
- Sugihara I., Furukawa T. Potassium currents underlying the oscillatory response in hair cells of the goldfish sacculus. J Physiol. 1995 Dec 1;489(Pt 2):443–453. doi: 10.1113/jphysiol.1995.sp021064. [DOI] [PMC free article] [PubMed] [Google Scholar]
- Tucker T. R., Fettiplace R. Monitoring calcium in turtle hair cells with a calcium-activated potassium channel. J Physiol. 1996 Aug 1;494(Pt 3):613–626. doi: 10.1113/jphysiol.1996.sp021519. [DOI] [PMC free article] [PubMed] [Google Scholar]
- Wu Y. C., Art J. J., Goodman M. B., Fettiplace R. A kinetic description of the calcium-activated potassium channel and its application to electrical tuning of hair cells. Prog Biophys Mol Biol. 1995;63(2):131–158. doi: 10.1016/0079-6107(95)00002-5. [DOI] [PubMed] [Google Scholar]
- Yellen G. Ionic permeation and blockade in Ca2+-activated K+ channels of bovine chromaffin cells. J Gen Physiol. 1984 Aug;84(2):157–186. doi: 10.1085/jgp.84.2.157. [DOI] [PMC free article] [PubMed] [Google Scholar]