Abstract
Triacylglycerol metabolism has been studied in kidney cortex tubules from starved rats, prepared by collagenase treatment. Triacylglycerol was determined by a newly developed fully enzymic method. Incubation of tubules in the absence of fatty acids led to a decrease of endogenous triacylglycerol by about 50% in 1h. Addition of albuminbound oleate or palmitate resulted in a steady increase of tissue triacylglycerol over 2h. The rate of triacylglycerol synthesis was linearly dependent on oleate concentration up to 0.8mm, reaching a saturation at higher concentrations. Triacylglycerol formation from palmitate was less than that from oleate. This difference was qualitatively the same when net synthesis was compared with incorporation of labelled fatty acids. Quantitatively, however, the difference was less with the incorporation technique. Gluconeogenic substrates, which by themselves had no effect on triacylglycerol concentrations, stimulated neutral lipid formation from fatty acids. Glucose and lysine did not have such a stimulatory effect. Inhibition of gluconeogenesis from lactate by mercaptopicolinic acid likewise inhibited triacylglycerol formation. This inhibitory effect was seen with oleate as well as with oleate plus lactate. When [2-14C]lactate was used the incorporation of label into triacylglycerol was found in the glycerol moiety exclusively. Addition of dl-β-hydroxybutyrate (5mm) to the incubation medium in the presence of oleate or oleate plus lactate led to a significant increase in triacylglycerol formation. In contrast with the gluconeogenic substrates, dl-β-hydroxybutyrate had no stimulatory effect on fatty acid uptake. The results suggest that renal triacylglycerol formation is a quantitatively important metabolic process. The finding that gluconeogenic substrates, but not glucose, increase lipid formation, indicates that the glycerol moiety is formed by glyceroneogenesis in the proximal tubules. The effect of ketone bodies seems to be caused by the sparing action of these substrates on fatty acid oxidation. The decrease of triacylglycerol in the absence of exogenous substrates confirms previous conclusions that endogenous lipids provide fatty acids for renal energy metabolism.
Full text
PDF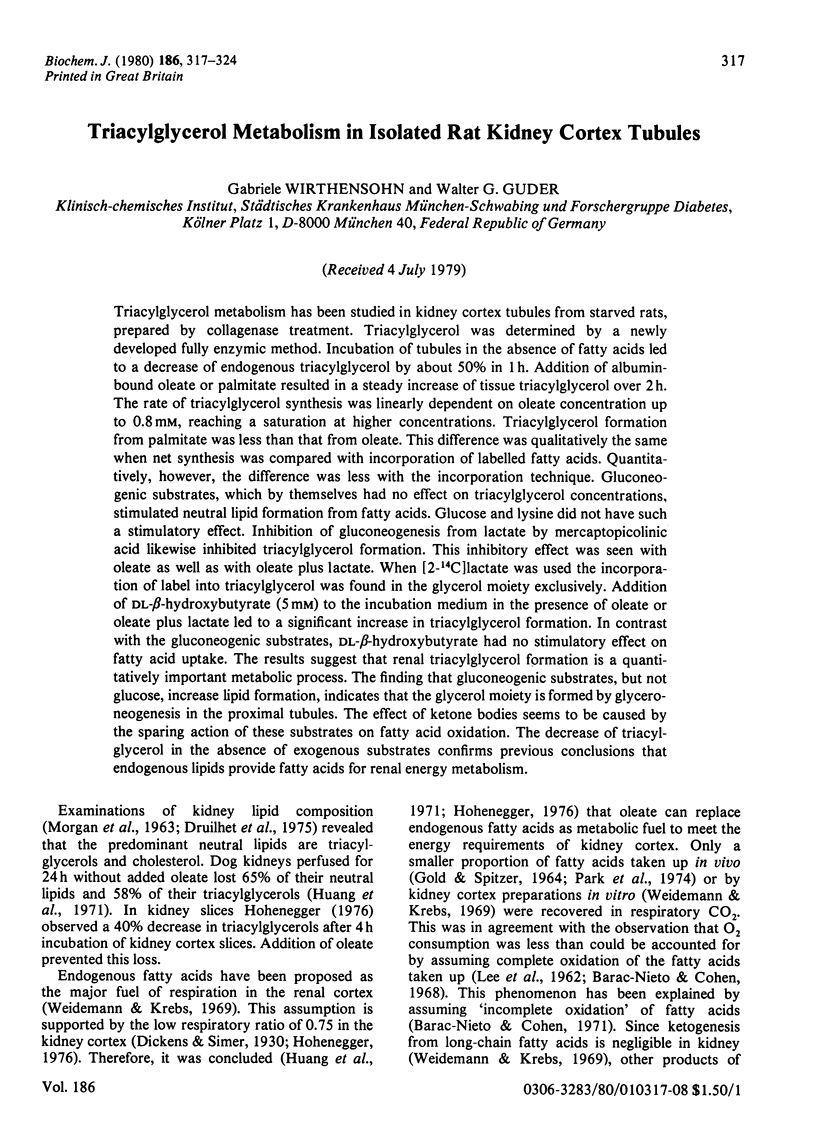
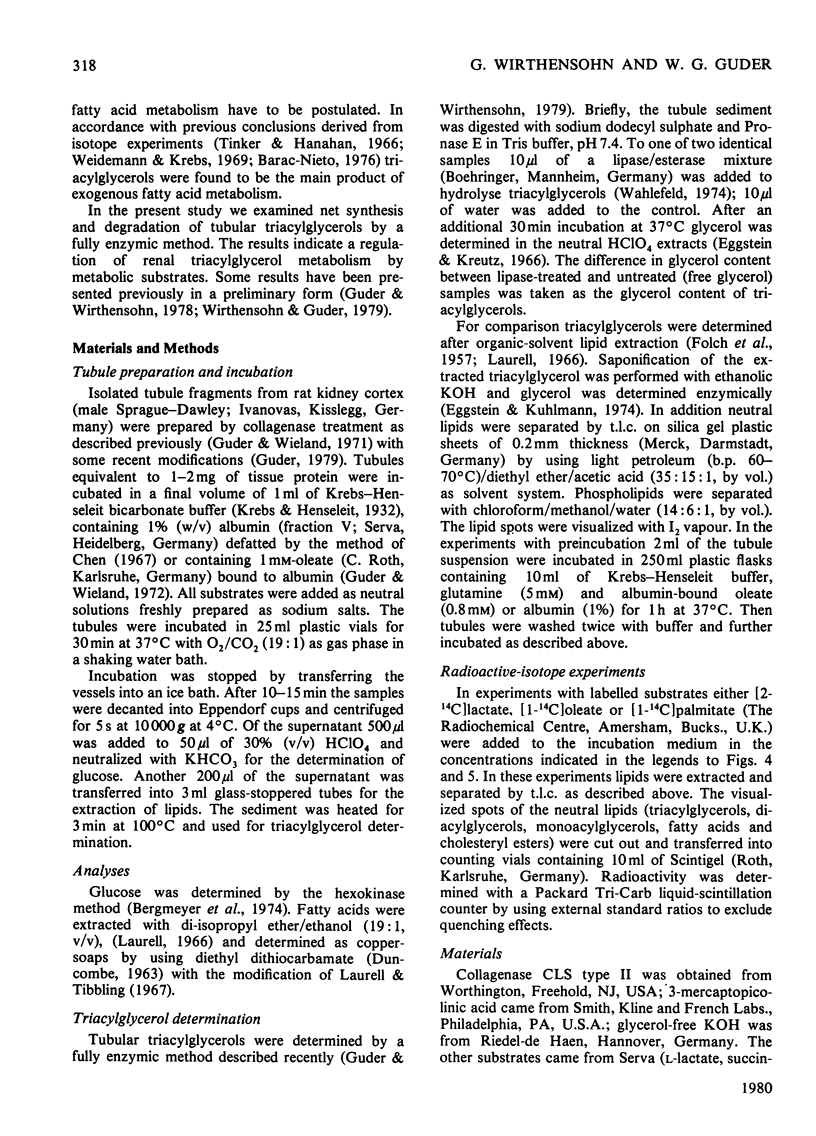
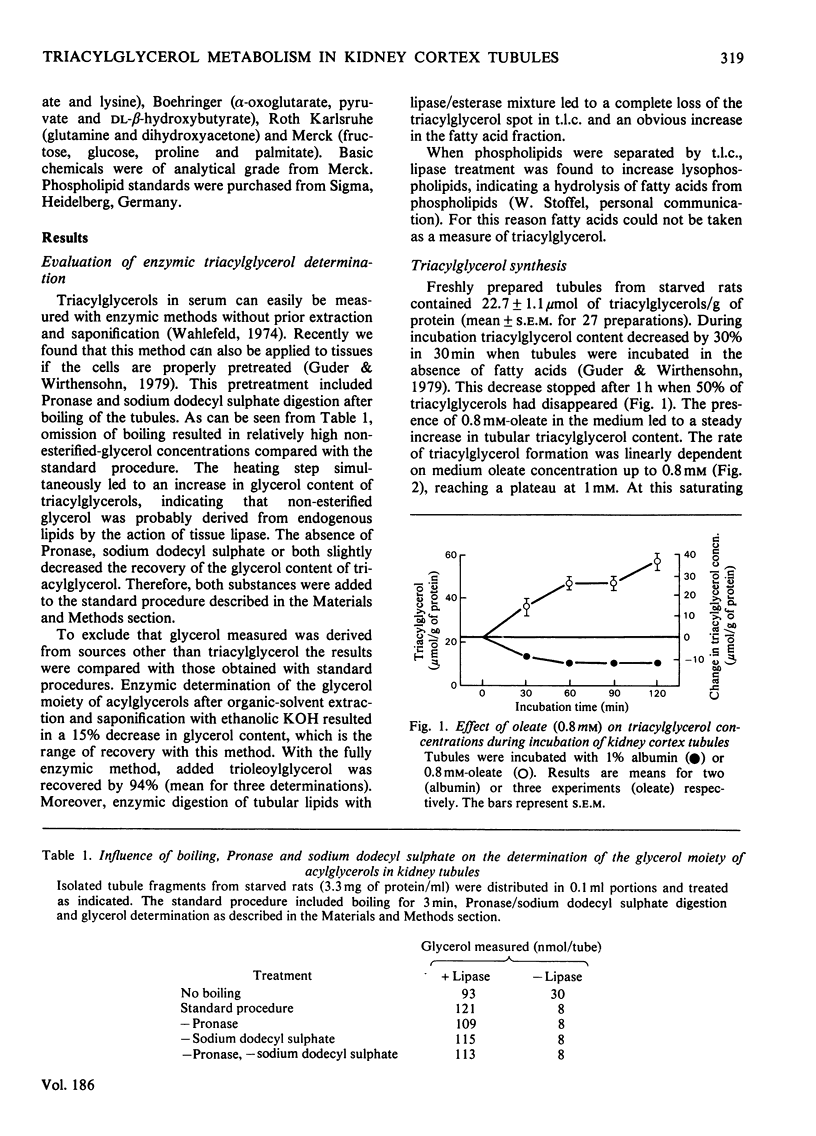
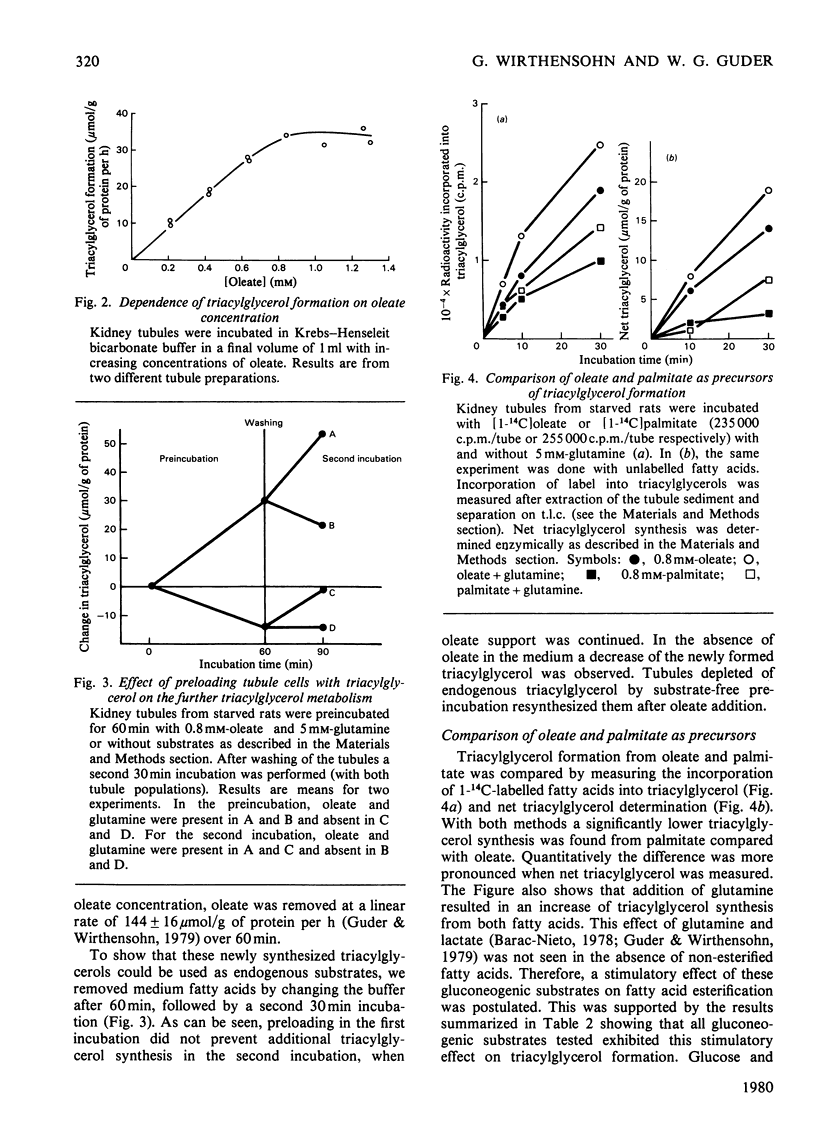
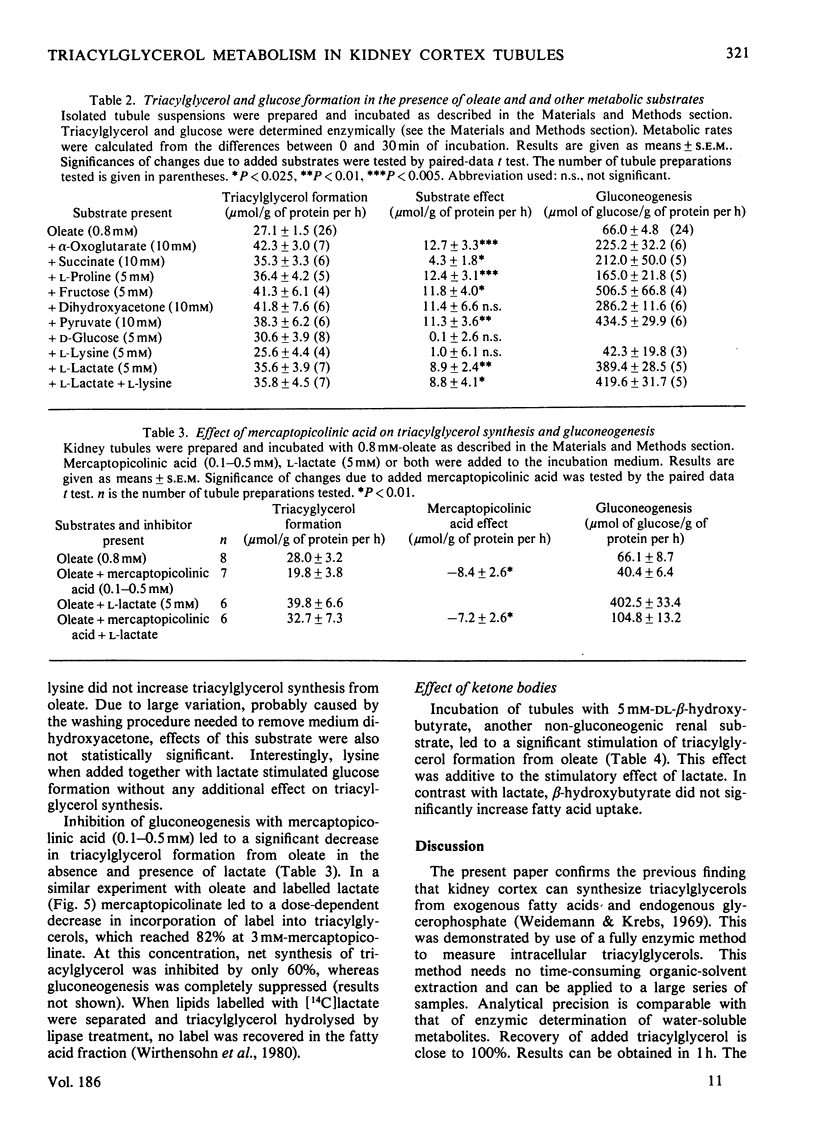
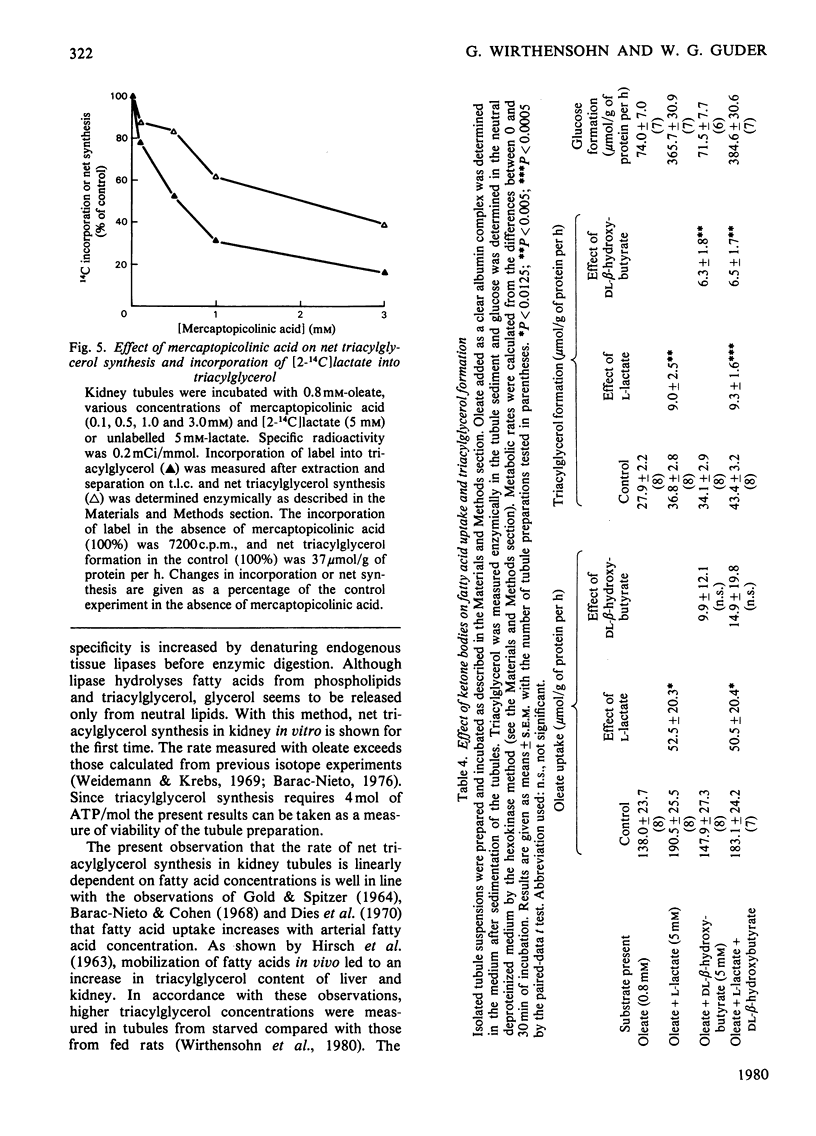
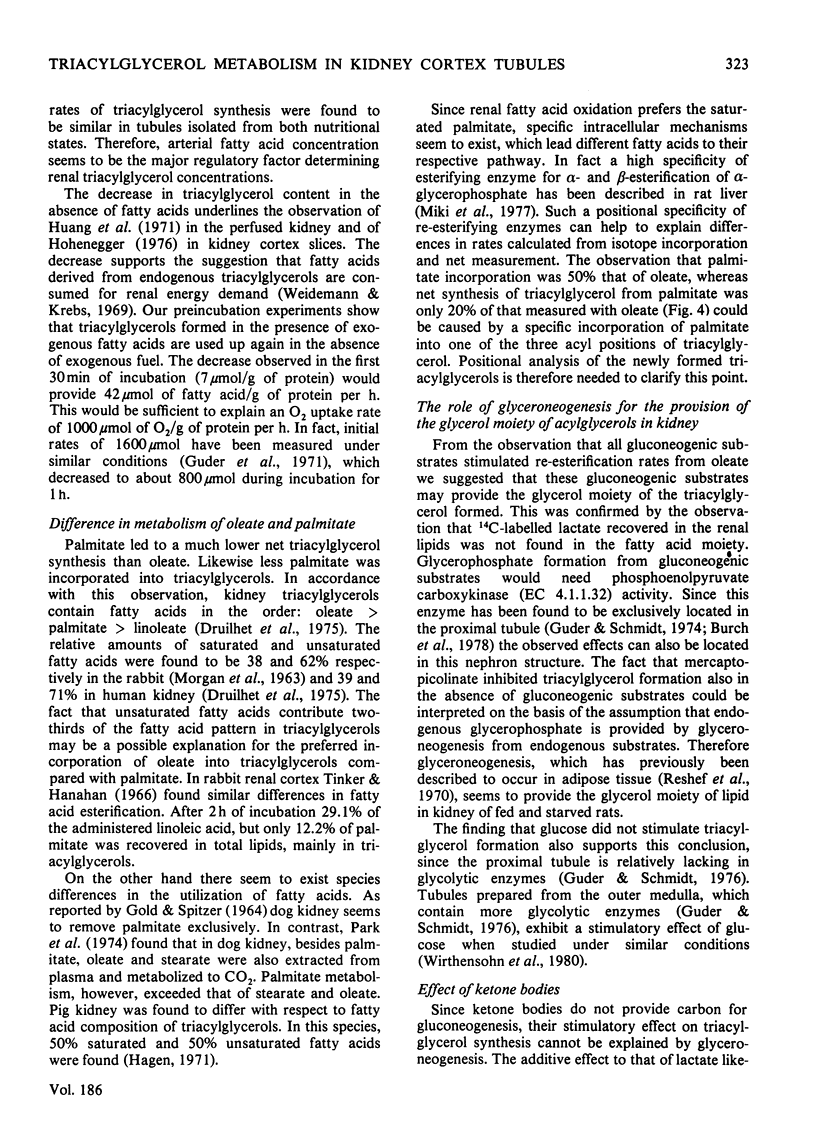
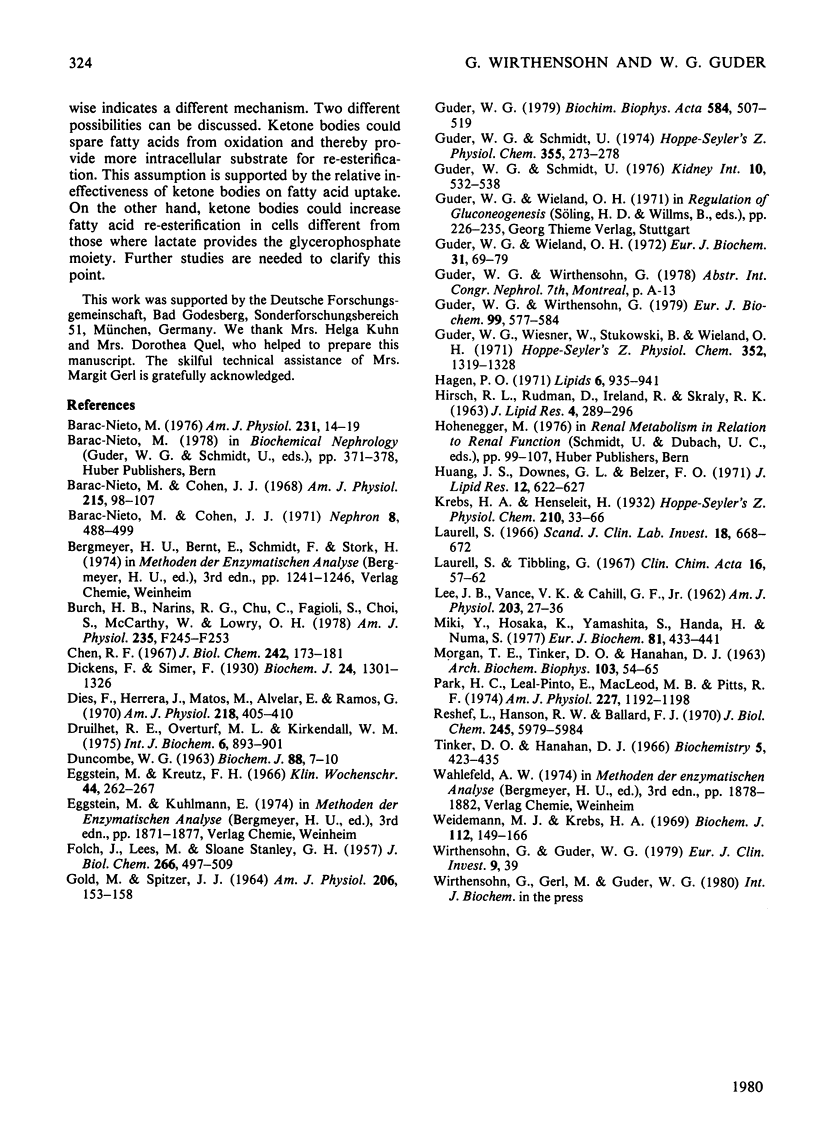
Selected References
These references are in PubMed. This may not be the complete list of references from this article.
- Barac-Nieto M., Cohen J. J. Nonesterified fatty acid uptake by dog kidney: effects of probenecid and chlorothiazide. Am J Physiol. 1968 Jul;215(1):98–107. doi: 10.1152/ajplegacy.1968.215.1.98. [DOI] [PubMed] [Google Scholar]
- Barac-Nieto M., Cohen J. J. The metabolic fates of palmitate in the dog kidney in vivo. Evidence for incomplete oxidation. Nephron. 1971;8(5):488–499. doi: 10.1159/000179952. [DOI] [PubMed] [Google Scholar]
- Barac-Nieto M. Effects of lactate and glutamine on palmitate metabolism in rat kindey cortex. Am J Physiol. 1976 Jul;231(1):14–19. doi: 10.1152/ajplegacy.1976.231.1.14. [DOI] [PubMed] [Google Scholar]
- Burch H. B., Narins R. G., Chu C., Fagioli S., Choi S., McCarthy W., Lowry O. H. Distribution along the rat nephron of three enzymes of gluconeogenesis in acidosis and starvation. Am J Physiol. 1978 Sep;235(3):F246–F253. doi: 10.1152/ajprenal.1978.235.3.F246. [DOI] [PubMed] [Google Scholar]
- Chen R. F. Removal of fatty acids from serum albumin by charcoal treatment. J Biol Chem. 1967 Jan 25;242(2):173–181. [PubMed] [Google Scholar]
- Dickens F., Simer F. The metabolism of normal and tumour tissue: The respiratory quotient, and the relationship of respiration to glycolysis. Biochem J. 1930;24(5):1301–1326. doi: 10.1042/bj0241301. [DOI] [PMC free article] [PubMed] [Google Scholar]
- Dies F., Herrera J., Matos M., Avelar E., Ramos G. Substrate uptake by the dog kidney in vivo. Am J Physiol. 1970 Feb;218(2):405–410. doi: 10.1152/ajplegacy.1970.218.2.405. [DOI] [PubMed] [Google Scholar]
- Duncombe W. G. The colorimetric micro-determination of long-chain fatty acids. Biochem J. 1963 Jul;88(1):7–10. doi: 10.1042/bj0880007. [DOI] [PMC free article] [PubMed] [Google Scholar]
- Eggstein M., Kreutz F. H. Eine neue Bestimmung der Neutralfette im Blutserum und Gewebe. I. Prinzip, Durchführung und Besprechung der Methode. Klin Wochenschr. 1966 Mar 1;44(5):262–267. doi: 10.1007/BF01747716. [DOI] [PubMed] [Google Scholar]
- FOLCH J., LEES M., SLOANE STANLEY G. H. A simple method for the isolation and purification of total lipides from animal tissues. J Biol Chem. 1957 May;226(1):497–509. [PubMed] [Google Scholar]
- GOLD M., SPITZER J. J. METABOLISM OF FREE FATTY ACIDS BY MYOCARDIUM AND KIDNEY. Am J Physiol. 1964 Jan;206:153–158. doi: 10.1152/ajplegacy.1964.206.1.153. [DOI] [PubMed] [Google Scholar]
- Guder W. G., Schmidt U. The localization of gluconeogenesis in rat nephron. Determination of phosphoenolpyruvate carboxykinase in microdissected tubules. Hoppe Seylers Z Physiol Chem. 1974 Mar;355(3):273–278. doi: 10.1515/bchm2.1974.355.1.273. [DOI] [PubMed] [Google Scholar]
- Guder W. G. Stimulation of renal gluconeogenesis by angiotensin II. Biochim Biophys Acta. 1979 May 16;584(3):507–519. doi: 10.1016/0304-4165(79)90123-5. [DOI] [PubMed] [Google Scholar]
- Guder W. G., Wieland O. H. Metabolism of isolated kidney tubules. Additive effects of parathyroid hormone and free-fatty acids on renal gluconeogenesis. Eur J Biochem. 1972 Nov 21;31(1):69–79. doi: 10.1111/j.1432-1033.1972.tb02502.x. [DOI] [PubMed] [Google Scholar]
- Guder W. G., Wirthensohn G. Metabolism of isolated kidney tubules. Interactions between lactate, glutamine and oleate metabolism. Eur J Biochem. 1979 Sep;99(3):577–584. doi: 10.1111/j.1432-1033.1979.tb13290.x. [DOI] [PubMed] [Google Scholar]
- Guder W., Wiesner W., Stukowski B., Wieland O. Metabolism of isolated kidney tubules. Oxygen consumption, gluconeogenesis and the effect of cyclic nucleotides in tubules from starved rats. Hoppe Seylers Z Physiol Chem. 1971 Oct;352(10):1319–1328. doi: 10.1515/bchm2.1971.352.2.1319. [DOI] [PubMed] [Google Scholar]
- HIRSCH R. L., RUDMAN D., IRELAND R., SKRALY R. MOVEMENT OF FREE FATTY ACIDS INTO AND OUT OF THE BLOOD STREAM IN NORMAL RABBITS AND IN RABBITS INJECTED SUBCUTANEOUSLY WITH THE PITUITARY ADIPOKINETIC SUBSTANCES, FRACTION H AND ADRENOCORTICOTROPIN. J Lipid Res. 1963 Jul;4:289–296. [PubMed] [Google Scholar]
- Hagen P. O. Structural comparison between triglycerides and phospholipids from pig kidney. Lipids. 1971 Dec;6(12):935–941. doi: 10.1007/BF02531178. [DOI] [PubMed] [Google Scholar]
- Huang J. S., Downes G. L., Belzer F. O. Utilization of fatty acids in perfused hypothermic dog kidney. J Lipid Res. 1971 Sep;12(5):622–627. [PubMed] [Google Scholar]
- LEE J. B., VANCE V. K., CAHILL G. F., Jr Metabolism of C14-labeled substrates by rabbit kidney cortex and medulla. Am J Physiol. 1962 Jul;203:27–36. doi: 10.1152/ajplegacy.1962.203.1.27. [DOI] [PubMed] [Google Scholar]
- Laurell S. A method for routine determination of plasma triglycerides. Scand J Clin Lab Invest. 1966;18(6):668–672. doi: 10.3109/00365516609049052. [DOI] [PubMed] [Google Scholar]
- Laurell S., Tibbling G. Colorimetric micro-determination of free fatty acids in plasma. Clin Chim Acta. 1967 Apr;16(1):57–62. doi: 10.1016/0009-8981(67)90269-0. [DOI] [PubMed] [Google Scholar]
- MORGAN T. E., TINKER D. O., HANAHAN D. J. PHOSPHOLIPID METABOLISM IN KIDNEY. I. ISOLATION AND IDENTIFICATION OF LIPIDS OF RABBIT KIDNEY. Arch Biochem Biophys. 1963 Oct;103:54–65. doi: 10.1016/0003-9861(63)90009-2. [DOI] [PubMed] [Google Scholar]
- Miki Y., Hosaka K., Yamashita S., Handa H., Numa S. Acyl-acceptor specificities of 1-acylglycerolphosphate acyltransferase and 1-acylglycerophosphorylcholine acyltransferase resolved from rat liver microsomes. Eur J Biochem. 1977 Dec;81(3):433–441. doi: 10.1111/j.1432-1033.1977.tb11968.x. [DOI] [PubMed] [Google Scholar]
- Park H. C., Leal-Pinto E., MacLeod M. B., Pitts R. F. CO2 production from plasma free fatty acids by the intact functioning kidney of the dog. Am J Physiol. 1974 Nov;227(5):1192–1198. doi: 10.1152/ajplegacy.1974.227.5.1192. [DOI] [PubMed] [Google Scholar]
- Reshef L., Hanson R. W., Ballard F. J. A possible physiological role for glyceroneogenesis in rat adipose tissue. J Biol Chem. 1970 Nov 25;245(22):5979–5984. [PubMed] [Google Scholar]
- Tinker D. O., Hanahan D. J. Phospholipid metabolism in kidney. 3. Biosynthesis of phospholipids from radioactive precursors in rabbit renal cortex slices. Biochemistry. 1966 Feb;5(2):423–435. doi: 10.1021/bi00866a006. [DOI] [PubMed] [Google Scholar]
- Weidemann M. J., Krebs H. A. The fuel of respiration of rat kidney cortex. Biochem J. 1969 Apr;112(2):149–166. doi: 10.1042/bj1120149. [DOI] [PMC free article] [PubMed] [Google Scholar]