Abstract
The effects of Ca2+ and acetylcholine on the degradation and synthesis of phosphatidylinositol in rabbit vas deferens was studied in vitro by a pulse--chase technique and by measuring the content of the phospholipid in the tissue. Ca2+-dependent degradation of phosphatidylinositol was found in slices and homogenates prelabelled with myo-[2-3H]inositol. The phosphatidylinositol content of the slices also decreased by a Ca2+-dependent mechanism. On the other hand, removal of intracellular Ca2+ with the ionophore A23187 and EGTA increased the amount of phosphatidylinositol. These results indicate that the intracellular Ca2+ concentration has an important role in regulating the phosphatidylinositol content of the tissue. Increasing the extracellular K+ concentration, which causes an increase in plasma-membrane Ca2+ permeability, did not enhance phosphatidylinositol breakdown nor decrease its tissue content. However, phosphatidylinositol synthesis was clearly inhibited. After stimulation of the smooth muscle with acetylcholine, degradation of phosphatidylinositol was enhanced. Furthermore, the content of phosphatidylinositol in the tissue also decreased. These phenomena were evident even in the absence of Ca2+. The acetylcholine-induced degradation of phosphatidylinositol was blocked by the muscarinic antagonist atropine, but not by the nicotinic antagonist (+)-tubocurarine. The acetylcholine-induced decrease in the phosphatidylinositol content of the tissue led to the compensatory synthesis of phosphatidylinositol. Synthesis was separated from degradation in the same tissue. Compensatory synthesis was inhibited by acetylcholine. The degradation of phosphatidylinositol induced by acetylcholine was not inhibited by 8-bromoguanosine 3':5'-cyclic monophosphate, indicating that the degradative process was not mediated by an increase in the cyclic nucleotide.
Full text
PDF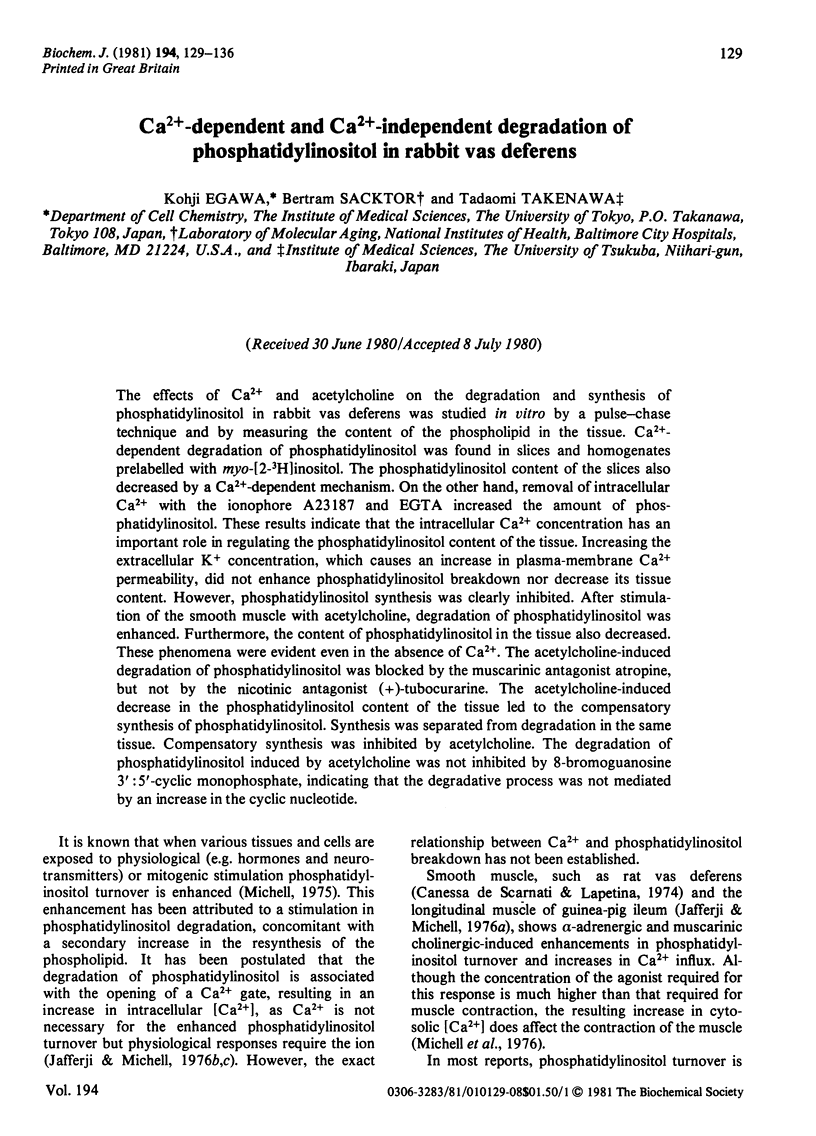
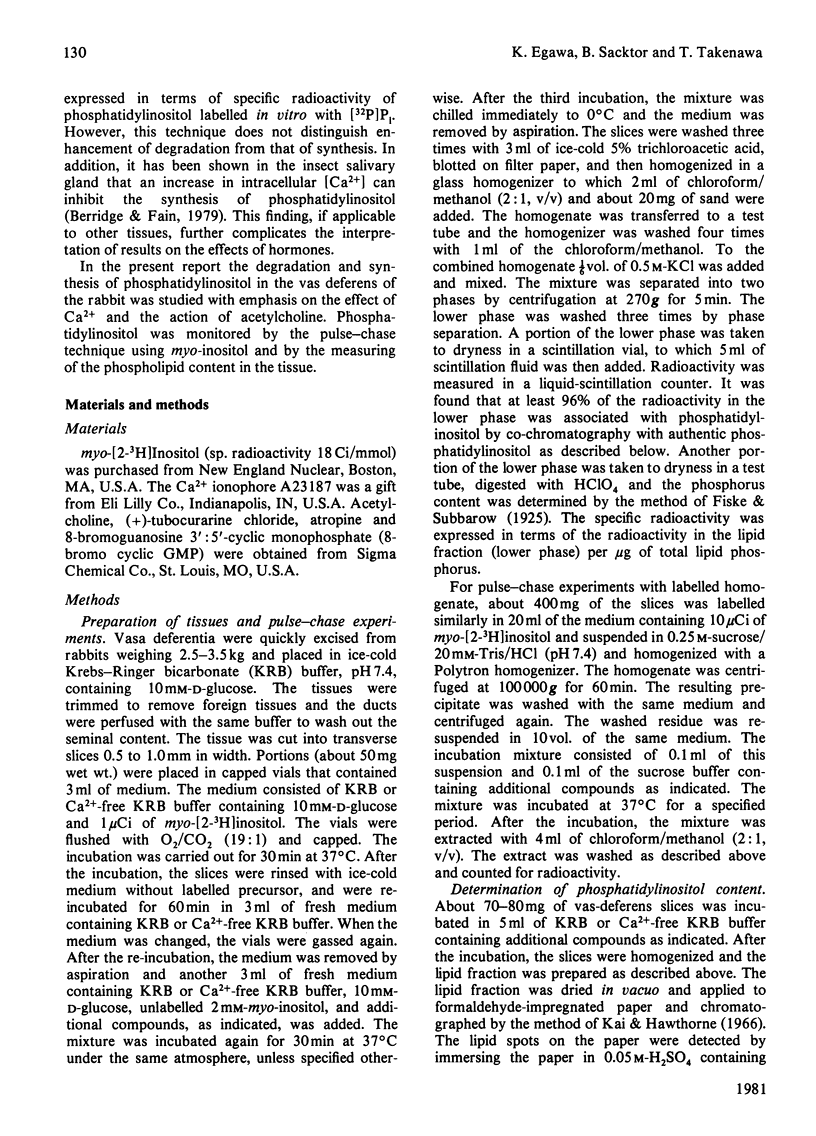
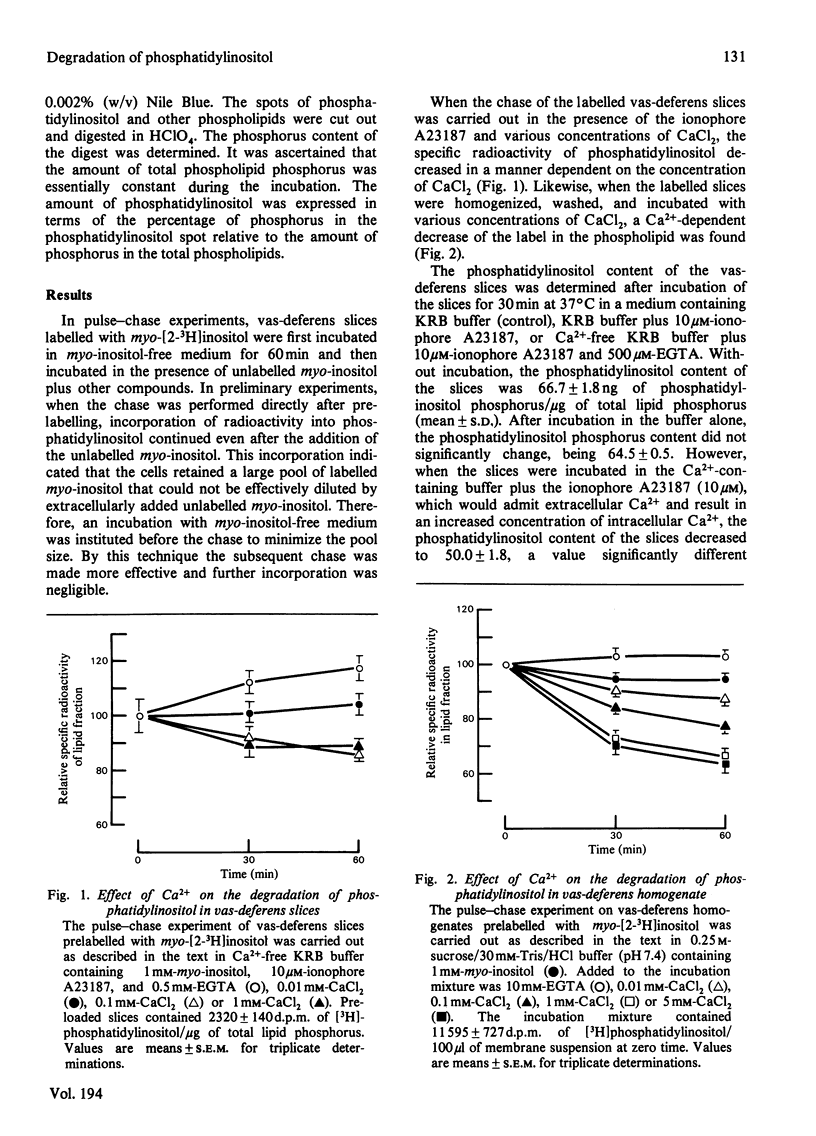
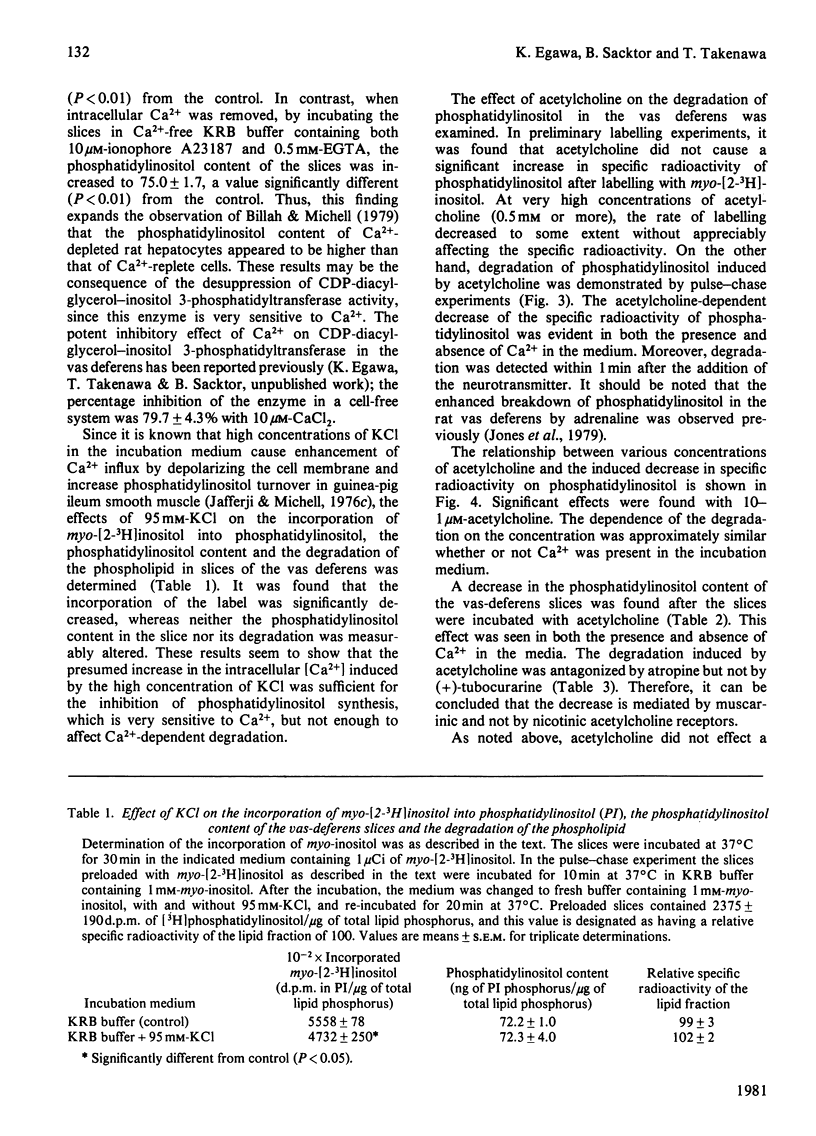
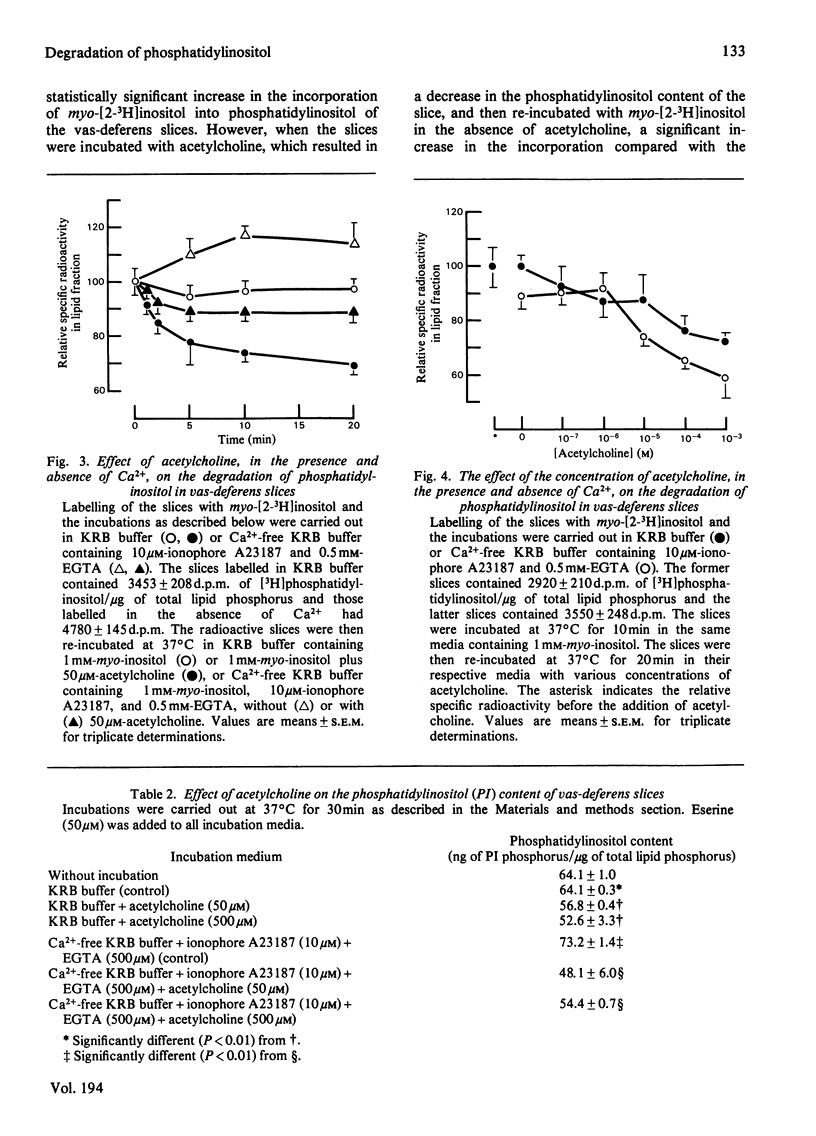
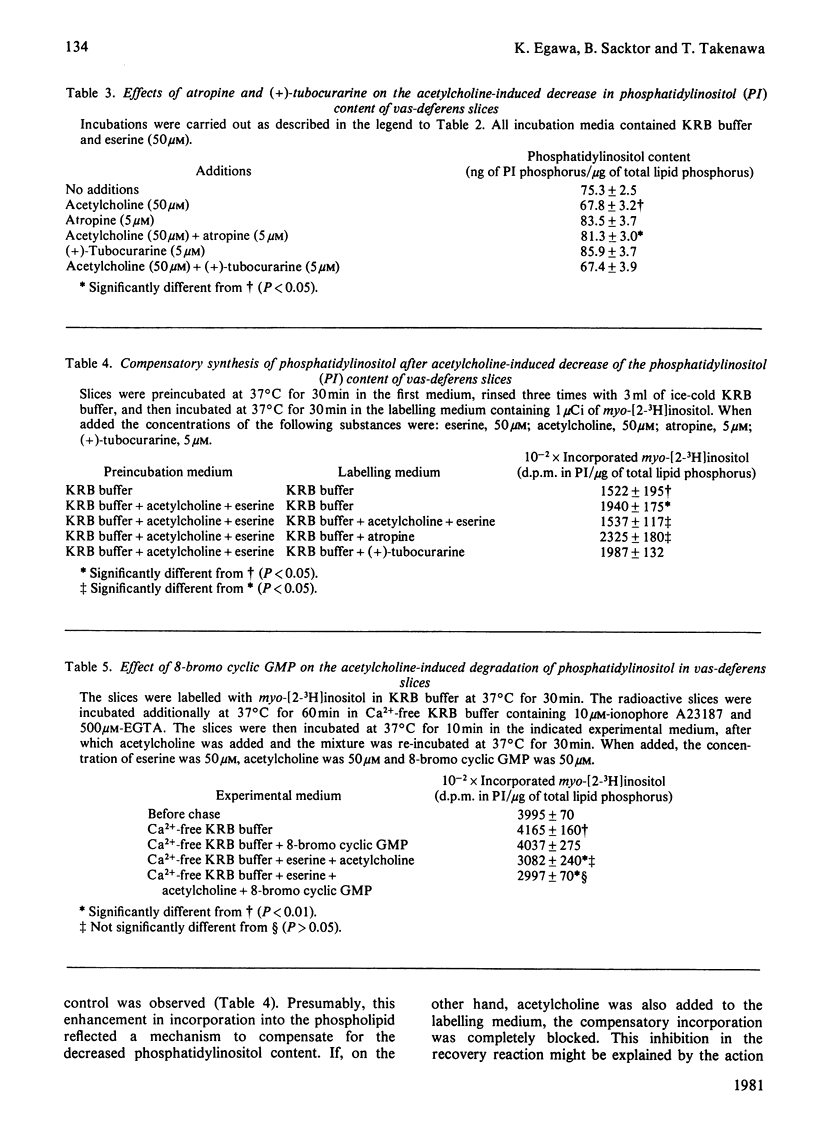
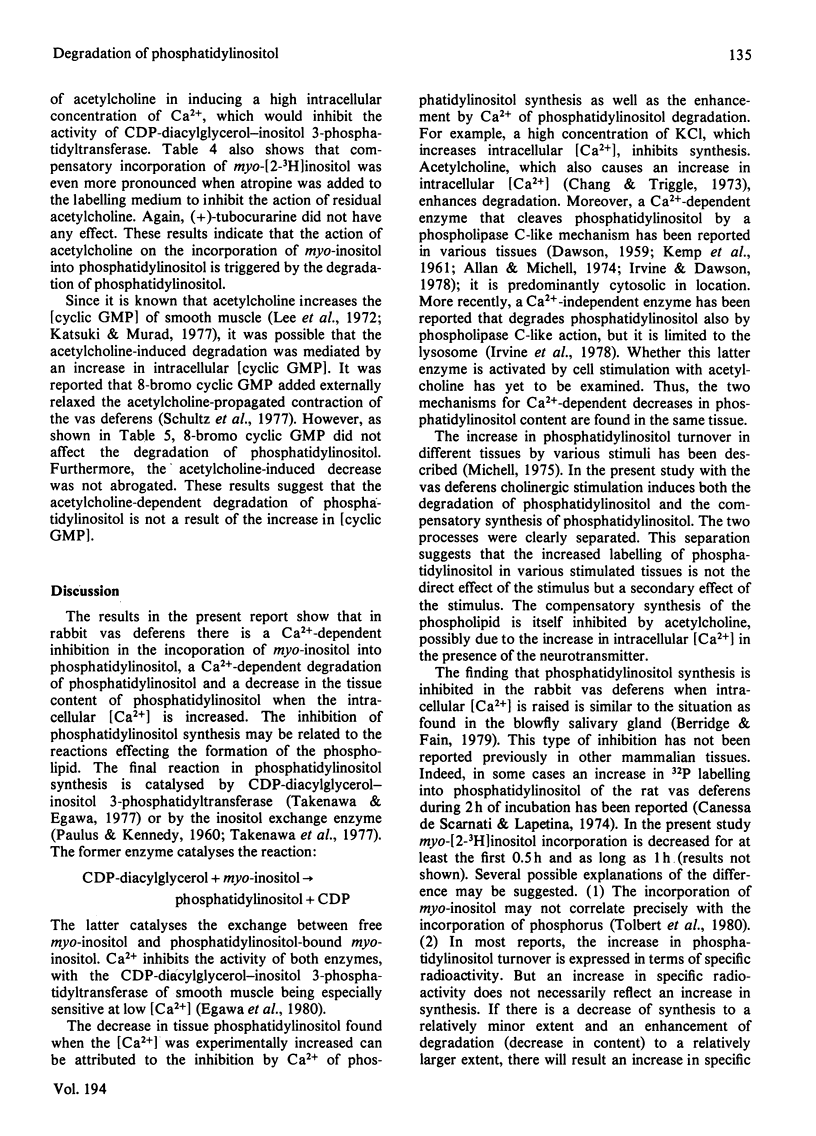
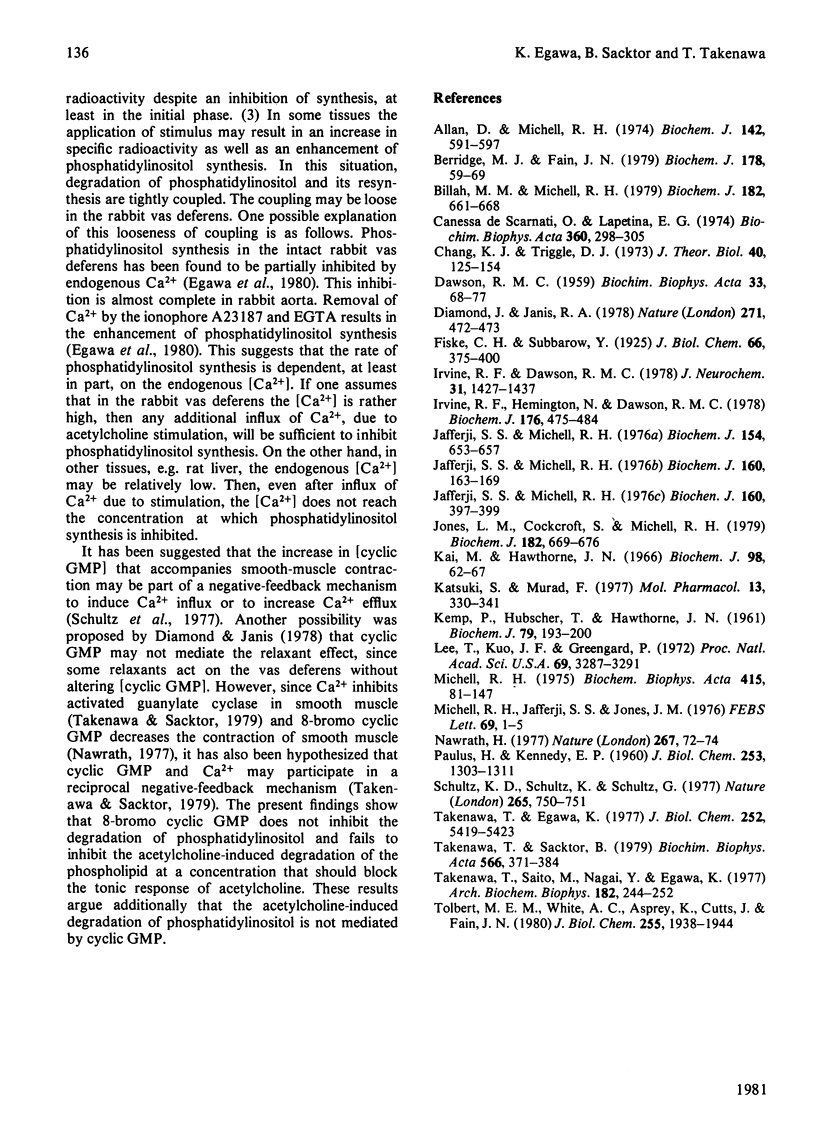
Selected References
These references are in PubMed. This may not be the complete list of references from this article.
- Allan D., Michell R. H. Phosphatidylinositol cleavage catalysed by the soluble fraction from lymphocytes. Activity at pH5.5 and pH7.0. Biochem J. 1974 Sep;142(3):591–597. doi: 10.1042/bj1420591. [DOI] [PMC free article] [PubMed] [Google Scholar]
- Berridge M. J., Fain J. N. Inhibition of phosphatidylinositol synthesis and the inactivation of calcium entry after prolonged exposure of the blowfly salivary gland to 5-hydroxytryptamine. Biochem J. 1979 Jan 15;178(1):59–69. doi: 10.1042/bj1780059. [DOI] [PMC free article] [PubMed] [Google Scholar]
- Billah M. M., Michell R. H. Phosphatidylinositol metabolism in rat hepatocytes stimulated by glycogenolytic hormones. Effects of angiotensin, vasopressin, adrenaline, ionophore A23187 and calcium-ion deprivation. Biochem J. 1979 Sep 15;182(3):661–668. doi: 10.1042/bj1820661. [DOI] [PMC free article] [PubMed] [Google Scholar]
- Canessa de Scarnati O., Lapetina E. G. Adrenergic stimulation of phosphatidylinositol labelling in rat vas deferens. Biochim Biophys Acta. 1974 Sep 19;360(3):298–305. doi: 10.1016/0005-2760(74)90059-9. [DOI] [PubMed] [Google Scholar]
- Chang K. J., Triggle D. J. Quantitative aspects of drug-receptor interactions. I. Ca2+ and cholinergic receptor activation in smooth muscle: a basic model for drug-receptor interactions. J Theor Biol. 1973 Jul;40(1):125–154. doi: 10.1016/0022-5193(73)90168-9. [DOI] [PubMed] [Google Scholar]
- DAWSON R. M. Studies on the enzymic hydrolysis of monophosphoinositide by phospholipase preparations from P. notatum and ox pancreas. Biochim Biophys Acta. 1959 May;33(1):68–77. doi: 10.1016/0006-3002(59)90499-8. [DOI] [PubMed] [Google Scholar]
- Diamond J., Janis R. A. Increases in cyclic GMP levels may not mediate relaxant effects of sodium nitroprusside, verapamil and hydralazine in rat vas deferens. Nature. 1978 Feb 2;271(5644):472–473. doi: 10.1038/271472a0. [DOI] [PubMed] [Google Scholar]
- Irvine R. F., Dawson R. M. The distribution of calcium-dependent phosphatidylinositol-specific phosphodiesterase in rat brain. J Neurochem. 1978 Dec;31(6):1427–1434. doi: 10.1111/j.1471-4159.1978.tb06568.x. [DOI] [PubMed] [Google Scholar]
- Irvine R. F., Hemington N., Dawson R. M. The hydrolysis of phosphatidylinositol by lysosomal enzymes of rat liver and brain. Biochem J. 1978 Nov 15;176(2):475–484. doi: 10.1042/bj1760475. [DOI] [PMC free article] [PubMed] [Google Scholar]
- Jafferji S. S., Michell R. H. Effects of calcium-antagonistic drugs on the stimulation by carbamoylcholine and histamine of phosphatidylinositol turnover in longitudinal smooth muscle of guinea-pig ileum. Biochem J. 1976 Nov 15;160(2):163–169. doi: 10.1042/bj1600163. [DOI] [PMC free article] [PubMed] [Google Scholar]
- Jafferji S. S., Michell R. H. Investigation of the relationship between cell-surface calcium-ion gating and phosphatidylinositol turnover by comparison of the effects of elevated extracellular potassium ion concentration on ileium smooth muscle and pancreas. Biochem J. 1976 Nov 15;160(2):397–399. doi: 10.1042/bj1600397. [DOI] [PMC free article] [PubMed] [Google Scholar]
- Jafferji S. S., Michell R. H. Muscarinic cholinergic stimulation of phosphatidylinositol turnover in the longitudinal smooth muscle of guinea-pig ileum. Biochem J. 1976 Mar 15;154(3):653–657. doi: 10.1042/bj1540653. [DOI] [PMC free article] [PubMed] [Google Scholar]
- Jones L. M., Cockcroft S., Michell R. H. Stimulation of phosphatidylinositol turnover in various tissues by cholinergic and adrenergic agonists, by histamine and by caerulein. Biochem J. 1979 Sep 15;182(3):669–676. doi: 10.1042/bj1820669. [DOI] [PMC free article] [PubMed] [Google Scholar]
- KEMP P., HUBSCHER G., HAWTHORNE J. N. Phosphoinositides. 3. Enzymic hydrolysis of inositol-containing phospholipids. Biochem J. 1961 Apr;79:193–200. doi: 10.1042/bj0790193. [DOI] [PMC free article] [PubMed] [Google Scholar]
- Kai M., Hawthorne J. N. Incorporation of injected [32P] phosphate into the phosphoinositides of subcellular fractions from young rat brain. Biochem J. 1966 Jan;98(1):62–67. doi: 10.1042/bj0980062. [DOI] [PMC free article] [PubMed] [Google Scholar]
- Katsuki S., Murad F. Regulation of adenosine cyclic 3',5'-monophosphate and guanosine cyclic 3',5'-monophosphate levels and contractility in bovine tracheal smooth muscle. Mol Pharmacol. 1977 Mar;13(2):330–341. [PubMed] [Google Scholar]
- Lee T. P., Kuo J. F., Greengard P. Role of muscarinic cholinergic receptors in regulation of guanosine 3':5'-cyclic monophosphate content in mammalian brain, heart muscle, and intestinal smooth muscle. Proc Natl Acad Sci U S A. 1972 Nov;69(11):3287–3291. doi: 10.1073/pnas.69.11.3287. [DOI] [PMC free article] [PubMed] [Google Scholar]
- Michell R. H. Inositol phospholipids and cell surface receptor function. Biochim Biophys Acta. 1975 Mar 25;415(1):81–47. doi: 10.1016/0304-4157(75)90017-9. [DOI] [PubMed] [Google Scholar]
- Michell R. H., Jafferji S. S., Jones L. M. Receptor occupancy dose--response curve suggests that phosphatidyl-inositol breakdown may be intrinsic to the mechanism of the muscarinic cholinergic receptor. FEBS Lett. 1976 Oct 15;69(1):1–5. doi: 10.1016/0014-5793(76)80640-0. [DOI] [PubMed] [Google Scholar]
- Nawrath H. Does cyclic GMP mediate the negative inotropic effect of acetylcholine in the heart? Nature. 1977 May 5;267(5606):72–74. doi: 10.1038/267072a0. [DOI] [PubMed] [Google Scholar]
- PAULUS H., KENNEDY E. P. The enzymatic synthesis of inositol monophosphatide. J Biol Chem. 1960 May;235:1303–1311. [PubMed] [Google Scholar]
- Schultz K., Schultz K., Schultz G. Sodium nitroprusside and other smooth muscle-relaxants increase cyclic GMP levels in rat ductus deferens. Nature. 1977 Feb 24;265(5596):750–751. doi: 10.1038/265750a0. [DOI] [PubMed] [Google Scholar]
- Takenawa T., Egawa K. CDP-diglyceride:inositol transferase from rat liver. Purification and properties. J Biol Chem. 1977 Aug 10;252(15):5419–5423. [PubMed] [Google Scholar]
- Takenawa T., Sacktor B. Tissue-specific effects of divalent cations and activators on soluble guanylate cyclase. Biochim Biophys Acta. 1979 Feb 9;566(2):371–384. doi: 10.1016/0005-2744(79)90041-x. [DOI] [PubMed] [Google Scholar]
- Takenawa T., Saito M., Nagai Y., Egawa K. Solubilization of the enzyme catalyzing CDP-diglyceride-independent incorporation of myo-inositol into phosphatidyl inositol and its comparison to CDP-diglyceride:inositol transferase. Arch Biochem Biophys. 1977 Jul;182(1):244–250. doi: 10.1016/0003-9861(77)90304-6. [DOI] [PubMed] [Google Scholar]
- Tolbert M. E., White A. C., Aspry K., Cutts J., Fain J. N. Stimulation by vasopressin and alpha-catecholamines of phosphatidylinositol formation in isolated rat liver parenchymal cells. J Biol Chem. 1980 Mar 10;255(5):1938–1944. [PubMed] [Google Scholar]