Abstract
1. The sterol, unsaturated fatty acid and cytochrome contents of cells of a δ-aminolaevulinate synthase mutant of Saccharomyces cerevisiae are manipulated by growing the organism in media containing defined supplements of δ-aminolaevulinate and other porphyrin intermediates. 2. If unsaturated fatty acids are added to the growth medium as Tween 80, sterol content and respiratory cytochromes alone are manipulated. 3. In the presence of δ-aminolaevulinate (10–50mg/1) cells exhibit moderate to high respiratory activity, but growth yields are low, indicating a loss of oxidative phosphorylation. This is associated with the depletion of membrane lipids, either unsaturated fatty acids and sterols together or sterols alone. 4. Sterol depletion leads to the loss of coupled mitochondrial oxidative phosphorylation in vitro. 5. The lesion in oxidative phosphorylation is associated with an increase in the passive permeability of sterol-depleted mitochondria to protons. 6. Arrhenius plots of mitochondrial permeability to protons indicate that the activation energy for proton entry increases as the sterol content of the membranes decreases. 7. Studies on a cytoplasmic petite mutant isolated from strain ole-3, which lacks a functional membrane-bound protein-translocating adenosine triphosphatase, indicate that proton permeability of the petite mitochondria varies as a function of sterol composition in the same way as that of ole-3 grande mitochondria. This indicates that sterols alone are probably directly responsible for the increased proton entry, owing to a reorganization of the lipid in the membrane. 8. Supplemented ole-3 cells with a normal lipid composition and normal or higher than normal respiratory activities have a growth efficiency only 65% of that of the wild-type, indicating that a further lesion in energy metabolism may be present.
Full text
PDF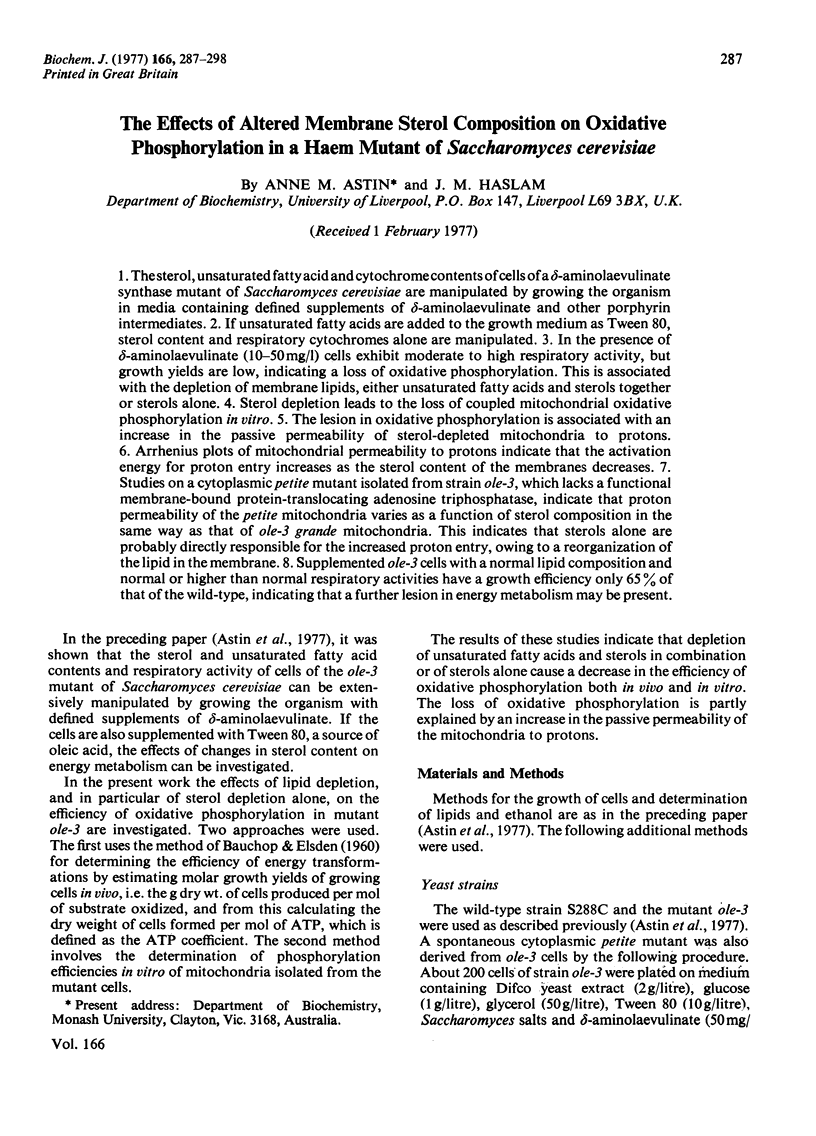
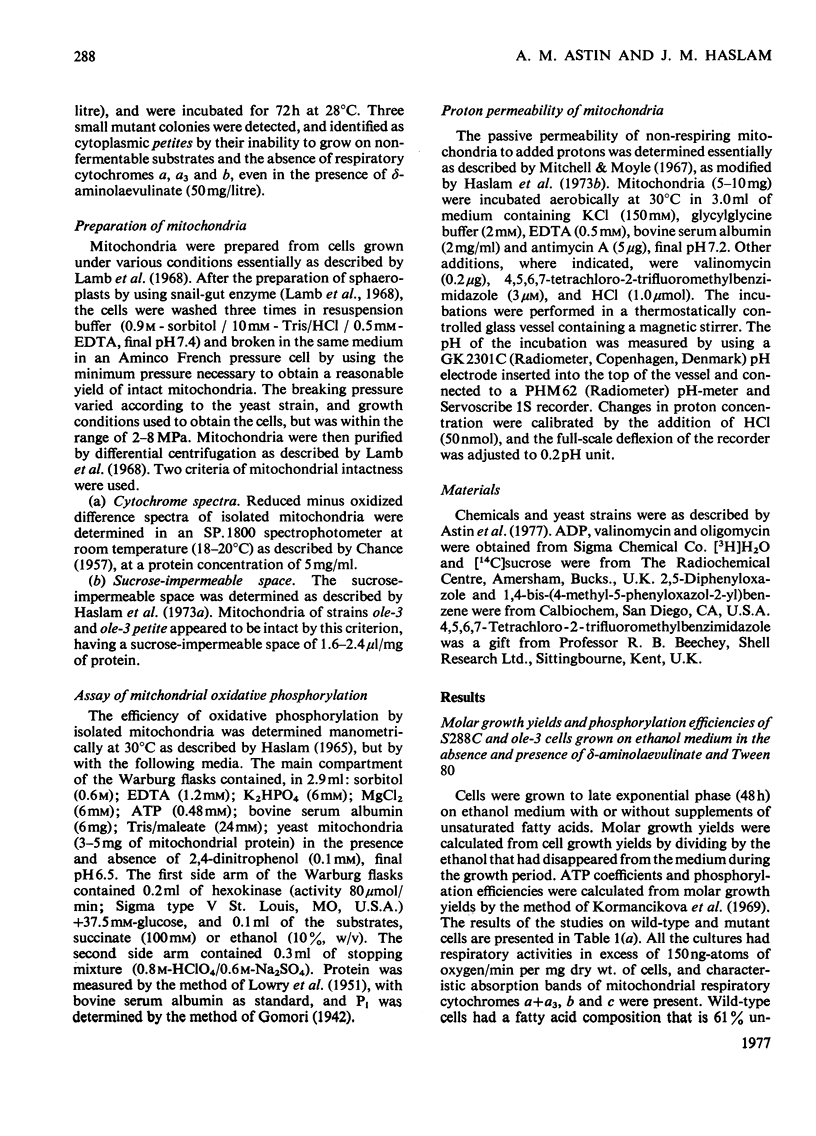
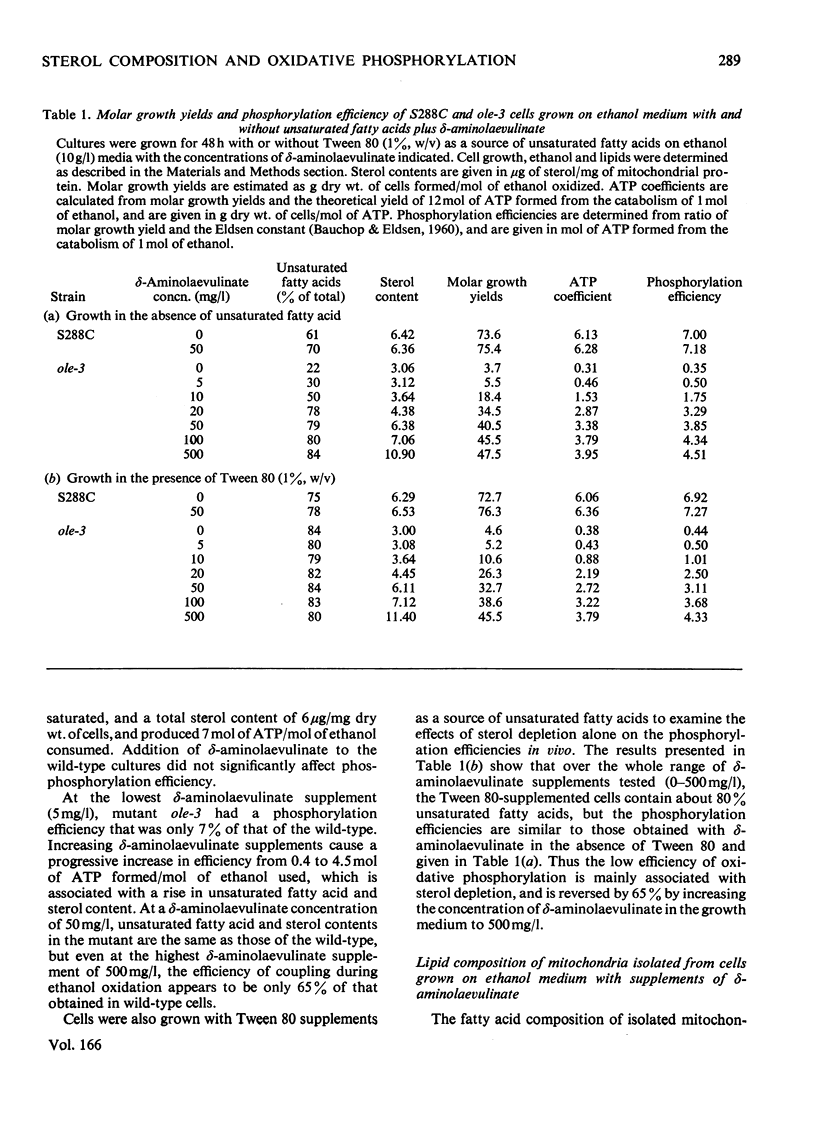
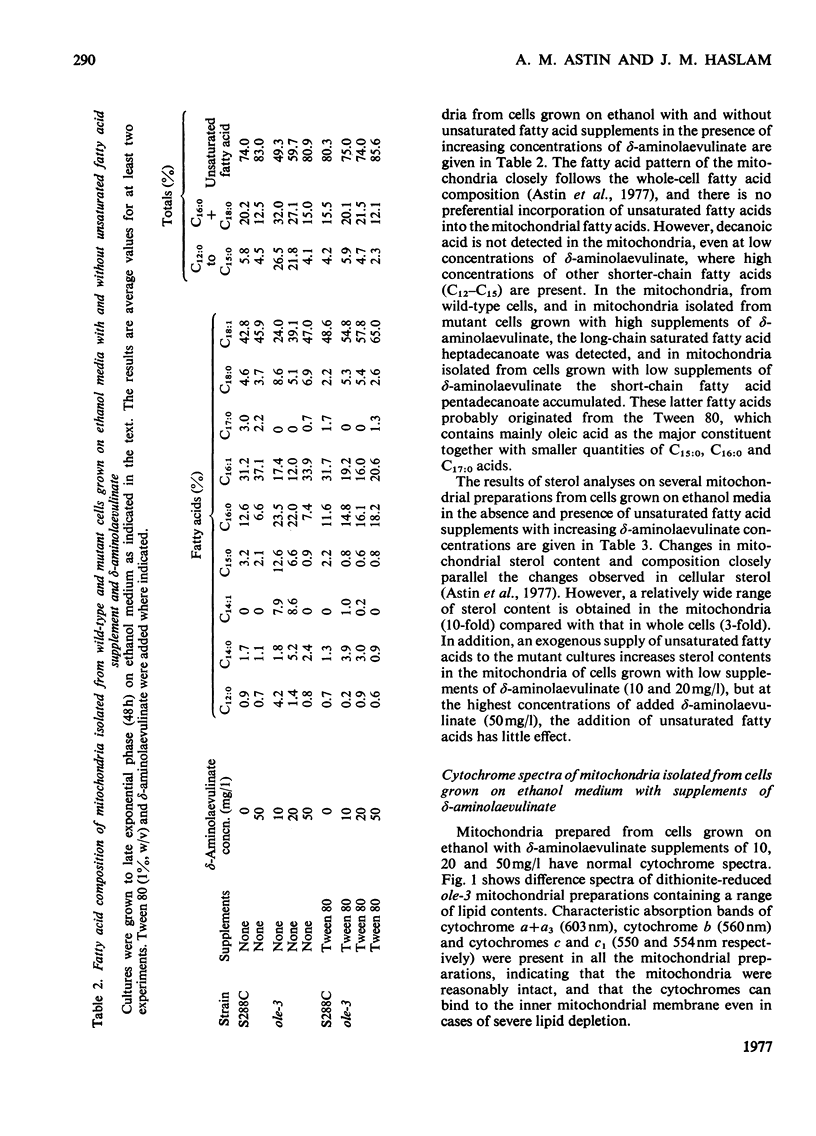
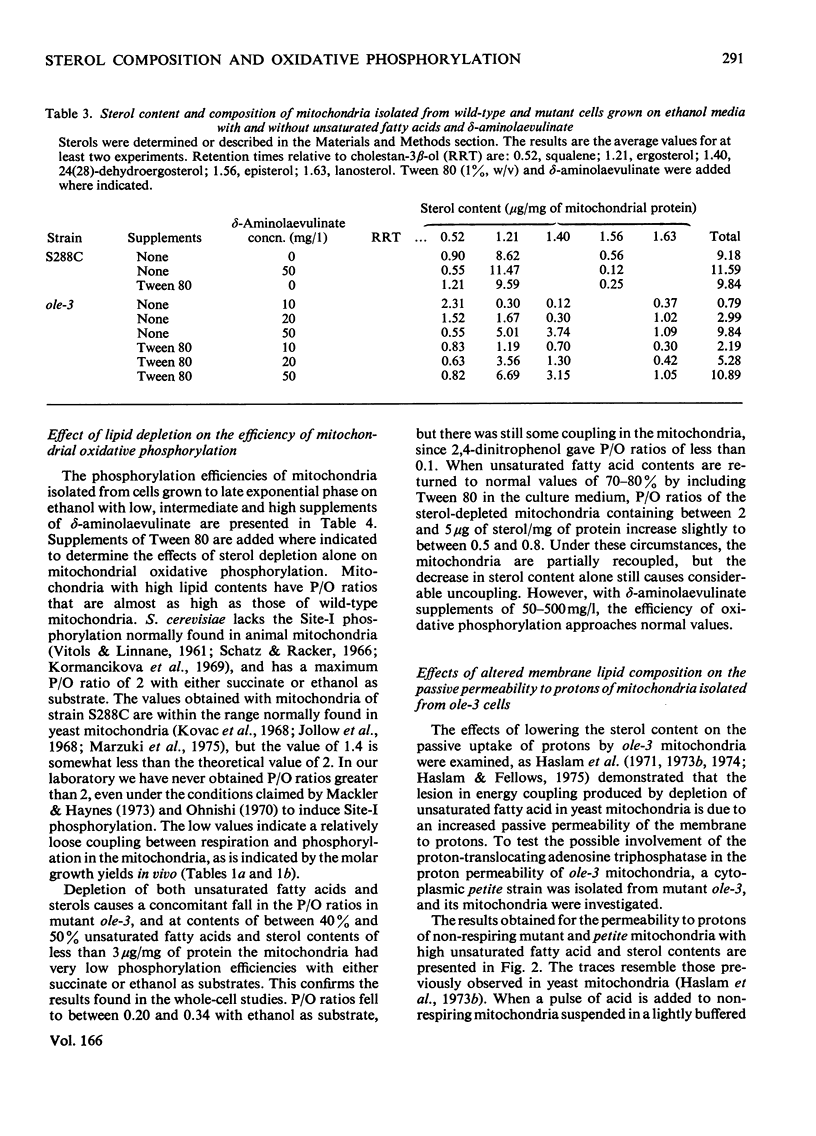
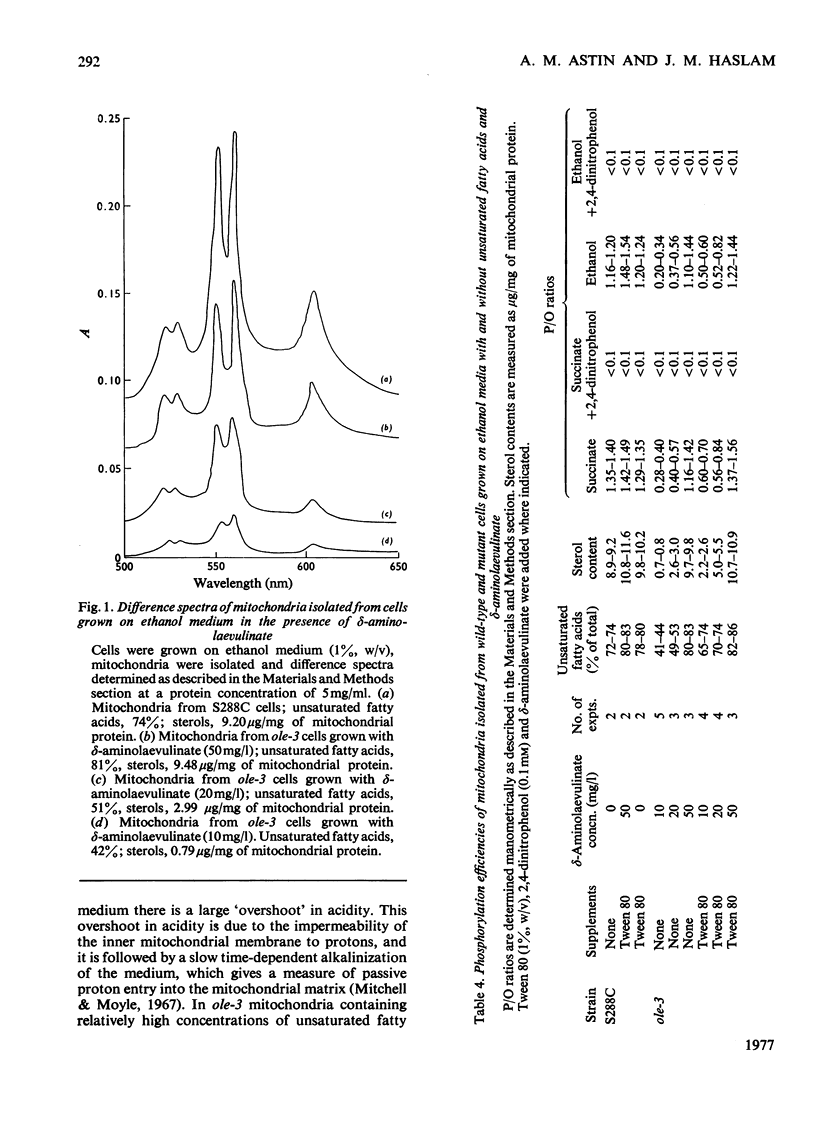
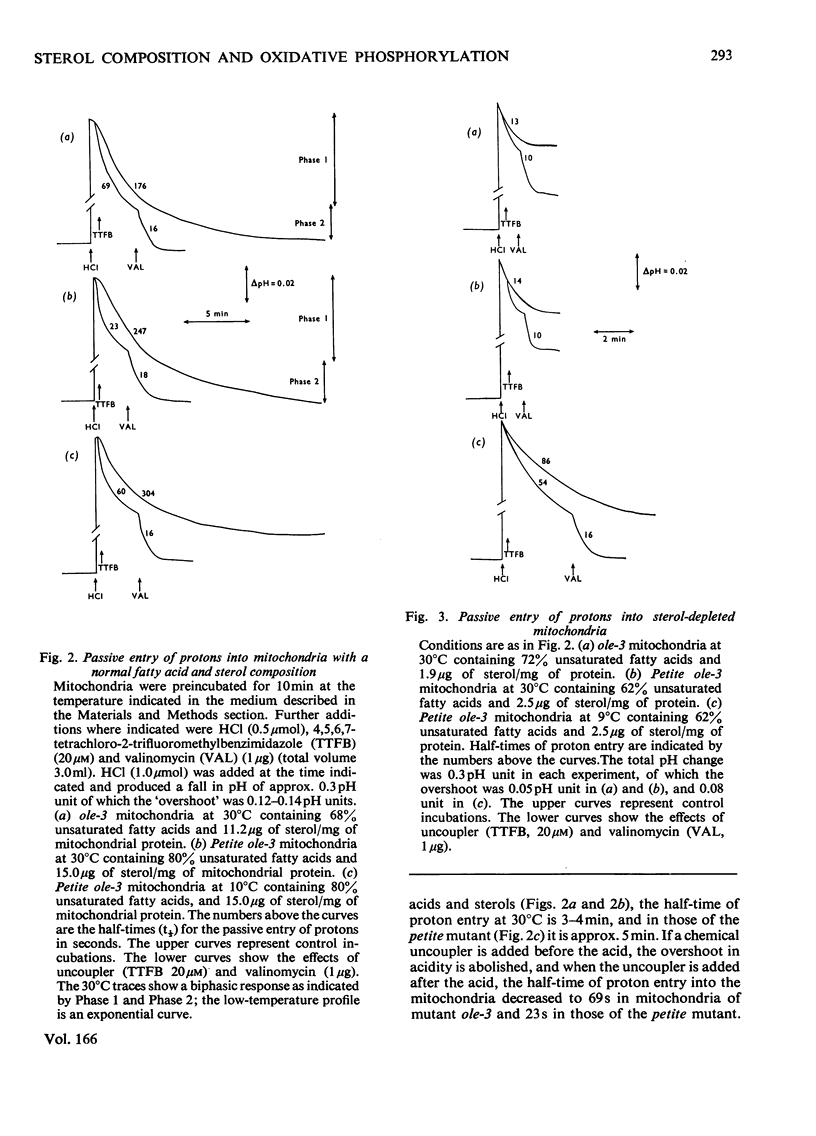
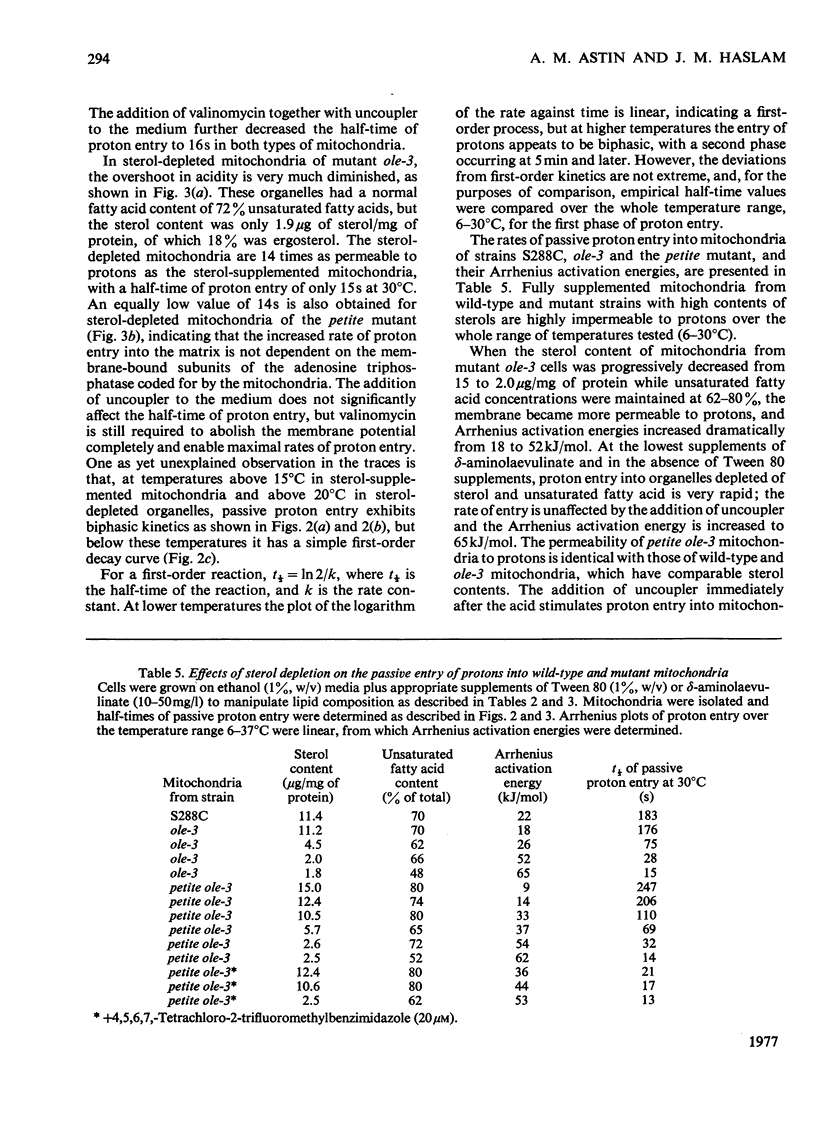
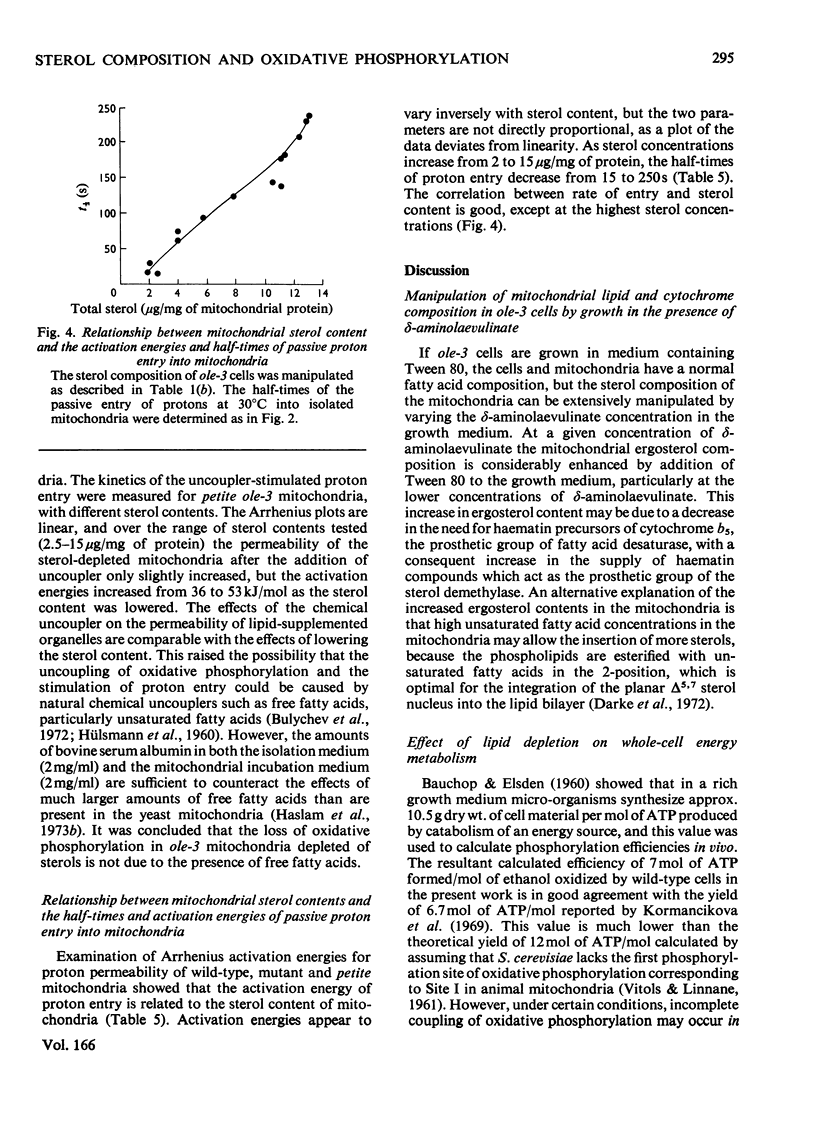
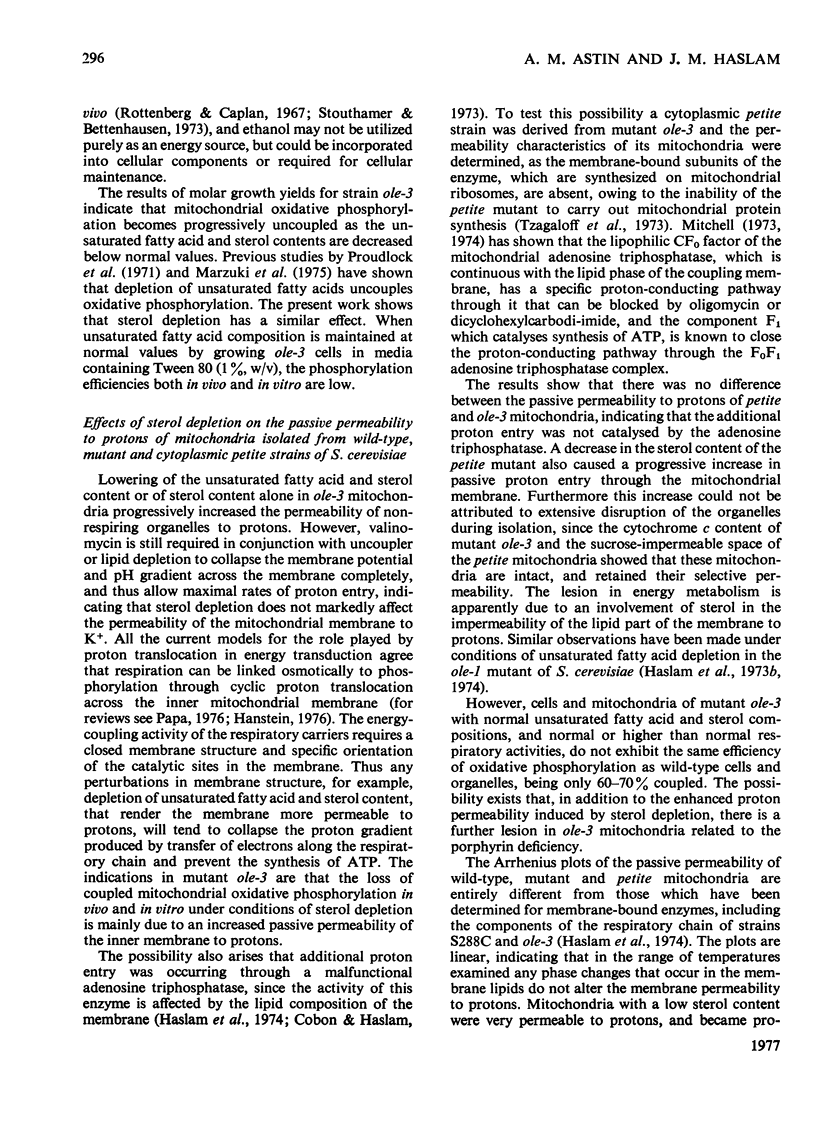
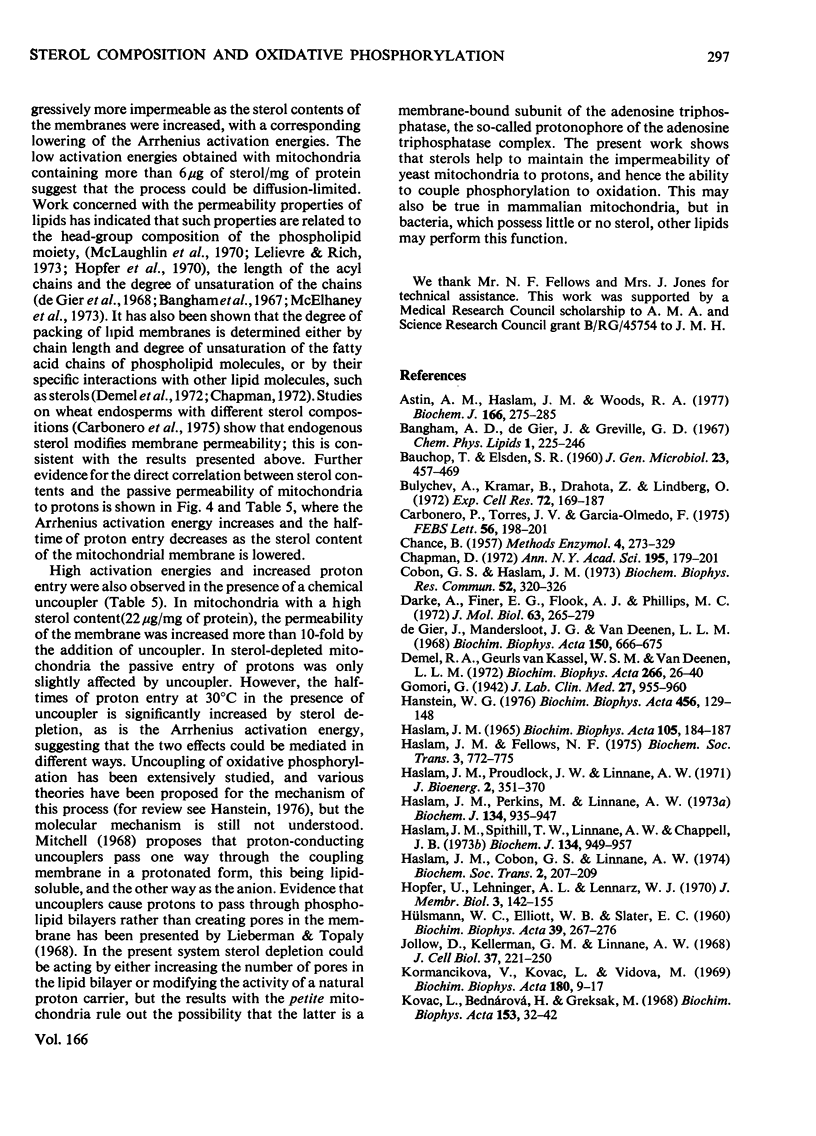
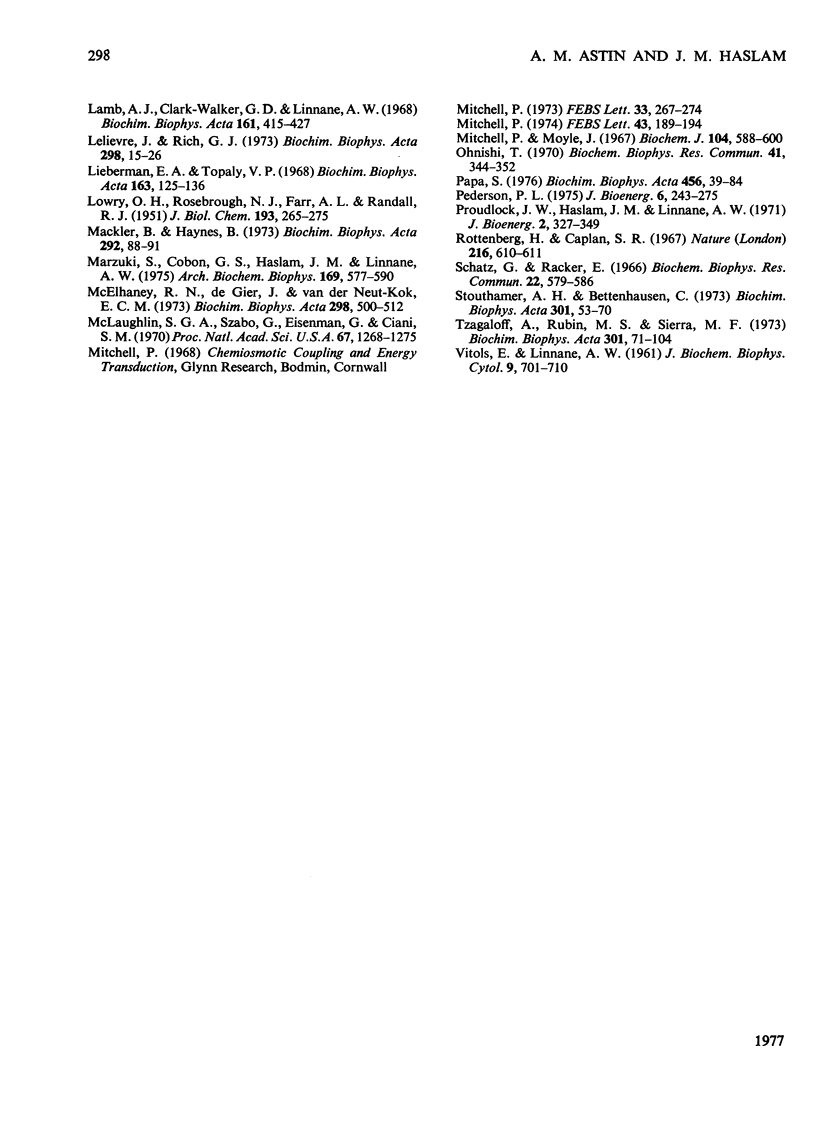
Selected References
These references are in PubMed. This may not be the complete list of references from this article.
- Astin A. M., Haslam J. M. The manipulation of cellular cytochrome and lipid composition in a haem mutant of Saccharomyces cerevisiae. Biochem J. 1977 Aug 15;166(2):275–285. doi: 10.1042/bj1660275. [DOI] [PMC free article] [PubMed] [Google Scholar]
- BAUCHOP T., ELSDEN S. R. The growth of micro-organisms in relation to their energy supply. J Gen Microbiol. 1960 Dec;23:457–469. doi: 10.1099/00221287-23-3-457. [DOI] [PubMed] [Google Scholar]
- Bulychev A., Kramar R., Drahota Z., Lindberg O. Role of a specific endogenous fatty acid fraction in the coupling-uncoupling mechanism of oxidative phosphorylation of brown adipose tissue. Exp Cell Res. 1972 May;72(1):169–187. doi: 10.1016/0014-4827(72)90579-4. [DOI] [PubMed] [Google Scholar]
- Carbonero P., Torres J. V., Garcia-Olmedo F. Effects of n-butanol and filipin on membrane permeability of developing wheat endosperms with different sterol phenotypes. FEBS Lett. 1975 Aug 15;56(2):198–201. doi: 10.1016/0014-5793(75)81090-8. [DOI] [PubMed] [Google Scholar]
- Chapman D. Nuclear magnetic resonance spectroscopic studies of biological membranes. Ann N Y Acad Sci. 1972 Jun 20;195:179–206. [PubMed] [Google Scholar]
- Cobon G. S., Haslam J. M. The effect of altered membrane sterol composition on the temperature dependence of yeast mitochondrial ATPase. Biochem Biophys Res Commun. 1973 May 1;52(1):320–326. doi: 10.1016/0006-291x(73)90990-x. [DOI] [PubMed] [Google Scholar]
- Darke A., Finer E. G., Flook A. G., Phillips M. C. Nuclear magnetic resonance study of lecithin-cholesterol interactions. J Mol Biol. 1972 Jan 28;63(2):265–279. doi: 10.1016/0022-2836(72)90374-9. [DOI] [PubMed] [Google Scholar]
- Demiel R. A., Guerts van Kessel W. S., van Deenen L. L. The properties of polyunsaturated lecithins in monolayers and liposomes and the interactions of these lecithins with cholesterol. Biochim Biophys Acta. 1972 Apr 14;266(1):26–40. doi: 10.1016/0005-2736(72)90116-2. [DOI] [PubMed] [Google Scholar]
- HULSMANN W. C., ELLIOTT W. B., SLATER E. C. The nature and mechanism of action of uncoupling agents present in mitochrome preparations. Biochim Biophys Acta. 1960 Apr 8;39:267–276. doi: 10.1016/0006-3002(60)90163-3. [DOI] [PubMed] [Google Scholar]
- Hanstein W. G. Uncoupling of oxidative phosphorylation. Biochim Biophys Acta. 1976 Sep 27;456(2):129–148. doi: 10.1016/0304-4173(76)90010-0. [DOI] [PubMed] [Google Scholar]
- Haslam J. M., Fellows N. F. The role of unsaturated fatty acids in mitochondrial membrane functions. Biochem Soc Trans. 1975;3(5):772–775. doi: 10.1042/bst0030772. [DOI] [PubMed] [Google Scholar]
- Haslam J. M., Perkins M., Linnane A. W. Biogenesis of mitochondria. A requirement for mitochondrial protein synthesis for the formation of a normal adenine nucleotide transporter in yeast mitochondria. Biochem J. 1973 Aug;134(4):935–947. doi: 10.1042/bj1340935. [DOI] [PMC free article] [PubMed] [Google Scholar]
- Haslam J. M., Proudlock J. W., Linnane A. W. Biogenesis of mitochondria. 20. The effects of altered membrane lipid composition on mitochondrial oxidative phosphorylation in Saccharomyces cerevisiae. J Bioenerg. 1971 Dec;2(5):351–370. doi: 10.1007/BF01963830. [DOI] [PubMed] [Google Scholar]
- Haslam J. M., Spithill T. W., Linnane A. W., Chappell J. B. Biogenesis of mitochondria. The effects of altered membrane lipid composition on cation transport by mitochondria of Saccharomyces cerevisiae. Biochem J. 1973 Aug;134(4):949–957. doi: 10.1042/bj1340949. [DOI] [PMC free article] [PubMed] [Google Scholar]
- Haslam J. Energy-linked reactions in mitochondria: manometric errors in the determination of P-o ratios. Biochim Biophys Acta. 1965 Jul 29;105(1):184–187. doi: 10.1016/s0926-6593(65)80187-4. [DOI] [PubMed] [Google Scholar]
- Jollow D., Kellerman G. M., Linnane A. W. The biogenesis of mitochondria. 3. The lipid composition of aerobically and anaerobically grown Saccharomyces cerevisiae as related to the membrane systems of the cells. J Cell Biol. 1968 May;37(2):221–230. doi: 10.1083/jcb.37.2.221. [DOI] [PMC free article] [PubMed] [Google Scholar]
- Kormancíkov'A V., Kovác L., Vidová M. Oxidative phosphorylation in yeast. V. Phosphorylation efficiencies in growing cells determined from molar growth yields. Biochim Biophys Acta. 1969 May;180(1):9–17. doi: 10.1016/0005-2728(69)90188-1. [DOI] [PubMed] [Google Scholar]
- LOWRY O. H., ROSEBROUGH N. J., FARR A. L., RANDALL R. J. Protein measurement with the Folin phenol reagent. J Biol Chem. 1951 Nov;193(1):265–275. [PubMed] [Google Scholar]
- Lamb A. J., Clark-Walker G. D., Linnane A. W. The biogenesis of mitochondria. 4. The differentiation of mitochondrial and cytoplasmic protein synthesizing systems in vitro by antibiotics. Biochim Biophys Acta. 1968 Jul 23;161(2):415–427. [PubMed] [Google Scholar]
- Lelievre J., Rich G. T. The permeability of lipid membranes to non-electrolytes. Biochim Biophys Acta. 1973 Feb 27;298(1):15–26. doi: 10.1016/0005-2736(73)90004-7. [DOI] [PubMed] [Google Scholar]
- Liberman E. A., Topaly V. P. Selective transport of ions through bimolecular phospholipid membranes. Biochim Biophys Acta. 1968 Sep 17;163(2):125–136. doi: 10.1016/0005-2736(68)90089-8. [DOI] [PubMed] [Google Scholar]
- Mackler B., Haynes B. Studies of oxidation phosphorylation in Saccharomyces cerevisiae and Saccharomyces carlsbergensis. Biochim Biophys Acta. 1973 Jan 18;292(1):88–91. doi: 10.1016/0005-2728(73)90253-3. [DOI] [PubMed] [Google Scholar]
- Marzuki S., Cobon G. S., Haslam J. M., Linnane A. W. Biogenesis of mitochondria. The effects of altered steady-state membrane lipid composition on mitochondrial-energy metabolism in Saccharomyces cerevisiae. Arch Biochem Biophys. 1975 Aug;169(2):577–590. doi: 10.1016/0003-9861(75)90202-7. [DOI] [PubMed] [Google Scholar]
- McLaughlin S. G., Szabo G., Eisenman G., Ciani S. M. Surface charge and the conductance of phospholipid membranes. Proc Natl Acad Sci U S A. 1970 Nov;67(3):1268–1275. doi: 10.1073/pnas.67.3.1268. [DOI] [PMC free article] [PubMed] [Google Scholar]
- Mcelhaney R. N., de Gier J., van der Neut-Kok E. C. The effect of alterations in fatty acid composition and cholesterol content on the nonelectrolyte permeability of Acholeplasma laidlawii B cells and derived liposomes. Biochim Biophys Acta. 1973 Mar 16;298(2):500–512. doi: 10.1016/0005-2736(73)90376-3. [DOI] [PubMed] [Google Scholar]
- Mitchell P. A chemiosmotic molecular mechanism for proton-translocating adenosine triphosphatases. FEBS Lett. 1974 Jul 15;43(2):189–194. doi: 10.1016/0014-5793(74)80997-x. [DOI] [PubMed] [Google Scholar]
- Mitchell P. Hypothesis: cation-translocating adenosine triphosphatase models: how direct is the participation of adenosine triphosphate and its hydrolysis products in cation translocation? FEBS Lett. 1973 Jul 15;33(3):267–274. doi: 10.1016/0014-5793(73)80209-1. [DOI] [PubMed] [Google Scholar]
- Mitchell P., Moyle J. Acid-base titration across the membrane system of rat-liver mitochondria. Catalysis by uncouplers. Biochem J. 1967 Aug;104(2):588–600. doi: 10.1042/bj1040588. [DOI] [PMC free article] [PubMed] [Google Scholar]
- Onishi T. Induction of the site I phosphorylation in vivo in Saccharomyces carlsbergensis. Biochem Biophys Res Commun. 1970 Oct 23;41(2):344–352. doi: 10.1016/0006-291x(70)90510-3. [DOI] [PubMed] [Google Scholar]
- Papa S. Proton translocation reactions in the respiratory chains. Biochim Biophys Acta. 1976 Apr 30;456(1):39–84. doi: 10.1016/0304-4173(76)90008-2. [DOI] [PubMed] [Google Scholar]
- Proudlock J. W., Haslam J. M., Linnane A. W. Biogenesis of mitochondria. 19. The effects of unsaturated fatty acid depletion on the lipid composition and energy metabolism of a fatty acid desaturase mutant of Saccharomyces cerevisiae. J Bioenerg. 1971 Dec;2(5):327–349. doi: 10.1007/BF01963829. [DOI] [PubMed] [Google Scholar]
- Rottenberg H., Caplan S. R., Essig A. Soichiometry and coupling: theories of oxidative phosphorylation. Nature. 1967 Nov 11;216(5115):610–611. doi: 10.1038/216610a0. [DOI] [PubMed] [Google Scholar]
- Schatz G., Racker E. Stable phosphorylating submitochondrial particles from baker's yeast. Biochem Biophys Res Commun. 1966 Mar 8;22(5):579–584. doi: 10.1016/0006-291x(66)90314-7. [DOI] [PubMed] [Google Scholar]
- Stouthamer A. H., Bettenhaussen C. Utilization of energy for growth and maintenance in continuous and batch cultures of microorganisms. A reevaluation of the method for the determination of ATP production by measuring molar growth yields. Biochim Biophys Acta. 1973 Feb 12;301(1):53–70. doi: 10.1016/0304-4173(73)90012-8. [DOI] [PubMed] [Google Scholar]
- Tzagoloff A., Rubin M. S., Sierra M. F. Biosynthesis of mitochondrial enzymes. Biochim Biophys Acta. 1973 Feb 12;301(1):71–104. doi: 10.1016/0304-4173(73)90013-x. [DOI] [PubMed] [Google Scholar]
- VITOLS E., LINNANE A. W. Studies on the oxidative metabolism of Saccharomyces cerevisiae. II. Morphology and oxidative phosphorylation capacity of mitochondria and derived particles from baker's yeast. J Biophys Biochem Cytol. 1961 Mar;9:701–710. doi: 10.1083/jcb.9.3.701. [DOI] [PMC free article] [PubMed] [Google Scholar]
- de Gier J., Mandersloot J. G., van Deenen L. L. Lipid composition and permeability of liposomes. Biochim Biophys Acta. 1968 Jun 11;150(4):666–675. doi: 10.1016/0005-2736(68)90056-4. [DOI] [PubMed] [Google Scholar]