Abstract
1. The hydrolyses of the p-nitrophenyl esters of N-benzyloxycarbonylglycine, alpha-N-benzyloxycarbonyl-L-lysine and N-methoxycarbonyl-L-phenylalanylglycine catalysed by papain (EC 3.4.22.2) have been studied in solvents having a variable composition of 2H2O and H2O. 2. kcat., which represents deacylation in the papain-catalysed hydrolysis of reactive esters, is some 2.3-fold less in 2H2O compared with H2O. The magnitude of kcat. has been determined as a function of the 2H atom fraction of the solvent. 3. Both linear and non-linear methods of least-square regression analysis have been applied to the data in order to obtain best-fit parameter values for several three-parameter models which express kcat. in terms of the 2H atom fraction of the solvent. These models represent some possible modes of restructuring of the active site protonic configuration consequent upon transition state formation. 4. The results of curve fitting reveal an essentially linear dependence of kcat. upon the 2H atom fraction, and it may therefore be concluded that the isotope effect originates from a single proton which is in the process of transfer in the transition state. 5. It is postulated on the basis of this and other evidence that the mobile proton is transferred from an attacking water molecule to the imidazole side chain of His-159 during tetrahedral intermediate formation. This has the effect of stabilizing the transition state and promoting catalysis. The role of His-159 in deacylation is therefore to provide general base catalysis. 6. Models that involve two or more protons, such as a two-proton relay system analogous to that proposed for the serine proteinases, or a multiproton 'medium' effect, are considered unlikely on the basis of the data reported in this paper. 7. A more detailed examination of possible transition state structures reveals that the only structure compatible with available experimental data and consistent with certain theoretical predictions is one in which the proton translocated in concern with reorganization of the heavy atom framework. In addition, the transition state vibrations of the mobile proton are strongly coupled to those of the heavy atoms. These properties of the transition state are also manifest in the transition state for the deacylation of serine proteinases.
Full text
PDF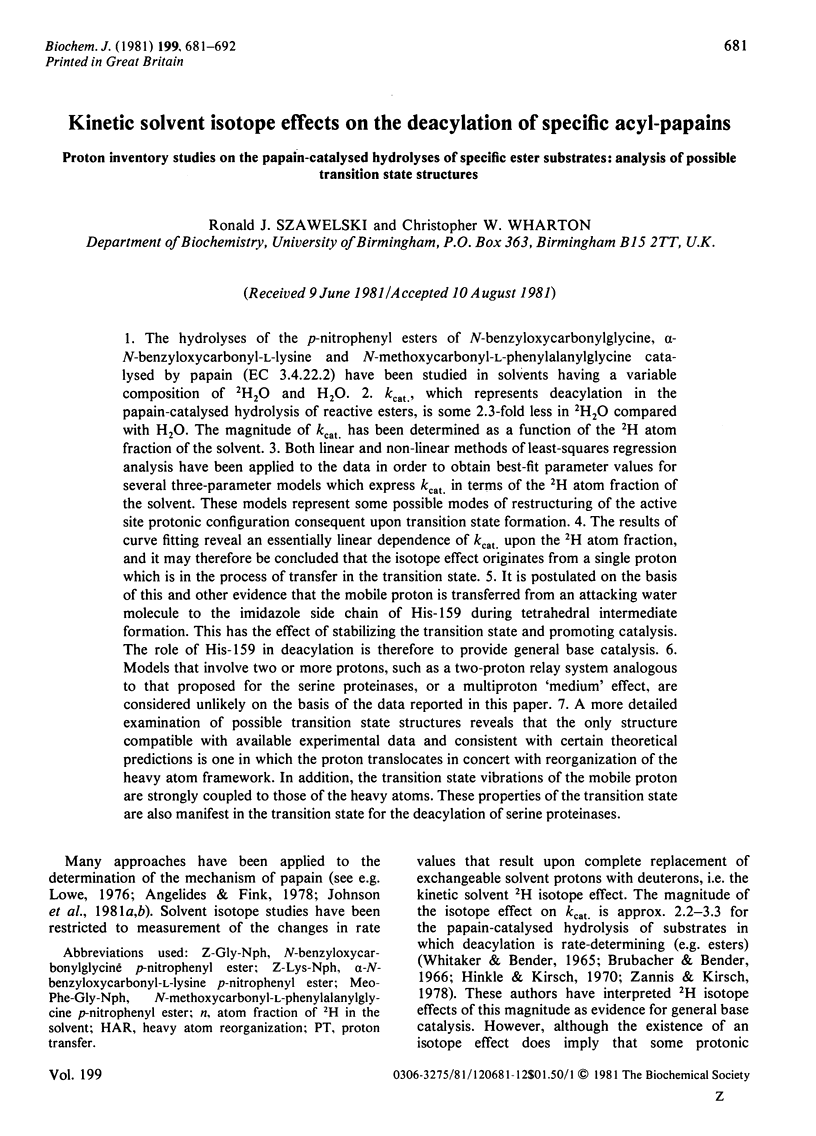
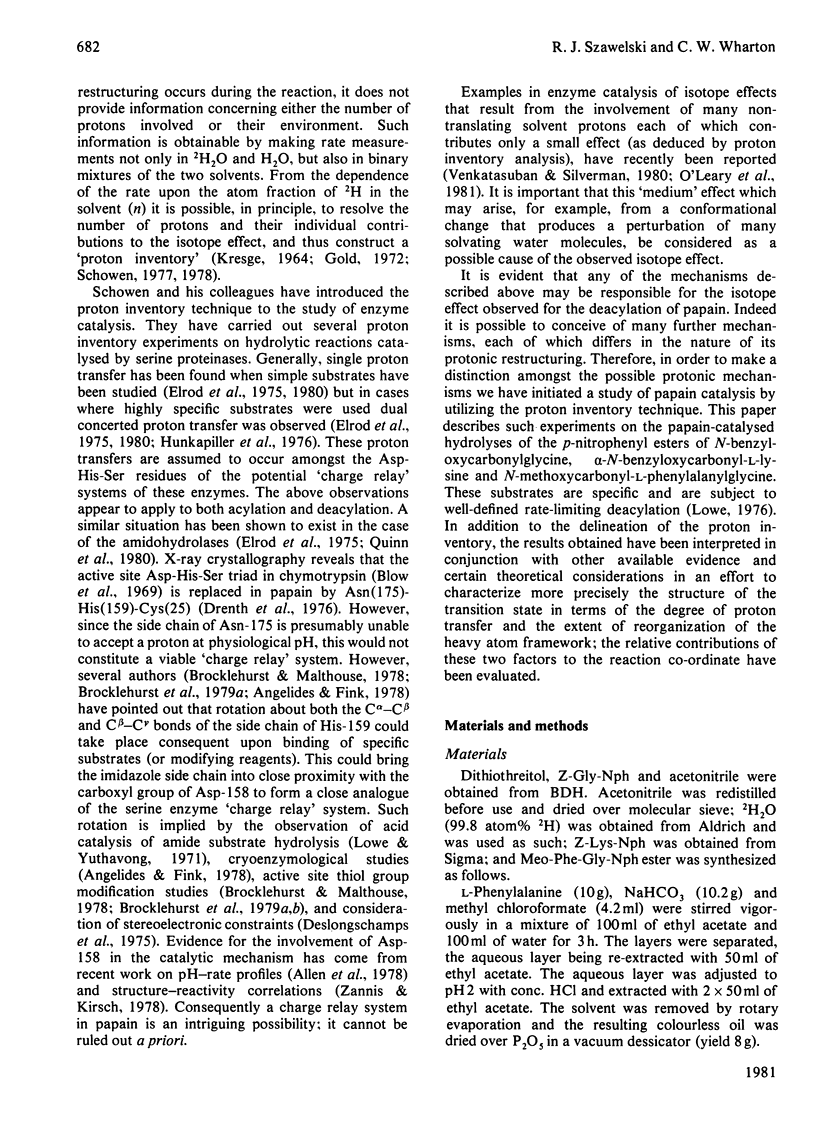
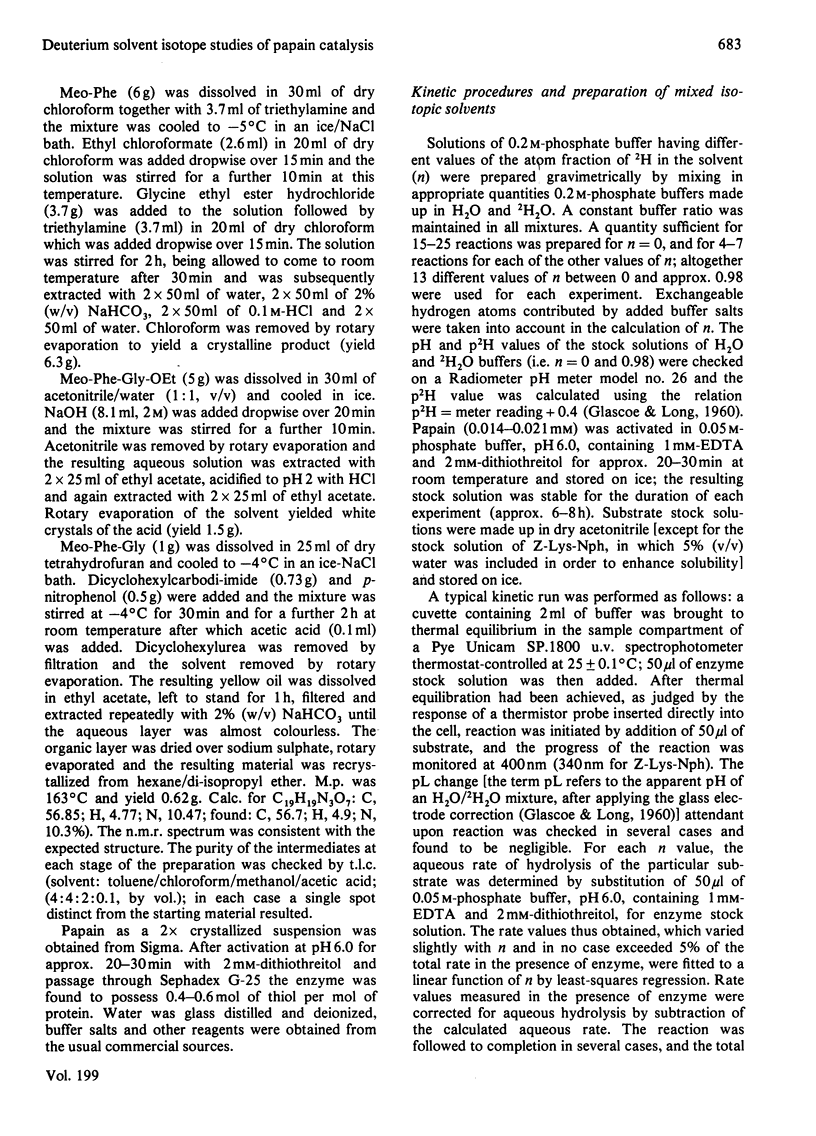
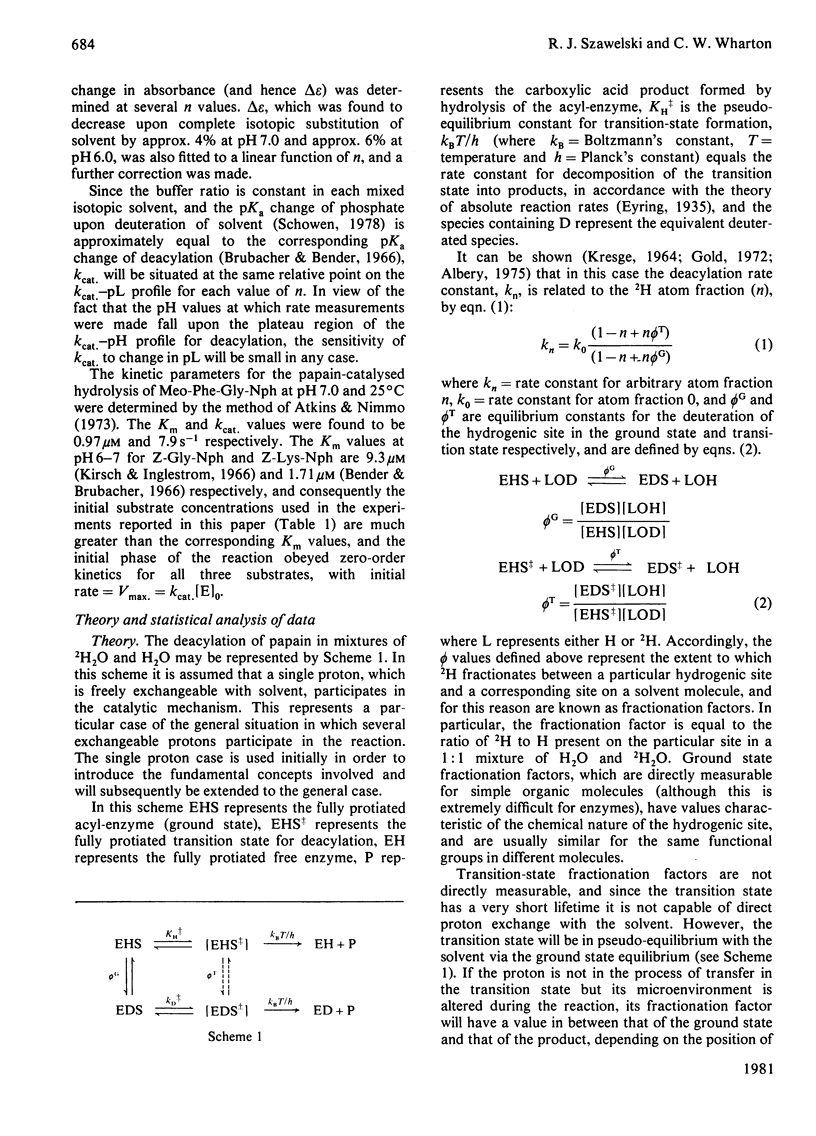
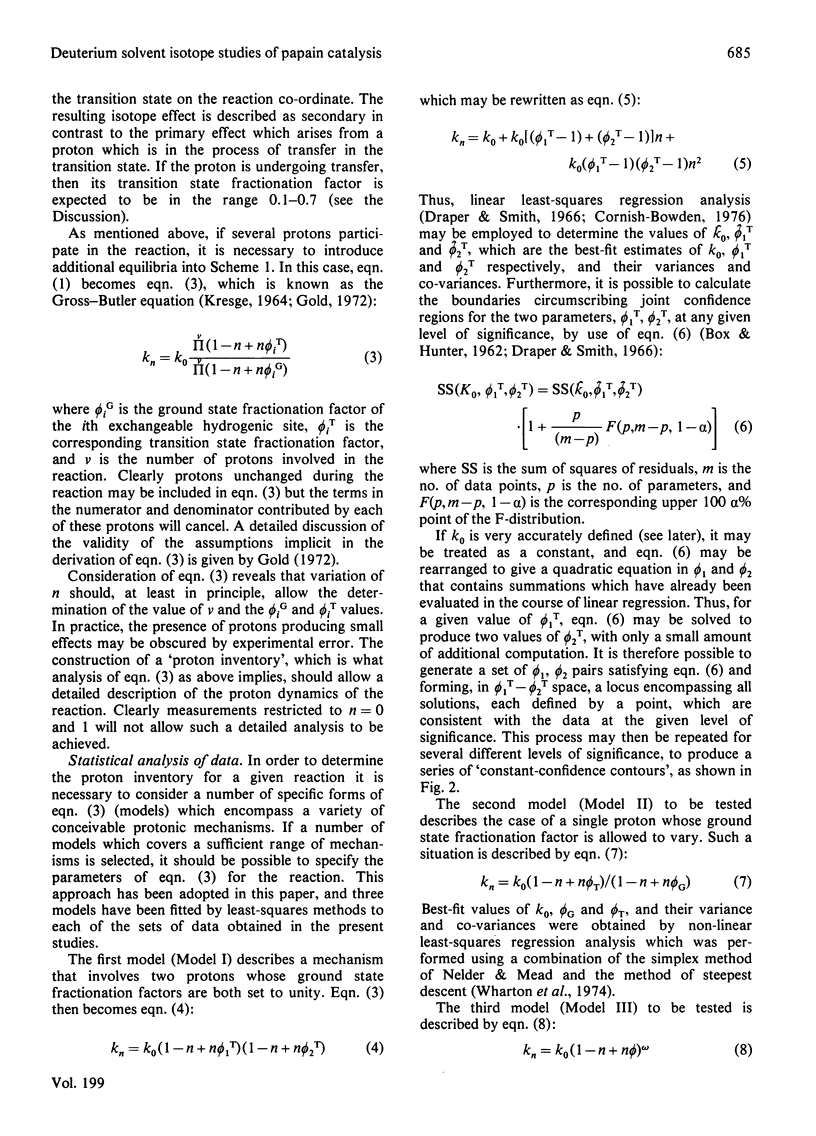
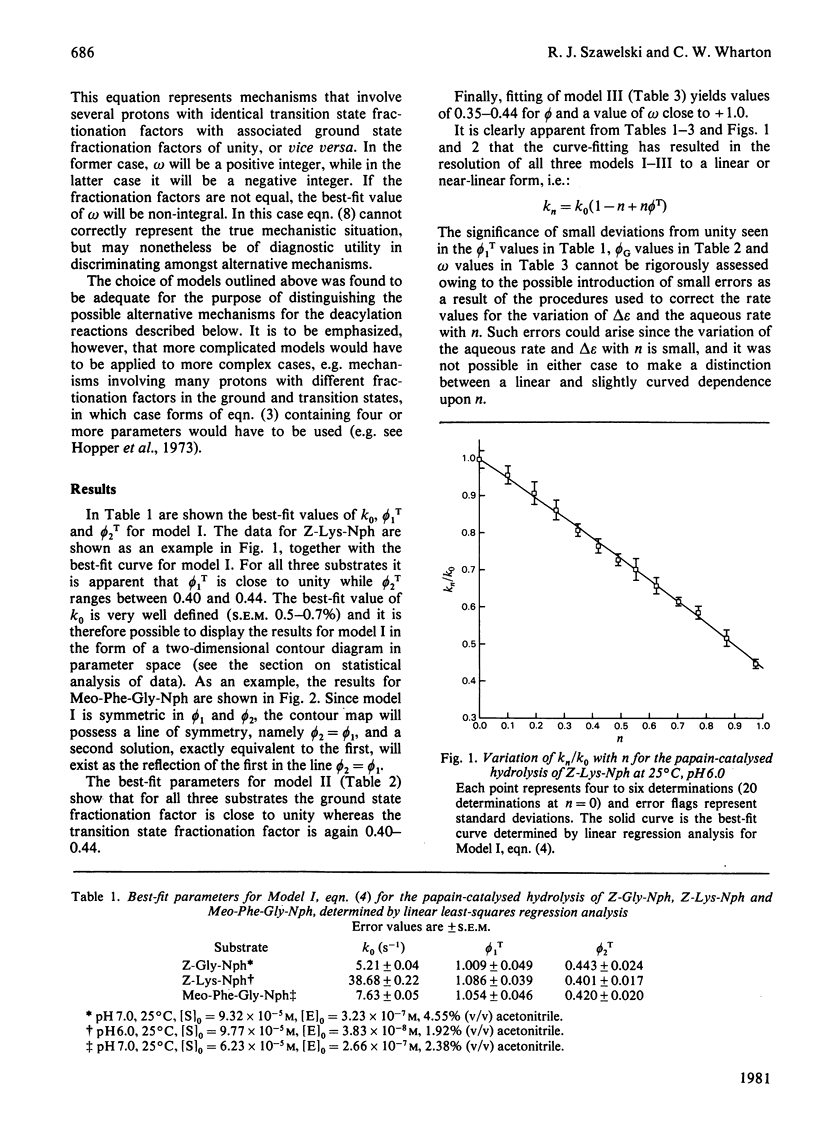
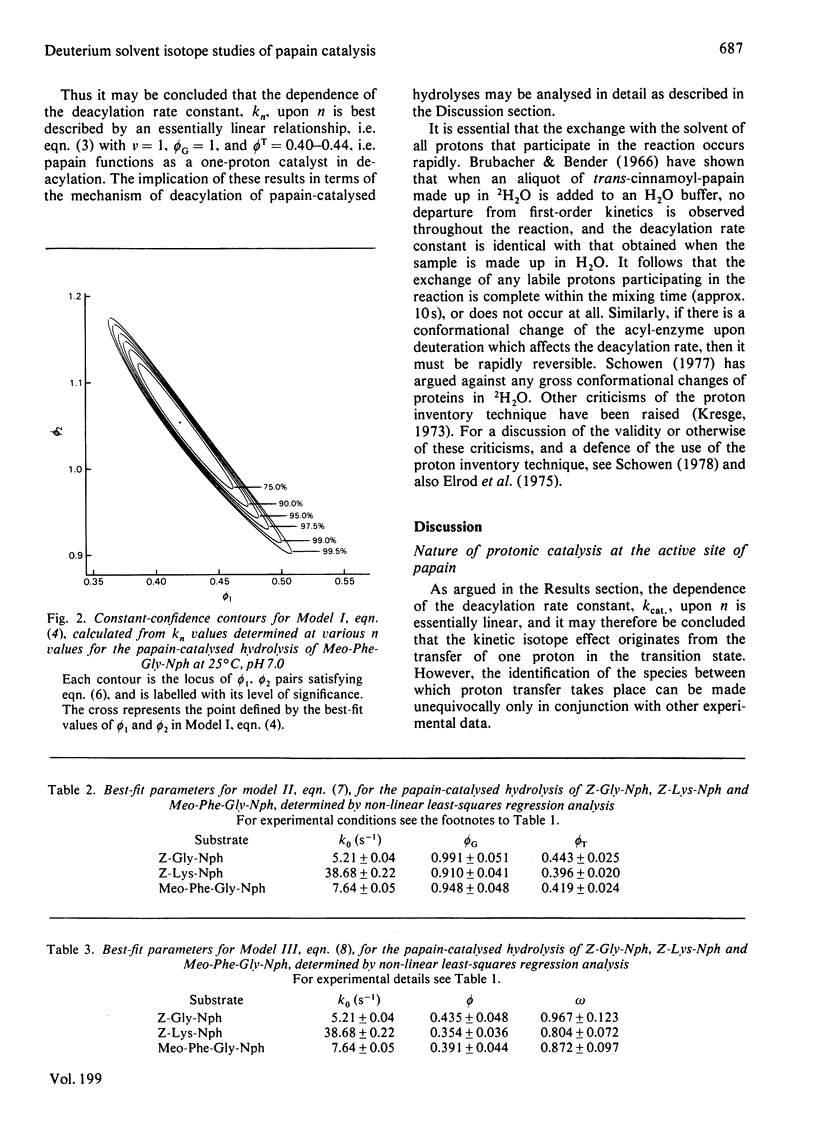
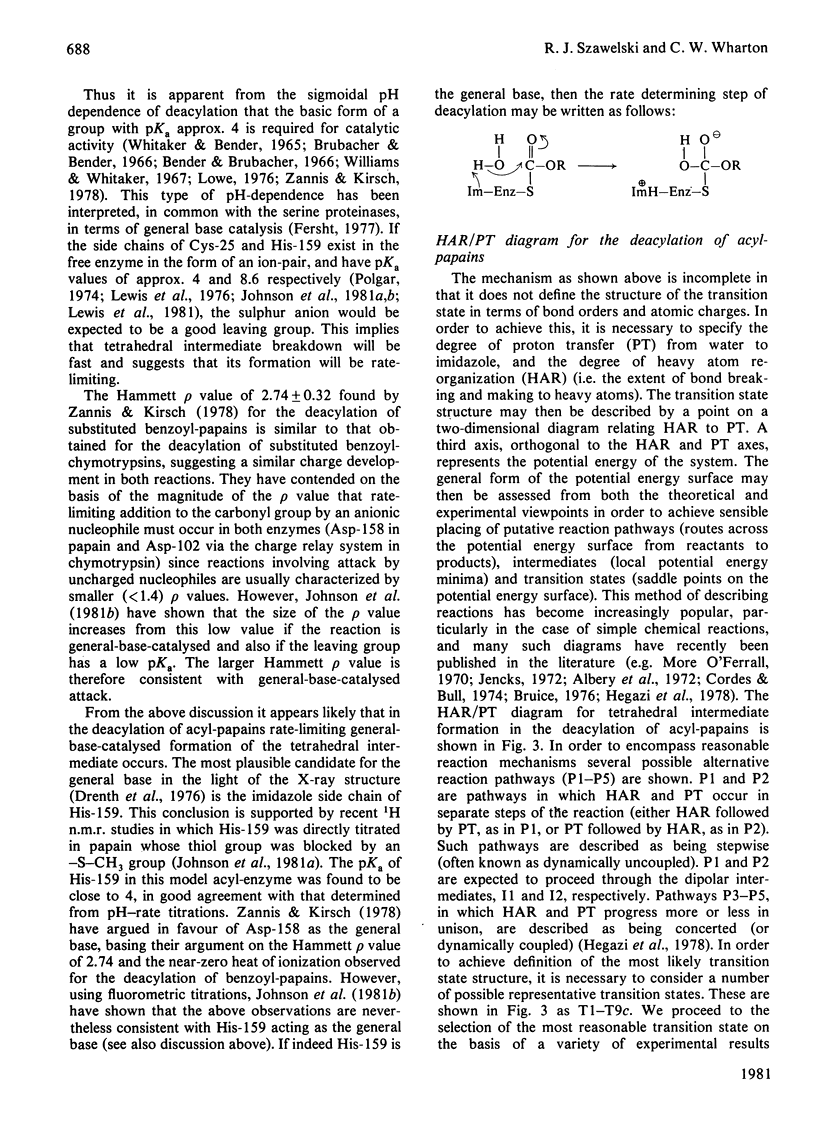
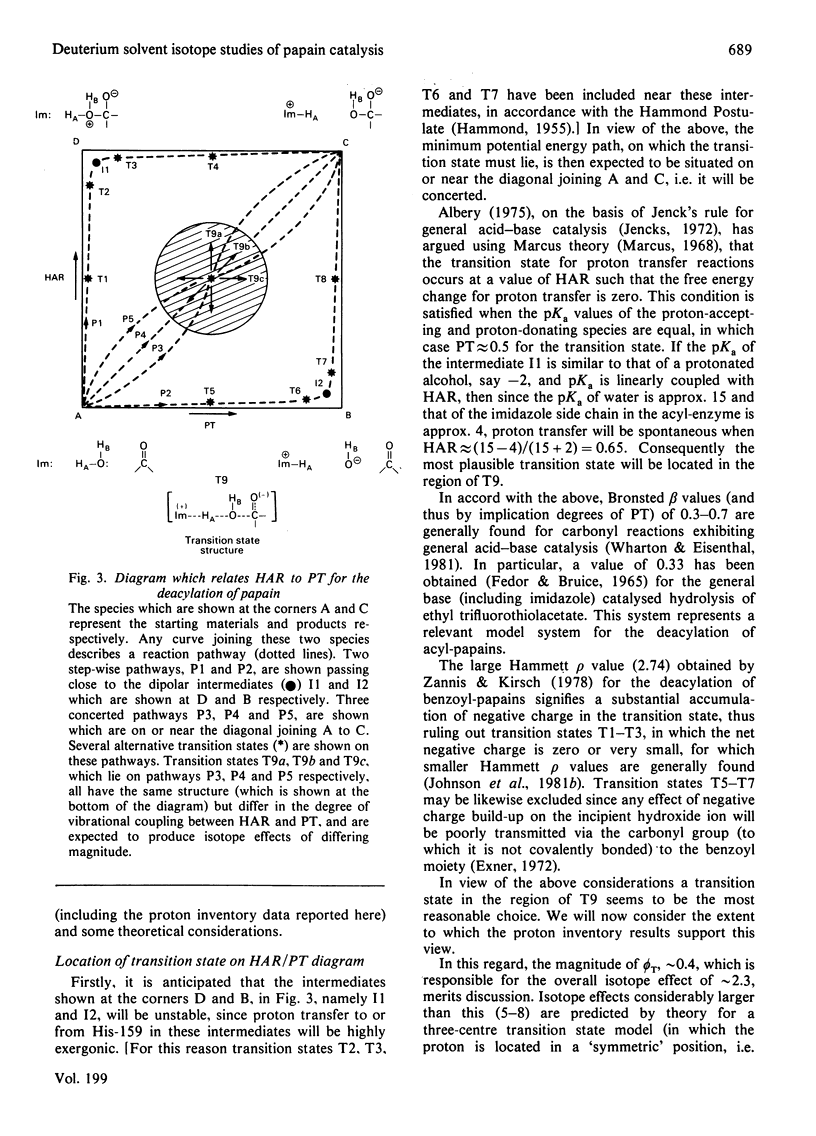
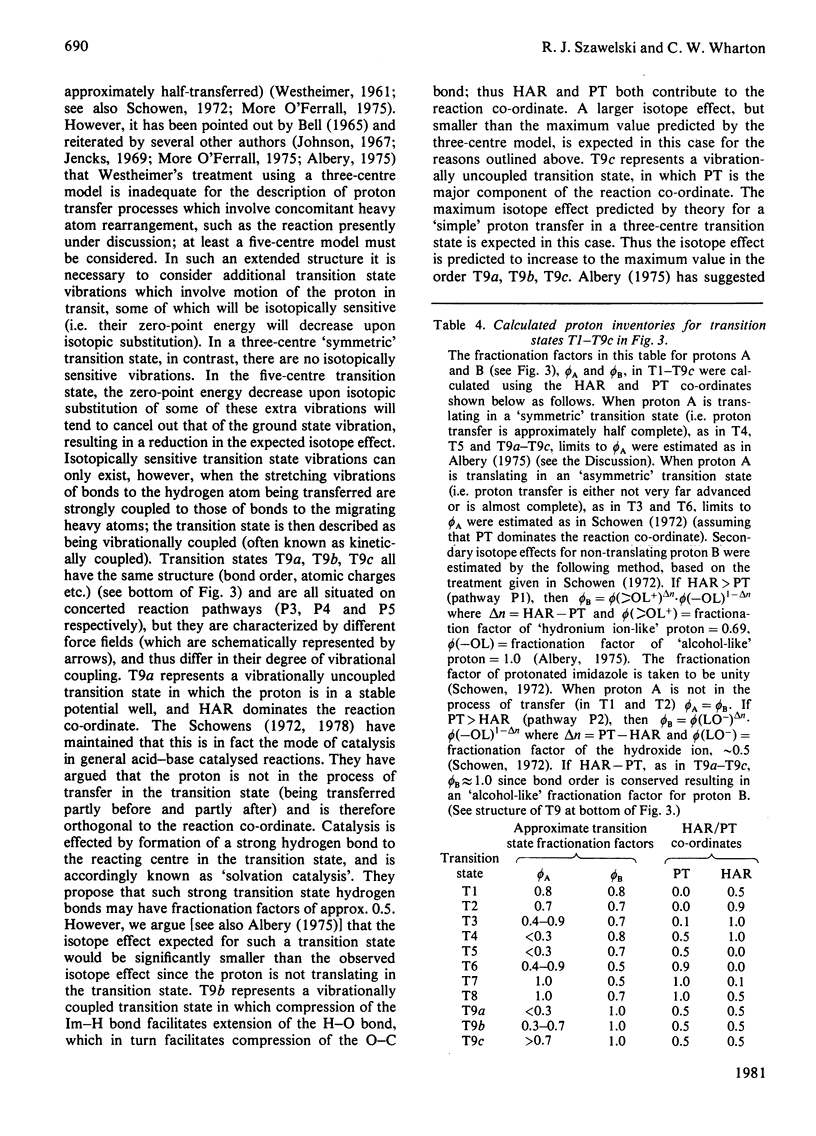
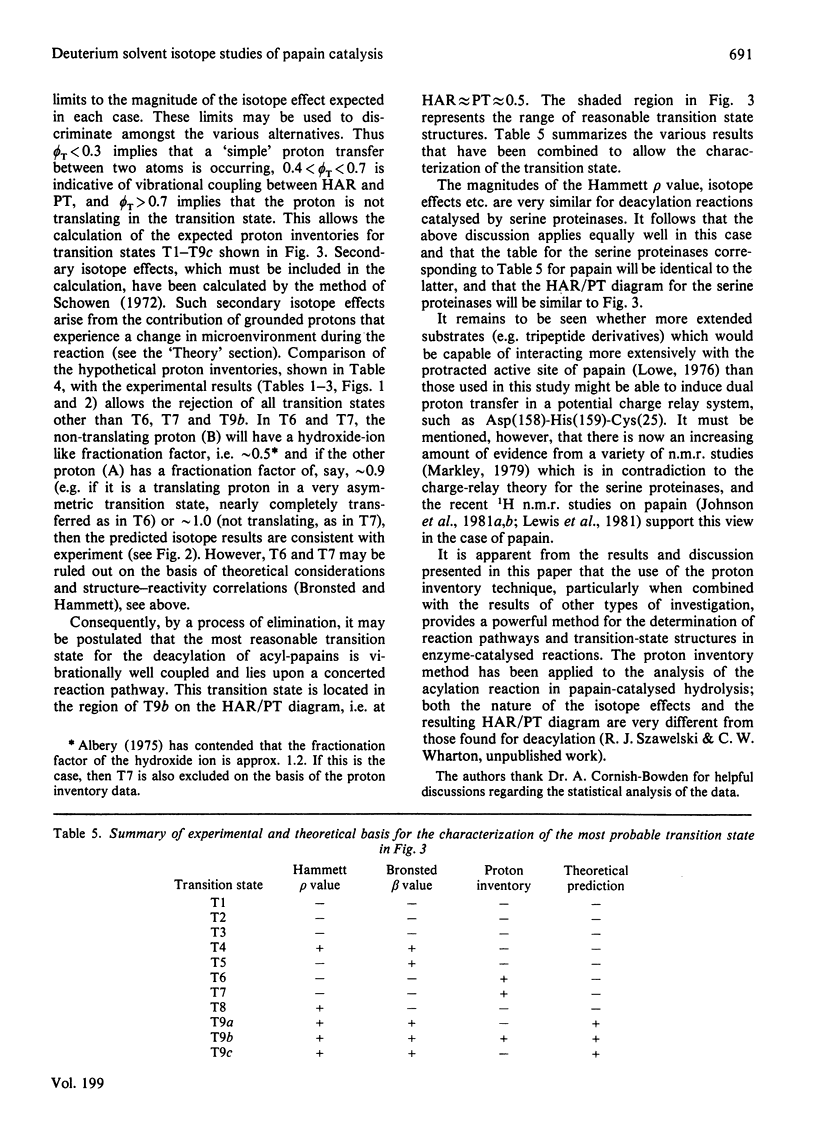
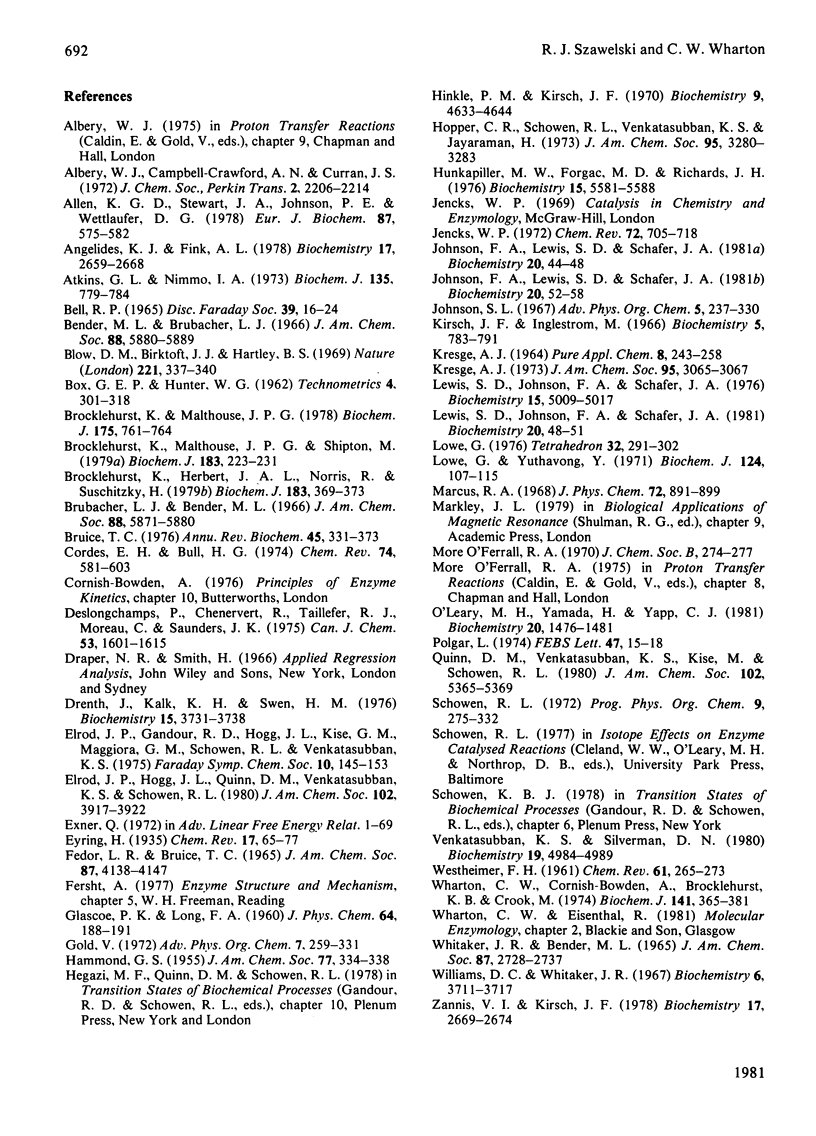
Selected References
These references are in PubMed. This may not be the complete list of references from this article.
- Allen K. G., Stewart J. A., Johnson P. E., Wettlaufer D. G. Identification of the functional ionic groups of papain by pH/rate profile analysis. Eur J Biochem. 1978 Jul 3;87(3):575–582. doi: 10.1111/j.1432-1033.1978.tb12409.x. [DOI] [PubMed] [Google Scholar]
- Angelides K. J., Fink A. L. Cryoenzymology of papain: reaction mechanism with an ester substrate. Biochemistry. 1978 Jun 27;17(13):2659–2668. doi: 10.1021/bi00606a032. [DOI] [PubMed] [Google Scholar]
- Atkins G. L., Nimmo I. A. The reliability of Michaelis constants and maximum velocities estimated by using the integrated Michaelis-Menten equation. Biochem J. 1973 Dec;135(4):779–784. doi: 10.1042/bj1350779. [DOI] [PMC free article] [PubMed] [Google Scholar]
- Blow D. M., Birktoft J. J., Hartley B. S. Role of a buried acid group in the mechanism of action of chymotrypsin. Nature. 1969 Jan 25;221(5178):337–340. doi: 10.1038/221337a0. [DOI] [PubMed] [Google Scholar]
- Brocklehurst K., Herbert J. A., Norris R., Suschitzky H. Evidence for association-activation effects in reactions of papain from studies on its reactivity towards isomeric two-protonic-state reactivity probes. Biochem J. 1979 Nov 1;183(2):369–373. doi: 10.1042/bj1830369. [DOI] [PMC free article] [PubMed] [Google Scholar]
- Brocklehurst K., Malthouse J. P. Mechanism of the reaction of papain with substrate-derived diazomethyl ketones. Implications for the difference in site specificity of halomethyl ketones for serine proteinases and cysteine proteinases and for stereoelectronic requirements in the papain catalytic mechanism. Biochem J. 1978 Nov 1;175(2):761–764. doi: 10.1042/bj1750761. [DOI] [PMC free article] [PubMed] [Google Scholar]
- Brocklehurst K., Malthouse J. P., Shipton M. Evidence that binding to the s2-subsite of papain may be coupled with catalytically relevant structural change involving the cysteine-25-histidine-159 diad. Kinetics of the reaction of papain with a two-protonic-state reactivity probe containing a hydrophobic side chain. Biochem J. 1979 Nov 1;183(2):223–231. doi: 10.1042/bj1830223. [DOI] [PMC free article] [PubMed] [Google Scholar]
- Brubacher L. J., Bender M. L. The preparation and properties of trans-cinnamoyl-papain. J Am Chem Soc. 1966 Dec 20;88(24):5871–5880. doi: 10.1021/ja00976a032. [DOI] [PubMed] [Google Scholar]
- Brubacher L. J., Bender M. L. The preparation and properties of trans-cinnamoyl-papain. J Am Chem Soc. 1966 Dec 20;88(24):5871–5880. doi: 10.1021/ja00976a032. [DOI] [PubMed] [Google Scholar]
- Bruice T. C. Some pertinent aspects of mechanism as determined with small molecules. Annu Rev Biochem. 1976;45:331–373. doi: 10.1146/annurev.bi.45.070176.001555. [DOI] [PubMed] [Google Scholar]
- Hunkapiller M. W., Forgac M. D., Richards J. H. Mechanism of action of serine proteases: tetrahedral intermediate and concerted proton transfer. Biochemistry. 1976 Dec 14;15(25):5581–5588. doi: 10.1021/bi00670a024. [DOI] [PubMed] [Google Scholar]
- Johnson F. A., Lewis S. D., Shafer J. A. Determination of a low pK for histidine-159 in the S-methylthio derivative of papain by proton nuclear magnetic resonance spectroscopy. Biochemistry. 1981 Jan 6;20(1):44–48. doi: 10.1021/bi00504a008. [DOI] [PubMed] [Google Scholar]
- Johnson F. A., Lewis S. D., Shafer J. A. Perturbations in the free energy and enthalpy of ionization of histidine-159 at the active site of papain as determined by fluorescence spectroscopy. Biochemistry. 1981 Jan 6;20(1):52–58. doi: 10.1021/bi00504a600. [DOI] [PubMed] [Google Scholar]
- Kirsch J. F., Igelström M. The kinetics of the papain-catalyzed hydrolysis of esters of carbobenzoxyglycine. Evidence for an acyl-enzyme intermediate. Biochemistry. 1966 Feb;5(2):783–791. doi: 10.1021/bi00866a053. [DOI] [PubMed] [Google Scholar]
- Kurachi K., Fujikawa K., Schmer G., Davie E. W. Inhibition of bovine factor IXa and factor Xabeta by antithrombin III. Biochemistry. 1976 Jan 27;15(2):373–377. doi: 10.1021/bi00647a021. [DOI] [PubMed] [Google Scholar]
- Lewis S. D., Johnson F. A., Shafer J. A. Effect of cysteine-25 on the ionization of histidine-159 in papain as determined by proton nuclear magnetic resonance spectroscopy. Evidence for a his-159--Cys-25 ion pair and its possible role in catalysis. Biochemistry. 1981 Jan 6;20(1):48–51. doi: 10.1021/bi00504a009. [DOI] [PubMed] [Google Scholar]
- Lewis S. D., Johnson F. A., Shafer J. A. Potentiometric determination of ionizations at the active site of papain. Biochemistry. 1976 Nov 16;15(23):5009–5017. doi: 10.1021/bi00668a010. [DOI] [PubMed] [Google Scholar]
- Lowe G., Yuthavong Y. Kinetic specificity in papain-catalysed hydrolyses. Biochem J. 1971 Aug;124(1):107–115. doi: 10.1042/bj1240107. [DOI] [PMC free article] [PubMed] [Google Scholar]
- O'Leary M. H., Yamada H., Yapp C. J. Multiple isotope effect probes of glutamate decarboxylase. Biochemistry. 1981 Mar 17;20(6):1476–1481. doi: 10.1021/bi00509a011. [DOI] [PubMed] [Google Scholar]
- Polgár L. Mercaptide-imidazolium ion-pair: the reactive nucleophile in papain catalysis. FEBS Lett. 1974 Oct 1;47(1):15–18. doi: 10.1016/0014-5793(74)80415-1. [DOI] [PubMed] [Google Scholar]
- Venkatasubban K. S., Silverman D. N. Carbon dioxide hydration activity of carbonic anhydrase in mixtures of water and deuterium oxide. Biochemistry. 1980 Oct 28;19(22):4984–4989. doi: 10.1021/bi00563a008. [DOI] [PubMed] [Google Scholar]
- WHITAKER J. R., BENDER M. L. KINETICS OF PAPAIN-CATALYZED HYDROLYSIS OF ALPHA-N-BENZOYL-L-ARGININE ETHYL ESTER AND ALPHA-N-BENZOYL-L-ARGININAMIDE. J Am Chem Soc. 1965 Jun 20;87:2728–2737. doi: 10.1021/ja01090a034. [DOI] [PubMed] [Google Scholar]
- Wharton C. W., Cornish-Bowden A., Brocklehurst K., Crook E. M. Kinetics of the hydrolysis of N-benzoyl-L-serine methyl ester catalysed by bromelain and by papain. Analysis of modifier mechanisms by lattice nomography, computational methods of parameter evaluation for substrate-activated catalyses and consequences of postulated non-productive binding in bromelain- and papain-catalysed hydrolyses. Biochem J. 1974 Aug;141(2):365–381. doi: 10.1042/bj1410365. [DOI] [PMC free article] [PubMed] [Google Scholar]
- Williams D. C., Whitaker J. R. Kinetics of papain-catalyzed hydrolyses of neutral substrates. Biochemistry. 1967 Dec;6(12):3711–3717. doi: 10.1021/bi00864a013. [DOI] [PubMed] [Google Scholar]
- Zannis V. I., Kirsch J. F. Effects of substituents on the rates of deacylation of substituted benzoyl papains. Role of a carboxylate residue in the catalytic mechanism. Biochemistry. 1978 Jun 27;17(13):2669–2674. doi: 10.1021/bi00606a033. [DOI] [PubMed] [Google Scholar]