Abstract
Rabbit muscle pyruvate kinase was irreverisbly inactivated by 5-chloro-4-oxopentanoic acid with a pKa of 9.2. The inhibition was time-dependent and was related to the 5-chloro-4-oxopentanoic acid concentration. Analysis of the kinetics of inhibition showed that the binding of the inhibitor showed positive co-operativity (n = 1.5 +/- 0.2). Inhibition of pyruvate kinase by 5-chloro-4-oxopentanoic acid was prevented by ligands which bind to the active site. Their effectiveness was placed in the order Mg2+ greater than phosphoenolpyruvate greater than ATP greater than ADP greater than pyruvate. Inhibitor-modified pyruvate kinase was unable to catalyse the detritiation of [3-(3)H]pyruvate in the ATP-promoted reaction, but it did retain 5-10% of the activity with either phosphate or arsenate as promoters. 5-Chlor-4-oxo-[3,5-(3)H]pentanoic acid was covalently bound to pyruvate kinase and demonstrated a stoicheiometry of 1 mol of inhibitor bound per mol of pyruvate kinase protomer. The incorporation of the inhibitor and the loss of enzyme was proportional. These results are discussed in terms of 5-chloro-4-oxopentanoic acid alkylating a functional group in the phosphoryl overlap region of the active site, and a model is presented in which this compound alkylates an active-site thiol in a reaction that is controlled by a more basic group at the active site.
Full text
PDF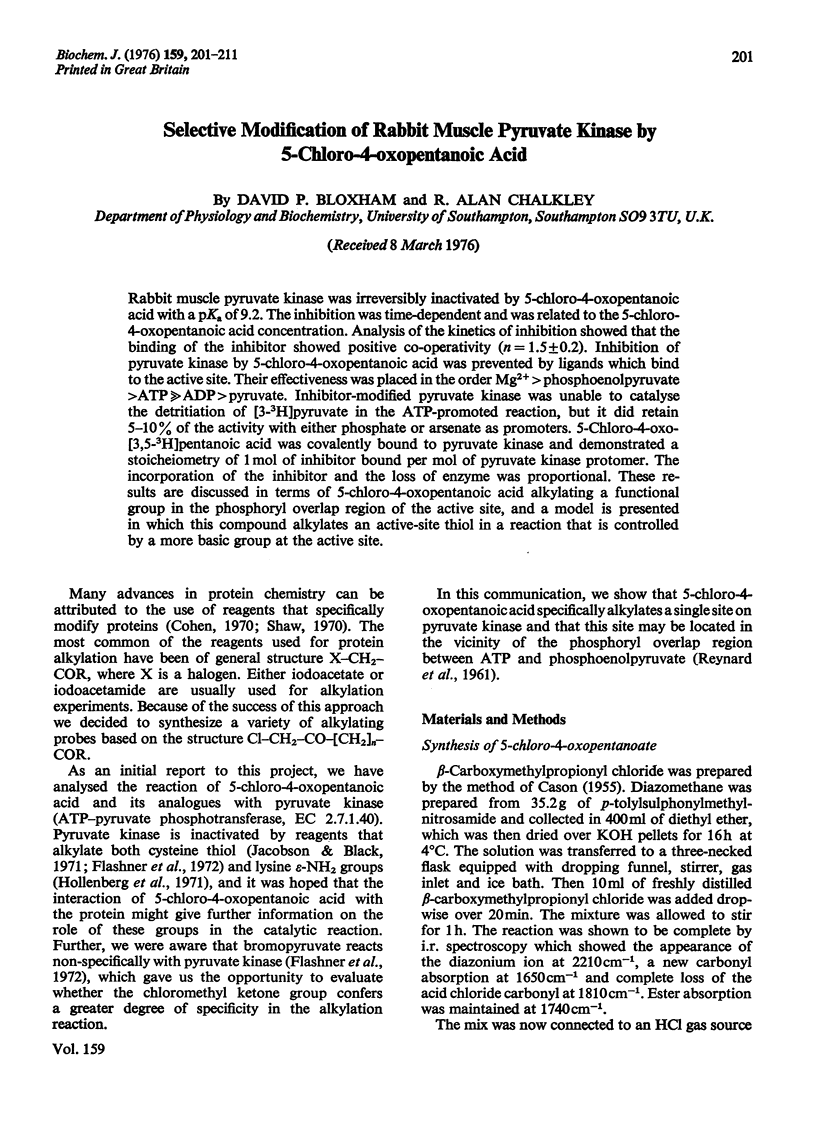
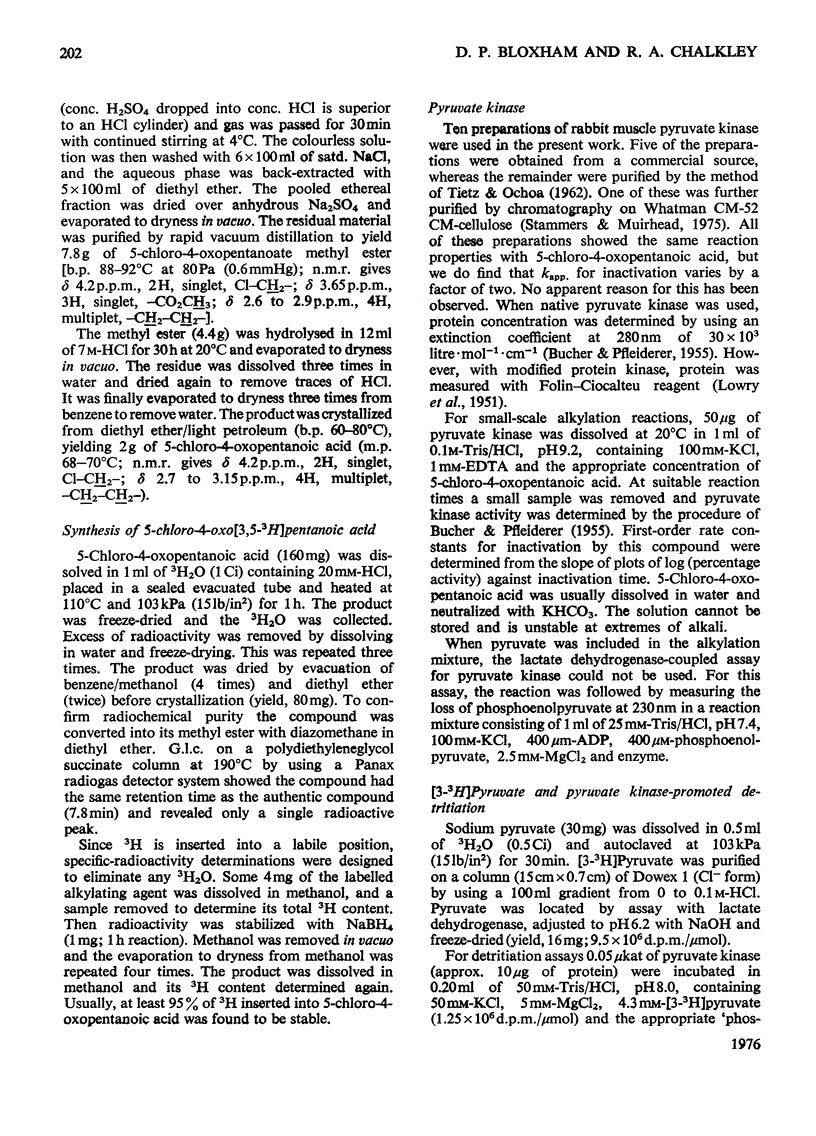
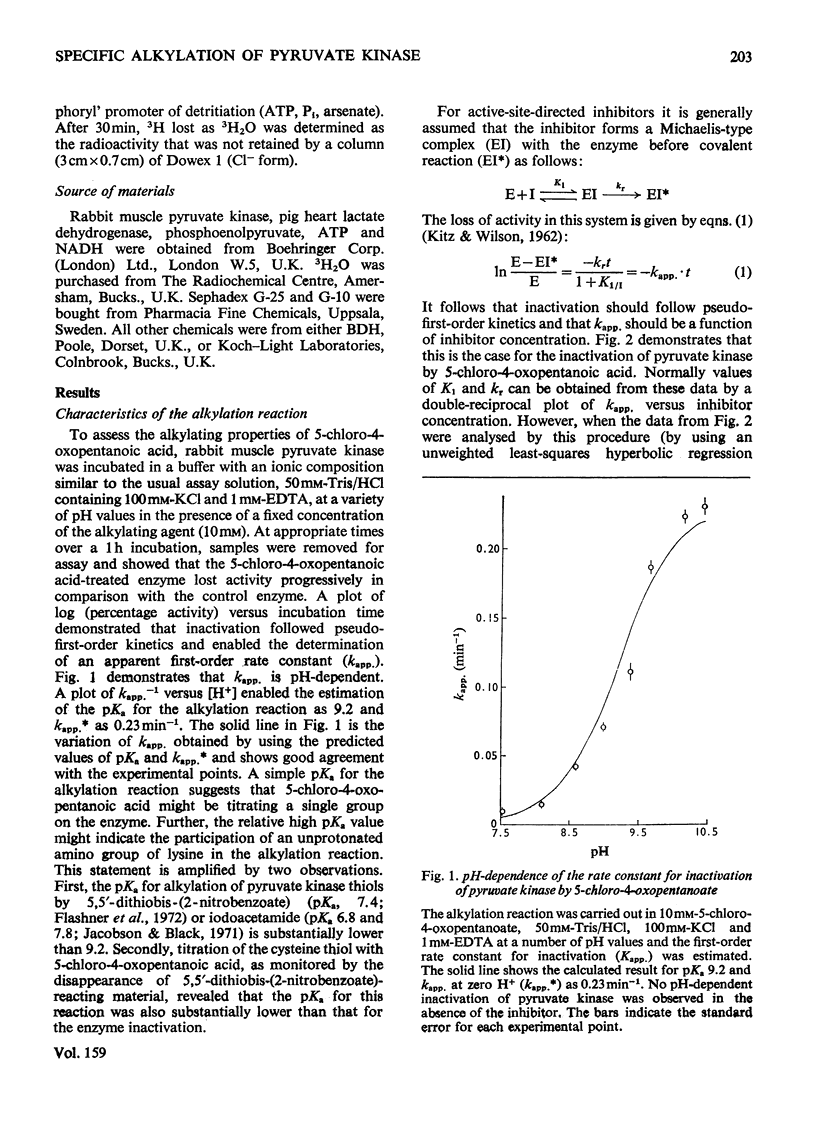
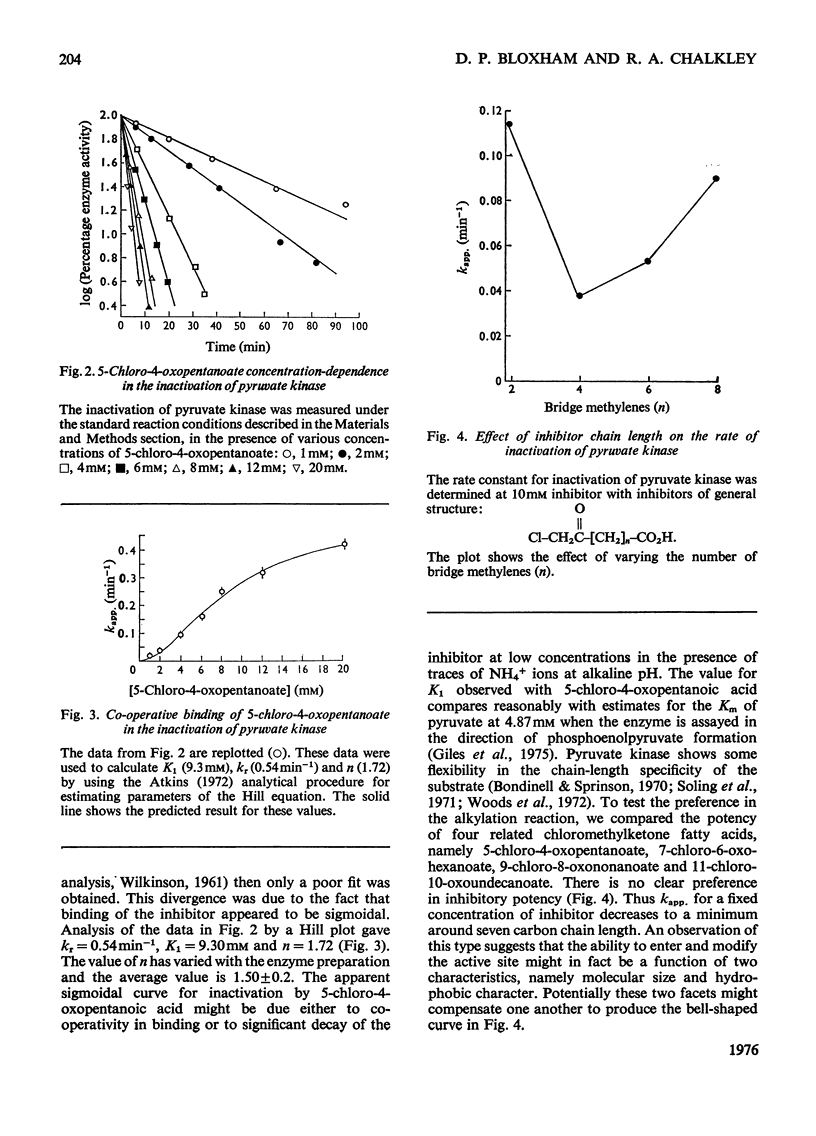
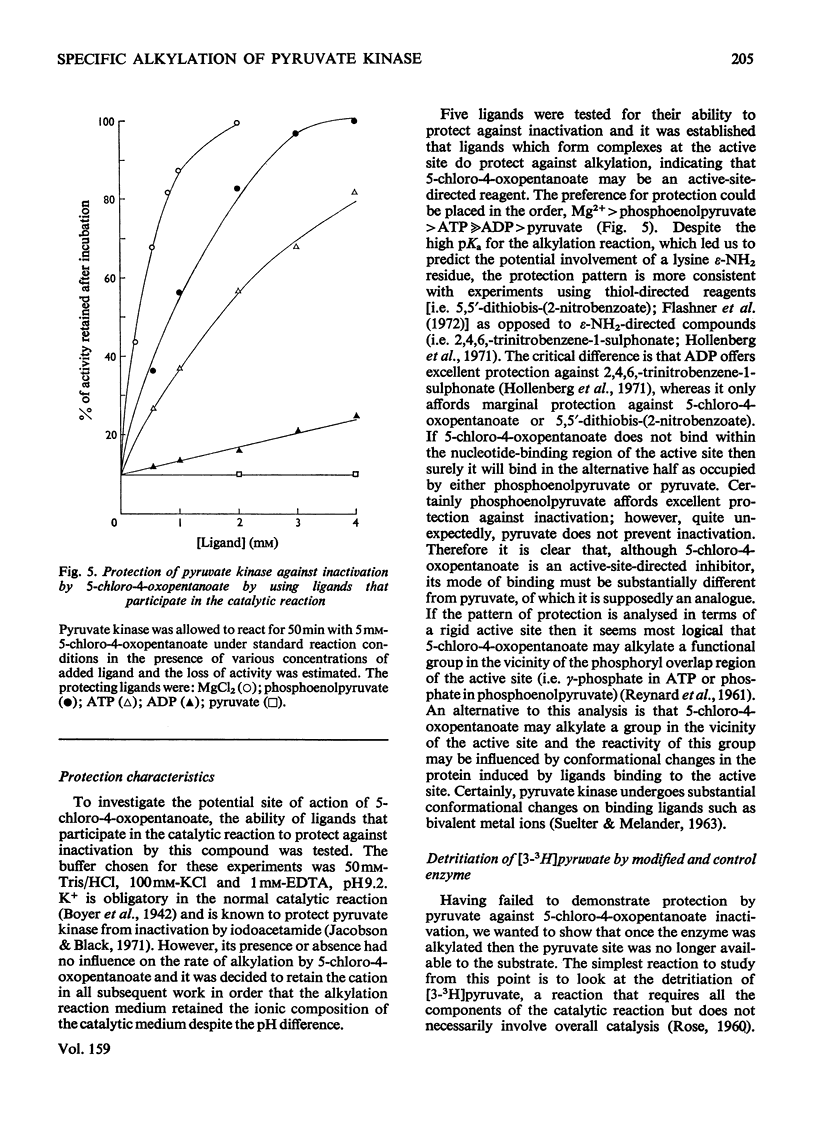
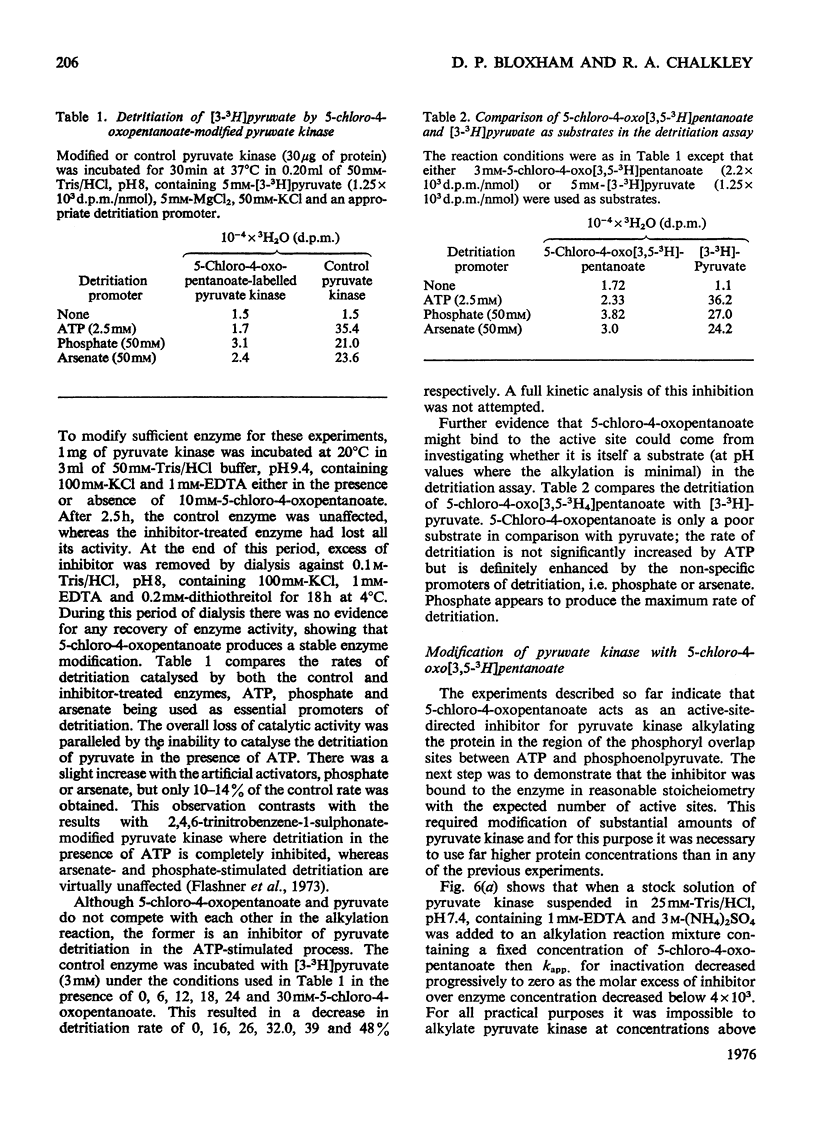
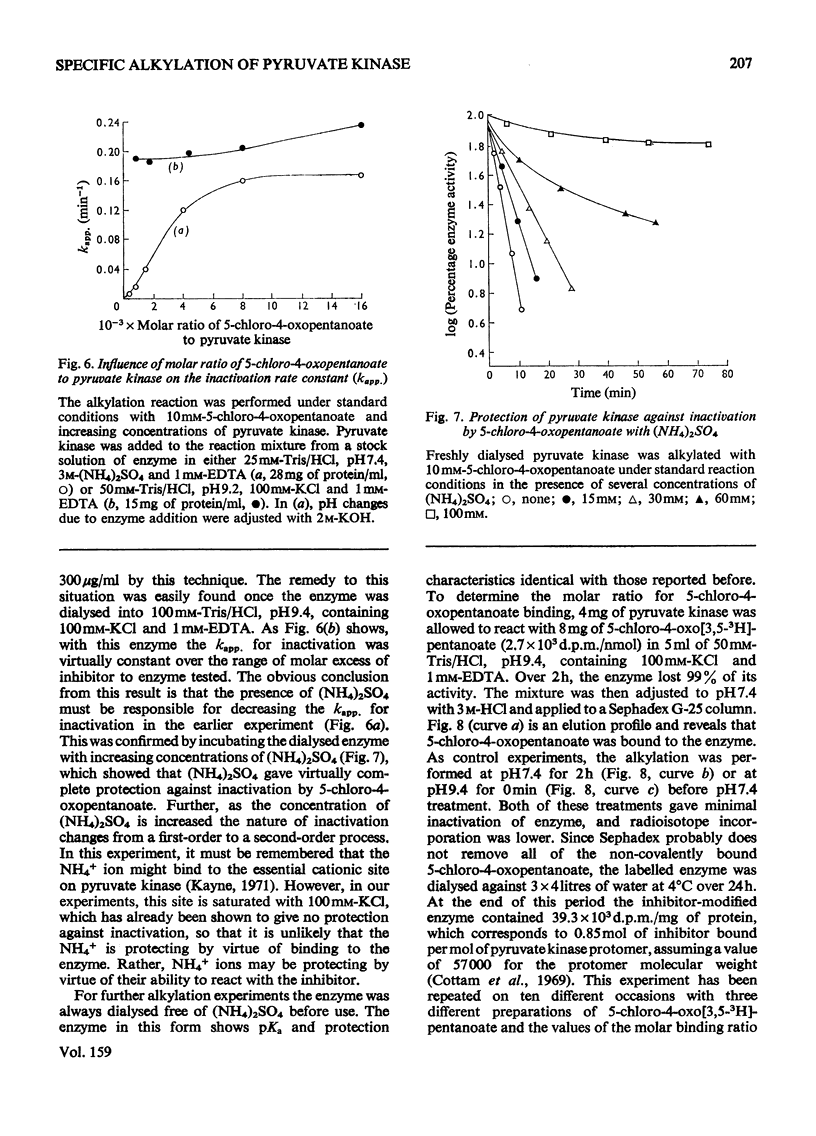
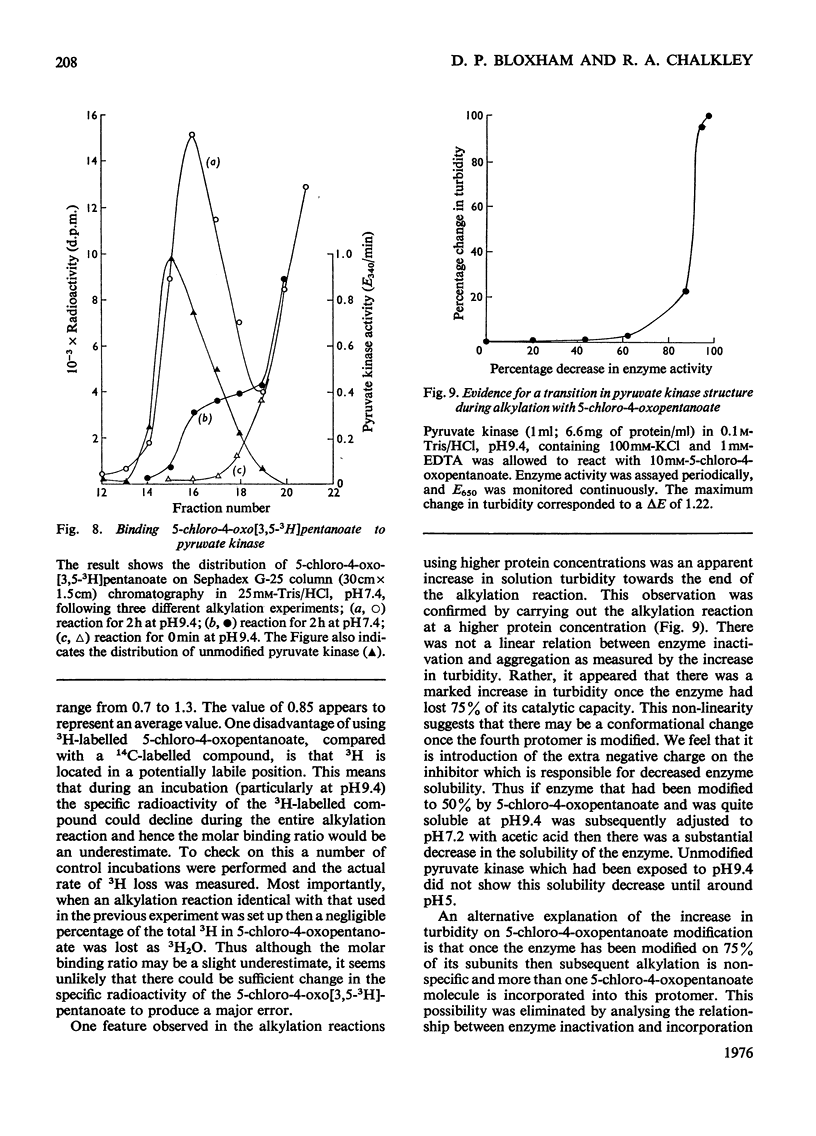
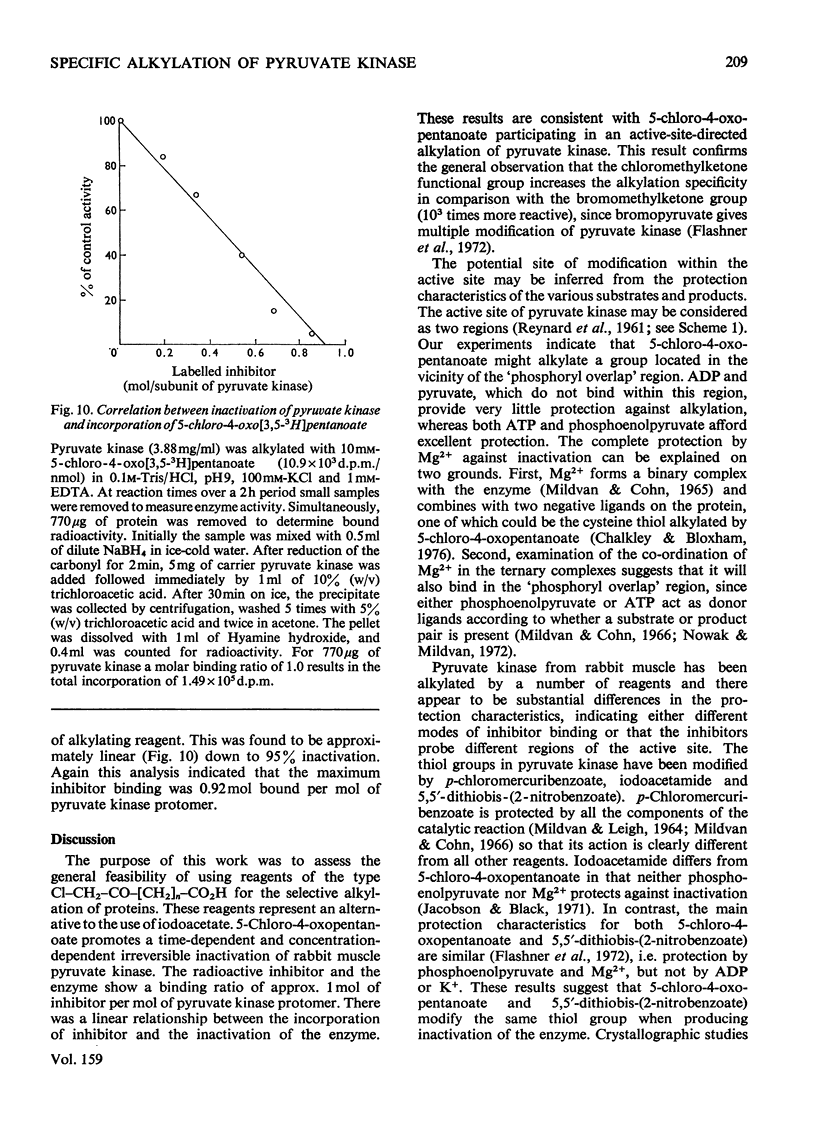
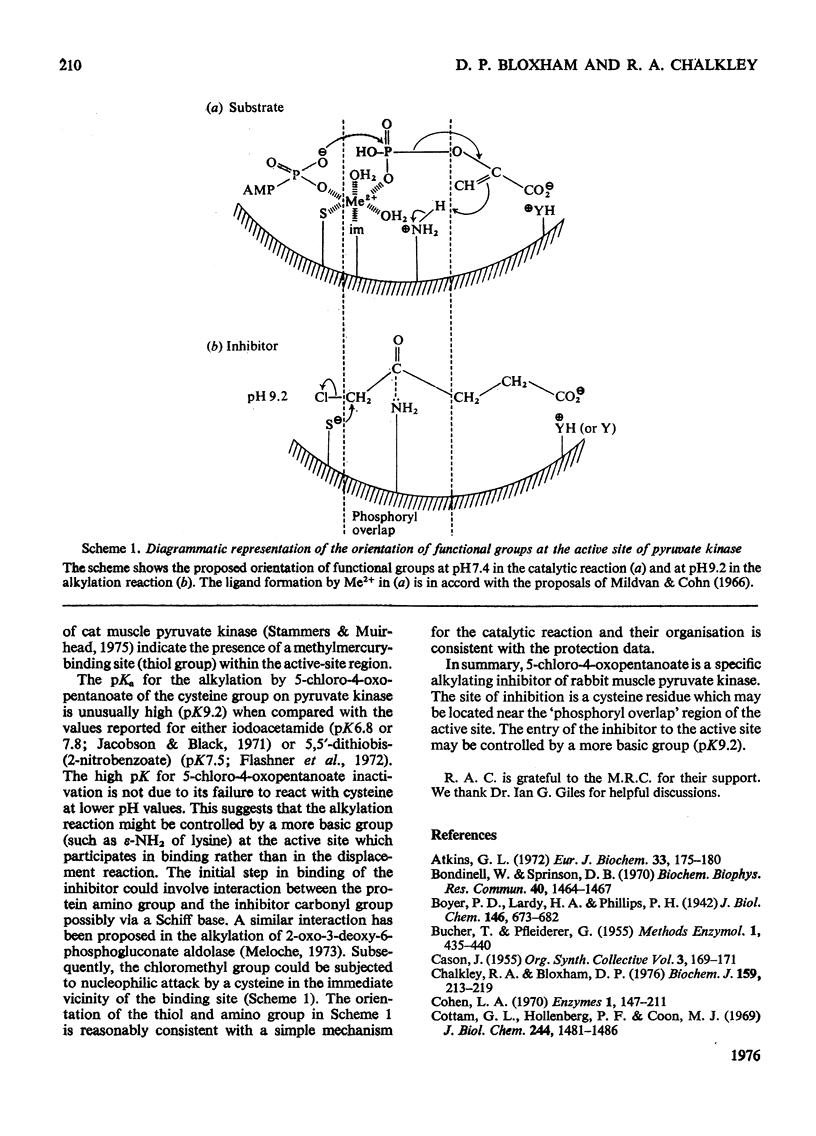
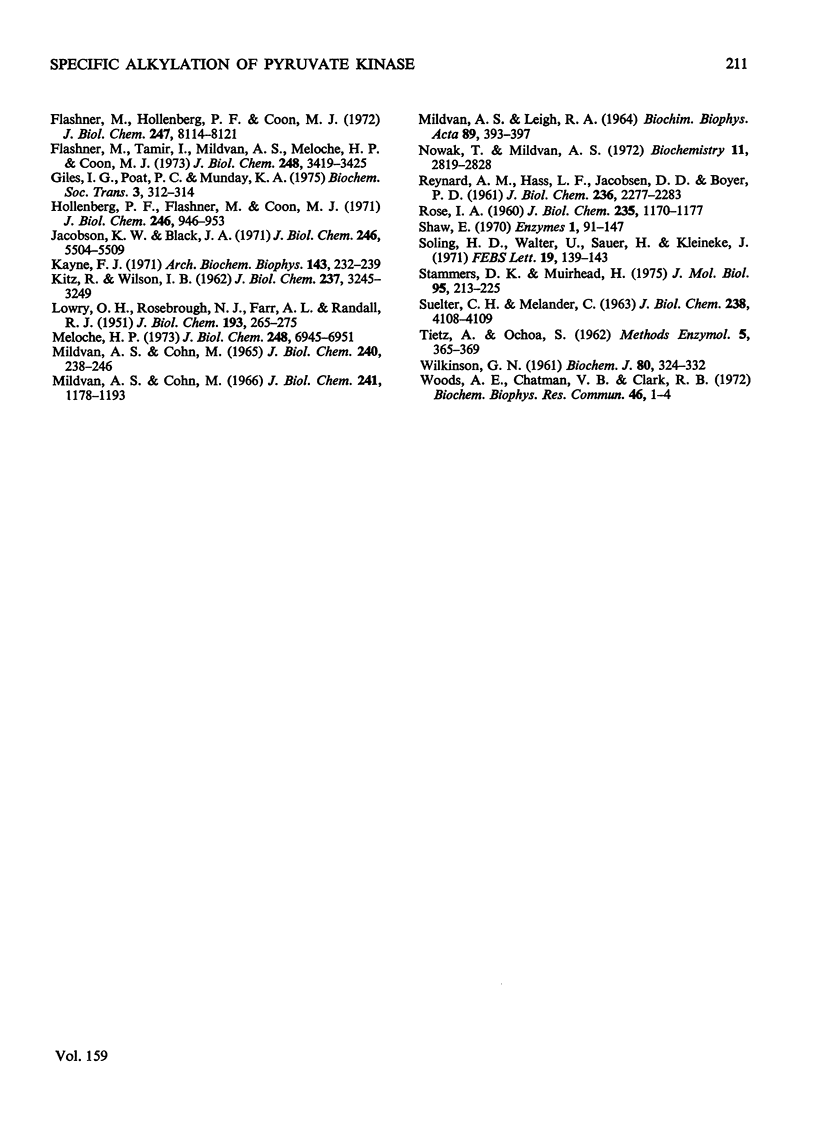
Selected References
These references are in PubMed. This may not be the complete list of references from this article.
- Atkins G. L. A simple digital-computer program for estimating the parameters of the hill equation. Eur J Biochem. 1973 Feb 15;33(1):175–180. doi: 10.1111/j.1432-1033.1973.tb02667.x. [DOI] [PubMed] [Google Scholar]
- Bondinell W. E., Sprinson D. B. The stereochemistry of pyruvate kinase. Biochem Biophys Res Commun. 1970 Sep 30;40(6):1464–1467. doi: 10.1016/0006-291x(70)90032-x. [DOI] [PubMed] [Google Scholar]
- Chalkley R. A., Bloxham D. P. Identification of cysteine as the reactive group in pyruvate kinase alkylated by 5-chloro-4-oxopentanoic acid. Biochem J. 1976 Nov;159(2):213–219. doi: 10.1042/bj1590213. [DOI] [PMC free article] [PubMed] [Google Scholar]
- Cottam G. L., Hollenberg P. F., Coon M. J. Subunit structure of rabbit muscle pyruvate kinase. J Biol Chem. 1969 Mar 25;244(6):1481–1486. [PubMed] [Google Scholar]
- Flashner M., Hollenberg P. F., Coon M. J. Mechanism of action of pyruvate kinase. Role of sulfhydryl groups in catalytic activity as determined by disulfide interchange. J Biol Chem. 1972 Dec 25;247(24):8114–8121. [PubMed] [Google Scholar]
- Flashner M., Tamir I., Mildvan A. S., Meloche H. P., Coon M. J. Magnetic resonance and catalytic studies of pyruvate kinase with essential sulfhydryl or lysyl epsilon-amino groups chemically modified. J Biol Chem. 1973 May 25;248(10):3419–3425. [PubMed] [Google Scholar]
- Giles I. G., Poat P. C., Munday K. A. A kinetic analysis of rabbit muscle pyruvate kinase in the reverse direction. Biochem Soc Trans. 1975;3(2):312–314. doi: 10.1042/bst0030312. [DOI] [PubMed] [Google Scholar]
- Hollenberg P. F., Flashner M., Coon M. J. Role of lysyl epsilon-amino groups in adenosine diphosphate binding and catalytic activity of pyruvate kinase. J Biol Chem. 1971 Feb 25;246(4):946–953. [PubMed] [Google Scholar]
- Jacobson K. W., Black J. A. Conformational differences in the active sites of muscle and erythrocyte pyruvate kinase. J Biol Chem. 1971 Sep 10;246(17):5504–5509. [PubMed] [Google Scholar]
- KITZ R., WILSON I. B. Esters of methanesulfonic acid as irreversible inhibitors of acetylcholinesterase. J Biol Chem. 1962 Oct;237:3245–3249. [PubMed] [Google Scholar]
- Kayne F. J. Thallium (I) activation of pyruvate kinase. Arch Biochem Biophys. 1971 Mar;143(1):232–239. doi: 10.1016/0003-9861(71)90204-9. [DOI] [PubMed] [Google Scholar]
- LOWRY O. H., ROSEBROUGH N. J., FARR A. L., RANDALL R. J. Protein measurement with the Folin phenol reagent. J Biol Chem. 1951 Nov;193(1):265–275. [PubMed] [Google Scholar]
- MILDVAN A. S., COHN M. KINETIC AND MAGNETIC RESONANCE STUDIES OF THE PYRUVATE KINASE REACTION. I. DIVALENT METAL COMPLEXES OF PYRUVATE KINASE. J Biol Chem. 1965 Jan;240:238–246. [PubMed] [Google Scholar]
- MILDVAN A. S., LEIGH R. A. DETERNATION OF CO-FACTOR DISSOCIATION CONSTANTS FROM THE KINETICS OF INHIBITION OF ENZYMES. Biochim Biophys Acta. 1964 Sep 18;89:393–397. doi: 10.1016/0926-6569(64)90065-3. [DOI] [PubMed] [Google Scholar]
- Meloche H. P. The substrate analog, bromopyruvate, as a bridging agent for the active site of 2-keto-3-deoxy-6-phosphogluconic aldolase. Chemical evidence for a carboxylate adjacent to the Schiff's base-forming lysine. J Biol Chem. 1973 Oct 25;248(20):6945–6951. [PubMed] [Google Scholar]
- Mildvan A. S., Cohn M. Kinetic and magnetic resonance studies of the pyruvate kinase reaction. II. Complexes of enzyme, metal, and substrates. J Biol Chem. 1966 Mar 10;241(5):1178–1193. [PubMed] [Google Scholar]
- Nowak T., Mildvan A. S. Nuclear magnetic resonance studies of the function of potassium in the mechanism of pyruvate kinase. Biochemistry. 1972 Jul 18;11(15):2819–2828. doi: 10.1021/bi00765a014. [DOI] [PubMed] [Google Scholar]
- REYNARD A. M., HASS L. F., JACOBSEN D. D., BOYER P. D. The correlation of reaction kinetics and substrate binding with the mechanism of pyruvate kinase. J Biol Chem. 1961 Aug;236:2277–2283. [PubMed] [Google Scholar]
- ROSE I. A. Studies on the enolization of pyruvate by pyruvate kinase. J Biol Chem. 1960 Apr;235:1170–1177. [PubMed] [Google Scholar]
- SUELTER C. H., MELANDER W. USE OF PROTEIN DIFFERENCE SPECTROPHOTOMETRY TO DETERMINE ENZYME-COFACTOR DISSOCIATION CONSTANTS. J Biol Chem. 1963 Dec;238:4108–4109. [PubMed] [Google Scholar]
- Stammers D. K., Muirhead H. Three-dimensional structure of cat muscle pyruvate kinase at 6 Angstrom resolution. J Mol Biol. 1975 Jun 25;95(2):213–225. doi: 10.1016/0022-2836(75)90391-5. [DOI] [PubMed] [Google Scholar]
- Söling H. D., Walter U., Sauer H., Kleineke J. Effects of synthetic analogues of phosphoenolpyruvate on muscle and liver pyruvate kinase, muscle enolase, liver phosphoenolpyruvate carboxykinase and on the intra-/extra-mitochondrial tricarboxylic acid carrier transport system. FEBS Lett. 1971 Dec 1;19(2):139–143. doi: 10.1016/0014-5793(71)80498-2. [DOI] [PubMed] [Google Scholar]
- WILKINSON G. N. Statistical estimations in enzyme kinetics. Biochem J. 1961 Aug;80:324–332. doi: 10.1042/bj0800324. [DOI] [PMC free article] [PubMed] [Google Scholar]