Abstract
1. Either l-[4,5-3H]leucine or [Me-3H]choline, or both l-[U-14C]leucine and [Me-3H]-choline, were injected into the ninth dorsal root ganglion of the frog, and peripheral transport of labelled proteins and/or phospholipids, mostly phosphatidylcholine, was studied by analysis of consecutive segments of the sciatic nerve. 2. At 25°C, approx. 5% of the 3H-labelled protein was transported at the rate of 152mm/day. The rate was temperature-dependent with the Q10 value of 2.6. The flow was completely blocked by the local application of colchicine, but was unaffected by cytochalasin D. 3. [Me-3H]-Choline was incorporated into phosphatidylcholine at a comparatively slow rate, but was transported in the nerve at a rate equivalent to that for 3H-labelled proteins. 4. The simultaneous transport of phosphatidylcholine and the protein was further supported in the double-labelling experiments by an identical transport rate of 3H-labelled phosphatidylcholine and 14C-labelled proteins, by their identical temperature dependence, by simultaneous blockade with colchicine, and also by the parallel distribution of the two labels in subcellular fractions. Specific radioactivities on a protein basis of both 3H and 14C labels were highest in microsomal subfractions enriched with Na+-plus-K+-stimulated adenosine triphosphatase and acetylcholinesterase. It is suggested that 3H-labelled phosphatidylcholine and 14C-labelled proteins transported in the nerve reside in the same structural entity, most probably a membrane component.
Full text
PDF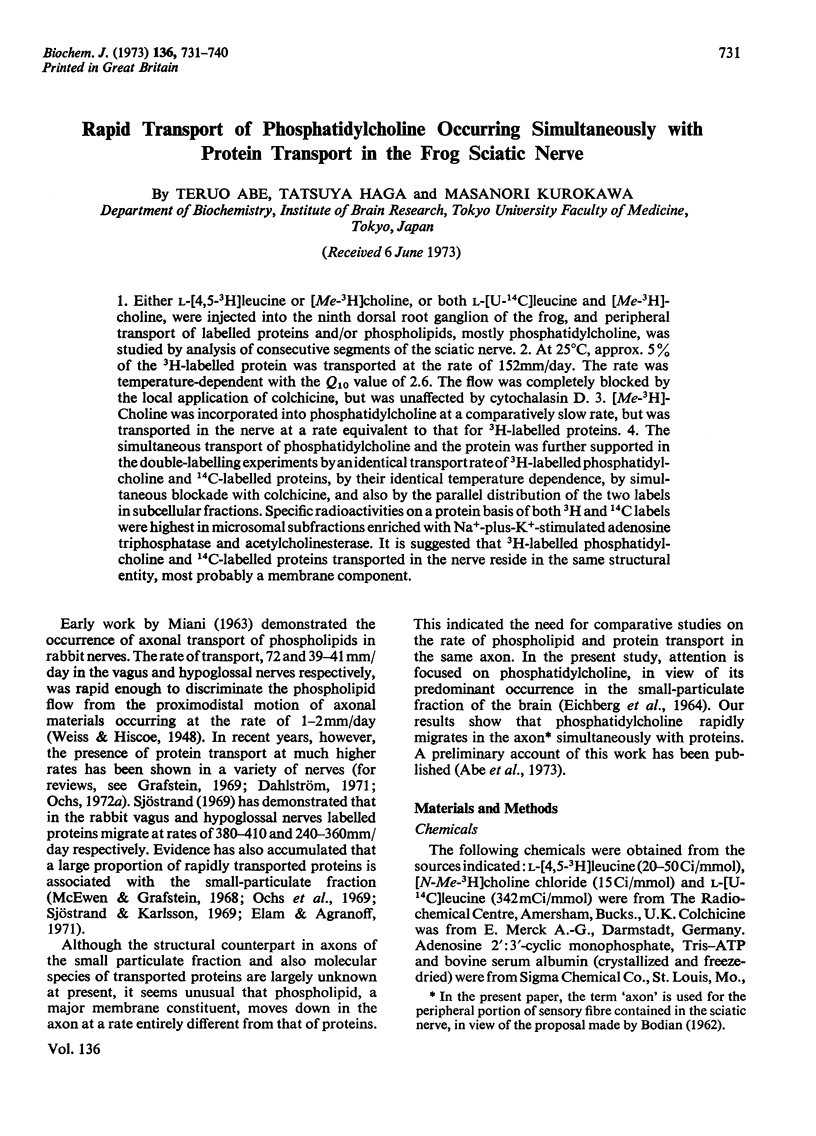
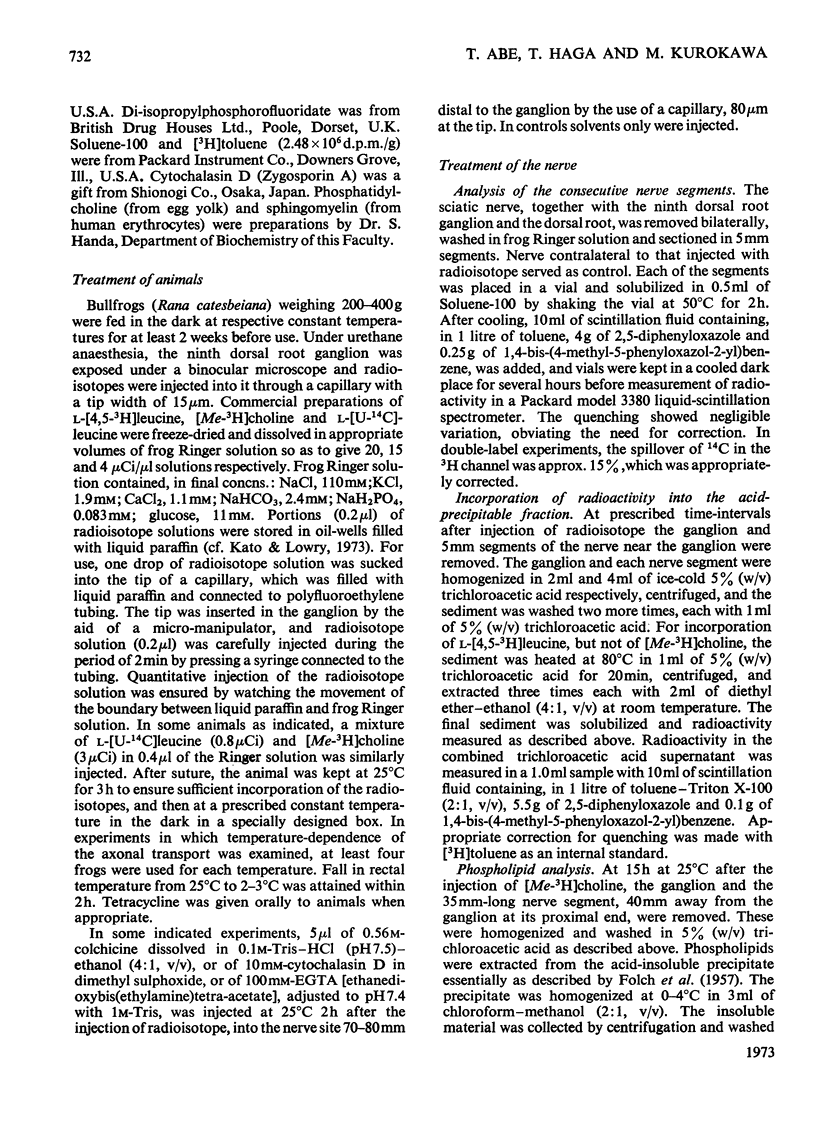
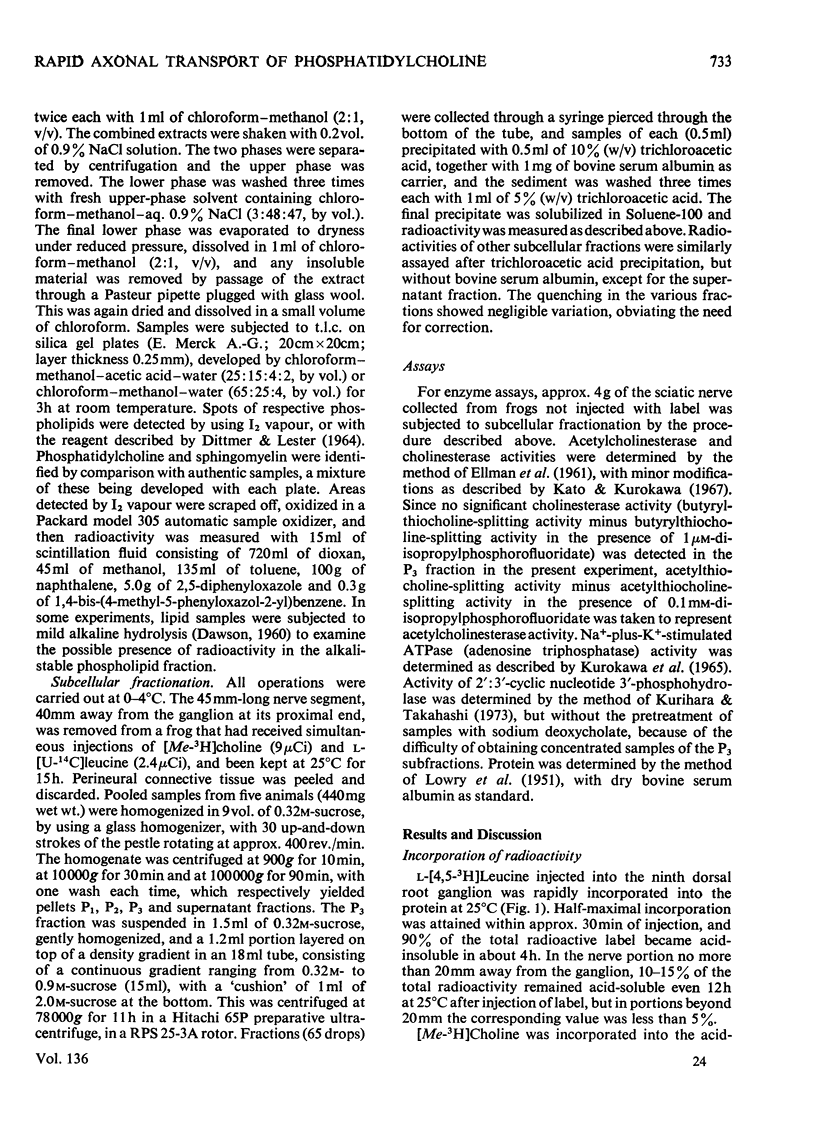
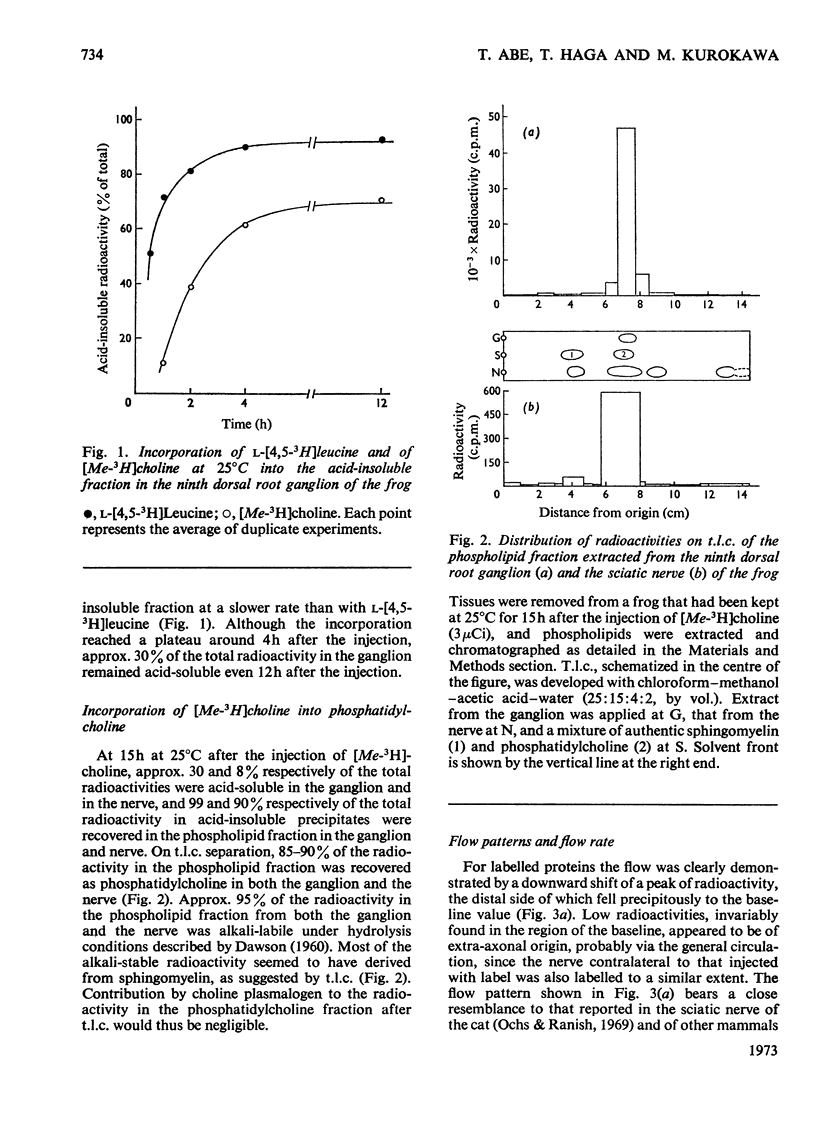
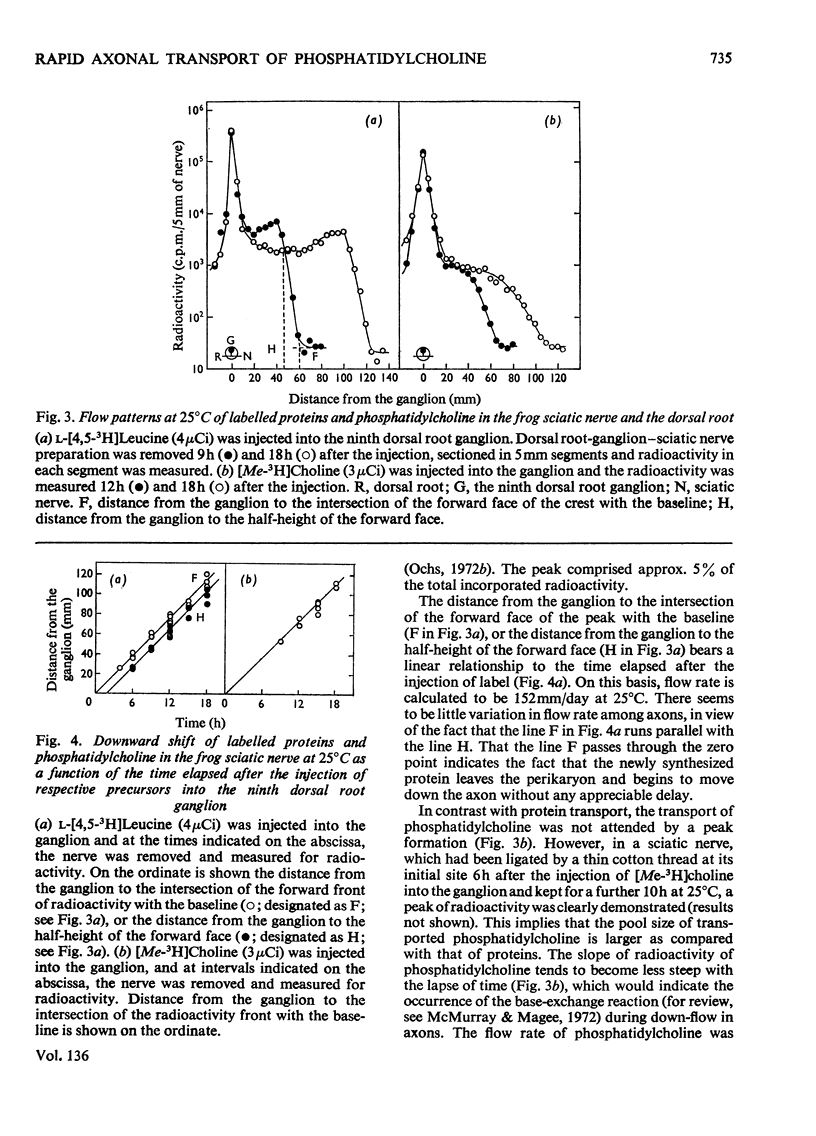
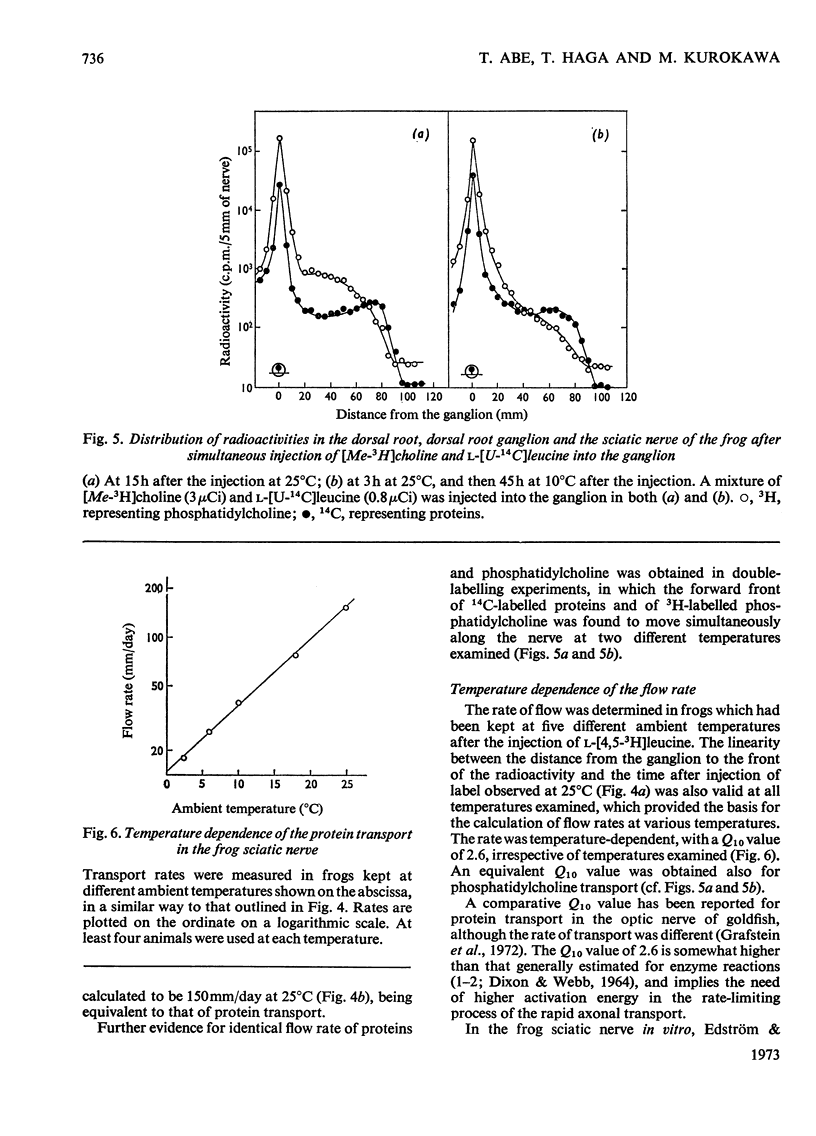
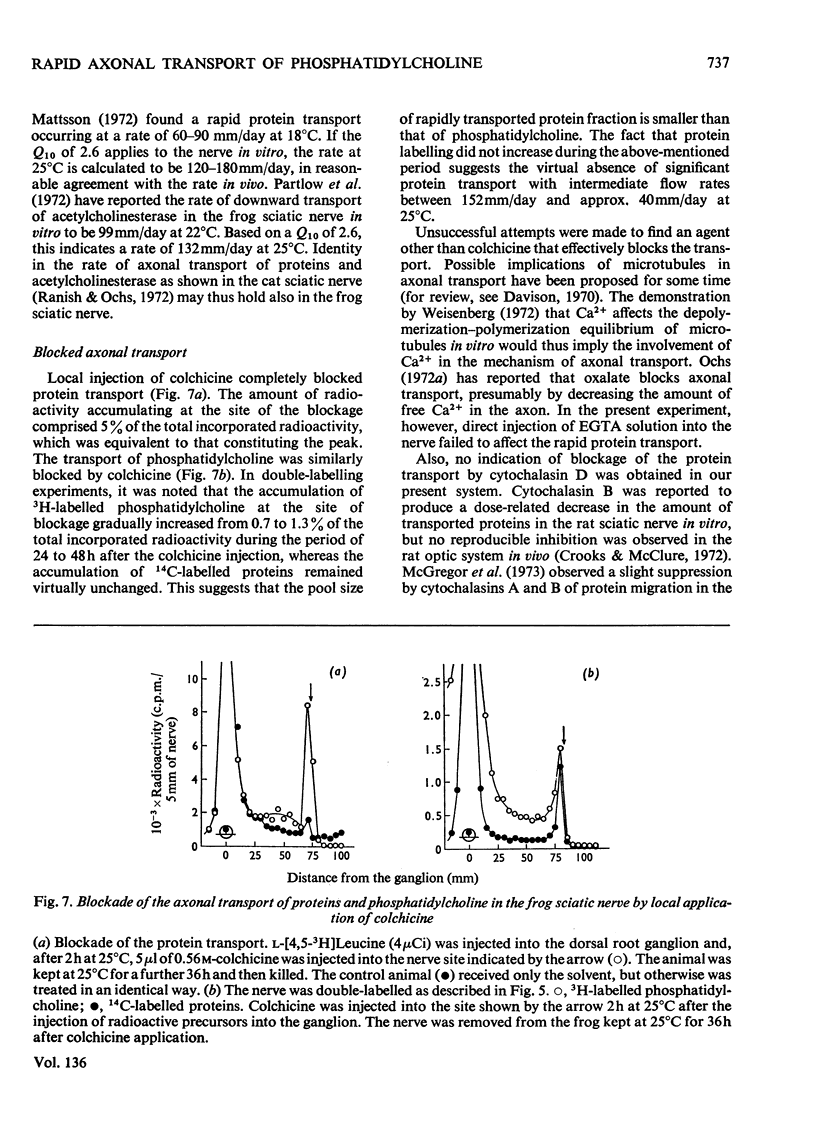
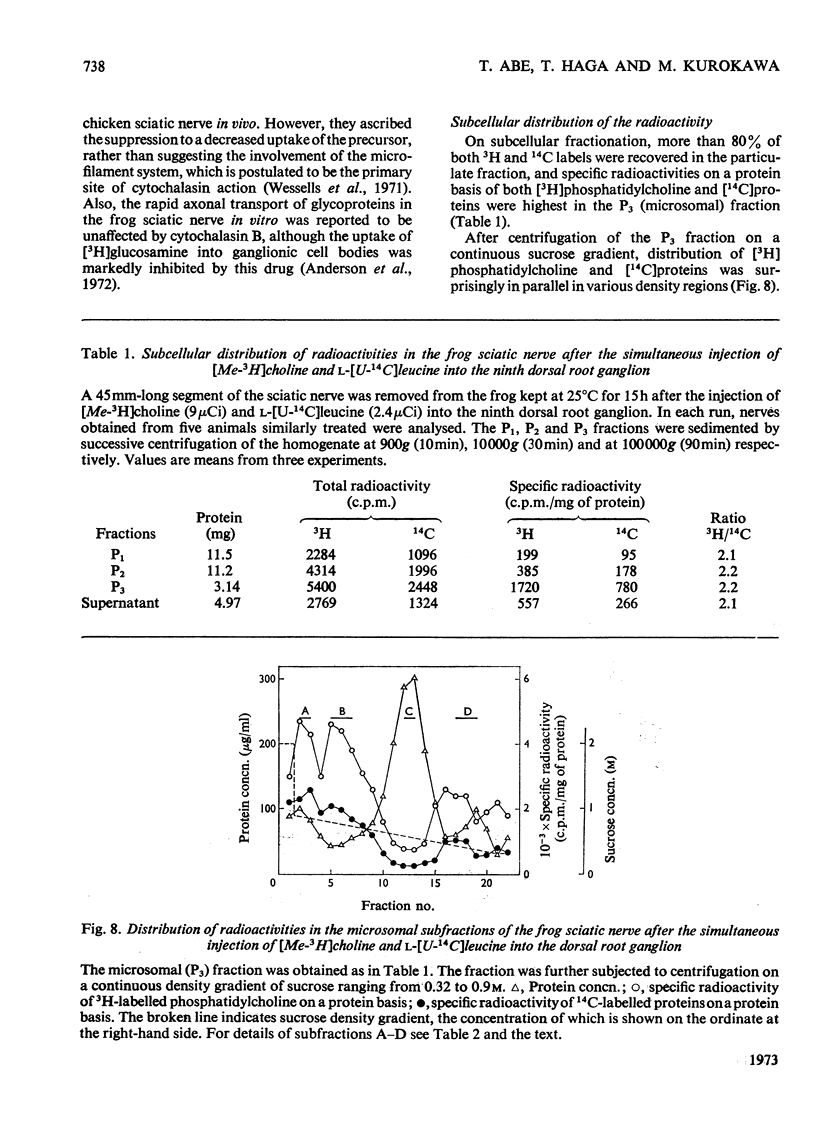
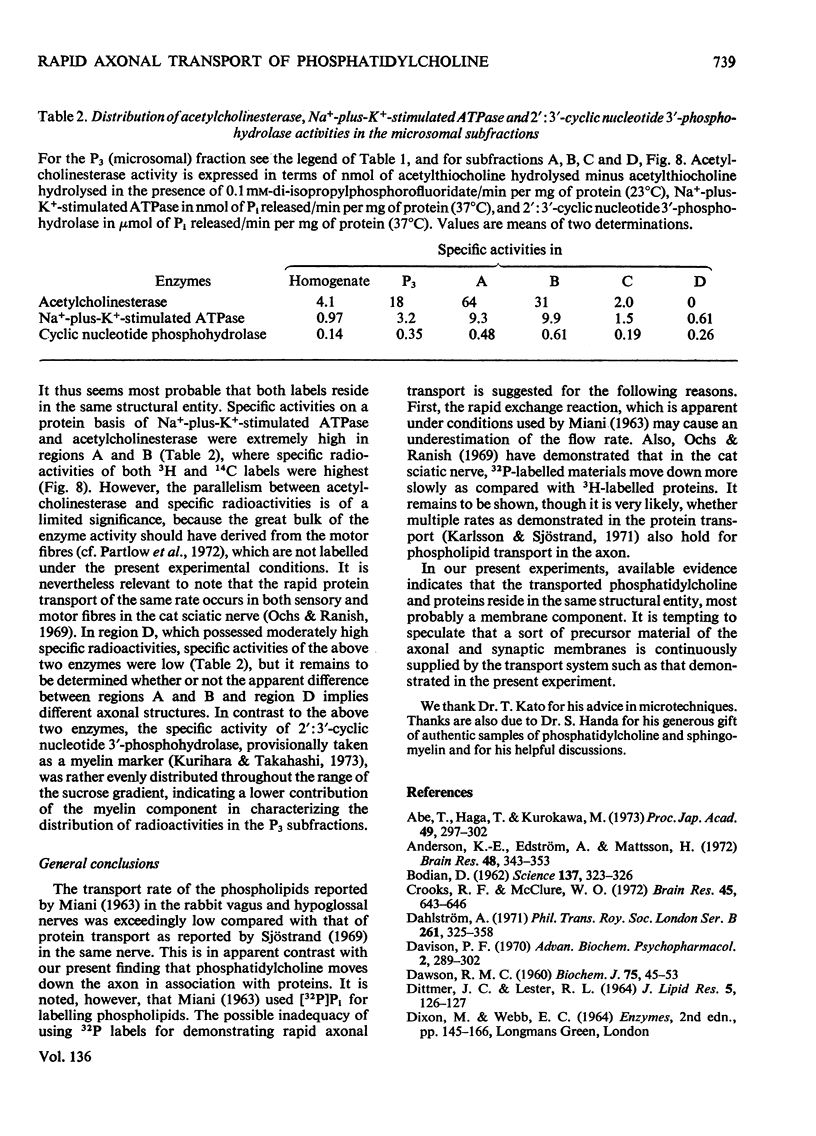
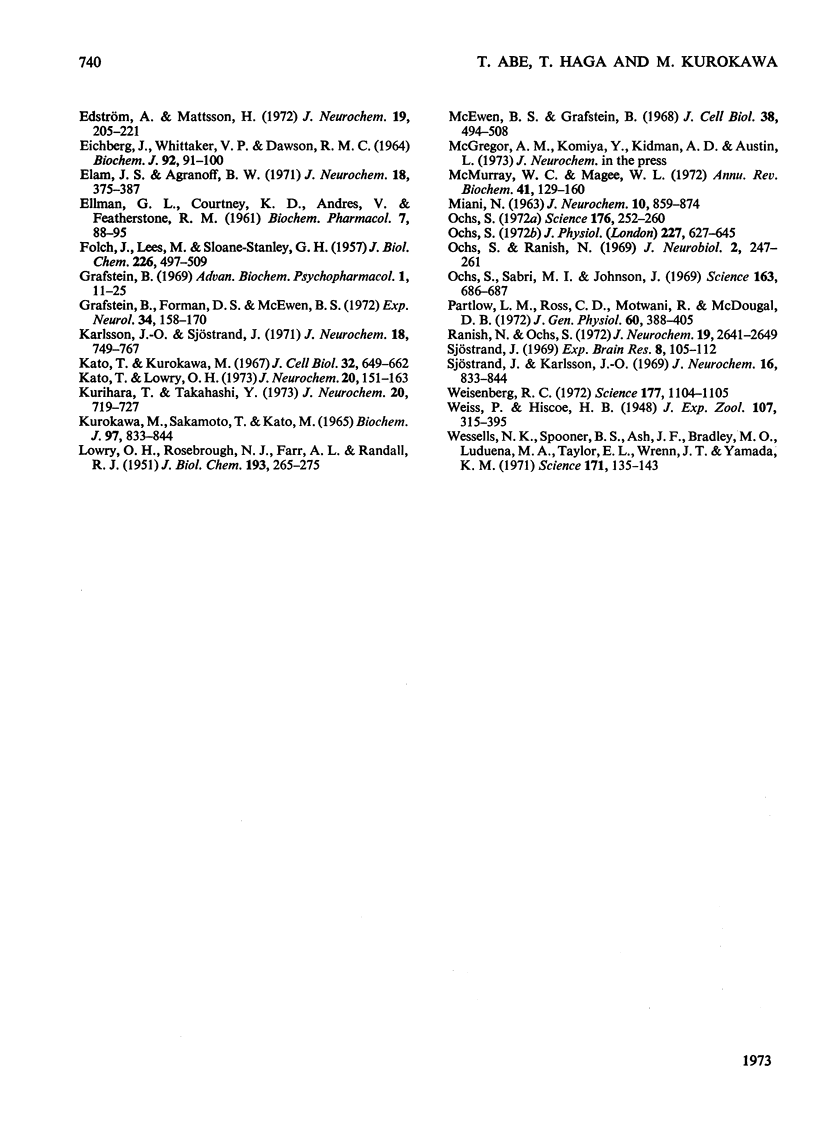
Selected References
These references are in PubMed. This may not be the complete list of references from this article.
- Anderson K. E., Edström A., Mattsson H. Effects of cytochalasin B on uptake of glucosamine, leucine and sulphate into nerve cells; incorporation into glycoproteins and rapid axonal transport. Brain Res. 1972 Dec 24;48:343–353. doi: 10.1016/0006-8993(72)90188-6. [DOI] [PubMed] [Google Scholar]
- BODIAN D. The generalized vertebrate neuron. Science. 1962 Aug 3;137(3527):323–326. doi: 10.1126/science.137.3527.323. [DOI] [PubMed] [Google Scholar]
- Crooks R. F., McClure W. O. The effect of cytocholasin B on fast axoplasmic transport. Brain Res. 1972 Oct 27;45(2):643–646. doi: 10.1016/0006-8993(72)90499-4. [DOI] [PubMed] [Google Scholar]
- DAWSON R. M. A hydrolytic procedure for the identification and estimation of individual phospholipids in biological samples. Biochem J. 1960 Apr;75:45–53. doi: 10.1042/bj0750045. [DOI] [PMC free article] [PubMed] [Google Scholar]
- DITTMER J. C., LESTER R. L. A SIMPLE, SPECIFIC SPRAY FOR THE DETECTION OF PHOSPHOLIPIDS ON THIN-LAYER CHROMATOGRAMS. J Lipid Res. 1964 Jan;5:126–127. [PubMed] [Google Scholar]
- Davison P. F. Microtubules and neurofilaments: possible implications in axoplasmic transport. Adv Biochem Psychopharmacol. 1970;2:289–302. [PubMed] [Google Scholar]
- ELLMAN G. L., COURTNEY K. D., ANDRES V., Jr, FEATHER-STONE R. M. A new and rapid colorimetric determination of acetylcholinesterase activity. Biochem Pharmacol. 1961 Jul;7:88–95. doi: 10.1016/0006-2952(61)90145-9. [DOI] [PubMed] [Google Scholar]
- Edstrom A., Mattsson H. Fast axonal transport in vitro in the sciatic system of the frog. J Neurochem. 1972 Jan;19(1):205–221. doi: 10.1111/j.1471-4159.1972.tb01270.x. [DOI] [PubMed] [Google Scholar]
- Eichberg J., Whittaker V. P., Dawson R. M. Distribution of lipids in subcellular particles of guinea-pig brain. Biochem J. 1964 Jul;92(1):91–100. doi: 10.1042/bj0920091. [DOI] [PMC free article] [PubMed] [Google Scholar]
- Elam J. S., Agranoff B. W. Rapid transport of protein in the optic system of the goldfish. J Neurochem. 1971 Mar;18(3):375–387. doi: 10.1111/j.1471-4159.1971.tb11965.x. [DOI] [PubMed] [Google Scholar]
- FOLCH J., LEES M., SLOANE STANLEY G. H. A simple method for the isolation and purification of total lipides from animal tissues. J Biol Chem. 1957 May;226(1):497–509. [PubMed] [Google Scholar]
- Grafstein B. Axonal transport: communication between soma and synapse. Adv Biochem Psychopharmacol. 1969;1:11–25. [PubMed] [Google Scholar]
- Grafstein B., Forman D. S., McEwen B. S. Effects of temperature on axonal transport and turnover of protein in goldfish optic system. Exp Neurol. 1972 Jan;34(1):158–170. doi: 10.1016/0014-4886(72)90196-3. [DOI] [PubMed] [Google Scholar]
- Karlsson J. O., Sjöstrand J. Synthesis, migration and turnover of protein in retinal ganglion cells. J Neurochem. 1971 May;18(5):749–767. doi: 10.1111/j.1471-4159.1971.tb12005.x. [DOI] [PubMed] [Google Scholar]
- Kato T., Kurokawa M. Isolation of cell nuclei from the mammalian cerebral cortex and their assortment on a morphological basis. J Cell Biol. 1967 Mar;32(3):649–662. doi: 10.1083/jcb.32.3.649. [DOI] [PMC free article] [PubMed] [Google Scholar]
- Kato T., Lowry O. H. Enzymes of energy-converting systems in individual mammalian nerve cell bodies. J Neurochem. 1973 Jan;20(1):151–163. doi: 10.1111/j.1471-4159.1973.tb12112.x. [DOI] [PubMed] [Google Scholar]
- Kurihara T., Takahashi Y. Potentiometric and colorimetric methods for the assay of 2',3'-cyclic nucleotide 3'-phosphohydrolase. J Neurochem. 1973 Mar;20(3):719–727. doi: 10.1111/j.1471-4159.1973.tb00032.x. [DOI] [PubMed] [Google Scholar]
- Kurokawa M., Kato M., Sakamoto T. Distribution of sodium-plus-potassium-stimulated adenosine-triphosphatase activity in isolated nerve-ending particles. Biochem J. 1965 Dec;97(3):833–844. doi: 10.1042/bj0970833. [DOI] [PMC free article] [PubMed] [Google Scholar]
- LOWRY O. H., ROSEBROUGH N. J., FARR A. L., RANDALL R. J. Protein measurement with the Folin phenol reagent. J Biol Chem. 1951 Nov;193(1):265–275. [PubMed] [Google Scholar]
- MIANI N. ANALYSIS OF THE SOMATO-AXONAL MOVEMENT OF PHOSPHOLIPIDS IN THE VAGUS AND HYPOGLOSSAL NERVES. J Neurochem. 1963 Dec;10:859–874. doi: 10.1111/j.1471-4159.1963.tb11913.x. [DOI] [PubMed] [Google Scholar]
- McEwen B. S., Grafstein B. Fast and slow components in axonal transport of protein. J Cell Biol. 1968 Sep;38(3):494–508. doi: 10.1083/jcb.38.3.494. [DOI] [PMC free article] [PubMed] [Google Scholar]
- McMurray W. C., Magee W. L. Phospholipid metabolism. Annu Rev Biochem. 1972;41(10):129–160. doi: 10.1146/annurev.bi.41.070172.001021. [DOI] [PubMed] [Google Scholar]
- Ochs S. Fast transport of materials in mammalian nerve fibers. Science. 1972 Apr 21;176(4032):252–260. doi: 10.1126/science.176.4032.252. [DOI] [PubMed] [Google Scholar]
- Ochs S., Ranish N. Characteristics of the fast transport system in mammalian nerve fibers. J Neurobiol. 1969;1(2):247–261. doi: 10.1002/neu.480010211. [DOI] [PubMed] [Google Scholar]
- Ochs S. Rate of fast axoplasmic transport in mammalian nerve fibres. J Physiol. 1972 Dec;227(3):627–645. doi: 10.1113/jphysiol.1972.sp010051. [DOI] [PMC free article] [PubMed] [Google Scholar]
- Ochs S., Sabri M. I., Johnson J. Fast transport system of materials in mammalian nerve fibers. Science. 1969 Feb 14;163(3868):686–687. doi: 10.1126/science.163.3868.686. [DOI] [PubMed] [Google Scholar]
- Partlow L. M., Ross C. D., Motwani R., McDougal D. B., Jr Transport of axonal enzymes in surviving segments of frog sciatic nerve. J Gen Physiol. 1972 Oct;60(4):388–405. doi: 10.1085/jgp.60.4.388. [DOI] [PMC free article] [PubMed] [Google Scholar]
- Ranish N., Ochs S. Fast axoplasmic transport of acetylcholinesterase in mammalian nerve fibres. J Neurochem. 1972 Nov;19(11):2641–2649. doi: 10.1111/j.1471-4159.1972.tb01323.x. [DOI] [PubMed] [Google Scholar]
- Sjöstrand J., Karlsson J. O. Axoplasmic transport in the optic nerve and tract of the rabbit: a biochemical and radioautographic study. J Neurochem. 1969 Jun;16(3):833–844. doi: 10.1111/j.1471-4159.1969.tb08971.x. [DOI] [PubMed] [Google Scholar]
- WEISS P., HISCOE H. B. Experiments on the mechanism of nerve growth. J Exp Zool. 1948 Apr;107(3):315–395. doi: 10.1002/jez.1401070302. [DOI] [PubMed] [Google Scholar]
- Weisenberg R. C. Microtubule formation in vitro in solutions containing low calcium concentrations. Science. 1972 Sep 22;177(4054):1104–1105. doi: 10.1126/science.177.4054.1104. [DOI] [PubMed] [Google Scholar]
- Wessells N. K., Spooner B. S., Ash J. F., Bradley M. O., Luduena M. A., Taylor E. L., Wrenn J. T., Yamada K. Microfilaments in cellular and developmental processes. Science. 1971 Jan 15;171(3967):135–143. doi: 10.1126/science.171.3967.135. [DOI] [PubMed] [Google Scholar]